-
PDF
- Split View
-
Views
-
Cite
Cite
Monique Lillis, Nathan J Zaccardi, Maxwell G Heiman, Axon-dendrite and apical-basolateral sorting in a single neuron, Genetics, Volume 221, Issue 1, May 2022, iyac036, https://doi.org/10.1093/genetics/iyac036
- Share Icon Share
Abstract
Cells are highly organized machines with functionally specialized compartments. For example, membrane proteins are localized to axons or dendrites in neurons and to apical or basolateral surfaces in epithelial cells. Interestingly, many sensory cells—including vertebrate photoreceptors and olfactory neurons—exhibit both neuronal and epithelial features. Here, we show that Caenorhabditis elegans amphid neurons simultaneously exhibit axon-dendrite sorting like a neuron and apical-basolateral sorting like an epithelial cell. The distal ∼5–10 µm of the dendrite is apical, while the remainder of the dendrite, soma, and axon are basolateral. To determine how proteins are sorted among these compartments, we studied the localization of the conserved adhesion molecule SAX-7/L1CAM. Using minimal synthetic transmembrane proteins, we found that the 91-aa cytoplasmic tail of SAX-7 is necessary and sufficient to direct basolateral localization. Basolateral localization can be fully recapitulated using either of 2 short (10-aa or 19-aa) tail sequences that, respectively, resemble dileucine and Tyr-based motifs known to mediate sorting in mammalian epithelia. The Tyr-based motif is conserved in human L1CAM but had not previously been assigned a function. Disrupting key residues in either sequence leads to apical localization, while “improving” them to match epithelial sorting motifs leads to axon-only localization. Indeed, changing only 2 residues in a short motif is sufficient to redirect the protein between apical, basolateral, and axonal localization. Our results demonstrate that axon-dendrite and apical-basolateral sorting pathways can coexist in a single cell, and suggest that subtle changes to short sequence motifs are sufficient to redirect proteins between these pathways.
Introduction
A cell presents many faces to the world: for example, the outward-facing (apical) surface of an epithelial cell is decorated with transmembrane proteins that are different from those on its inward-facing (basolateral) surface, reflecting the specialized functions of each compartment. To achieve these cell-surface differences, many transmembrane proteins contain sequences that are recognized by sorting machinery in the secretory pathway in order to direct the proteins to their appropriate destinations. Classical studies identified short cytoplasmic sequences that are responsible for basolateral sorting, namely dileucine ([D/E]xxxL[L/I]) and tyrosine (Tyr)-based motifs (YxxΦ or NPxY) (Duffield et al. 2008). However, as these studies were conducted in homogeneous cultured epithelial cells, it remains unclear how proteins are trafficked in vivo in cells with more complex organization.
Neurons are highly compartmentalized cells whose major surfaces—axons and dendrites—have different protein compositions. Initial studies suggested that neurons use the same sorting mechanisms as epithelial cells because epithelial proteins with known basolateral motifs localized to dendrites when mis-expressed in neurons (Bentley and Banker 2016). However, further investigation revealed a more complicated relationship, with some basolateral proteins localizing uniformly to axons and dendrites rather than in a polarized manner (Silverman et al. 2005). In principle, neuronal proteins are likely to require more complex trafficking mechanisms, as they are often localized to specific subcompartments within axons (the axon initial segment, nodes of Ranvier, or presynaptic sites) or dendrites (proximal or distal branches or specific classes of postsynaptic spines) (Donato et al. 2019). Previous work has identified a wide variety of mechanisms for polarized localization of neuronal proteins, including local translation, transcytosis, selective retention, and many others (Bentley and Banker 2016). Defining the relationship between the mechanisms that govern protein sorting in neurons and epithelial cells may reveal how such complicated subcellular organization evolved from simple apical-basolateral polarity.
Recently, we discovered that sensory neurons in Caenorhabditis elegans exhibit epithelial characteristics including apical-basolateral polarity (Low et al. 2019), providing a unique opportunity to directly compare apical-basolateral and axon-dendrite sorting in a single cell. Here, by analyzing the localization of a minimal synthetic transmembrane protein in a single sensory neuron in vivo, we identify 2 short cytoplasmic sequences that are sufficient to direct basolateral localization. These basolateral sequences contain Ile-Leu and Tyr residues that are required for their function, but they do not conform to typical dileucine or Tyr-based motifs. Surprisingly, changing these sequences to typical dileucine or YxxΦ motifs re-directs their localization from basolateral to axon-only. In the most striking example, we find that changing just 2 residues in a short cytoplasmic sequence is sufficient to redirect a synthetic transmembrane protein between 3 cellular compartments (Ile-Leu, basolateral; Ala-Ala, apical; Leu-Leu, axon only). The Tyr motif is highly conserved in human L1CAM and the human sequence is also sufficient for basolateral localization in C. elegans, suggesting it represents a conserved regulatory sequence. Taken together, our results show that variants of typical dileucine and Tyr-based motifs are sufficient to switch transmembrane proteins between basolateral and axon localization, and suggest a way in which typical sorting signals from epithelia may have diversified to allow more complex multicompartment sorting in neurons.
