-
PDF
- Split View
-
Views
-
Cite
Cite
Yiyuan Liu, Yuqian Li, Lanlan Meng, Kuokuo Li, Yang Gao, Mingrong Lv, Rui Guo, Yuping Xu, Ping Zhou, Zhaolian Wei, Xiaojin He, Yunxia Cao, Huan Wu, Yueqiu Tan, Rong Hua, Bi-allelic human TEKT3 mutations cause male infertility with oligoasthenoteratozoospermia owing to acrosomal hypoplasia and reduced progressive motility, Human Molecular Genetics, Volume 32, Issue 10, 15 May 2023, Pages 1730–1740, https://doi.org/10.1093/hmg/ddad013
- Share Icon Share
Abstract
Oligoasthenoteratozoospermia (OAT) can result in male infertility owing to reduced sperm motility and abnormal spermatozoan morphology. The Tektins are a family of highly conserved filamentous proteins expressed in the axoneme and associated structures in many different metazoan species. Earlier studies on mice identified Tektin3 (Tekt3) as a testis-enriched gene, and knockout of Tekt3 resulted in asthenozoospermia in the mice. Here, whole-exome sequencing of 100 males with asthenozoospermia from unrelated families was performed, followed by Sanger sequencing, leading to the identification of TEKT3 as a candidate gene in two of these patients and their associated family members. In total, three mutations in the TEKT3 gene were identified in both these patients, including one homozygous deletion–insertion mutation (c.543_547delinsTTGAT: p.Glu182*) and one compound heterozygous mutation (c.[548G > A]; [752A > C], p.[Arg183Gln]; [Gln251Pro]). Both of these mutations resulted in the complete loss of TEKT3 expression. The patients were both found to produce sperm that, although those showed no apparent defects in the flagellar structure, had reduced progressive motility. In contrast to mice, most sperm from these two patients exhibited acrosomal hypoplasia, although this did not prevent the use of the sperm for in vitro fertilization through an ICSI approach. TEKT3 was found to bind to other TEKT proteins, suggesting that these proteins form a complex within human spermatozoa. Overall, these results suggest that a loss of TEKT3 function can contribute to OAT incidence in humans. TEKT3 deficiencies can reduce sperm motility and contribute to severe acrosomal hypoplasia in spermatozoa, compromising their normal function.
Introduction
Male infertility is a complicated pathological condition with a multifactorial etiology. Genetic abnormalities have been identified in just ~15% of all cases, whereas the causes of male infertility in upwards of 73% of cases remain unknown (1–3). Asthenozoospermia, defined as a reduction in sperm motility (<32% progressive forward motility or <40% total motility), is among the most common causes of male infertility, having been identified as a contributing factor in ~40% of all cases (4). Most cases of infertility involving compromised sperm motility show a complex phenotype consisting of a combination of asthenozoospermia and oligo- and/or teratozoospermia, collectively referred to as oligoasthenoteratozoospermia (OAT) (5). Several pathogenic mutations have been linked to the incidence of OAT to date, underscoring the potential for single-gene mutations to cause this form of infertility (6–14).
Tektins are a family of sarkosyl-insoluble coiled-coil filamentous proteins that were initially purified from sperm flagella from the purple sea urchin Strongylocentrotus purpuratus where they were found to stably associate with three to four tubulin protofilaments in A and B outer doublet microtubule walls (15,16). More recent work has also shown the association of Tektins with flagella, centrioles, basal bodies and nuclear lamins (16–18).
Mammalian Tektins are present in the retina, brain, testis and other tissue types that harbor ciliated cells (19). In total, five Tetkins (TEKT1–5) have been identified to date in mammals, with most of these proteins involved in sperm motility (19–23). Tekt2-knockout mice produce sperm with decreased motility and impaired flagellar bending (24), while mice lacking Tekt4 produce sperm with dramatically reduced forward progressive velocity and uncoordinated flagellar waveform propagation (22). These results suggest that Tektins are closely related to the motility and structural integrity of sperm flagella. In one recent study of bovine ciliary microtubules, TEKT1–4 were found to form a complex consisting of four or eight Tetkins that were localized to microtubular ribbons, which is in line with their predicted function in the sperm of sea urchins (25), offering a promising basis for the exploration of the functional role of Tetkins in human sperm flagella.
Tektin3 (TEKT3) maps to human chromosome 17 and mouse chromosome 11 and has been reported to play an essential role in the forward progressive motility of mammalian sperm, with the loss of Tekt3 expression in mice resulting in flagellar bending defects (26). TEKT3 proteins have also been detected in bovine sperm heads where they are associated with the processes of hyperactivation and capacitation (27,28). However, no studies to date have examined the physiological functions of TEKT3 in human spermatozoa.
Here, a whole-exome sequencing (WES) approach was used to evaluate 100 patients from unrelated families affected by severe asthenozoospermia. This analysis ultimately led to the identification of biallelic TEKT3 mutations in two individuals, including one patient harboring a homozygous deletion–insertion mutation (c.543_547delinsTTGAT:p.Glu182*) and another harboring a compound heterozygous mutation (c.[548G > A]; [752A > C], p.[Arg183Gln]; [Gln251Pro]). Sperm from these patients exhibited significantly decreased progressive motility. However, TEKT3defects in human sperm resulted in acrosomal hypoplasia without any apparent structural defects in the flagella, which is in contrast to the phenotypes observed in mice. Through the use of in vitro fertilization, both patients were able to successfully achieve clinical pregnancies. Overall, this study offers insight into the functional role of TEKT3 in the context of human sperm motility, offering a theoretical foundation for genetic counseling and assisted reproductive treatment options for patients diagnosed with OAT.
