-
PDF
- Split View
-
Views
-
Cite
Cite
Gunapala Shetty, Jennifer M Mitchell, Truong Nguyen Anh Lam, Zhuang Wu, Jie Zhang, Lorraine Hill, Ramesh C Tailor, Karen A Peters, Maria Cecilia Penedo, Kyle E Orwig, Marvin L Meistrich, Donor spermatogenesis in de novo formed seminiferous tubules from transplanted testicular cells in rhesus monkey testis, Human Reproduction, Volume 33, Issue 12, December 2018, Pages 2249–2255, https://doi.org/10.1093/humrep/dey316
- Share Icon Share
Abstract
Can transplanted primate testicular cells form seminiferous tubules de novo, supporting complete spermatogenesis?
Cryopreserved testicular cells from a prepubertal monkey can reorganize in an adult monkey recipient testis forming de novo seminiferous tubular cords supporting complete spermatogenesis.
De novo morphogenesis of testicular tissue using aggregated cells from non-primate species grafted either subcutaneously or in the testis can support spermatogenesis.
Two postpubertal rhesus monkeys (Macaca mulatta) were given testicular irradiation. One monkey was given GnRH-antagonist treatment from 8 to 16 weeks after irradiation, while the other received sham injections. At 16 weeks, cryopreserved testicular cells from two different prepubertal monkeys [43 × 106 viable (Trypan-blue excluding) cells in 260 μl, and 80 × 106 viable cells in 400 μl] were transplanted via ultrasound-guided injections to one of the rete testis in each recipient, and immune suppression was given. The contralateral testis was sham transplanted. Testes were analyzed 9 months after transplantation.
Spermatogenic recovery was assessed by testicular volume, weight, histology and immunofluorescence. Microsatellite genotyping of regions of testicular sections obtained by LCM determined whether the cells were derived from the host or transplanted cells.
Transplanted testis of the GnRH-antagonist-treated recipient, but not the sham-treated recipient, contained numerous irregularly shaped seminiferous tubular cords, 89% of which had differentiating germ cells, including sperm in a few of them. The percentages of donor genotype in different regions of this testis were as follows: normal tubule, 0%; inflammatory, 0%; abnormal tubule region, 67%; whole interior of abnormal tubules, >99%; adluminal region of the abnormal tubules, 92%. Thus, these abnormal tubules, including the enclosed germ cells, were derived de novo from the donor testicular cells.
Not applicable.
The de novo tubules were observed in only one out of the two monkeys transplanted with prepubertal donor testicular cells.
These findings may represent a promising strategy for restoration of fertility in male childhood cancer survivors. The approach could be particularly useful in those exposed to therapeutic agents that are detrimental to the normal development of the tubule somatic cells affecting the ability of the endogenous tubules to support spermatogenesis, even from transplanted spermatogonial stem cells.
This work was supported by research grants P01 HD075795 from Eunice Kennedy Shriver National Institute of Child Health & Human Development (NICHD/NIH) to K.E.O and Cancer Center Support Grant P30 CA016672 from NCI/NIH to The University of Texas MD Anderson Cancer Center. The authors declare that they have no competing interests.
Introduction
Cancer treatments endanger the fertility of young men of reproductive age. In the USA alone each year, over 2000 additional boys will be rendered sterile due to the complete loss of spermatogonial stem cells (SSC) from chemotherapy and/or radiotherapy. These prepubertal patients cannot produce semen for cryopreservation and do not currently have any fertility preservation choices that have proven effective. New methods to restore normal reproductive function after cytotoxic treatment are of great importance to these young male cancer survivors.
