-
PDF
- Split View
-
Views
-
Cite
Cite
Qi Li, Yize Wang, Wei Zheng, Jing Guo, Shunji Zhang, Fei Gong, Guang-Xiu Lu, Ge Lin, Jing Dai, Biallelic variants in IQCN cause sperm flagellar assembly defects and male infertility, Human Reproduction, Volume 38, Issue 7, July 2023, Pages 1390–1398, https://doi.org/10.1093/humrep/dead079
- Share Icon Share
Abstract
What is the effect of defects in the manchette protein IQ motif-containing N (IQCN) on sperm flagellar assembly?
Deficiency in IQCN causes sperm flagellar assembly defects and male infertility.
The manchette is a transient structure that is involved in the shaping of the human spermatid nucleus and protein transport within flagella. Our group recently reported that the manchette protein IQCN is essential for fertilization. Variants in IQCN lead to total fertilization failure and defective acrosome structure phenotypes. However, the function of IQCN in sperm flagellar assembly is still unknown.
Fifty men with infertility were recruited from a university-affiliated center from January 2014 to October 2022.
Genomic DNA was extracted from the peripheral blood samples of all 50 individuals for whole-exome sequencing. The ultrastructure of the spermatozoa was assessed by transmission electron microscopy. Computer-assisted sperm analysis (CASA) was used to test the parameters of curvilinear velocity (VCL), straight-line velocity (VSL), and average path velocity (VAP). An Iqcn knockout (Iqcn−/−) mouse model was generated by CRISPR–Cas9 technology to evaluate sperm motility and the ultrastructure of the flagellum. Hyperactivation and sperm fertilizing ability were assessed in a mouse model. Immunoprecipitation followed by liquid chromatography–mass spectrometry was used to detect IQCN-binding proteins. Immunofluorescence was used to validate the localization of IQCN-binding proteins.
Biallelic variants in IQCN (c.3913A>T and c.3040A>G; c.2453_2454del) were identified in our cohort of infertile men. The sperm from the affected individuals showed an irregular ‘9 + 2’ structure of the flagellum, which resulted in abnormal CASA parameters. Similar phenotypes were observed in Iqcn−/− male mice. VSL, VCL, and VAP in the sperm of Iqcn−/− male mice were significantly lower than those in Iqcn+/+ male mice. Partial peripheral doublet microtubules (DMTs) and outer dense fibers (ODFs) were absent, or a chaotic arrangement of DMTs was observed in the principal piece and end piece of the sperm flagellum. Hyperactivation and IVF ability were impaired in Iqcn−/− male mice. In addition, we investigated the causes of motility defects and identified IQCN-binding proteins including CDC42 and the intraflagellar transport protein families that regulate flagellar assembly during spermiogenesis.
More cases are needed to demonstrate the relation between IQCN variants and phenotypes.
Our findings expand the genetic and phenotypic spectrum of IQCN variants in causing male infertility, providing a genetic marker for sperm motility deficiency and male infertility.
This work was supported by the National Natural Science Foundation of China (81974230 and 82202053), the Changsha Municipal Natural Science Foundation (kq2202072), the Hunan Provincial Natural Science Foundation (2022JJ40658), and the Scientific Research Foundation of Reproductive and Genetic Hospital of CITIC-Xiangya (YNXM-202114 and YNXM-202201). No conflicts of interest were declared.
N/A.
Introduction
Male infertility is a major global health problem, affecting approximately 50% of infertile couples (Agarwal et al., 2021). Asthenozoospermia, a common cause of male infertility, is characterized by attenuated sperm motility (Jiao et al., 2021). During spermiogenesis, the maturation of spermatids into sperm cells involves the formation of acrosomes, chromatin condensation and head shaping, and sperm flagellar assembly (Teves et al., 2020). Flagellar assembly is crucial for normal sperm motility and male fertility.
The flagellum is an important organelle that comprises a ‘9 + 2’ structure with a bundle of nine peripheral doublet microtubules (DMTs) that surround a pair of central singlets (Loreng and Smith, 2017). More than 700 proteins have been identified in human sperm flagella (Baker et al., 2013), which highlights the complexity of an etiological study on sperm flagella deficiencies. The genetic causes of asthenozoospermia have been investigated using whole-exome sequencing (WES), (Jiao et al., 2021). Some genes associated with sperm flagellar assembly have been identified, including the coiled-coil domain-containing (CCDC) family, the dynein axonemal heavy chain (DNAH) family, and the A kinase-anchoring protein (AKAP) family (Tu et al., 2021; Zhang et al., 2022; Liu et al., 2023). Defects in these genes mainly affect the assembly of the dynein arm heavy chain or dynein regulator complex (Coutton et al., 2015). However, novel pathogenic mechanisms or novel genes that participate in sperm flagellar assembly during spermiogenesis need to be investigated further.