Results
TEKT3 is a candidate gene associated with human OAT-related male infertility
Here, WES and bioinformatics approaches were used to analyze 100 males affected by asthenozoospermia. A number of potentially pathogenic variations were identified (Supplementary Material, Table S4), including variations in the TEKT3 gene in two unrelated patients (Fig. 1A). The first of these probands (A001: II-1, 26 years old) was born of a consanguineous family (Family A001), with 98.5% of sperm presenting with abnormal head morphology and a progressive motility of just 13.6% (Table 1). Routine semiological analyses of A006: II-1 (30 years old), who was a member of a non-consanguineous family (Family A006), similarly revealed a severe OAT phenotype characterized by 96.5% of sperm presenting with abnormal head morphology and a progressive motility rate of only 2.2% (Table 1).
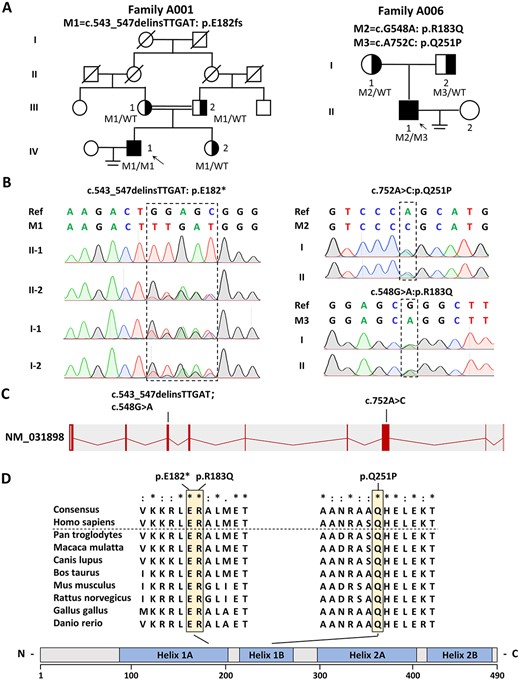
Identification of TEKT3 mutations in human patients with OAT. (A) Pedigrees of families (A001 and A006) presenting with OAT associated with TEKT3 mutations. A black arrow is used to mark the probands in these two families. WT, wild-type; M1/M2/M3, mutant alleles. (B) Sanger sequencing was used to verify mutations identified by WES. (C) Mutated site locations within the TEKT3 gene. (D) Amino acid substitutions in the TEKT3 protein are presented along with an overview of the evolutionary conservation of the mutation-containing protein domains.
Clinical semen parameters and assisted reproductive outcomes in TEKT3-mutation patients
. | Patient . | Reference values . | |||
---|---|---|---|---|---|
. | A001 II-1 . | A006 II-1 . | . | ||
Semen parameters | |||||
Age | 26 | 30 | |||
Semen volume (ml) | 3.5 | 4.6 | 3.0 | 1.7 | >1.5 |
Sperm concentration (106/ml) | 72.5 | 76.7 | 0.6 | 1.0 | >15.0 |
Progressive motility (%) | 12.7 | 14.5 | 2.2 | 0 | >32.0 |
Motility (%) | 43.1 | 49.5 | 6.8 | 0 | >40.0 |
Morphologically normal sperm (%) | 0 | 0 | 4 | 3.0 | >4 |
Sperm with head defect (%) | 99.01 | 98 | 96 | 97 | |
Sperm with tail defect (%) | 15 | 14 | 23.9 | 22.3 | |
Bent flagella (%) | 8.3 | 6.5 | 3.0 | 3.2 | <13.0 |
Outcomes of ICSI | |||||
Male age (year) | 26 | 30 | |||
Female age (year) | 25 | 28 | |||
Number of ICSI cycles | 1 | 1 | |||
Number of oocytes injected | 3 | 5 | |||
Number (and rate) of fertilized oocytes | 3 (100%) | 5 (100%) | |||
Number (and rate) of cleavage embryos | 3 (100%) | 5 (100%) | |||
Number (and rate) of eight cells | 2 (66%) | 2 (40%) | |||
Number of transfer cycles | 1 | 2 | |||
Number of embryos transferred per cycle | 1 | 1 | |||
Implantation rate | 100% | 50% | |||
Clinical pregnancy rate | 100% | 50% | |||
Miscarriage | N | N |
. | Patient . | Reference values . | |||
---|---|---|---|---|---|
. | A001 II-1 . | A006 II-1 . | . | ||
Semen parameters | |||||
Age | 26 | 30 | |||
Semen volume (ml) | 3.5 | 4.6 | 3.0 | 1.7 | >1.5 |
Sperm concentration (106/ml) | 72.5 | 76.7 | 0.6 | 1.0 | >15.0 |
Progressive motility (%) | 12.7 | 14.5 | 2.2 | 0 | >32.0 |
Motility (%) | 43.1 | 49.5 | 6.8 | 0 | >40.0 |
Morphologically normal sperm (%) | 0 | 0 | 4 | 3.0 | >4 |
Sperm with head defect (%) | 99.01 | 98 | 96 | 97 | |
Sperm with tail defect (%) | 15 | 14 | 23.9 | 22.3 | |
Bent flagella (%) | 8.3 | 6.5 | 3.0 | 3.2 | <13.0 |
Outcomes of ICSI | |||||
Male age (year) | 26 | 30 | |||
Female age (year) | 25 | 28 | |||
Number of ICSI cycles | 1 | 1 | |||
Number of oocytes injected | 3 | 5 | |||
Number (and rate) of fertilized oocytes | 3 (100%) | 5 (100%) | |||
Number (and rate) of cleavage embryos | 3 (100%) | 5 (100%) | |||
Number (and rate) of eight cells | 2 (66%) | 2 (40%) | |||
Number of transfer cycles | 1 | 2 | |||
Number of embryos transferred per cycle | 1 | 1 | |||
Implantation rate | 100% | 50% | |||
Clinical pregnancy rate | 100% | 50% | |||
Miscarriage | N | N |
Clinical semen parameters and assisted reproductive outcomes in TEKT3-mutation patients
. | Patient . | Reference values . | |||
---|---|---|---|---|---|
. | A001 II-1 . | A006 II-1 . | . | ||
Semen parameters | |||||
Age | 26 | 30 | |||
Semen volume (ml) | 3.5 | 4.6 | 3.0 | 1.7 | >1.5 |
Sperm concentration (106/ml) | 72.5 | 76.7 | 0.6 | 1.0 | >15.0 |
Progressive motility (%) | 12.7 | 14.5 | 2.2 | 0 | >32.0 |
Motility (%) | 43.1 | 49.5 | 6.8 | 0 | >40.0 |
Morphologically normal sperm (%) | 0 | 0 | 4 | 3.0 | >4 |
Sperm with head defect (%) | 99.01 | 98 | 96 | 97 | |
Sperm with tail defect (%) | 15 | 14 | 23.9 | 22.3 | |
Bent flagella (%) | 8.3 | 6.5 | 3.0 | 3.2 | <13.0 |