Materials and methods
Strains and plasmids
C. elegans strains were maintained at 20°C on nematode growth medium (NGM) plates with Escherichia coli OP50 bacteria (Brenner 1974). All transgenic strains were generated in the N2 background using standard methods (Mello et al. 1991) with injection of 100 ng/µl total DNA (25–75 ng/µl per plasmid). Strains, alleles, and plasmids in this study are listed in Supplementary Tables 1–3, respectively.
Image acquisition and analysis
Fourth larval stage (L4) animals were mounted on 2% agarose pads in 50 mM sodium azide with a No. 1.5 coverslip. Image stacks were collected on a DeltaVision Core deconvolution imaging system (Applied Precision) with the InsightSSI light source; UApo 40x/1.35 NA oil immersion objective, PlanApo 60x/1.42 NA oil immersion objective, or UPlanSApo 100x/1.40 NA oil immersion objective (Olympus); the standard DeltaVision live cell excitation and emission filter set; and a Photometrics CoolSnap HQ2 CCD camera (Roper Scientific). Image stacks were acquired and deconvolved with Softworx 5.5 (Applied Precision). Maximum intensity projections were generated with contiguous optical sections in ImageJ (Fiji), then linearly adjusted for brightness in Adobe Photoshop CS5. Multicolored images were created by placing each channel in a separate false-colored screen layer in Photoshop. Figures were assembled in Adobe Illustrator CS5.
Quantification of polarity index
Quantification of polarity index was performed on strains expressing a protein tagged with sfGFP and the tight junction marker AJM-1-mApple, which was used to identify the boundary between apical and basolateral regions. Unprocessed images were analyzed using Image J (NIH). The brightest point of AJM-1-mApple signal at the dendrite ending was identified and 5 optical sections above and below that image plane were used to create an average intensity projection consisting of 11 total contiguous optical sections. The integrated pixel intensity was measured using a 1.5 µm line positioned parallel to and beside the dendrite ending (noise, N), in the region of the dendrite distal to the AJM-1-mApple signal (apical, A), or proximal to the AJM-1-mApple signal (basolateral, B). A one-pixel-wide line was chosen to minimize variation in measurements due to the morphology of the dendrite ending. The polarity index was quantified as (B-N)/[(B-N)+(A-N)] (Supplementary Fig. 1). In animals with no apical localization, the background noise was sometimes greater than the apical fluorescence (N > A), resulting in a polarity index >1. In these cases, the polarity index was set to 1, and the mean and standard deviation of the population were calculated following this correction. The Wilcoxon Rank-Sum test was used to calculate P-values. Graphs were generated in Microsoft Excel and color adjusted in Adobe Illustrator CS5.
Results
Amphid neurons exhibit both axon-dendrite and apical-basolateral polarity
Sensory neurons in the amphid, the major sense organ of C. elegans, exhibit morphological features of both neurons and epithelial cells (Low et al. 2019). As neurons, they have a dendrite that receives sensory information from the nose tip and an axon that transmits information to the “brain” (nerve ring) (Fig. 1a) (Ward et al. 1975). However, like epithelial cells, they also have an apical surface that is directly exposed to the external environment and a basolateral surface that is exposed to the internal environment (pseudocoelom) (Fig. 1a) (Ward et al. 1975). The apical surface consists of the sensory cilium and the terminal ∼5 µm of the dendrite and is separated by tight junctions from the basolateral surface which consists of the remaining ∼90 µm of the dendrite as well as the cell body and axon (Fig. 1a) (Ward et al. 1975).
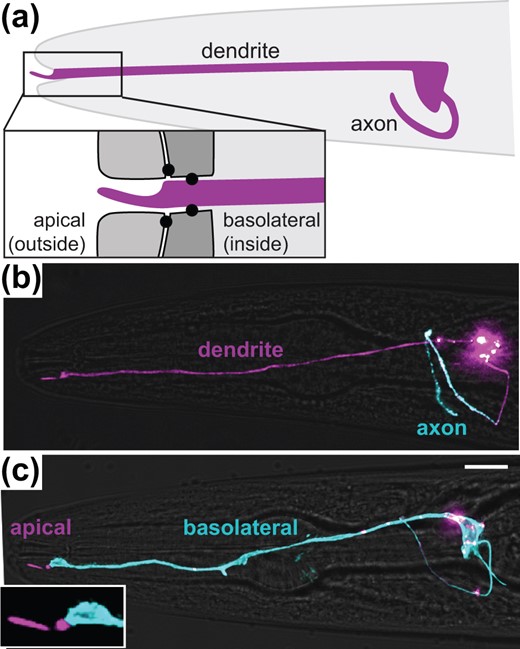
Amphid neurons exhibit both axon-dendrite and apical-basolateral polarity. a) Schematic showing the axon and dendrite of a single amphid neuron (ASER, purple) in the head (gray, anterior drawn to the left). The dendrite ending protrudes through a glial channel and terminates in a sensory cilium that is exposed to the external environment. Inset shows tight junctions (black circles) that separate an outward-facing apical region from an inward-facing basolateral region. b, c) Polarized localization of proteins exclusively to (b) the axon (sfGFP-TM-SER-1, blue) or (c) the basolateral surface (SAX-7-sfGFP, blue). The entire cell is labeled with myristyl-mCherry (purple). Scale bar, 10 µm.