Most recently, the IQ motif containing N (IQCN) protein was identified by our group, and its function in manchette assembly and fertilization was demonstrated (Dai et al., 2022). Variations in IQCN lead to abnormalities of acrosomal structure, resulting in oocyte activation deficiency and fertilization failure (Dai et al., 2022). The manchette is a transient structure that is involved in shaping of the spermatid nucleus and protein transport within the flagellum (Kierszenbaum, 2002). However, the role of IQCN in sperm flagellar assembly during spermiogenesis remains unknown.
Here, we report that biallelic variants in IQCN are associated with abnormal flagellar assembly. Similar phenotypes were also observed in Iqcn−/− male mice. In addition, we found a decreased interaction between IQCN and the hub protein CDC42 and the intraflagellar transport (IFT) protein family in the testes of Iqcn−/− mice, which led to defects in sperm flagellar assembly and motility. In conclusion, our results shed light on the mechanism of action of IQCN in flagellar assembly, further expanding the phenotypic and genetic spectrum of IQCN and providing novel diagnostic indicators for sperm motility deficiency and male infertility.
Materials and methods
Clinical subjects
We recruited 50 men with infertility with total fertilization failure (TFF) from January 2014 to October 2022 from the Reproductive and Genetic Hospital of China International Trust Investment Corporation (CITIC)-Xiangya. The human study was approved by the Ethics Committee of the Reproductive and Genetic Hospital of CITIC-Xiangya (LL-SC-2017-009). Written informed consent was obtained from all subjects who participated in the study. The inclusion criteria were as follows: men with primary infertility who had undergone at least one ICSI attempt; more than five mature oocytes retrieved in a single attempt; fertilization rate <20%; and sperm-borne TFF based on the mouse oocyte activation test, as described previously (Heindryckx et al., 2005, 2008). The exclusion criterion was globozoospermia.
Sequencing, variant screening, and validation
Genomic DNA (gDNA) was extracted from the peripheral blood of 50 males with infertility in our cohort and subjected to WES. The variants were filtered using the following inclusion criteria: variations with minor allele frequencies <1% in the genome aggregation database (gnomAD) and Exome Aggregation Consortium (ExAC) database; exonic nonsynonymous or splice site variants encoding indels; and specifically expressed or highly expressed in the testis. The variants were validated by Sanger sequencing with the specific primers listed in Supplementary Table S1. Protein domains were determined by the Simple Modular Architecture Research Tool (SMART).
Assessment of human sperm motility
Three men with variants in IQCN donated semen samples for research: two males identified in this study (Patient 1: c.3913A>T and c.3040A>G; Patient 2: c.2453_2454del) and one male identified in a previous study (Patient 3: c.910C>T) (Dai et al., 2022). Human semen samples were collected following 2–7 days of sexual abstinence. After liquefaction, the motility parameters curvilinear velocity (VCL), straight-line velocity (VSL), and average path velocity (VAP) were analyzed using a computer-assisted sperm analysis (CASA) system (SAS Medical, SAS-II, Beijing, China). The concentration of sperm was adjusted to 5–25×106/ml. Five microliters of semen was placed into the slide chamber (SAS medical, SC-IV, Beijing, China). The CASA parameters were assessed under a negative phase-contrast objective (10×) at a final magnification of 100×. At least 200 motile sperm were assessed.
The mean±SD values of sperm motility parameters from 1876 males in the hospital during routine semen examination were used as reference CASA values. The inclusion criteria for males were as follows: males with proven fertility who underwent ART owing to female factors; sperm volume ≥1.5 ml; sperm concentration ≥15×106/ml; total motility ≥40%; progressive motility ≥32%; total sperm count >39×106; and aged ≤40 years.
Transmission electron microscopy
Human and mouse sperm were analyzed using transmission electron microscopy (TEM), as previously described (Dai et al., 2021). Briefly, the sperm were fixed with 2.5% glutaraldehyde (Sigma–Aldrich, G5882, St. Louis, MO, USA) at 4°C overnight. The samples were postfixed with 1% OsO4 and dehydrated, followed by infiltration by 100% acetone and Epon resin mixture (1:1). Ultrathin sections were stained with uranyl acetate and lead citrate. The ultrastructure of each part of the sperm flagellum was observed and photographed via a G2 Spirit TWIN transmission electron microscope (FEI, Tecnai, Czech Republic) with a GatanCCD camera (Gatan, Orius, Pleasanton, CA, USA) system.