Outcomes of ICSI | |||||
Male age (year) | 26 | 30 | |||
Female age (year) | 25 | 28 | |||
Number of ICSI cycles | 1 | 1 | |||
Number of oocytes injected | 3 | 5 | |||
Number (and rate) of fertilized oocytes | 3 (100%) | 5 (100%) | |||
Number (and rate) of cleavage embryos | 3 (100%) | 5 (100%) | |||
Number (and rate) of eight cells | 2 (66%) | 2 (40%) | |||
Number of transfer cycles | 1 | 2 | |||
Number of embryos transferred per cycle | 1 | 1 | |||
Implantation rate | 100% | 50% | |||
Clinical pregnancy rate | 100% | 50% | |||
Miscarriage | N | N |
. | Patient . | Reference values . | |||
---|---|---|---|---|---|
. | A001 II-1 . | A006 II-1 . | . | ||
Semen parameters | |||||
Age | 26 | 30 | |||
Semen volume (ml) | 3.5 | 4.6 | 3.0 | 1.7 | >1.5 |
Sperm concentration (106/ml) | 72.5 | 76.7 | 0.6 | 1.0 | >15.0 |
Progressive motility (%) | 12.7 | 14.5 | 2.2 | 0 | >32.0 |
Motility (%) | 43.1 | 49.5 | 6.8 | 0 | >40.0 |
Morphologically normal sperm (%) | 0 | 0 | 4 | 3.0 | >4 |
Sperm with head defect (%) | 99.01 | 98 | 96 | 97 | |
Sperm with tail defect (%) | 15 | 14 | 23.9 | 22.3 | |
Bent flagella (%) | 8.3 | 6.5 | 3.0 | 3.2 | <13.0 |
Outcomes of ICSI | |||||
Male age (year) | 26 | 30 | |||
Female age (year) | 25 | 28 | |||
Number of ICSI cycles | 1 | 1 | |||
Number of oocytes injected | 3 | 5 | |||
Number (and rate) of fertilized oocytes | 3 (100%) | 5 (100%) | |||
Number (and rate) of cleavage embryos | 3 (100%) | 5 (100%) | |||
Number (and rate) of eight cells | 2 (66%) | 2 (40%) | |||
Number of transfer cycles | 1 | 2 | |||
Number of embryos transferred per cycle | 1 | 1 | |||
Implantation rate | 100% | 50% | |||
Clinical pregnancy rate | 100% | 50% | |||
Miscarriage | N | N |
Sanger sequencing was used to verify the TEKT3 mutations detected in the initial WES analyses (Fig. 1B). Proband A006 II-I harbored a homozygous TEKT3 deletion insertion mutation c. 543_547delinsTTGAT (p.Glu182*), while his sister and parents were heterozygous carriers for this mutation (Fig. 1B). The mutation results in the generation of a premature stop codon at position 182 of the 490 amino acid coding sequence (Fig. 1C). In contrast, proband A006 II-I presented with a compound heterozygous TEKT3 mutation (c.548G > A [p. Arg183Gln], c.752A > C [p. Gln251Pro]), with both of his parents being heterozygous carriers (Fig. 1B). The observed missense mutations altered the identity of highly conserved TEKT3 amino acids (Fig. 1C and D) and were predicted to be potentially deleterious by the SIFT, PolyPhen-2 and Variant Taster tools (Supplementary Material, Table S5). Neither c.543_547delinsTTGAT nor c.A752C was present in the human population datasets included in the 1000 Genomes Project, ExAC and the Genome Aggregation Database, and the frequency of c.G548A is also <0.001 in these human databases (Supplementary Material, Table S5). Accordingly, these novel homozygous and compound heterozygous TEKT3 mutations were considered as the likely candidates for the OAT phenotypes in these proband patients.
The identified mutations result in a loss of TEKT3 protein expression in spermatozoa
To further explore the potential pathogenicity of the TEKT3 mutations identified in these probands, both mRNA and protein levels were detected in the probands’ samples. The qPCR data showed that TEKT3 mRNA was significantly decreased in the spermatozoa of A001 II-1 (Fig. 2A). Immunofluorescent staining and western immunoblotting were used to detect the TETK3 protein levels in the sperm samples from these patients. While normal control sperm exhibited a ~56 kDa protein band corresponding to TEKT3 in the western immunoblotting analyses, this band was wholly absent in both probands (Fig. 2B), which is consistent with the loss of TEKT3 expression. In line with these findings, control spermatozoa exhibited TEKT3 expression localized to the head and the entirety of the flagella, while sperm from the probands only exhibited a non-specific signal associated with the mitochondrial sheath (Fig. 2C). TEKT3 plasmids were introduced into the 293 T cell line. Interestingly, neither of the mutation sites disrupted the expression of TEKT3 or even affected the TEKT3 half-life in vitro (Supplementary Material, Fig. S1). These results suggested that these two missense mutations probably do not interfere with TEKT3 expression but may trigger the degradation of TEKT3 in spermatozoa by impairing the specific function of TEKT3. Future studies are required to address this hypothesis.