To determine whether there are in fact molecular differences between these compartments, we expressed GFP-tagged axonal or basolateral membrane proteins and a membrane-targeted cell surface marker (myristyl-mCherry) using a promoter specific to a single amphid neuron [gcy-5pro, ASER neuron (Yu et al. 1997)]. We found that the serotonin receptor SER-1 localizes to the axon and is excluded from the dendrite (Fig. 1b), consistent with previous studies in other neuron types (Li et al. 2016). In contrast, the cell adhesion molecule SAX-7 localizes to the basolateral surface (Fig. 1c) and is excluded from the apical surface, consistent with our previous observations in the amphid and other studies in epithelia (Chen et al. 2001; Grana et al. 2010; Low et al. 2019). Thus, amphid neurons appear to simultaneously perform axon-dendrite sorting like a neuron, as well as apical-basolateral sorting like an epithelial cell. This raises the question of how these cells maintain the proper composition of membrane proteins in each cellular compartment.
The SAX-7 cytoplasmic tail is necessary and sufficient for basolateral sorting
Unlike axon-dendrite sorting, apical-basolateral sorting has not been explored in the context of neurons. To identify protein sequences required for basolateral sorting, we used SAX-7 as a model basolateral transmembrane protein. SAX-7 is a single-pass Type I transmembrane protein that consists of a 1,028-aa ectodomain containing adhesive immunoglobulin (Ig) domains and Fibronectin Type III (FN) domains; a 25-aa transmembrane (TM) segment; and a 91-aa cytoplasmic tail containing protein–protein interaction motifs (Chen et al. 2001). Its homologs include Drosophila Neuroglian, avian NgCAM, and the mammalian proteins L1CAM, CHL1, NrCAM, and Neurofascin (Mualla et al. 2013). SAX-7 has been most extensively studied in the context of neuronal adhesion but it is expressed both in neurons and epithelia (Chen et al. 2001).
First, we developed a method to visualize the polarized apical-basolateral localization of SAX-7. We expressed AJM-1-mApple to mark the position of tight junctions, which create a diffusion barrier separating apical and basolateral surfaces, together with GFP-tagged SAX-7 in a single amphid neuron. SAX-7 localizes throughout the basolateral surface of the neuron but does not extend past the tight junction (Fig. 2, a–c). Because the dendrite is essentially a cylinder of constant diameter, this imaging approach allowed us to quantify the relative concentration of SAX-7 in the apical and basolateral compartments by measuring the fluorescence intensity per unit length (see Materials and Methods). We measured the fluorescence intensity in 1.5 µm apical (A) and basolateral (B) segments flanking the tight junction (Supplementary Fig. 1) and defined a polarity index , such that a polarity index of 1 indicates exclusively basolateral localization and a polarity index of 0 indicates exclusively apical localization. The polarity index of SAX-7 is 0.93 ± 0.08 (mean ± SD), reflecting its basolateral localization.
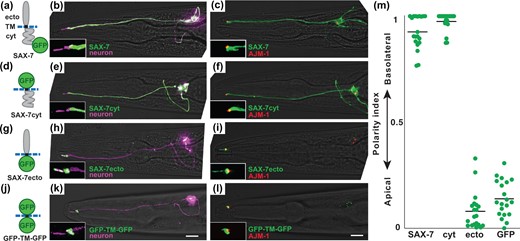
The SAX-7 cytoplasmic tail is necessary and sufficient for basolateral localization. Localization of sfGFP-tagged proteins containing (a–c) full-length SAX-7, (d–f) the SAX-7 cytoplasmic tail, (g–i) the SAX-7 extracellular domain, or (j–l) neither. a, d, g, j) Schematics of the protein variants that were tested. ecto, extracellular region; TM, transmembrane; cyt, cytoplasmic region. Blue dashed line represents the membrane. Localization of each protein (green) in ASER is shown relative to (b, e, h, k) the entire cell labeled with myristyl-mCherry (purple) or (c, f, i, l) tight junctions labeled with AJM-1-mApple (red). Apical proteins differ in the extent of entry into the sensory cilium. Puncta in the cell body may represent newly synthesized protein at an early stage in the secretory pathway. Scale bar, 10 µm. m) The polarity index of each protein was quantified as described in the text and Supplementary Fig. 1. Colored bars represent individual animals; black bars represent the population average. Mean polarity index ± SD: SAX-7, 0.93 ± 0.08; cyt, 0.98 ± 0.04; ecto, 0.08 ± 0.09 (P < 0.0001); GFP, 0.14 ± 0.09 (P < 0.0001). n = 20 for each protein.