Mouse model
Iqcn knockout mice were generated by CRISPR/Cas9-mediated genome editing, as previously described (Dai et al., 2022). Briefly, guide RNAs (gRNAs) targeting exon 3 and exon 4 were used to generate Iqcn−/− mice. The gRNAs and Cas9 mRNA were coinjected into zygotes of C57B6J mice. Genotypes of the resulting pups were determined by Sanger sequencing. Heterozygous Iqcn knockout (Iqcn+/−) founder mice were crossed to generate Iqcn−/− mice for the experiment. This study was conducted in accordance with our facility recommendations in the Guide for the Care and Use of Laboratory Animals. The study of animal subjects was approved by the Animal Welfare Ethics Committee of Central South University (XMSB-2022-0057).
Assessment of mouse sperm motility
Mouse sperm were released from the epididymides into 1 ml of human tubal fluid (HTF; Aibei Biotechnology, M1130, Nanjing, China) medium for 15 min at 37°C. An aliquot of sperm suspension (5 μl) was loaded onto a prewarmed chamber slide and analyzed by the CASA system (SAS medical, SAS-MS). The sperm motility parameters VCL, VSL, and VAP were examined. At least 200 motile sperm were assessed. For assessment of hyperactivated motility, sperm were cultured in HTF medium for 2 h in 6% CO2 at 37°C. Hyperactivated motility was defined by distinctive rapid star spin, figure-eight, and sawtooth-circle swimming patterns (Mortimer, 2013). Manual counts are used to show the percentage of hyperactivated sperm in each group (Jin et al., 2007).
IVF
Three-week-old C57BL/6J female mice were superovulated by injecting 10 IU pregnant mare serum gonadotropin (PMSG, Solarbio, P9970, Beijing, China), followed by 10 IU hCG (Livzon, Zhuhai, China) 48 h later. The sperm from 8- to 10-week-old WT and Iqcn−/− mice were collected from the cauda epididymides into 1 ml HTF medium for 45 min at 37°C. For IVF, cumulus-intact oocytes were obtained from the oviduct of superovulated females and transferred into the HTF containing sperm. After 4–6 h incubation, the oocytes were washed in HTF drops and cultured in KSOM medium (Aibei Biotechnology, M1430, Nanjing, China) in 6% CO2, 5% O2, and 89% N2 at 37°C. The two pronuclei (2PN) rate, 2-cell embryo rate, and blastocyst rate were evaluated at 6 h, 26 h, and 96 h after insemination, respectively.
Immunoprecipitation followed by liquid chromatography–mass spectrometry
We performed immunoprecipitation (IP) on both Iqcn+/+ and Iqcn−/− mouse testes and then subjected the immunoprecipitants to liquid chromatography–mass spectrometry (LC–MS) analysis. IQCN IP was performed in mouse testes using a Pierce™ Crosslink Magnetic IP kit (Thermo Fisher, 88805, Waltham, MA, USA) as described previously, with some modifications (Yuan et al., 2015). According to the manufacturer’s instructions, rabbit polyclonal IQCN antibody (YouKe Biotechnology, Shanghai, China) was cross-linked to Protein A/G-coated magnetic beads and then incubated with Iqcn+/+ and Iqcn−/− mouse testicular tissue lysates overnight. After elution and neutralization, the eluents were used for LC–MS.
The LC–MS data were analyzed by Q Exactive™ HF-X (Thermo Fisher Scientific, Waltham, MA, USA) with a nanoelectrospray ion source in the Jingjie PTM BioLabs (Hangzhou, China). The raw data files were compared against Mus_musculus_10090_SP_20210721.fasta (17 089 entries) using the MaxQuant search engine (v.1.6.15.0) and its incorporated Andromeda search engine to identify proteins and their respective label-free quantification values using standard parameters. A 1.5-fold change (FL) between the wildtype and Iqcn−/− groups defined differentially expressed proteins (DEPs). An enrichment analysis was performed using Metascape for the Gene Ontology (GO) database against the mouse reference proteome. An adjusted P-value cutoff of 0.05 was set to determine the significantly enriched GO term. The protein–protein interaction network was predicted using the STRING database (http://string-db.org/) with the highest confidence (0.9) and was visualized in Cytoscape (Version 3.7.2, Boston, MA, USA) (degree >3).