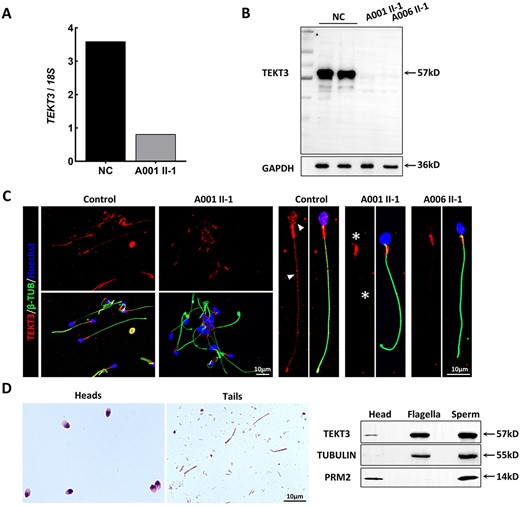
TEKT3 expression profiles in human spermatozoa. (A) TEKT3 expression was confirmed by qPCR to be reduced in the proband A001 II-1. (B) Western immunoblotting analysis of TEKT3 in human spermatozoa from control and proband individuals. (C) Anti-TEKT3 antibody staining of spermatozoa from a fertile control patient and the two probands, with anti-β-tubulin (β-TUB) serving as a control. (D) Spermatozoa were separated into fractions enriched for head and tail domains, with representative images shown on the left, and western immunoblotting results for TEKT3 protein levels in these head and tail fractions in spermatozoa obtained from multiple healthy control individuals.
Given that the observed TEKT3 signal in the sperm head domains was relatively weak (Fig. 2B), sperm head and tail fractions were collected separately, and the ~56 kDa protein band was evident in both these sample fractions (Fig. 2D). This suggested that TEKT3 was expressed in both the tail and head domains of spermatozoa. The loss of TEKT3 expression in the sperm of these probands was thus identified as the presumed pathogenic cause of OAT, which is in line with the phenotypes observed in knockout mice (26).
Spermatozoa harboring TEKT3 mutations exhibit acrosomal hypoplasia
Next, changes in the morphology and ultrastructural characteristics of spermatozoa from the probands harboring the TEKT3 mutations were assessed. Using light microscopy and H&E staining (Fig. 3A), no apparent differences in the sperm flagella were observed when comparing TEKT3 mutant sperm with those from healthy controls. However, a small or absent acrosome was observed for 97.5% of these TEKT3-deficient spermatozoa (Fig. 3B). Acrosomal ultrastructural characteristics in the sperm from these probands were then assessed with SEM (Fig. 3C), which revealed that while control sperm exhibited an acrosome comprising 40–70% of the head domain, this acrosomal morphology was largely absent in the TEKT3-mutant sperm.
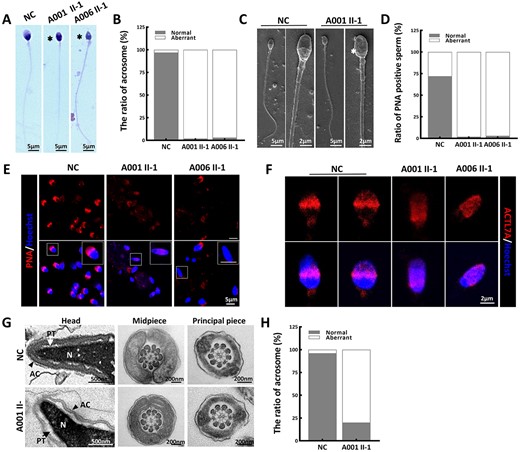
TEKT3-mutant spermatozoa show small thin acrosomes. (A–C) Microscopic analyses were used to assess the differences in spermatozoa from normal control and proband samples harboring TEKT3 mutations. Spermatozoa were analyzed by light microscopy following H&E staining (A) or SEM (C). Sperm exhibiting acrosomal abnormalities are denoted with asterisks. (B) The frequencies of morphologically normal acrosomes in spermatozoa in the indicated individuals are shown by light microscopy. (D and E) Confocal laser scanning microscopy was used to image spermatozoa following acrosomal and nuclear staining with PNA and DAPI, respectively. (F) Immunofluorescent staining of ACTL7A in spermatozoa from a normal donor and probands harboring TEKT3 mutations. (G and H) Ultrastructural characteristics of spermatozoa in samples from a fertile donor and probands harboring TEKT3 mutations. AC, acrosome; N, nucleus; PT, perinuclear theca.
Immunofluorescent staining was used to further probe the morphological characteristics of the acrosomal region in spermatozoa from these probands, revealing that, relative to control sperm, sperm harboring the TEKT3 mutations exhibited a significantly weaker PNA signal corresponding to the sub-acrosomal layer, although this signal was still detectable (Fig. 3D and E). In addition, an ACTL7A signal was evident outside of the nucleus in control cells, which was enriched in the equatorial segment of the head and neck regions, whereas in TEKT3-mutant sperm, this ACT7A signal was decreased and more diffuse over the sperm head (Fig. 3F). TEM analyses suggested that TEKT3-mutant spermatozoa harbored acrosomes that were crumpled and detached from the nuclear envelope (Fig. 3G and Supplementary Material, Fig. S2). The mutant cells exhibited increased thickening and irregularities of the perinuclear theca relative to control spermatozoa (Fig. 3G and H and Supplementary Material, Fig. S2). In summary, spermatozoa harboring TEKT3 mutations did not exhibit any apparent morphological abnormalities in the flagella but did exhibit acrosomal hypoplasia and detachment from the sperm head.