Techniques being tested for potential future production of sperm in a cancer survivor, including SSC transplantation, testicular tissue grafting, and in vitro development of sperm (Gassei and Orwig, 2016), all have advantages and disadvantages. Because exposure of prepubertal primate testis to high therapeutic doses of radiation causes irreversible damage to the somatic components of the seminiferous tubule, as observed histologically (de Rooij et al., 2002), by failure of tubular extension during puberty (Jahnukainen et al., 2011), and by gene expression changes (Trondle et al., 2017), the damaged tubules may not efficiently support sperm production from the transplanted SSCs. Although autologous grafting of fragments of prepubertal testicular tissue appears to be an attractive strategy, there is the possibility of reintroducing tumor cells since the grafts cannot be subjected to elimination by cell sorting. The production of monkey offspring from sperm produced in xenografts of testicular tissue has been demonstrated (Liu et al., 2016), but there is the possibility of infection by murine retroviruses. In vitro production of functional sperm from prepubertal testis tissues could overcome these limitations; however, the process has not yet been demonstrated in primates.
Isolated prepubertal testicular cells are capable of reorganizing into testicular cords (Gassei et al., 2006). Implantation of aggregates of prepubertal testis cells from rodents, sheep and pigs under the skin (Honaramooz et al., 2007; Kita et al., 2007; Arregui et al., 2008) or in the testis (Toyooka et al., 2003) results in de novo organization of seminiferous tubules that produce spermatids. Given that isolated cells are employed, separation of normal from tumor cells is possible.
Transplantation into the testis, rather than subcutaneously, has advantages of exposure to high testosterone levels and other paracrine factors as well as normal thermoregulation for germ cells. In this study, we report that cryopreserved testicular cells from a prepubertal monkey can undergo morphogenetic reorganization in the interstitial area of an irradiated recipient macaque testis forming de novo seminiferous tubules that support complete spermatogenesis derived from donor SSCs.
Materials and Methods
Two male rhesus monkeys (Macaca mulatta) (M1 and M2) were purchased from Keeling Center for Comparative Medicine and Research, MD Anderson Cancer Center, Bastrop, TX, USA and maintained under standard conditions (Shetty et al., 2013). All animal care and treatment protocols were approved by the Institutional Animal Care and Use Committees of MD Anderson Cancer Center and Magee Womens Research Institute.
After the monkeys reached puberty, their testes were irradiated with 7 Gy (Supplementary Fig. S1A), which eliminates nearly all the germ cells including most of the SSCs (Shetty et al., 2013). GnRH-antagonist (GnRH-ant) treatment with Acyline (obtained from the Contraceptive Development Program of the NICHD, Rockville, MD, USA) was given to one monkey (M1) from 8 to 16 weeks after irradiation as twice-weekly injections, to enhance engraftment and recovery of the transplanted germ cells (Shetty et al., 2013). The second monkey (M2) only received the sham injections.
The donor cells for transplantation were recovered from the testicular parenchyma of two prepubertal rhesus monkeys using a two-step enzymatic digestion procedure in the Orwig lab at Magee Womens Research Institute (Hermann et al., 2007, 2009). Cells were cryopreserved and shipped on liquid nitrogen to Houston. The donor monkey for M1 was 40 months old and had 1.1 g testes; the donor for M2 was 46 months old with 1.2 g testes. Further, neither suspension contained elongating spermatids, which was consistent with their prepubertal status.
Testicular transplantation was performed at 16 weeks after irradiation, into one testis of each monkey (Hermann et al., 2012). Allogeneic transplantation was used so that donor and host cells could be distinguished by their DNA fingerprints. The cryopreserved cells were thawed, washed and were resuspended at ~2.6 × 108 cells/ml in Minimum Essential Media α (Life Technologies Corporation, Grand Island, New York, USA) containing 10% fetal bovine serum (Hyclone Laboratories, Logan, UT, USA), 0.4 mg Trypan-blue/ml (Sigma Aldrich, St. Louis, MO, USA), 20% (v/v) Optison ultrasound contrast agent (GE Healthcare, Waukesha, WI, USA), 1% antibiotic-antimycotic (ThermoFisher Waltham, MA USA), and 0.1 mg DNase I/ml (Sigma Aldrich, St. Louis, MO, USA). The cells were transplanted via ultrasound-guided injections into the rete testis. The contralateral testis was sham transplanted. M1 was injected with 43 × 106 viable (Trypan-blue excluding) cells in 260 μl; M2 received 80 × 106 viable cells in 400 μl.