Sperm immunofluorescence and lectin/α-tubulin staining
Sperm from humans and mice were fixed with 4% paraformaldehyde for 30 min, permeabilized with Triton X-100 (Sigma, T8787, St. Louis, MO, USA) and then blocked with 5% donkey serum albumin (Jackson ImmunoResearch, 017-000-121, Philadelphia, PA, USA). The primary rabbit polyclonal KIAA1683 (IQCN) antibody (1:200; Thermo Fisher, PA5-59972, Waltham, MA, USA), rabbit polyclonal CDC42 antibody (1:50; Proteintech, 10155-1-AP, Chicago, IL, USA), and IFT74 polyclonal antibody (1:1000; Proteintech, 27334-1-AP, Chicago, IL, USA) were incubated with the samples at 4°C overnight. The secondary antibodies were incubated with the sections for 1 h at room temperature. PNA (a marker of acrosomal outer membrane)/lectin staining was performed using FITC-conjugated lectin from Arachis hypogaea (1:100; Sigma Aldrich, L7381, St. Louis, MO, USA) and α-tubulin staining was performed using FITC-conjugated α-tubulin (1:200; Sigma Aldrich, F2168, St. Louis, MO, USA). A Cell Discoverer 7 fluorescence microscope (Zeiss, Oberkochen, Germany) and Zen 2 software (Zeiss, Oberkochen, Germany) were used to photograph the fluorescence signals.
Statistical analysis
Data are expressed as the mean ± SD. Statistical analyses were performed using SPSS 19.0 statistical software (SPSS Inc., Chicago, IL, USA). For normally distributed variables, the independent-samples Student’s t-test was used to detect significant differences between the two groups, and one-way ANOVA was used to detect differences between three or more groups. For non-normally distributed variables, the Mann–Whitney test was used to detect significant differences between two groups, and the Kruskal–Wallis test was used to detect differences between three or more groups. Statistical significance was set at P < 0.05. All data were obtained from ≥3 independent experiments.
Results
Novel biallelic variants in IQCN identified in our cohort
In our TFF cohort, in addition to two males with homozygous variants (c.910C>T and c.2453_2454del) in IQCN that were identified previously (Dai et al., 2022), we identified two other males from two independent Chinese families carrying biallelic variants in IQCN (Fig. 1A). In all, we identified four males carrying variants in IQCN out of the 50 males with infertility (4/50). Sanger sequencing validated that Patient 1 (II-1 in Family 1) carried compound heterozygous variants in IQCN (c.3913A>T and c.3040A>G). The mother (I-1 in Family 1) and fertile brother (II-2 in Family 1) of the proband carried only one heterozygous variant in IQCN (c.3040A>G). Patient 2 (II-1 in Family 2) carried a recurrent frameshift variant in IQCN (c.2453_2454del) (Fig. 1A). The IQCN variant (c.2453_2454del) in Patient 2 resulted in the loss of five IQ motifs, and the variant (c.3913A>G) in Patient 1 was located in the fifth IQ motif (Supplementary Fig. S1).

Biallelic variants in IQCN cause human sperm flagellar assembly defects. (A) Pedigree analysis of two families carrying biallelic variants in IQCN. The individuals (black squares) were probands; confirmation by Sanger sequencing is shown below the pedigrees. (B) Immunostaining of IQCN (red) and PNA (a marker of acrosomal outer membrane)/lectin staining (green) in spermatozoa from the affected individuals. (C) Representative TEM micrographs showing irregular ‘9 + 2’ structures, including disorganized arrangements and/or the absence of CPs, DMTs, and ODFs. Scale bars: 100 nm. (D) The percentages of sperm with defects in the ‘9 + 2’ structure from normal controls and Patient 1 (n = 3, each donor with three technical replicates). The data are presented as the mean±SD. ***P<0.001 by the independent-samples Student’s t-test (D). IQCN, IQ motif-containing N; TEM, transmission electron microscopy; CP, central-pair microtubule; DMT, peripheral doublet microtubule; ODF, outer dense fiber.
Biallelic variants in IQCN cause abnormal localization and defective sperm flagellar assembly
We collected semen samples from the two males identified in this study and one male identified in a previous study (Patient 3: c.910C>T) (Dai et al., 2022) to measure the effect of variants on the expression and localization of IQCN and found that IQCN was located in the acrosome of the head in normal sperm (Fig. 1B). However, the signal was absent in the spermatozoa from Patient 3, indicating that the variant led to no expression of IQCN (Fig. 1B). The signal was diffusely distributed in the acrosome of spermatozoa from Patients 1 and 2, resulting in abnormal localization of IQCN (Fig. 1B).
The acrosome marker lectin showed that the acrosome signal in these patients lost its cap-shaped structure (Fig. 1B). Regarding the spermatozoa from Patient 1, TEM revealed abnormal acrosome ultrastructure in sperm (Supplementary Fig. S2) as well as impaired sperm flagellar assembly (Fig. 1C). An irregular ‘9 + 2’ structure was detected in the principal piece (in 29.52% ± 2.50% of sperm) and end piece (47.86% ± 2.58%) of the flagella, which were significantly more common than in the principal piece (6.32% ± 0.80%) and end piece (15.32% ± 1.22%) of flagella from the normal control (Fig. 1D). In particular, some peripheral DMTs and outer dense fibers (ODFs) were missing. Some of the central-pair microtubules (CPs) were absent, causing a chaotic arrangement of DMTs (Fig. 1C).