Structural and functional analyses of TEKT3-mutant patients’ sperm flagella
TEKT3 expression levels were found to be markedly higher in the sperm flagellum relative to the head domain. Accordingly, the structural and functional characteristics of sperm flagella from the TEKT3-mutant probands were further analyzed. Western immunoblotting did not reveal any apparent abnormalities between control and TEKT3-mutant patient samples when assessing ODF2 and HSP60, which are respective markers for outer dense fibers and mitochondria (Fig. 4A and B). The A-kinase anchoring protein AKAP4 has been found to be associated with the maintenance of fibrous sheath integrity, but no apparent differences in the AKAP4 expression or localization were evident in relation to the TEKT3 mutations, localizing to the sperm principal piece in all samples (Fig. 4C).
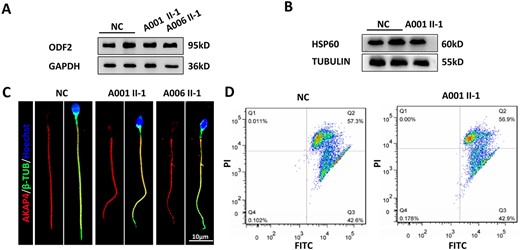
Structural characteristics of sperm flagella and the presence of intact mitochondrial sheath function in sperm from TEKT3-deficient patients. (A and B) Western immunoblotting was used to detect ODF2 and HSP60 in spermatozoa from fertile controls and the two probands. (C) Immunofluorescent staining was used to detect AKAP4 levels in spermatozoa. (D) MMP was determined by flow cytometry using JC-1, with FL1 and FL2 used to capture the FITC and PI signals, respectively.
The effective production of ATP is critical for normal sperm motility (29). Measurement of the MMP can directly reflect the function of mitochondria within spermatozoa, providing insight into the overall mitochondrial energy status of these cells (30). TEKT3-mutant spermatozoa showed MMPs that were similar to those of spermatozoa from control individuals (Fig. 4D). Based on these results, it was concluded that the impaired sperm motility was not caused by the obvious defects in these structures.
TEKT3 interacts with other Tektins in human and murine spermatozoa
Cryo-electron tomographic analyses of bovine respiratory cilia have revealed that Tektins form hyperstable polymers on the inner side of the A-tubule lumen of dynein decorated doublet microtubules (25). Given that TEKT5 expression is restricted to spermatozoa (31), Tektins may exhibit structural differences when comparing cilia and sperm flagella. To identify proteins with which TEKT3 was capable of interacting, a co-IP analysis was conducted using murine testis tissue extracts and mass spectrometry, revealing binding to other Tektins (Fig. 5B and Supplementary Material, Table S6). To further investigate the relationship between TEKT3 and other Tektins, TEKT4 and TEKT5 levels were detected in the patients’ spermatozoa. The data showed that the absence of TEKT3 led to a significant reduction in the levels of the other two Tektins (Fig. 5B). Overexpression of human Tektins in HEK293T cells followed by co-IP analyses showed that human TEKT3 interacted with TEKT1, 2, 4 and 5 (Fig. 5C). Together, these data suggest that Tektins may exhibit conserved functional activity in humans, mice and cattle, forming a multimeric protein complex that can control sperm motility.
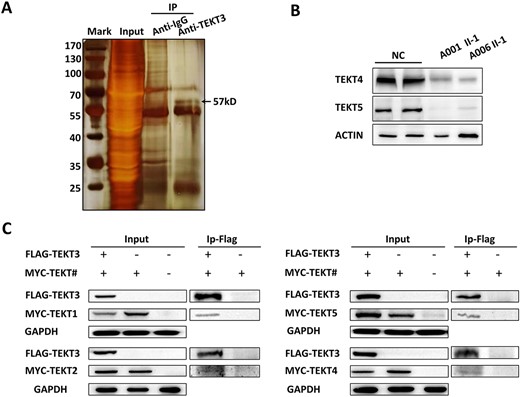
Mammalian TEKT family proteins form a range of complexes. (A) Silver-stained gel of TEKT3 and control immunoprecipitated (IP) samples from mouse testis extracts. Individual lanes were excised and analyzed by mass spectrometry. (B) The expression of TEKT4 and TEKT5 in spermatozoa was assessed by western blotting. β-Actin was used as a control for normalization. (C) Human TEKTINs were co-expressed in HEK293T cells, with co-IP used to detect interactions between TEKT3 and other members of the protein family.
ICSI can overcome infertility in males harboring deleterious TEKT3 mutations
The couples including the two probands both underwent ICSI treatment. For the A001 couple, three metaphase II oocytes were collected and microinjected with spermatozoa from A001 II-1, all three of which were successfully fertilized (2PN/injected oocytes = 100%). Similarly, for couple A006, five metaphase II oocytes were collected and microinjected with spermatozoa from A006 II-1, with all five having been successfully fertilized (2PN/injected oocytes = 100%) (Table 1). Both of the couples carried the baby to term and gave birth, confirming that TEKT3 deficiency can be resolved with ICSI technology.
Discussion
Over 4000 genes are estimated to be required for the production of spermatozoa (32), highlighting the value of high-throughput sequencing approaches as tools capable of aiding in the clinical diagnosis of male infertility and the exploration of the complex mechanisms underlying infertility phenotypes (33). Here, a WES approach was used to explore the genetic basis for infertility in 100 unrelated males diagnosed with asthenozoospermia. This analysis ultimately led to the identification of novel biallelic mutations in the TEKT3 gene, including a homozygous deletion–insertion variant (c.543_547delinsTTGAT: p. Glu182*) and a compound heterozygous variant (c.[548G > A]; [752A > C], p.[Arg183Gln]; [Gln251Pro]). This study is the first to our knowledge to have linked TEKT3 mutations and asthenozoospermia in human spermatozoa.
