-
PDF
- Split View
-
Views
-
Cite
Cite
Alexandra E DeSarno, Benjamin J Parcell, Peter J Coote, Repurposing the anti-viral drug zidovudine (AZT) in combination with meropenem as an effective treatment for infections with multi-drug resistant, carbapenemase-producing strains of Klebsiella pneumoniae, Pathogens and Disease, Volume 78, Issue 9, December 2020, ftaa063, https://doi.org/10.1093/femspd/ftaa063
- Share Icon Share
Abstract
Multi-drug resistant (MDR) Klebsiella pneumoniae represent a global threat to healthcare due to lack of effective treatments and high mortality rates. The aim of this research was to explore the potential of administering zidovudine (AZT) in combination with an existing antibiotic to treat resistant K. pneumoniae infections. Two MDR K. pneumoniae strains were employed, producing either the NDM-1 or KPC-3 carbapenemase. Efficacy of combinations of AZT with meropenem were compared with monotherapies against infections in Galleria mellonella larvae by measuring larval mortality and bacterial burden. The effect of the same combinations in vitro was determined via checkerboard and time-kill assays. In vitro, both K. pneumoniae strains were resistant to meropenem but were susceptible to AZT. In G. mellonella, treatment with either AZT or meropenem alone offered minimal therapeutic benefit against infections with either strain. In contrast, combination therapy of AZT with meropenem presented significantly enhanced efficacy compared to monotherapies. This was correlated with prevention of bacterial proliferation within the larvae but not elimination. Checkerboard assays showed that the interaction between AZT and meropenem was not synergistic but indifferent. In summary, combination therapy of AZT with meropenem represents a potential treatment for carbapenemase-producing MDR K. pneumoniae and merits further investigation.
INTRODUCTION
Klebsiella pneumoniae belongs to the family Enterobacteriaceae and is part of the normal intestinal flora of healthy humans. However, K. pneumoniae is also an important opportunistic pathogen and is a major cause of community and healthcare associated infections (HAIs) such as bloodstream, urinary tract infections and pneumonias (Navon-Venezia, Kondratyeva and Carattoli 2017). Infection is often associated with invasive medical devices or surgery in patients that are immunocompromized [reviewed in (Peleg and Hooper 2010)]. Compounding the problem, K. pneumoniae is adept at acquiring genes encoding a range of antibiotic resistance mechanisms via either mutations or mobile genetic elements (Navon-Venezia, Kondratyeva and Carattoli 2017). Acquisition of resistance to third generation cephalosporins via extended-spectrum beta-lactamases (ESBLs) led to increased use of the antibiotics of ‘last-resort’—the carbapenem class of broad-spectrum beta-lactams—resulting in the inevitable selection of resistance to these also. With increasing incidence of resistance to carbapenems, the prevalence of multi-drug, or extremely-drug resistant (MDR and XDR, respectively), K. pneumoniae strains that are resistant to nearly all available antibiotics is rising globally (Sanchez et al. 2013). Consequently, there are only a limited number of antibiotic treatments available (Morrill et al. 2015) resulting in higher mortality rates (Munoz-Price et al. 2013; Tamma et al. 2017) and unsurprisingly, MDR or XDR K. pneumoniae are considered an urgent public health threat and a major challenge to the delivery of safe healthcare (World Health Organisation 2017).
The dearth of novel antibiotic treatments in the pipeline for Gram-negative bacteria means that the development of alternative treatments based on innovative use of existing drugs is an option. Clinically, the administration of antibiotic combinations to patients suffering from MDR or XDR K. pneumoniae infection is often employed as a solution to improve therapeutic outcomes. However, agreement on the best combinations to use, and evidence of any real benefit, is contested (Tamma, Cosgrove and Maragakis 2012). Examples of combination therapies employed with some success include, dual carbapenem therapy (Souli et al. 2017), ceftazidime with avibactam (Lagacé-Wiens, Walkty and Karlowsky 2014), meropenem with vaborbactam (Lee and Baker 2018), and the polymyxin colistin combined with other antibiotics (Gutierrez-Gutierrez et al. 2017). Unfortunately, none of these options are ideal particularly because of the nephrotoxicity and rise of resistance associated with colistin (Capone et al. 2013), and the inability of either avibactam or vaborbactam to inhibit metallo-beta-lactamases such as NDM-1. Thus, there is an urgent clinical need to identify new treatment approaches for MDR or XDR K. pneumoniae strains that have acquired carbapenemases.
To improve options available for clinicians, the ‘repurposing’ of already approved drugs, whose primary use is not as antibacterials, as antibiotics could speed up the introduction of new treatment options, particularly if these compounds are administered in combinations with existing antibiotics (Ejim et al. 2011; Cheng, Williamson and Zheng 2019). One example of a drug that could be ‘repurposed’ as an antibiotic is the anti-viral drug zidovudine (AZT). Zidovudine is a nucleoside analogue with known bactericidal activity against Gram-negative bacteria, including K. pneumoniae (Elwell et al. 1987; Lewin and Aymes 1989; Peyclit et al. 2018). In addition, the drug was efficacious in a mouse model of systemic Escherichia coli infection (Keith, White and Wilson 1989). Upon entering Gram-negative bacterial cells, AZT is phosphorylated by thymidine kinases, incorporated into DNA and arrests replication by acting as a DNA-chain terminator (Doleans-Jordheim et al. 2011). A number of recent studies that screened libraries of approved drugs identified synergistic combinations against Gram-negative bacteria that included AZT (Wambaugh et al. 2017; Ng et al. 2018; Hind et al. 2019). Synergistic combinations of AZT that inhibited MDR, or XDR, K. pneumoniae in vitro included combinations with the lipopeptide antibiotics colistin (Hu, Liu and Coates 2019) or polymyxin B (Lin et al. 2019) that also showed enhanced efficacy compared to monotherapy in murine infection models. Despite these studies with the lipopeptide antibiotics, most studies of the effect of AZT in combination with existing antibiotics have been performed in vitro.