The basolateral localization of SAX-7 might be directed by its ectodomain or cytoplasmic tail, both of which contribute to trafficking of the chick homolog NgCAM (Sampo et al. 2003; Yap et al. 2008). The ectodomain could interact with adhesion molecules on neighboring cells and thus selectively stabilize SAX-7 at the basolateral surface, while the cytoplasmic tail could interact with vesicle trafficking proteins in the secretory pathway or with anchoring proteins at the basolateral surface. To assess the role of the ectodomain and the cytoplasmic tail, we examined the localization of SAX-7 variants in which the adhesive Ig and FN domains, the cytoplasmic tail, or both were replaced with GFP (Fig. 2, d, g, and j). SAX-7cyt, in which the Ig and FN domains are deleted, exhibits basolateral localization comparable to that of the full-length protein (Fig. 2, e, f, and m). In contrast, SAX-7ecto, in which the cytoplasmic tail is deleted, localizes apically and is excluded from the basolateral region (Fig. 2, h, i, and m). As a control, GFP-TM-GFP, in which both the ectodomain and cytoplasmic tail are replaced with sfGFP, also localizes apically (Fig. 2, k–m). Despite both localizing apically, SAX-7ecto enters the sensory cilium while GFP-TM-GFP is restricted to the periciliary compartment, which sometimes appeared enlarged, affecting the polarity index (SAX-7ecto, 18 of 20 in cilium; GFP-TM-GFP, 0 of 20 in cilium). The reasons for this difference are not known. Overall, these results suggest that apical localization occurs by default in the absence of sorting information and that the SAX-7 cytoplasmic tail is necessary and sufficient for basolateral localization.
It is surprising that apical, rather than uniform localization across the cell surface, is the default. This may reflect the bulk flow of the secretory pathway in these cells, consistent with the presence of a vesicle-rich compartment at the dendrite ending. Alternatively, because the apical surface of amphid neurons is ∼20x smaller than the basolateral surface, randomized 1:1 sorting of a protein into apical- or basolateral-directed vesicles would yield an apically enriched concentration of ∼20:1, consistent with the observed polarity index of ∼0.05 (Fig. 2m, “ecto”). In this simple model of protein sorting, the SAX-7 cytoplasmic tail would need to exhibit a remarkable 400-fold bias for basolateral-directed vesicles to achieve the observed polarity index of ∼0.95 (see schematic, Supplementary Fig. 1b). Thus, our observations suggest the presence of potent basolateral localization signals in the cytoplasmic tail.
Known motifs in the SAX-7 tail are not required for basolateral sorting
We dissected the GFP-TM-SAX-7 cytoplasmic tail protein (Fig. 2d) to identify sequences required for basolateral localization in a sensory neuron. The SAX-7 cytoplasmic tail contains 3 previously defined protein interaction motifs, called FERM-binding (FB), ankyrin-binding (AB), and PDZ-binding (PB) motifs (Fig. 3a) (Zhou et al. 2008). The FB and AB motifs are predicted to interact with cytoskeletal proteins such as NFM-1 and UNC-44/ankyrin, respectively, each of which localizes basolaterally in epithelial cells (Chen et al. 2001; Göbel et al. 2004), while the PB motif interacts with PDZ-domain scaffolding proteins, some of which promote basolateral anchoring of other transmembrane proteins in C. elegans epithelial cells (Kaech et al. 1998; Rongo et al. 1998). Thus, each of these motifs could mediate protein interactions that would contribute to SAX-7 basolateral localization.
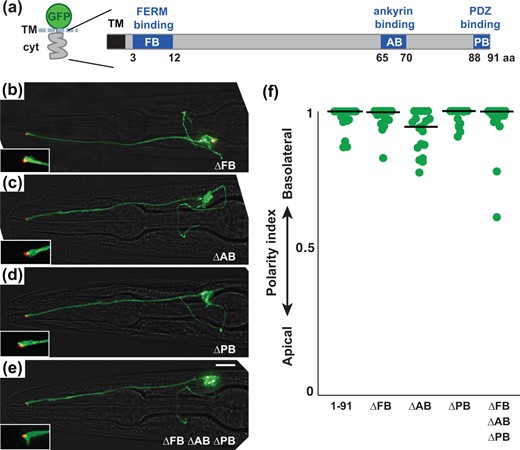
Known motifs in the SAX-7 tail are not required for basolateral localization (a) Schematic of the SAX-7 cytoplasmic tail (cyt) showing known motifs. FB, FERM-binding; AB, ankyrin-binding; PB, PDZ-binding. b–e) Localization of sfGFP-TM-cyt proteins in which these motifs were deleted individually or in combination. sfGFP-tagged proteins, green; tight junction (AJM-1-mApple), red. Scale bar, 10 µm. f) The polarity index of each protein, as in Fig. 2: sfGFP-TM-cyt (1–91), 0.98 ± 0.04; ΔFB, 0.98 ± 0.04; ΔAB, 0.93 ± 0.07; ΔPB, 0.98 ± 0.03; ΔFB ΔAB ΔPB, 0.96 ± 0.09. n = 20 for each protein.