Tektins were initially identified as integral microtubule components in sea urchin sperm flagella and have since been shown to comprise a mammalian protein family containing at least five members (21–23,26,34–36). These Tektins are highly conserved across mammalian species and exhibit a characteristic non-apeptide C-terminal signature sequence (37). TEKT1 was the first mammalian member of this family to be identified, and the loss of TEKT1 function results in the dysfunction of motile cilia (38). Mice lacking TEKT2, TEKT3 and TEKT4 exhibit asthenozoospermia and decreased progressive motility (22,24,26). The roles that Tetkins play in human spermatozoa, however, are poorly understood. Here, TEKT3 was found to localize to both the head and tail domains of healthy human spermatozoa. Sperm from two probands harboring TEKT3 mutations exhibited significantly decreased progressive motility with an acrosomal structure that was small or absent. These results suggest that TEKT3 mutations may represent a novel cause of male infertility in humans as a consequence of acrosomal hypoplasia and the impairment of progressive motility.
Prior research has shown that Tektins are present in the head domains of spermatozoa, indicating that they may participate in the acrosome reaction and gamete fusion (27,28,39). Here, sperm harboring TEKT3 mutations were found to exhibit pronounced acrosomal hypoplasia, with significant reductions in the PNA levels in the sperm head. Further, the acrosomal protein ATC7LA, was also found to be decreased in the sperm of these patients harboring the TEKT3 mutations . Several reports have suggested that sperm with hypoplastic or absent acrosomes cannot fertilize oocytes (40–42). Given that ICSI was sufficient to achieve clinical pregnancy for both couples in which the male partner harbored TEKT3 mutations, this suggests that the remaining acrosome and acrosomal proteins in these spermatozoa are sufficient to mediate fertilization in an assisted reproductive treatment setting.
The murine TEKT3 protein has been shown to be enriched in male germ cells, with the highest expression in pachytene spermatocytes and round spermatids in the testis (21). The sperm of Tekt3-knockout mice showed reduced progressive motility, with structural defects apparent in 30–50% of sperm together with abnormal flagellar bending from 90–180°. These sperm also exhibited thinning of the mitochondrial sheath containing midpiece region (26). In contrast to these murine phenotypes, spermatozoa from infertile human patients harboring TEKT3 mutations did not show any apparent flagellar abnormalities. TEM images of these sperm did not show any atypical flagellar, mitochondrial sheath, ODF, fibrous sheath or 9 + 2 microtubule structures. The structural and functional proteins in these flagella did not appear to be decreased or increased, and normal mitochondrial sheath function was observed. As such, while human and murine Tetkins are structurally similar to one another, it is possible that they may play different roles within the spermatozoa flagella from these different species. Further research exploring the molecular function of these flagellar Tetkins may yield novel insights regarding the structural basis for flagellar motility.
Tektins are among the most conserved and abundant ciliary proteins, forming hyperstable polymers similar to intermediate filaments. While they are important mediators of sperm motility (22,24,26), their structural characteristics, higher-order assembly, positioning and functional roles are still controversial owing to the great variety of paralogous Tektin-like proteins expressed in different species. Tektins have previously been reported to localize to tubules and outside the axoneme, and a recent cryoelectron microscopy-based analysis indicated that Tetkins can function in part by forming complexes within the tracheal cilia of cattle (25). Given that TEKT5 is expressed predominantly in the testis, it is possible that the structure of the complex may differ in spermatozoa. Here, TEKT3 was found to be capable of interacting with TEKT1, 2, 4 and 5 in both human spermatozoa and murine testes. In addition, loss of TEKT3 lead to decreases in TEKT4 and TEKT5, suggesting that Tektins may function in human sperm by forming multi-protein complexes. Given the observed expression of TEKT3 in the sperm head and sperm harboring TEKT3 mutations exhibited pronounced acrosomal hypoplasia, Tektins appear to form part of the scaffold that maintains the normal structure of acrosome. In the sperm tail, the absence of TEKT3 did not affect the flagellar morphology, including the mitochondrial sheath, fibrous sheath and outer dense fibers, although functionally the swing of the axial filaments was affected, leading to reduced sperm motility and male infertility. Further work will be essential to clarify where these complexes are formed, whether monomeric TEKT3 has a different function from that of its complexed state and whether the structure of this Tektin undergoes dynamic structural changes during sperm swimming.
In summary, two novel human biallelic TEKT3 mutations were identified as novel pathogenic drivers of severe asthenozoospermia and associated male factor infertility. These results extend current knowledge regarding the genetic basis for male infertility and may have key clinical implications for genetic counseling and assisted reproductive treatment of affected individuals.
Materials and Methods
Study participants
In total, 100 infertile males of Han ethnicity diagnosed with asthenospermia from the First Affiliated Hospital of Anhui Medical University and the Affiliated Hospital of Xiangya, Central South University in China, were enrolled in this study. All patients were between 20 and 50 years of age, suffered from primary infertility, exhibited asthenospermia as defined by <32% progressive motility, were karyotypically normal (46, XY) and exhibited normal secondary sex characteristics, bilateral testicular size distributions and hormone levels. Parental DNA was collected for 84 of these 100 enrolled patients, with parental relationships being confirmed using an EX20 kit (AGCU Scien Tech Incorporation).
Proband patients
Two patients (Fig. 1, IV-1and II-1) were identified among the 100 patients described before, including a 26-year-old male (Family A001 IV-1; A001 IV-1) and a 30-year-old male (Family A006 II-1; A006 II-1). Neither patient exhibited any evidence of other ciliopathies such as polycystic kidney disease, Bardet-Biedl syndrome or primary ciliary dyskinesia. Both were confirmed to exhibit somatic karyotypes (46; XY) without any large-scale deletions in the Y chromosome. Both provided informed consent to participate and are undergoing continuous follow-up.
Semenological and sperm morphology analyses
Analyses of patient semen were performed through routine protocols as per WHO guidelines (fifth edition, Kruger/Strict morphology criteria). H&E staining was used to assess sperm morphology, with a minimum of 200 spermatozoa being assessed. The percentages of morphologically abnormal spermatozoa were established as per WHO guidelines.