We further analyzed the sperm motility parameters by CASA. Although the motility and progressive motility of sperm showed no significant difference from the reference limit of the World Health Organization Standards (Cooper et al., 2010) (Supplementary Table S2), the VCL, VSL, and VAP of Patients 2 and 3 were lower than the reference values of normal controls (Table 1). These results show that variants in IQCN were associated with sperm flagellar assembly defects.
Motility parameters of sperm from normal control and affected individuals with IQCN variants.
. | Patient 1 (c.3040A>G; c.3913A>T) . | Patient 2 (c.2453_2454del) . | Patient 3 (c.910C>T) . | Normal (mean±SD) . |
---|---|---|---|---|
Curvilinear velocity (μm/s) | 51.17 | 43.14 | 25.15 | 55.83 ± 12.16 |
Straight-line velocity (μm/s) | 20.92 | 18.99 | 9.41 | 25.60 ± 6.60 |
Average path velocity (μm/s) | 26.97 | 23.82 | 12.56 | 31.65 ± 7.24 |
. | Patient 1 (c.3040A>G; c.3913A>T) . | Patient 2 (c.2453_2454del) . | Patient 3 (c.910C>T) . | Normal (mean±SD) . |
---|---|---|---|---|
Curvilinear velocity (μm/s) | 51.17 | 43.14 | 25.15 | 55.83 ± 12.16 |
Straight-line velocity (μm/s) | 20.92 | 18.99 | 9.41 | 25.60 ± 6.60 |
Average path velocity (μm/s) | 26.97 | 23.82 | 12.56 | 31.65 ± 7.24 |
IQCN, IQ motif-containing N.
Motility parameters of sperm from normal control and affected individuals with IQCN variants.
. | Patient 1 (c.3040A>G; c.3913A>T) . | Patient 2 (c.2453_2454del) . | Patient 3 (c.910C>T) . | Normal (mean±SD) . |
---|---|---|---|---|
Curvilinear velocity (μm/s) | 51.17 | 43.14 | 25.15 | 55.83 ± 12.16 |
Straight-line velocity (μm/s) | 20.92 | 18.99 | 9.41 | 25.60 ± 6.60 |
Average path velocity (μm/s) | 26.97 | 23.82 | 12.56 | 31.65 ± 7.24 |
. | Patient 1 (c.3040A>G; c.3913A>T) . | Patient 2 (c.2453_2454del) . | Patient 3 (c.910C>T) . | Normal (mean±SD) . |
---|---|---|---|---|
Curvilinear velocity (μm/s) | 51.17 | 43.14 | 25.15 | 55.83 ± 12.16 |
Straight-line velocity (μm/s) | 20.92 | 18.99 | 9.41 | 25.60 ± 6.60 |
Average path velocity (μm/s) | 26.97 | 23.82 | 12.56 | 31.65 ± 7.24 |
IQCN, IQ motif-containing N.
Characterization in Iqcn knockout (Iqcn−/−) male mice
Because the variants in exon 3 of IQCN (Patients 2 and 3) led to premature termination of translation, we generated a knockout mouse model with deletions of exons 3 and 4 in IQCN to mimic the variants in males. To investigate the relation between IQCN and flagellar assembly, we examined the motility parameters of sperm from Iqcn−/− mice. CASA analysis found that the motility and progressive motility of sperm from Iqcn−/− mice were significantly lower than those from Iqcn+/+ mice (Supplementary Table S3). The VCL (25.97 ± 0.54 μm/s), VSL (9.06 ± 0.40 μm/s), and VAP (12.76 ± 0.45 μm/s) of fresh sperm from Iqcn−/− male mice were lower than those of fresh sperm from Iqcn+/− male mice (39.45 ± 1.17, 14.77 ± 0.74, and 18.70 ± 1.15 μm/s, respectively) (Fig. 2A). In addition, the VCL, VSL, and VAP parameters in Iqcn+/− male mice were lower than those in Iqcn+/+ male mice (47.21 ± 1.31, 18.57 ± 1.60, and 23.78 ± 1.58 μm/s, respectively) (Fig. 2A).