To prevent T cell-mediated rejection of the grafted cells, transplant recipients were injected with human/mouse chimeric anti-CD154 IgG 5C8 (NIH Nonhuman Primate Reagent Resource, University of Massachusetts Medical School, Boston, MA, USA) (Hermann et al., 2012) at 20 mg/kg on the following schedule relative to transplantation: −1 day, days 0, 1, 3, 10, 18, 28 and 4-week intervals thereafter through week 24. The testes were harvested 40 weeks after transplantation for analyses.
Genomic DNA isolated from blood or tissue was used for microsatellite repeat genotyping according to methods previously described (Larsen et al., 2010; Hermann et al., 2012). Microsatellite markers were derived from human (27 markers with D prefix) and Macaca fuscata (two markers with MFGT prefix) sequences. Out of a panel of 29 microsatellites, D3S1768, D4S2365, D7S794, D13S765, D15S823, D17S1300 proved informative for distinguishing recipient and donor tissue. Microsatellites were amplified by multiplexed PCR and the products were separated by capillary electrophoresis on an ABI 3730 DNA Analyzer (Applied Biosystems, Foster City, CA, USA). Fragment size analysis and genotyping was performed with the computer software STRand (http://www.vgl.ucdavis.edu/informatics/strand.php).
To analyze different regions of the transplanted testis, laser capture microdissection (LCM) was performed on testis sections (Sluka et al., 2008) and DNA was extracted from the LCM caps. We used 10 μm paraffin embedded monkey testis sections fixed in 70% ethanol. They were mounted on PEN-membrane glass slides (LCM0522, ThermoFisher Scientific, Waltham, MA, USA), to enable the combined use of UV laser cutting and infrared LCM. Slides were stained with hematoxylin. The ArcturusXT Laser Capture Microdissection system (AB applied Biosystems by Life Technologies, Carlsbad, CA, USA) was used. A minimum of about 10 000 cells of interest were captured on CapSure (LCM0211) caps.
For histological analyses, the testis tissue was fixed in Bouin’s solution, embedded in paraffin and sections were stained with periodic-acid-Schiff reagent and hematoxylin. Seminiferous tubule cross-sections were analyzed for the presence or absence of germ cells and the most advanced germ cell type present, in at least three sections chosen systematically at different regions of the testis. The tubule differentiation index (TDI), which represents the percentage of seminiferous tubule cross-sections containing at least one differentiated germ cell type (B spermatogonia or later stages) (Shetty et al., 2013), was computed. While scoring the abnormal tubules with incomplete basement membrane, those with gaps of less than ~25 μ were considered as individual tubules.
For fluorescent immunostaining, paraffin sections of testes, fixed either in Bouin’s or 4% paraformaldehyde were used. The deparaffinized slides after antigen retrieval (Shetty et al., 2016) were rinsed and blocked, sections were then stained with the following primary antibodies. Goat anti-VASA (1:200) marks all germ cells (Castrillon et al., 2000). Goat anti-PIWIL1 (Piwi Like RNA-Mediated Gene Silencing 1) (1:200; R & D systems, catalog number: AF6548), which is also known as MIWI or HIWI, marks spermatocytes and spermatids (Deng and Lin, 2002). Rabbit anti-acrosin (1:300; Novus bio, catalog number: NBP1-85407) marks the acrosomes of spermatids (Reda et al., 2016). The sections were then counterstained with DAPI (Vector Laboratories). Positive immunoreactivity was validated by omission of primary antibody.
Additional details of Methods are presented in Supplementary Data.
Results
The monkeys’ testes were irradiated at 51 months of age when their testis volumes were 11.1 and 12.2 ml and their serum testosterone levels were 1.9 and 4.6 ng/ml for M1 and M2, respectively, confirming that they were postpubertal. GnRH-ant was given to M1 for 8 weeks prior to the time of transplantation. Testis volumes were reduced 40–60% in these monkeys, consistent with the radiation-induced germ cell loss and the hormone-suppression induced somatic cell shrinkage (Supplementary Fig. S1B and C). Starting 30 weeks after transplantation, the volume of the transplanted testis in the GnRH-ant treated monkey (M1) began increasing at a greater rate than that of the contralateral testis, and at the end of the study, it weighed 9% more than that of the contralateral testis (Supplementary Fig. S1D). There were no differences in volume or weight between the two testes in the other recipient, M2.