WES and genetic analyses
WES was performed on both probands. A QIAamp DNA Blood Mini Kit (Qiagen, Germany) was used to isolate DNA from the peripheral blood of all the enrolled patients and available members of their immediate family (siblings and parents). The Sure SelectXT Human All Exons V6 kit (Agilent, USA) was used in accordance with the provided directions for exon capture, and the abi3730 platform (Thermo Fisher, USA) was used for WES. Reads were mapped to the hg19 human reference genome using Burrows-Wheeler Aligner software (43). Picard software was used for the removal of duplicates to obtain effective reads, effective base, average coverage depth and 90 × −120 × coverage ratio. The Genome Analysis Toolkit (v2.20) was used for SNV and indel identification, with filtering out of SNVs with fewer than four read depths. Mutation allele frequencies in the general population were determined using the 1000 Genomes Project and Exome Aggregation Consortium (ExAC) Browser (44,45). Conservation of the identified mutation sites and the likely effects of the mutations on protein function were examined using the SIFT, PolyPhen-2, and Variant Taster programs (46–48). Mutations considered as common (allele frequency > 0.01) were not included in these analyses. Primary mutations of interest were loss-of-function variants, such as stop-gain, stop-loss, frameshift indel and splicing (≤2 bp) mutations, with an additional focus on any deleterious missense mutations. Candidate mutations for both included probands are compiled in Supplementary Material, Tables S1 and S2. Ultimately, TEKT3 was selected for further study as it harbored pathogenic mutations in both probands.
Real-time quantitative PCR and reverse-transcription PCR
For real-time quantitative PCR, the total RNA from human spermatozoa and mouse testes was extracted using the Allprep DNA/RNA/Protein Mini Kit (QIAGEN). Approximately, 1 μg of this RNA was reverse-transcribed to cDNA with HiScript II Q RT SuperMix for quantitative PCR (Vazyme). The cDNA was individually diluted 5-fold to be used as templates for subsequent real-time quantitative PCR with AceQ quantitative PCR SYBR Green Master Mix (Vazyme) on a CFX Connect Real-Time PCR Detection System. The 18S was used as an internal control, and primers for real-time quantitative PCR are listed in Supplementary Material, Table S3.
Electron microscopy
Before electron microscopy analyses, sperm from the proband patients and healthy control males were fixed for 1–2 h at room temperature with 2.5% glutaraldehyde, followed by incubation for a minimum of 12 h at 4°C. The samples were then rinsed with PBS and were fixed for 1 h with 1% osmium acid. For scanning electron microscopy (SEM), the osmium solution was removed and samples were rinsed with PBS, followed by dehydration with an ethanol gradient (15 min/concentration). After sample coating, a Nova nano 450 instrument (Thermo Fisher, MA, USA) was employed for imaging. Transmission electron microscopy (TEM) was performed by the removal of the osmium solution, rinsing the samples with PBS and fixing them for 30 min in an aqueous 2% uranium acetate solution. This was followed by dehydration in an ethanol gradient, overnight embedding, cutting into ultra-thin sections and imaging using a Cryoelectron microscope Talos L120C G2 (Thermo Fisher, USA).
Immunofluorescent staining
The human spermatozoa were placed on slides and were fixed for 5–10 min with cold methanol. They were then treated for 1 h with 10% donkey serum albumin and 0.1% Triton X-100 in 1 × PBS at room temperature, followed by overnight incubation with rabbit polyclonal anti-TEKT3 (pa5–49 713, Thermo Fisher, 1:200), rabbit polyclonal anti-AKAP4 (HPA020046 Sigma-Aldrich; 1:200), or mouse anti-acetylated tubulin (5335S, CST, 1:200) at 4°C. After three washes with PBS (10 min/wash), the slides were incubated for 1 h with Alexa Fluor 647 anti-rabbit IgG (Yeasen) or Alexa Fluor 488 anti-mouse IgG (Invitrogen) at room temperature, with DAPI (0.5%) used for nuclear counterstaining. A fluorescence microscope was then used to detect DNA and FITC-peanut agglutinin (PNA) acrosomal staining.
Analyses of sperm mitochondria
The mitochondrial membrane potential (MMP) of the sperm was assessed using JC-1 dye (Solarbio-M8650). Briefly, sperm were rinsed with PBS or treated with CCCP as appropriate, after which 2 × 106 spermatozoa were incubated for 20 min in 1 ml of PBS supplemented with 5 μg/ml JC-1 at 37°C while being protected from light. Spermatozoa were then washed twice with PBS and evaluated via flow cytometry (FACSCanto, BD Biosciences, CA, USA). High MMP levels result in the formation of JC-1 aggregates that yield an orange-red fluorescent signal (FL2 channel), whereas JC-1 remains in a monomeric form exhibiting green fluorescence (FL1 channel) under conditions of low MMP.
Head-tail separation
Sperm heads and tails were separated by suspending spermatozoa from the cauda epididymis in 1 ml of PBS and by sonicating them for three times (10 s each; Sonifier SLPe, Branson Ultrasonics), followed by centrifugated for 5 min at 10 000g. The resultant pellet was suspended in 200 μl of PBS, combined with 1.8 ml of 90% Percoll (GE Healthcare) in PBS and centrifuged for 15 min at 15 000g. The upper and lower layers of the Percoll solutions were isolated as the tail- and head-containing fractions, respectively. Each of these fractions was then separately diluted with PBS (1:5), centrifuged for 5 min at 10 000g, rinsed twice with PBS and suspended in an appropriate sample buffer for Western immunoblotting.
Western immunoblotting
A 200 μl volume of RIPA buffer (Beyotime) was used to lyse cellular samples with a pellet pestle motor homogenizer. Samples were then heated for 15 min to 100°C, separated on 10% SDS-PAGE and transferred to PVDF membranes. The blots were then blocked with 5% non-fat milk in TBST for 1 h at room temperature followed by overnight incubation with anti-TEKT3 (1:2000), rabbit anti-HSP60 (ab46798, Abcam, 1:2000) or rabbit anti-ODF2 (HPA001874, Sigma, 1:2000) at 4°C. The protein bands were detected with an ECL system (Kodak) and were normalized to GAPDH levels.