To determine if any of these motifs contribute to the basolateral localization of SAX-7, we deleted each motif from the cytoplasmic tail individually (ΔFB, ΔAB, ΔPB) or in tandem (ΔFB ΔAB ΔPB). Surprisingly, we found that each of the resulting protein variants exhibits basolateral localization comparable to the full-length SAX-7 tail (1-91) (Fig. 3), suggesting that previously defined physical interactions with partner proteins are not required for SAX-7 localization. Furthermore, we found that soluble and membrane-tethered (myristylated) forms of the wild-type SAX-7 tail localize uniformly across the cell (Supplementary Fig. 2), suggesting that interactions with proteins at the basolateral surface are not sufficient to localize the SAX-7 tail through a selective retention mechanism. Instead, basolateral localization is likely to involve novel motifs in the SAX-7 tail and may require sorting events that occur during transit through the secretory pathway.
The SAX-7 cytoplasmic tail contains 2 independent basolateral sorting sequences
Because the SAX-7 tail does not contain canonical dileucine ([D/E]xxxL[L/I]) or Tyr-based (YxxΦ or NPxY) basolateral sorting motifs (Fig. 4a), we reasoned that it might use other sequences for basolateral sorting. To identify the relevant sequences, we divided the SAX-7 tail into three ∼30-aa fragments, expressed them as fusions to a GFP-TM scaffold, and quantified the polarity index of each (Fig. 4a). We designed the fragments to avoid breaking up blocks of conserved sequence (Fig. 4a, purple), which are mostly limited to the FB and AB motifs and an uncharacterized motif from residue 47 to 64. We found that fragments consisting of residues 1–29 or 30–64 exhibit basolateral localization equivalent to that of the full-length tail (Fig. 4, b, c and e), suggesting the presence of 2 independent basolateral sorting sequences. It is important to note that our experiments test the sufficiency of isolated sequences to confer localization to a minimal transmembrane protein, and more complex regulation may exist in the full-length protein.
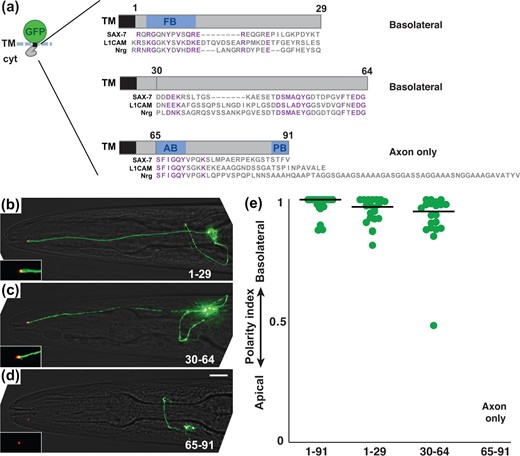
Two regions of the SAX-7 cytoplasmic tail are each sufficient for basolateral localization. a) Schematic of sfGFP-TM fusions to fragments that correspond to residues 1–29, 30–64, or 65–91 of the SAX-7 cytoplasmic tail. In all cases, the 4-aa sequence RQRG was left in place to avoid disturbing juxtamembrane charge distribution that could affect insertion topology. The sequence of each fragment is shown with an alignment to human L1CAM and Drosophila Neuroglian (Nrg). Conserved sequences are in purple. Note the conserved sequences in the second fragment that do not correspond to a known protein binding motif. A summary of the observed localization is shown. b–d) Localization of each protein. sfGFP-tagged proteins, green; tight junction (AJM-1-mApple), red. Scale bar, 10 µm. e) The polarity index of each protein, as in Fig. 2: 1-91, 0.93 ± 0.08; 1-29, 0.95 ± 0.06; 30-64, 0.92±0.12. n = 20 for each protein. A polarity index was not obtained for the 65–91 fragment because it localized exclusively to the axon.
Surprisingly, the carboxy-terminal region consisting of residues 65–91 exhibits axon-only localization (Fig. 4d). Axon localization requires the AB and PB motifs, as deletion of either motif leads to increased apical localization, and deletion of both together (ΔAB ΔPB) leads to almost exclusively apical localization (Supplementary Fig. 3). Notably, the AB motif in SAX-7 and other L1 family proteins undergoes phosphorylation, with phosphorylated and nonphosphorylated forms localizing differently (Garver et al. 1997; Chen et al. 2001). The presence of multiple independently acting motifs within these sequences may represent additional points of regulation that coordinately affect localization.
Changes to an Ile-Leu motif convert basolateral localization to apical or axon-only
To further define the basolateral sorting sequence in residues 1–29, we systematically tested smaller fragments from this region (Fig. 5a). We designed the fragments to avoid breaking up the FB motif. We found that fragments ending in EPIL (1–22 and 13–22) were expressed at nearly undetectable levels but could be stabilized by adding a synthetic 5-aa carboxy-terminus from GFP (DELYK). Through these experiments, we defined a 10-aa fragment (EREQGREPIL, 13–22) that is sufficient to confer basolateral localization (Fig. 5, a and c).