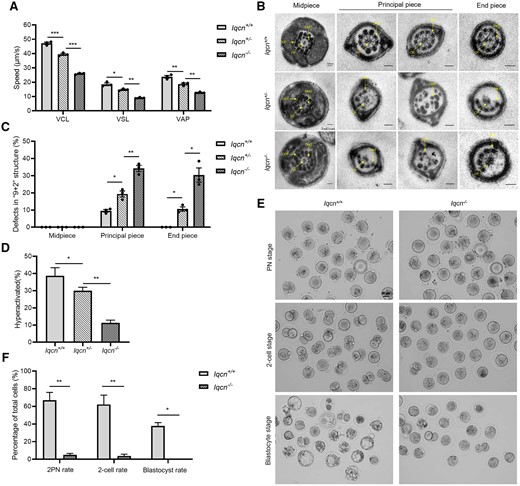
Characterization in Iqcn−/−male mice. (A) VCL, VSL, and VAP of sperm from Iqcn+/+, Iqcn+/−, and Iqcn−/− mice (n = 3). (B) Representative TEM micrographs of defects in the ‘9 + 2’ structure, including disorganized arrangements and/or absence of CPs, peripheral DMTs, and ODFs. Scale bars: 100 nm. (C) The percentages of sperm with defects in the ‘9 + 2’ structure from Iqcn+/+, Iqcn+/−, and Iqcn−/− mice (n = 3). (D) The percentage of hyperactivated sperm from Iqcn+/+, Iqcn+/− and Iqcn−/− mice (n = 3). (E) Representative 2PN zygotes, 2-cell embryos, and blastocysts after IVF with sperm from Iqcn+/+ and Iqcn−/− male mice. Scale bars, 40 μm. (F) Percentage of 2PN zygotes, 2-cell embryos, and blastocysts after IVF in Iqcn+/+ and Iqcn−/− male mice (n = 3). The data are presented as the mean ± SD. *P<0.05, **P<0.01, ***P<0.001 by the independent-samples Student’s t-test (F), one-way ANOVA (A, C, D), Mann–Whitney U-test (F), or Kruskal–Wallis test (C). IQCN, IQ motif-containing N; VCL, curvilinear velocity; VSL, straight line velocity; VAP, average path velocity; TEM, transmission electron microscopy; CP, central-pair microtubule; DMT, peripheral doublet microtubule; ODF, outer dense fiber.
We then observed the ultrastructure of flagella in mouse spermatozoa. The abnormal flagellar phenotypes observed were consistent with those of the affected men. In spermatozoa from Iqcn+/+ male mice, most of the midpiece, principal piece, and end piece of flagella showed a ‘9 + 2’ structure with a pair of CPs, nine ODFs, and DMTs. However, a high proportion of spermatozoa exhibited abnormal ‘9 + 2’ structures in the principal piece (34.22% ± 3.13%) and end piece (30.37% ± 7.13%) of flagella in Iqcn−/− male mice, which were significantly higher than the rates in Iqcn+/− male mice (19.34% ± 3.04% and 10.57% ± 2.21%, respectively) and Iqcn+/+ male mice (9.54% ± 1.37% and 0.00% ± 0.00%, respectively) (Fig. 2C). The main abnormal phenotype was the absence of some DMTs and ODFs or the chaotic arrangement of DMTs (Fig. 2B). These results indicate that a deficiency of IQCN leads to defects in flagellar assembly and sperm motility.
We further examined the hyperactivation ability of spermatozoa and found that hyperactivated motility (11.33% ± 1.53%) of spermatozoa from Iqcn−/− male mice was weaker than that of spermatozoa from Iqcn+/− male mice (30.00% ± 2.00%) and Iqcn+/+ male mice (38.67% ± 4.73%) (Fig. 2D). For IVF, the 2PN and 2-cell rates in Iqcn−/− mice were significantly lower than those in Iqcn+/+ mice. The blastocyst rate was zero in Iqcn−/− mice (Fig. 2E and F). These results indicate that the hyperactivation ability of sperm from Iqcn−/− male mice was impaired, leading to fertilization failure in IVF.
IQCN regulates the cytoskeleton-related pathway in spermiogenesis
To explore the mechanism by which IQCN regulates spermiogenesis, we used IP followed by LC–MS to find the differentially expressed IQCN-binding proteins in the testes of Iqcn+/+ and Iqcn−/− male mice. After normalization of raw data, DEPs (FC > 1.5) were identified between Iqcn+/+ and Iqcn−/− testes. Specifically, 1388 downregulated DEPs and 423 upregulated DEPs were identified. GO analysis revealed that downregulated DEPs were enriched in cytoskeleton-related pathways, such as actin filament-based movement (GO: 0030029), cytoskeleton-dependent intracellular transport (GO: 0030705), and axonemal dynein complex assembly (GO: 0070286) (Fig. 3A). We further investigated the predicted regulatory networks between cytoskeleton-related DEPs. The results showed that differentially expressed IQCN-binding proteins could be divided into two main groups (Fig. 3B). The regulated ratio of DEPs enriched in these two groups is listed in Supplementary Table S4. One group was the IFT protein family and its motor proteins (IFT74, IFT57, IFT81, IFT43, IFT27, IFT172, IFT22, IFT122, IFT140, IFT52, WDR19, WDR35, TTC30A1, TTC26, TTC21B, DYNC2LI1, and DYNC2H1) (Fig. 3B). In another group of differential IQCN-binding proteins, we found three hub proteins, namely CDC42, ACTB, and ACTG1, which might play essential functions in the regulation of spermiogenesis.