Cycloheximide test
The cycloheximide test was performed as described previously (5). Cycloheximide (Sigma-Aldrich) was introduced 12 h after cell transfection and fluid change. The cells in the 0 min groups were immediately harvested, and a protease inhibitor cocktail (Thermo Scientific) was added.
Co-immunoprecipitation
Mouse testes were used for co-immunoprecipitation (co-IP) using anti-TEKT3 and anti-IgG. Briefly, 2 ml of RIPA buffer (P0013B, Beyotime) supplemented with phosphatase inhibitors (F1 II-1082, Beyotime) and PMSF (ST505, Beyotime) was used to lyse 200 mg samples of the mouse testis tissue on ice for 40 min. Samples were then centrifuged for 20 min at 12 000g at 4°C. The supernatants were transferred to new tubes, with 50 μl reserved, and the remainder was incubated overnight with either anti-TEKT3 or anti-IgG at 4°C, followed by incubation for 3 h with protein A-Sepharose (GE, 17–1279-03) at 4°C. Precipitated samples were then rinsed twice using IP buffer (20 mM Tris, pH 7.4, containing 2 mM EGTA and 1% NP-40), after which sample buffer supplemented with 1% SDS was used to elute the immune complexes for 10 min at 95°C.
To identify the human TEKT3 binding partners, co-IP analyses were conducted using HEK293T cells that had been co-transfected with appropriate expression plasmids. For this approach, total RAN was isolated from samples of testicular tissue from a human patient with obstructive azoospermia and used to generate cDNA for TEKT1–5 using the primers listed in Supplementary Material, Table S3. The resultant amplicons were then inserted into BamHI restriction sites in the p3 × FLAG-CMV-24 vector. Lipofectamine 2000 (Invitrogen) was then used to co-transfect HEK293T cells with either individual plasmids or TEKT3 plasmids together with other expression plasmid constructs. On day 2 following transfection, cells were lysed for 40 min using RIPA buffer containing phosphatase inhibitors and PMSF as beforementioned, followed by centrifugation for 20 min at 12 000g. Then, 50 μl from each sample was reserved as input, while the remainder was incubated at 4°C overnight with anti-FLAG or anti-Myc. This was followed with an additional 3 h incubation with protein A-Sepharose (GE, 17–1279-03) at 4°C, and the precipitated samples were washed twice with IP buffer, and immune complex elution was performed as beforementioned.
Mass spectrometry
Following co-IP analyses of the mouse testes, protein samples were separated on gel electrophoresis, and a Fast Silver Stain Kit (p0017s, Beyotime) was used to detect the protein bands. Enzymatic gel hydrolysis was then used to extract target peptide fragments, which were then eluted, ionized and analyzed in tandem with an Orbitrap Fusion Lumos mass spectrometer. The raw mass spectral data were imported into Maxquant software for subsequent analyses.
Assisted reproductive treatment
Both probands and their respective partners, with consent, underwent ICSI procedures performed in the Reproductive Medicine Center, Department of Obstetrics and Gynecology at the First Affiliated Hospital of Anhui Medical University in Hefei, China, and the Affiliated Hospital of Xiangya, Central South University in China. Briefly, female partners in both couples were treated with 0.9–1.1 mg of long-acting GnRH antagonist (triptorelin, diphereline 3.75 mg; Ipsen Pharma Biotech; France) beginning on days 21–23 of the prior menstrual cycle. Every 2 days following recombinant-FSH injection (Gonal-F; Serono, Geneva, Switzerland), follicular development and plasma estradiol levels were monitored. When two or more follicles were at least 18 mm in diameter, a single injection of 10 000 IU human chorionic gonadotrophin (Livzon Pharmaceutical; China) was administered, and oocyte retrieval was performed after 36 h. A micromanipulator (Olympus, Tokyo, Japan) was used to select motile sperm and metaphase II stage oocytes for ICSI. All embryos were initially cryopreserved and were thawed for transplantation after 2 or more months had passed. At 12 weeks following each transfer, ultrasound analyses were conducted, with clinical pregnancy being confirmed by the observation of cardiac tube beating.
Acknowledgements
We would like to thank the families for participating and supporting this study and Ke Zheng from Nanjing medical university for the anti-TEKT5 antibody.
Conflict of Interest statement. The authors declare no conflict of interest with the publication of this article.
Data Availability
All data relevant to the study are included in the article or uploaded as supplementary information.
Ethics approval
This study received approval from the Institutional Review Boards of the First Affiliated Hospital of Anhui Medical University, the Affiliated Hospital of Xiangya and Central South University in China (Permit Number P2020-12-36). This study was consistent with the Declaration of Helsinki, and all patients provided consent to participate.
Funding
National Key R&D Program of China (2021YFC2700901); National Natural Science Foundation of China (32000584, 81901541 and 81971441); Natural Science Foundation of Anhui Province (2208085Y31 and 1908085QH313); University Synergy Innovation Program of Anhui Province (GXXT-2019-044); Nonprofit Central Research Institute Fund of the Chinese Academy of Medical Sciences (2019PT310002).
Author Contributions
Y.C., X.H. and Y.T. initiated the project. H.W. and R.H. designed the study. Y.T., H.W., L.M., Y.X., P.Z. and Z.W. provided patients’ data and performed clinical assessments. Y.L., Y.L. and L.M. performed most of the experiments. K.L. conducted the variant identification. Y.G., M.L. and R.G. performed some of the experiments. Y.L., H.W. and R.H. analyzed data. Y.L. drafted the initial manuscript. R.H. wrote and revised the manuscript. Y.C. and X.H. supervised the study. All authors read and approved the final manuscript.
References
Author notes
Yiyuan Liu, Yuqian Li and Lanlan Meng Contributed equally.