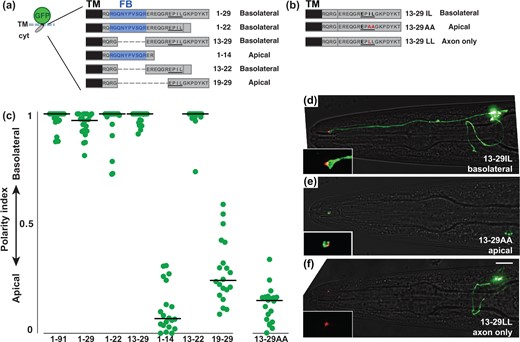
An Ile-Leu motif directs basolateral localization. a, b) Schematics showing cytoplasmic sequences that were fused to a sfGFP-TM scaffold. For each protein, the corresponding residues of the SAX-7 tail and its localization are given. The FERM-binding (FB) motif is in blue and the dileucine-like sequence EPIL is underlined. Internal deletions, dashed lines. Because proteins ending in EPIL (1–22, 13–22) were expressed at nearly undetectable levels, the carboxy-terminus of GFP (DELYK) was added to stabilize expression. b) The dileucine-like sequence EPIL is underlined. Mutated residues, red. c) The polarity index for each protein, as in Fig. 2: 1–91, 0.93 ± 0.08; 1–29, 0.95 ± 0.05; 13–29, 0.98 ± 0.03; 1–14, 0.11±0.11 (P < 0.0001, compared to 1–29); 13-22, 0.99 ± 0.07; 19–29, 0.28 ± 0.15 (P < 0.0001, compared to 1–29); 13-29AA, 0.13 ± 0.08 (P < 0.0001, compared to 13–29). n = 20 for each protein. d–f) Localization of proteins containing (d) the wild-type Ile-Leu (basolateral), (e) mutant Ala-Ala (apical), or (f) mutant Leu-Leu (axon only). sfGFP-tagged proteins, green; tight junction (AJM-1-mApple), red. Scale bar, 10 µm. Puncta in the cell body in (e) may represent newly synthesized protein at an early stage in the secretory pathway.
Our attention was drawn to the sequence EPIL, which is reminiscent of the canonical dileucine motifs LL or [DE]xxxL[LI] (Matter et al. 1994; Höning et al. 1998). Although the EPIL sequence is not sufficient for basolateral localization when positioned close to the TM (Fig. 5a, 19–29), it is shared by all basolaterally localized fragments (Fig. 5, a and c). We hypothesized that the EPIL sequence might act as a basolateral sorting signal in amphid neurons, similar to canonical dileucine motifs in epithelial cells.
To test this hypothesis, we altered the EPIL sequence to abolish (EPAA) or improve (EPLL) its similarity to a typical dileucine motif (Fig. 5b). We also tested a cytoplasmic tail with a well-characterized dileucine motif (ExxxLL) from the HIV Nef protein (Supplementary Fig. 4a) (Bresnahan et al. 1998). We found that abolishing the Ile-Leu motif resulted in apical localization (Fig. 5, c and e) while, surprisingly, improved or canonical versions of the motif led to axon-only localization (Fig. 5f, Supplementary Fig. 4a). By contrast, abolishing the Ile-Leu motif in the context of full-length SAX-7 did not affect localization, consistent with the presence of redundant localization signals in the tail (Supplementary Fig. 4b). Thus, in the context of this 17-aa sequence, the Ile-Leu residues are required to confer basolateral localization while a typical Leu-Leu motif confers axon-only localization. A limitation of our approach is that we cannot definitively distinguish what fraction of the observed protein localization is at the cell surface or in vesicles. Nevertheless, it is striking that altering just 2 residues (IL, AA, or LL) is sufficient to switch protein localization between 3 compartments—basolateral, apical, or axon-only (Fig. 5, d–f).
A conserved Tyr-based sequence promotes basolateral localization
Next, to define the basolateral sorting sequence present in residues 30–64, we inspected this sequence for similar dileucine-like motifs. We identified a putative dileucine-like motif of the form ExxxL (EKRSL, Fig. 6a), however, this sequence is not required for basolateral localization (41–64 and 46–64, Fig. 6, a and b). In addition, a smaller fragment containing this sequence localizes to the axon (30–54, Fig. 6a) similar to the HIV Nef sequence (ExxxLL, Supplementary Fig. 4), further suggesting that it is not a basolateral sorting signal. Rather, we noted a highly conserved bipartite sequence (DSMAQYG…FTEDG, Fig. 4a) that has no previously known function and found that it is sufficient for basolateral localization (46–64, Fig. 6, a, c, and d). The homologous 19-aa sequence from human L1CAM also confers basolateral localization when expressed in amphid neurons (L1CAM, Fig. 6, a and c), suggesting that this sequence represents a novel conserved sorting signal.