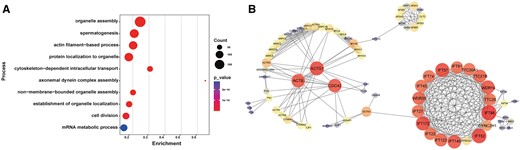
Potential downstream molecular changes regulated by IQCN. (A) Gene Ontology term enrichment of downregulated DEPs. (B) Protein–protein interaction network of downregulated DEPs enriched in microtubule-related processes. The plot was represented using STRING. The PPI dot size and color represent the degree of the network, and red indicates the hub genes. IQCN, IQ motif-containing N; DEP, differentially expressed proteins; PPI, pixel per inch.
IQCN interacts with CDC42 and IFT family proteins to participate in sperm flagellar assembly
We further investigated the hub protein CDC42 in mouse and human spermatozoa. In spermatozoa from Iqcn+/+ male mice, CDC42 was localized in the equatorial and post-equatorial regions of the head and the flagellum. However, in spermatozoa from Iqcn−/− male mice, CDC42 was absent in epididymis spermatozoa (Fig. 4A and Supplementary Fig. S3A). In normal human spermatozoa, CDC42 was localized to the equatorial region of the head and the flagellum, however in spermatozoa from Patients 1 and 2, CDC42 was only located in the midpiece of the flagella, and was absent in spermatozoa from Patient 3 (Fig. 4C).
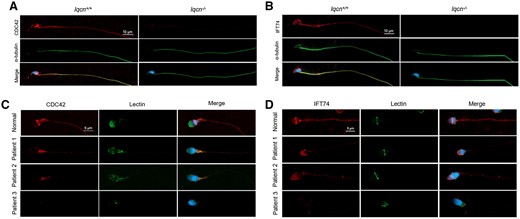
Immunostaining of CDC42 and IFT74 in spermatozoa from mice and humans. (A) Immunostaining of CDC42 (red) and α-tubulin (green) in spermatozoa from Iqcn+/+ and Iqcn−/− male mice. Scale bars, 10 μm. (B) Immunostaining of IFT74 (red) and α-tubulin staining (green) in spermatozoa from Iqcn+/+ and Iqcn−/− male mice. Scale bars, 10 μm. (C) Immunostaining of CDC42 (red) and α-tubulin staining (green) in spermatozoa from normal controls and affected individuals. Scale bars, 5 μm. (D) Immunostaining of IFT74 (red) and α-tubulin staining (green) in spermatozoa from normal controls and affected individuals. Scale bars, 5 μm. IQCN, IQ motif-containing N; CDC42, cell division cycle 42; IFT74, intraflagellar transport 74.
In the IFT protein family, we selected one IFT protein, IFT74, for validation, which is a known flagellar protein in spermatozoa (Shi et al., 2019). In spermatozoa from Iqcn+/+ male mice, IFT74 was localized in the acrosome and flagellum (Fig. 4B and Supplementary Fig. S3B). However, in spermatozoa from Iqcn−/− male mice, IFT74 was absent. We next tested the localization of IFT74 in human spermatozoa. In normal spermatozoa, IFT74 was found in the equatorial region of the head and the flagellum (Fig. 4D), while in spermatozoa from Patients 1 and 2, IFT74 was only localized in the acrosome region of the head (Fig. 4D). In spermatozoa from Patient 3, IFT74 was totally absent. These results indicate that a deficiency in IQCN causes the loss of binding with CDC42 and IFT74, which leads to the absence or abnormal localization of CDC42 and IFT74 in mouse and human spermatozoa.
Discussion
The manchette is a transient structure that is involved in shaping the spermatid nucleus and in protein transport within the flagellum. In a previous study, we found that IQCN was a novel protein located in the manchette structure during spermiogenesis (Dai et al., 2022). Homozygous variants in IQCN resulted in head deformity in epididymal sperm and abnormal localization of phospholipase Cζ (Dai et al., 2022). In this study, we identified two novel missense variants and one recurrent variant in IQCN. Sperm from men carrying variants in IQCN exhibited flagellar assembly defects with irregular ‘9 + 2’ structures, which led to male infertility. Therefore, this study expanded the mutation spectrum and explored the varieties of phenotypes in IQCN.