Sections of the transplanted testis in the GnRH-ant-treated monkey (M1) revealed irregular cords that resembled seminiferous tubules (henceforth called de novo tubules), which were distinctly different from the normal endogenous irradiated tubules (Fig. 1A). Areas of inflammatory cells were interspersed between these abnormal tubules, possibly as a result of the cessation of the anti-CD154 treatment about 3 months prior to tissue harvest. The abnormal tubules occupied about 13% of total testicular space and were most extensive at one pole of the testis (Fig. 1B). Generally, there was a clear boundary between the area packed with these abnormal tubules and the endogenous tubules. However, there were a few isolated endogenous atrophic or recovering normal tubules in between these unusual tubules (Supplementary Fig. S2A and B). Occasionally, incompletely formed basal laminae extended into these irregular tubules suggesting that tubule formation might be ongoing (red arrows, Fig. 1D and E).
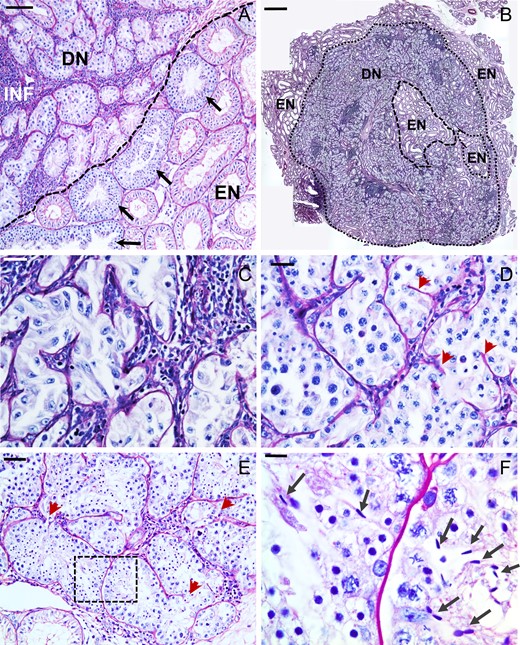
Histology of the abnormal tubules observed in the transplanted monkey (Macaca mulatta) testis. (A) Section of transplanted testis showing disorganized and misshapen tubules, likely derived de novo (DN) from the transplanted testicular cells. These tubules are interspersed with inflammatory (INF) cells. There generally was a clear boundary (dashed line) between the area packed with these abnormal tubules and the endogenous (EN) tubules. The endogenous tubules at this boundary were enriched with repopulated tubules (arrows). (B) The de novo tubules invaded a major area in this section near the pole of the testis. De novo tubules were either Sertoli cell-only (C) or contained stages until spermatocytes (D), round spermatids (E) or mature spermatids (arrows in F, which is enlarged from boxed portion in E). Note the incomplete basal laminae within the tubules (red arrowheads). Scale bars: A: 50 μm, B: 1 mm, C,D,E: 25 μm and F: 10 μm.
About 11% of these unique tubules contained only Sertoli cells (Fig. 1C) and the rest (89%) had normal appearing differentiating germ cells. In most of these tubules differentiation, only progressed to the spermatocyte stage (Fig. 1D), but there were spermatids in 6% of such tubules (Fig. 1E) and even condensed sperm in 0.4% (five tubules in the three regional sections studied) of the tubules (Fig. 1F, arrows). Specific cells in these abnormal tubules were positive for VASA, PIWIL1 and acrosin (Fig. 2) demonstrating that they support normal germ cell development.