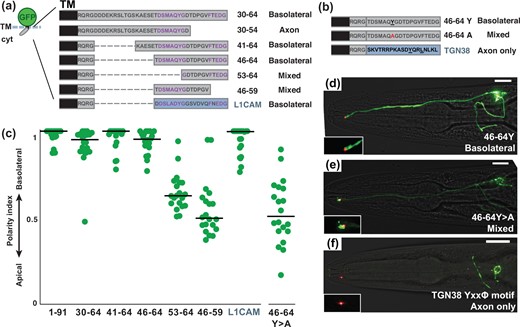
A conserved Tyr motif also promotes basolateral localization. a, b) Schematics showing cytoplasmic sequences that were fused to a sfGFP-TM scaffold. For each protein, the corresponding residues of the SAX-7 tail and its localization are given. A homologous 19-aa sequence from the human L1CAM cytoplasmic tail was also tested, as shown. Conserved sequences are in purple. In all cases, the juxtamembrane sequence RQRG was left in place to avoid disrupting membrane topology. Internal deletions, dashed lines. b) Mutation, red. A 19-aa sequence with a well-characterized Tyr-based motif (YxxΦ) from mouse TGN38, as shown, was also tested. c) The polarity index for each protein, as in Fig. 2: 1–91, 0.93 ± 0.08; 30–64, 0.92 ± 0.12; 41–64, 0.96 ± 0.07; 46–64, 0.94 ± 0.07; 53–64, 0.65 ± 0.11 (P < 0.0001, compared to 30–64); 46–59, 0.53 ± 0.14 (P < 0.0001, compared to 30–64); L1CAM, 0.94 ± 0.08; 46–64Y>A, 0.53 ± 0.18 (P < 0.0001, compared to 46–64). n = 20 for each protein except n = 19 for 46–59. d–f) Localization of proteins containing (d) the wild-type Tyr (basolateral), (e) mutant Ala (mixed; the individual shown here is apically enriched with polarity index = 0.31), or (f) canonical YxxΦ motif (axon only). sfGFP-tagged proteins, green; tight junction (AJM-1-mApple), red. Scale bar, 10 µm.
Smaller fragments lacking the DSMAQYG or FTEDG sequences localize in a mixed apical-basolateral manner (53–64 and 46–59, respectively; Fig. 6, a and c), suggesting that both halves of this sequence contribute to its basolateral localization. Because Tyr-based sequences are important basolateral sorting signals (YxxΦ or NPxY), we tested the role of Tyr in this sequence (Y > A) and found that it is required for basolateral localization (Fig. 6, b–e). Finally, we tested a well-characterized Tyr-based motif [YQRL, or YxxΦ, from the mammalian protein TGN38 (Owen and Evans 1998)] and found that it confers axon-only localization (Fig. 6, b and f). Together, our results suggest a surprising relationship between motifs that confer apical-basolateral or dendrite-axon sorting in a multicompartment neuron: variant Ile-Leu and Tyr-based motifs are required for basolateral sorting whereas, unexpectedly, typical Leu-Leu and Tyr-based motifs result in sorting to the axon.
Discussion
Our results suggest that short cytoplasmic sequence tags are sufficient to re-direct transmembrane proteins between at least 3 distinct sorting pathways in multicompartment amphid neurons (Fig. 7). Transmembrane proteins lacking a sorting motif traffic to the apical region, which is thus the default pathway. Proteins with a typical dileucine or Tyr-based motif (ExxxLL, YxxΦ) traffic to the axon. Variants of these motifs direct proteins to the basolateral region, which includes most of the dendrite, the soma, and the axon. We have not identified any examples of transmembrane proteins that localize exclusively to amphid dendrites. These sorting pathways may be shared by other neurons that reside in epithelia, such as vertebrate olfactory neurons and photoreceptors. Furthermore, the general principle that variant motifs confer localization to distinct subcompartments could apply broadly across many cell types.
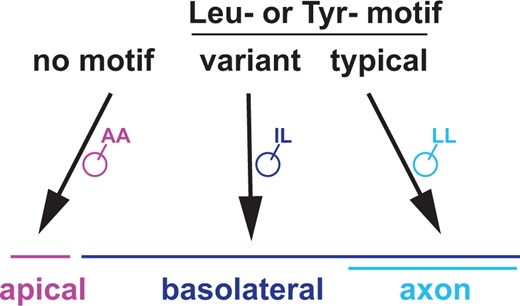
Three sorting pathways in multicompartment neurons. Summary model showing sorting between apical, basolateral, and axonal regions in a multicompartment neuron. Proteins with no motif localize to the apical region, which can thus be considered a default pathway. Proteins with variant Leu- or Tyr-based motifs localize throughout the basolateral region, including the axon. Proteins with canonical Leu- or Tyr-based motifs localize exclusively to the axon.