In recent years, CASA, as an instrument for sperm motility assessment, has been increasingly used in reproductive clinics. Unlike the traditional manual semen routine analysis that produces variable, and possibly unreliable, results owing to the subjective judgment of the observer, CASA has a higher consistency and accuracy and can provide quantitative data on sperm dynamics and parameters (Dearing et al., 2021; Choi et al., 2022). However, few studies have objectively evaluated sperm kinematics using CASA technology (Veron et al., 2018). Further research is needed to determine whether abnormal CASA parameters are related to male infertility. We found that the parameters of motility and progressive motility in the patient samples were ‘normal’, although VCL, VSL, and VAP parameters were lower than those in men with normal fertility. This result suggests that male infertility, in some cases, may be related to abnormal CASA parameters, which are difficult to identify by routine semen analysis. Establishing a standardized range of CASA parameters will provide a basis for the clinical diagnosis of motility-related sperm defects.
Using IP followed by LC–MS to investigate the cause of the defects in motility, two clusters of differential IQCN-binding proteins that participate in cytoskeleton-related biological processes were identified. Importantly, we also identified a hub protein, CDC42. CDC42 localizes to the perinuclear region of the manchette at the head of the elongating spermatid, to the neck region of the elongated spermatid, and to the midpiece of mature spermatozoa, which are involved in terminal structure formation of sperm heads (Huang et al., 2018). CDC42 is a regulatory protein that also localizes to the CatSper nanodomain complex and has a critical function in the activity of this channel by modulating cAMP production through soluble adenylate cyclase (Luque et al., 2021). In this study, defects in IQCN led to the loss of its interaction with CDC42, resulting in impaired manchette-related functions and sperm flagellar assembly.
When compared to the LC–MS by IP with calmodulin antibody in our previous study (Dai et al., 2022), a cluster of IFT family proteins identified in both data sets included IFT22, IFT43, IFT74, IFT81, IFT140, IFT172, WDR19, TTC21B, and DYNC2LI1. It is hypothesized that IFT family proteins are calmodulin-binding proteins that engage in sperm flagellum-related functions. We selected IFT74 for further investigation. IFT74 is a component of the core intra-flagellar transport complex, involved in a bidirectional movement of large particles along axoneme microtubules for cilia formation (Shi et al., 2019). Deficiency in the Ift74 gene in male germ cells resulted in infertility with a low sperm count and immotile sperm in mice (Shi et al., 2019). In our study, IFT74 was absent or aberrantly localized in the flagellum of mutant sperm from mice and humans, which might be related to their abnormal axoneme microtubules and irregular ‘9 + 2’ structures. This study also provides possible avenues for future intervention and treatment, including the discovery of upstream regulatory molecules.
We speculate that variants in IQCN lead to defects in both acrosome and sperm flagellar assembly. However, phenotypic differences still exist owing to heterogeneity of the variant sites and types. The nonsense variant (c.910C>T) in IQCN had the most severe effect, with a significant decrease in CASA parameters and the absence of the interacting proteins CDC42 and IFT74. Moreover, the frameshift variant (c.2453_2454del) resulted in a slight decrease in CASA parameters and abnormal localization of CDC42 and IFT74. Weaker effects were observed for the compound heterozygous variants (c.3040A>G and c.3913A>T), which caused no significant abnormalities in CASA parameters but caused abnormal localization of the interacting proteins CDC42 and IFT74. As Patient 1, who had these two variants, presented normal CASA parameters, we speculate that the variants were missense variants located near the C-terminus of IQCN and may have weaker effects. Although they cause impairment of sperm flagellar assembly, they are not severe enough to affect sperm motility. Of course, a larger sample size is needed to verify this speculation.
In conclusion, in this study we found that IQCN regulates sperm flagellar assembly through the interaction of the hub protein CDC42 with the IFT protein family. This study therefore provides a reference for the diagnosis of sperm motility disorders and male infertility.
Data availability
Data available on request: the data underlying this article will be shared on reasonable request to the corresponding author.
Acknowledgements
We would like to thank the patients for participating in and supporting this study. We also thank the Center of Cryo-Electron Microscopy at Central South University for their technical support.
Authors’ roles
All authors contributed to the study design. G.L. and J.D. performed conceptualization and design; J.D. and Q.L. performed most of the experiments and analyzed the data; J.G. assisted with the animal housing; Y.W., W.Z., S.Z., and F.G. collected the human samples and clinical data; G.-X.L. was responsible for supervision; J.D. and Q.L. wrote the draft of manuscript; G.L. supervised the study and the manuscript writing.
Funding
This work was supported by the National Natural Science Foundation of China (81974230 and 82202053), the Changsha Municipal Natural Science Foundation (kq2202072), the Hunan Provincial Natural Science Foundation (2022JJ40658), and the Scientific Research Foundation of Reproductive and Genetic Hospital of CITIC-Xiangya (YNXM-202114 and YNXM-202201). No conflicts of interest were declared.
Conflict of interest
None declared.