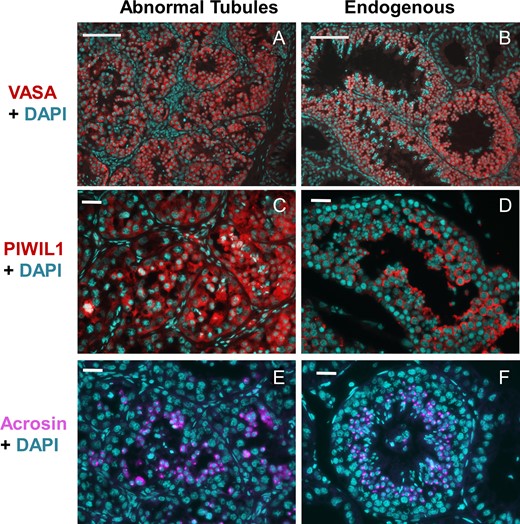
Immunofluorescent staining of the transplanted monkey testis. Markers for germ cells (VASA) (A and B), spermatocytes/spermatids (PIWIL1: (Piwi Like RNA-Mediated Gene Silencing 1)) (C and D) and spermatids (acrosin) (E and F) were used. Note that the germ cells in the abnormal tubules stain positively for all the markers (A, C, and E), as observed in the normal endogenous tubules (B, D and F) and the acrosin staining is localized to the acrosomal cap of spermatids. DAPI was used to counterstain the nuclei. Scale bars: A,B: 100 μm, C–F: 25 μm.
The percentage of normal endogenous tubules showing differentiated germ cells (TDI) in the transplanted testis of monkey M1 was 4.2% compared to 3% in the contralateral sham testis, which did not show any abnormal tubules. Interestingly, the endogenous tubules at the boundary (Fig. 1A) and within the areas invaded by the de novo tubules (Supplementary Fig. S2B) showed a TDI of 14% as compared to 3% in other regions. This result suggests a paracrine influence of de novo tubules on the recovery of spermatogenesis in the neighboring endogenous tubules, as observed previously in a rat model (Zhang et al., 2009).
The transplanted testis of monkey M2 did not show any signs of such abnormal tubule formation (Supplementary Fig. S2C). Furthermore, the TDI in the transplanted (0.3%) and sham-transplanted testis (0.4%) were similar.
To test whether the abnormal tubules observed in the transplanted testis of M1 were formed de novo from donor testicular cells, we collected tissues from the different areas of the testis by LCM (Fig. 3) and analyzed the DNA microsatellites. Areas consisting of endogenous (Fig. 3A and A′), inflammatory (Fig. 3B and B′) and de novo tubules (Fig. 3C and C′) were dissected. Figures 3D and D′, and 3E and E′ show representative sections from which the entire tubule or just the adluminal cells leaving the basal layer, respectively, were dissected away (D and E) and the pooled tubule portions from these respective regions (D′ and E′).
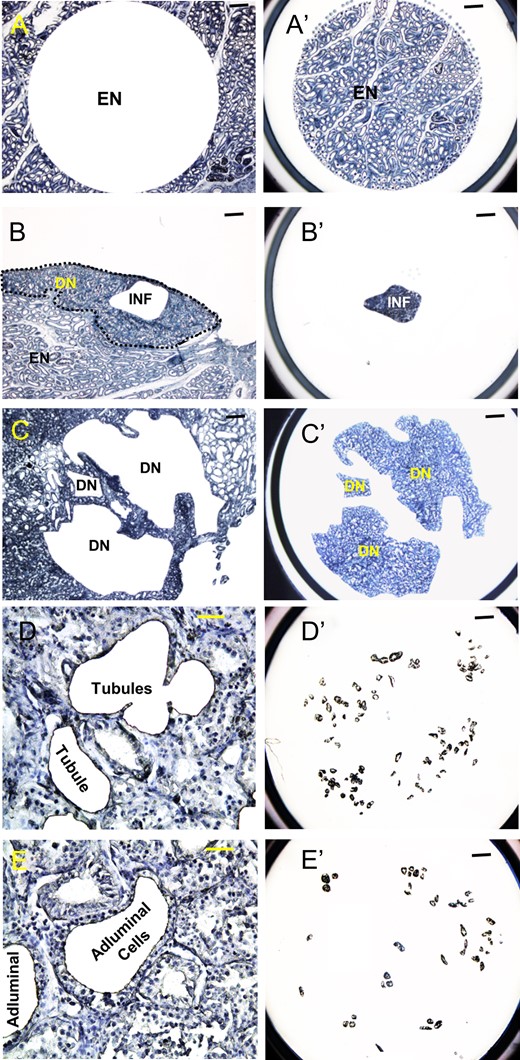
Laser capture microdissection of different regions from tissue sections of the transplanted testis of monkey M1. (A, B, and C) Remaining tissue after dissection. (A′, B′ and C′) Dissected tissues on caps. (D, E) High magnification images showing outlines of representative tubules from which the entire tubule and just the adluminal cells leaving the basal layer, respectively, were dissected away. (D′, E′) Caps showing large number of such tubule portions dissected from multiple regions similar to those shown in D and E. (A, A′) Area containing exclusively EN. (B, B′) Area with INF found in regions of abnormal tubules. (C, C′) Areas with irregular tubules that are suspected to be DN. (D, D′) Dissection of whole tubules (Tubules) containing germ and somatic cells from the abnormal tubular regions. (E, E′) Dissection of the adluminal regions (Adluminal) of these tubules presumably containing only germ cells. Scale bars: A–C, A′–C′, D′, E′: 400 μm; D, E: 50 μm.