It is surprising that a change as subtle as Ile>Leu profoundly alters protein localization. How are otherwise identical Ile-Leu and Leu-Leu motifs discriminated? In principle, they could bind to different sorting receptors or to the same receptor with different affinity. Experiments using combinations of Ile-Leu and Leu-Leu motifs or concatenated Ile-Leu motifs may help to resolve this. Leu-Leu motifs are typically recognized by the cytoplasmic clathrin adaptor protein complexes AP1, AP2, and AP3 (Heilker et al. 1999). We found that a mutant in the AP1 µ1 subunit unc-101 exhibits a complete loss of polarization for our apical, basolateral and axon localized proteins, suggesting an overall loss of neuronal polarity (Supplementary Fig. 5). While this is consistent with observations from previous studies (Dwyer et al. 2001; Li et al. 2016), it precluded us from determining if UNC-101 plays a direct role in sorting the motifs we identified. By contrast, mutants affecting the AP3 β1/β2 subunit apb-3 showed no change in sorting of our apical, basolateral or axon localized proteins (Supplementary Fig. 5). Identifying the sorting receptors that directly bind the SAX-7 motifs will be key to understanding how subtle differences in sequence lead to profound changes in localization.
The motifs we identified can be compared to a previous study that examined a C. elegans neuron that is not part of an epithelium and does not exhibit apical-basal polarity (Li et al. 2016). Briefly, the RIA interneuron is unipolar, with a single neurite that contains regions that are more enriched for presynaptic or postsynaptic markers and can thus be considered axonal or dendritic, respectively. Li and colleagues examined the localization of 2 transmembrane proteins, SER-1 and CAM-1, in RIA (Li et al. 2016). Using GFP-tagged proteins with long cytoplasmic tails (223-aa or 155-aa tails for SER-1; a 438-aa tail for CAM-1), they found that SER-1 contains a single Leu-Ile motif (ExxxLI) that is necessary for axon localization while CAM-1 contains 3 Leu-Leu motifs (ExxxLL, DxxLL, and DxxxLL) that are required in combination for dendrite localization—that is, disrupting any single motif abolishes dendrite localization—although paradoxically any one of these motifs on its own is sufficient to confer dendrite localization when transplanted into the SER-1 tail (Li et al. 2016). The SER-1 and CAM-1 dileucine motifs are differentially recognized by AP3 and AP1, respectively (Li et al. 2016). In comparing these findings to our results, a shared theme is that subtle differences in dileucine-like motifs can dramatically affect localization. However, the logic of which motif localizes to which region is different: whereas in RIA the SER-1 Leu-Ile motif localizes to axons and the Leu-Leu motif to dendrites, in amphid neurons the SER-1 tail and Leu-Leu motifs both localize to axons (Figs. 1b and 5f, and Supplementary Fig. 4). Further, the AP3 subunit apb-3 is required for axon localization in RIA (Li et al. 2016) but not in amphid neurons (Supplementary Fig. 5). Thus, both studies support the idea that axon-dendrite sorting is driven by variants of canonical motifs, but the sorting rules themselves may be flexible and appear to differ even between closely related cell types. This flexibility may be important for the evolution of new subcellular compartments.
Intriguingly, mammalian homologs of SAX-7 (L1CAM, CHL1, NrCAM, and Neurofascin) exhibit complex compartment-specific localization in neurons and glia. In axons, L1CAM is distributed along the axon length while NrCAM and Neurofascin localize to nodes of Ranvier (Lambert et al. 1997; Lustig et al. 2001). Neurofascin also localizes to the axon initial segment of Purkinje neurons, where it guides subcellular patterns of synaptic innervation (Ango et al. 2004). In dendrites, CHL1 and NrCAM localize to distinct populations of postsynaptic spines and differentially regulate spine stability (Mohan et al. 2019). In Bergmann glia, NrCAM localizes to lamellar glial processes that wrap Purkinje neuron cell bodies while CHL1 localizes to the radial fibers of these glia (Ango et al. 2008). These localization patterns underscore the idea that axon-dendrite sorting is only 1 layer of neuronal polarity, and in fact most neurons and glia are complex multicompartment cells. Specialized subcellular localization patterns have been observed for other adhesion molecules as well, and can vary depending on cellular and developmental context. Our identification of multiple independently acting localization motifs in SAX-7, and their similarities and differences from typical motifs, offers a possible explanation for how compartment-specific sorting signals may have evolved. Variant motifs may provide independent points of regulation that act in a context-dependent manner to direct a transmembrane protein to the appropriate destination within multicompartment neurons and glia.
Data availability
Strains and plasmids are available upon request. The authors affirm that all data necessary for confirming the conclusions of the article are present within the article, figures, and tables.
Supplemental material is available at GENETICS online.
Acknowledgments
The authors thank Kang Shen, Erik Jorgensen, and the C. elegans Genetics Center [CGC, funded by NIH Office of Research Infrastructure Programs (P40 OD010440)] for plasmids and strains. They thank Pascal Kaeser, Josh Kaplan, Jonah Chan, Elizabeth Cebul, Wendy Fung, Karolina Mizeracka, and Leigh Wexler for comments.
Funding
This work was supported by National Institutes of Health R01GM108754 and R01NS112343 to MGH.
Conflicts of interest
None declared.