Microsatellite analysis showed that in the endogenous area, which contained normal appearing tubules that were mostly atrophic due to irradiation, the cells were entirely of recipient origin, with no evidence of colonization by donor germ cells (Table I). In addition, >90% of the cells in the inflammatory regions were of the recipient origin. However, the area containing abnormal tubules contained an average of 67% of donor genotype. The cells obtained in whole tubule sections dissected from the interior of the abnormal tubules, which contain germ and tubular somatic cells, were exclusively of donor origin. Thus, the higher percentage of host cells in the larger pieces of tissue from the de novo tubule area must be a result of inflammatory and perhaps host Leydig and vascular cells. Finally, 92% of the cells dissected from the adluminal region of the abnormal tubules, which should contain exclusively germ cells, were of the donor genotype. These results suggest that the abnormal tubules, including the enclosed germ cells, were indeed derived de novo from the donor testicular cells.
Results of microsatellite analysis of the recipient rhesus monkey (Macaca mulatta) testes cells 9 months after transplantation.
Area . | TissueBlock . | Microsatellite loci used . | Percentage of Donor Genotype . | Percentage of Recipient Genotype . |
---|---|---|---|---|
Endogenous | 1 | D4S2365, D13S765 D17S1300 | <1% | >99% |
1 | <0.5% | >99.5% | ||
Inflammation | 1 | D4S2365, D13S765 D17S1300 | 9.4 ± 3.9% | 90.6 ± 3.9% |
1 | <1% | >99% | ||
Abnormal tubules | 1 | D3S1768, D4S2365, D13S765, D17S1300 | 48.8 ± 1.6% | 51.2 ± 1.6% |
1 | 65.4 ± 1.5% | 34.6 ± 1.5% | ||
2 | 72.9 ± 2.2% | 27.1 ± 2.2% | ||
Tubule interior | 2 | D3S1768, D4S2365 D13S765, D15S823 | >98% | <2% |
Adluminal Region of Tubule | 2 | D4S2365, D7S794, D13S765 | 92.1 ± 1.0% | 7.9 ± 1.0% |
Area . | TissueBlock . | Microsatellite loci used . | Percentage of Donor Genotype . | Percentage of Recipient Genotype . |
---|---|---|---|---|
Endogenous | 1 | D4S2365, D13S765 D17S1300 | <1% | >99% |
1 | <0.5% | >99.5% | ||
Inflammation | 1 | D4S2365, D13S765 D17S1300 | 9.4 ± 3.9% | 90.6 ± 3.9% |
1 | <1% | >99% | ||
Abnormal tubules | 1 | D3S1768, D4S2365, D13S765, D17S1300 | 48.8 ± 1.6% | 51.2 ± 1.6% |
1 | 65.4 ± 1.5% | 34.6 ± 1.5% | ||
2 | 72.9 ± 2.2% | 27.1 ± 2.2% | ||
Tubule interior | 2 | D3S1768, D4S2365 D13S765, D15S823 | >98% | <2% |
Adluminal Region of Tubule | 2 | D4S2365, D7S794, D13S765 | 92.1 ± 1.0% | 7.9 ± 1.0% |
Cells were microdissected from areas of endogenous tubules, areas containing inflammatory cells, areas containing abnormal (de novo) tubules, the entire contents of individual abnormal tubules (germ and somatic cells), and adluminal regions of these abnormal tubules (germ cells). Different slides were used for each sample. The percentages of donor and recipient cells were calculated from the heights of donor and recipient peaks, respectively. Results are averages and SEM of analyses of three or four microsatellite loci.
Results of microsatellite analysis of the recipient rhesus monkey (Macaca mulatta) testes cells 9 months after transplantation.
Area . | TissueBlock . | Microsatellite loci used . | Percentage of Donor Genotype . | Percentage of Recipient Genotype . |
---|---|---|---|---|
Endogenous | 1 | D4S2365, D13S765 D17S1300 | <1% | >99% |
1 | <0.5% | >99.5% | ||
Inflammation | 1 | D4S2365, D13S765 D17S1300 | 9.4 ± 3.9% | 90.6 ± 3.9% |
1 | <1% | >99% | ||
Abnormal tubules | 1 | D3S1768, D4S2365, D13S765, D17S1300 | 48.8 ± 1.6% | 51.2 ± 1.6% |
1 | 65.4 ± 1.5% | 34.6 ± 1.5% | ||
2 | 72.9 ± 2.2% | 27.1 ± 2.2% | ||
Tubule interior | 2 | D3S1768, D4S2365 D13S765, D15S823 | >98% | <2% |
Adluminal Region of Tubule | 2 | D4S2365, D7S794, D13S765 | 92.1 ± 1.0% | 7.9 ± 1.0% |
Area . | TissueBlock . | Microsatellite loci used . | Percentage of Donor Genotype . | Percentage of Recipient Genotype . |
---|---|---|---|---|
Endogenous | 1 | D4S2365, D13S765 D17S1300 | <1% | >99% |
1 | <0.5% | >99.5% | ||
Inflammation | 1 | D4S2365, D13S765 D17S1300 | 9.4 ± 3.9% | 90.6 ± 3.9% |
1 | <1% | >99% | ||
Abnormal tubules | 1 | D3S1768, D4S2365, D13S765, D17S1300 | 48.8 ± 1.6% | 51.2 ± 1.6% |
1 | 65.4 ± 1.5% | 34.6 ± 1.5% | ||
2 | 72.9 ± 2.2% | 27.1 ± 2.2% | ||
Tubule interior | 2 | D3S1768, D4S2365 D13S765, D15S823 | >98% | <2% |
Adluminal Region of Tubule | 2 | D4S2365, D7S794, D13S765 | 92.1 ± 1.0% | 7.9 ± 1.0% |
Cells were microdissected from areas of endogenous tubules, areas containing inflammatory cells, areas containing abnormal (de novo) tubules, the entire contents of individual abnormal tubules (germ and somatic cells), and adluminal regions of these abnormal tubules (germ cells). Different slides were used for each sample. The percentages of donor and recipient cells were calculated from the heights of donor and recipient peaks, respectively. Results are averages and SEM of analyses of three or four microsatellite loci.
Discussion
We have shown that allogeneic transplantation of cryopreserved testicular cells from a prepubertal monkey to the irradiated testis of a postpubertal monkey resulted in the development of irregularly shaped seminiferous tubular structures. Histological and immunohistochemical studies confirmed that these de novo tubules contained spermatogenic cells of all types including the mature spermatids. Further, microsatellite analyses of the microdissected cells revealed that these tubules including the germ cells are derived from the donor cells. These results for the first time demonstrate the formation of de novo seminiferous tubules from transplanted testicular cells in a primate that are capable of supporting complete spermatogenesis from donor cells.
In the current study, even at 9 months after transplantation, spermatids were produced in only 6% of the de novo tubules and condensed sperm in only 0.4% of tubules. In contrast, in the same monkey 94% of the recovering endogenous tubules had elongating or condensed spermatids. The production of round spermatids in the allogeneic intratesticular injection procedure is comparable to that produced after xenogeneic subcutaneous grafting of prepubertal rhesus monkey tissue in nude mice (Honaramooz et al., 2004; Ehmcke et al., 2011). However, the yield of condensed spermatids is lower. The reasons for the failure to have complete spermatogenesis in many de novo tubules may be due to insufficient time for complete spermatogenesis in recently formed de novo tubules and incomplete immune suppression; if the latter is true, the spermatogenic outcome may be better with autologous transplantation of testicular cells.
All previous studies employing de novo morphogenesis of testicular tissue capable of supporting spermatogenesis were carried out in other species and used cells that were aggregated, either spontaneously in culture or by pelleting the cells and grafting the aggregates in vivo (Toyooka et al., 2003; Gassei et al., 2006; Honaramooz et al., 2007; Kita et al., 2007; Arregui et al., 2008). This is the first study in which a single-cell suspension aggregated in vivo and formed tubules supporting complete spermatogenesis. Previously, injection of testis cell suspensions were demonstrated to form tubules either with no evidence of germ cells within them (Zhang et al., 2009) or with only spermatogonia (Aeckerle et al., 2013).
Although the cells were injected into the rete testis and on ultrasound appeared to enter the tubules, some transplanted cells must have inadvertently leaked into the interstitial space, as shown previously with rete injection in human tissue (Faes et al., 2017). In our rat studies, de novo tubule formation was enhanced when interstitial injection was employed as opposed to the attempted rete testis injection (Zhang et al., 2009). We would expect the same in the primate model and that should be tested.
Possible factors involved in the formation of these de novo tubules were considered. It seems to be essential that the donor cells be from a prepubertal monkey (Aeckerle et al., 2013). The two monkeys in the current study were part of a larger study involving 16 recipients treated with the same radiation dose. The 14 other monkeys were injected with cells from more mature monkeys, as evidenced by spermatozoa in all their cell suspensions, and none demonstrated de novo tubule formation. Thus, it is possible that the failure of the donor for M2 to form de novo tubules was because it was 6 months older and its testes weighed 0.1 g more than did the donor for M1. Another possible factor is that the hormone suppression given to M1 just prior to transplantation stimulated de novo tubule formation. A third reason for different results between M1 and M2 may be the variation in interstitial leakage during rete testis injection (Faes et al., 2017).
In conclusion, the present findings validate the feasibility of inducing de novo tubules for the production of donor sperm in a non-human primate. They represent a new and promising strategy for the restoration of fertility in male childhood cancer survivors by autologous transplantation of their cryopreserved somatic and germ testicular cells. The approach should be particularly useful in those exposed to high doses of therapeutic agents that can be detrimental to the normal development of the testicular somatic cells, affecting the ability of the endogenous tubules to support spermatogenesis from transplanted SSC. Since these newly formed tubules are unlikely to connect to the rete testis, sperm will need to be collected by testicular sperm extraction and used for ICSI.
Acknowledgements
We thank Jennifer M. Meyer, and Lisa M. Moore who managed the treatment, handling, and health of the monkeys and Dr Min S. Lee, National Institute for Child Health and Human Development, for providing the Acyline.
Authors’ roles
G.S. conceptualized and designed the study, analyzed the immunohistochemistry and other results, and prepared the manuscript. J.M.M and L.H. oversaw the treatment of the monkeys, and surgeries. T.N.A.L., Z.W. and J.Z. did most of the experimental work. R.C.T. performed the dosimetry for testicular radiation. M.C.P. did microsatellite analysis. K.E.O., with the assistance of K.A.P., performed the transplantation. M.L.M. participated in the analysis of results and preparation of the manuscript.
Funding
This work was supported by research grants P01 HD075795 from Eunice Kennedy Shriver National Institute of Child Health & Human Development (NICHD/NIH) to K.E.O and Cancer Center Support Grant P30 CA016672 from NCI/NIH to The University of Texas MD Anderson Cancer Center.
Conflict of interest
None declared.