The aim of this research was to explore further the potential of administering AZT in combinations with existing antibiotics to treat infections with MDR pathogens using the Galleria mellonella larvae infection model. This system permits screening of antibacterial activity in vivo against real infections, in the presence of a functioning immune system, without the high costs and ethical issues associated with mammalian infection models. Thus, combination treatments consisting of AZT with the carbapenem beta-lactam, meropenem, were screened for enhanced efficacy compared to monotherapies in G. mellonella larvae infected with carbapenemase-producing strains of K. pneumoniae.
MATERIALS AND METHODS
Bacteria and growth media
Klebsiella pneumoniae strains were obtained from the National Collection of Type Cultures (NCTC; https://www.phe-culturecollections.org.uk/collections/nctc.aspx): NCTC 9633T, an antibiotic susceptible Type strain, NCTC 13443, producing the NDM-1 metallo-beta-lactamase and NCTC 13438, producing the KPC-3 carbapenemase (Woodford 2008). NCTC 9633T was included as a control to illustrate the resistance of the two carbapenemase strains to meropenem. All strains were grown to stationary phase in Mueller–Hinton broth (MHB; Merck, Darmstadt, Germany) at 37°C with shaking (at 200 rpm) overnight to prepare inocula for antibiotic efficacy testing in vitro or in vivo.
Drugs and G. mellonella larvae
Meropenem and zidovudine were purchased from Sigma–Aldrich Ltd (Dorset, UK). Stock solutions of meropenem or AZT were prepared in sterile deionized water with 10% dimethylsulphoxide. Sub-stocks of each drug for injection into larvae were prepared in deionized water. Galleria mellonella larvae were obtained from UK Waxworms Ltd (Sheffield, UK).
Antibiotic susceptibility testing in vitro
Minimum inhibitory concentrations (MICs) of antibiotics against the K. pneumoniae strains were determined in 96-well microplates as previously described (Hill, Veli and Coote 2014). Briefly, doubling dilutions of meropenem or zidovudine were prepared in MHB and subsequently inoculated with 1.0 × 106 cfu/mL of either K. pneumoniae strain. Microplates were incubated at 37°C and the MIC was defined as the concentration(s) present in the first optically clear well after 24 h.
Testing combinations for synergy in vitro
The effect of combinations of meropenem with AZT against both K. pneumoniae strains was carried out using 96-well microplate assays prepared via doubling dilution of meropenem in MHB followed by subsequent addition of AZT to form a combination checkerboard. Each well was then inoculated with 1.0 × 106 cfu/mL of either K. pneumoniae strain and microplates were incubated at 37°C. After 24 h, each well was scored for visible growth and fractional inhibitory concentration index (FICI) values were calculated for each combination tested. Synergy was defined as FICI ≤ 0.5 (Eliopoulos and Moellering 1996). Each K. pneumoniae strain was tested in duplicate.
The effect of meropenem and AZT combinations against both K. pneumoniae strains was also measured using a time-kill assay. Briefly, tubes containing concentrations of AZT, meropenem or a combination of both drugs were prepared in MHB broth. A control tube contained only sterile water in MHB. Drug concentrations were prepared at MIC50 for each strain. Tubes were then inoculated with 1.0 × 107 cfu/mL of either K. pneumoniae strain. Viability was determined after 0, 2, 4 and 6 h incubation at 37°C by serial dilution in MHB and plating on Nutrient Agar (Merck, Darmstadt, Germany). Each experiment was performed in duplicate and results expressed as mean ± standard error of the mean (SEM).
G. mellonella infection model
Efficacy of meropenem or AZT alone or in combination versus G. mellonella larvae infected with the K. pneumoniae strains was carried out exactly as described previously (Hill, Veli and Coote 2014; Krezdorn, Adams and Coote 2014; Adamson, Krikstopaityte and Coote 2015). Galleria mellonella at their final instar larval stage were kept at room temperature in darkness. Larvae weighing within the range of 250–350 mg were selected for each experiment to ensure consistency in subsequent drug administration and were used within 1 week of receipt.
Groups of 15 larvae were infected with inocula (10 µL) of either K. pneumoniae strain containing increasing numbers of bacteria to determine an appropriate infectious dose for subsequent drug efficacy studies. Control experiments using a heat-killed inoculum of each strain was carried out in which the bacterial suspension was heated at 98°C for 10 min prior to infection of a group of larvae. For all studies of drug efficacy, an inoculum of 5.6 × 105 cfu, or 9.1 × 107 cfu, was used for K. pneumoniae NCTC 13443 (NDM-1) and NCTC 13438 (KPC-3), respectively. A single treatment with phosphate-buffered saline (PBS), meropenem, AZT or a combination of both drugs was administered 2 h post-infection. The experiments were repeated in duplicate using larvae from a different batch and the data from these replicate experiments were pooled to give n = 30. Survival data were plotted using the Kaplan–Meier method (Bland and Altman 1998) and comparisons made between groups using the log-rank test (Bland 2004). In all comparisons with the negative control it was the uninfected control (rather than the unmanipulated control) that was used. Holm's correction was applied to account for multiple comparisons in all tests and P ≤ 0.05 was considered significant (Holm 1979).
Bacterial burden within larvae from each treatment group was measured exactly as described previously (Krezdorn, Adams and Coote 2014; Adamson, Krikstopaityte and Coote 2015; Ballard and Coote 2016). Groups of 30 larvae were infected with either strain of K. pneumoniae using the same inoculum sizes as described above. Meropenem, AZT or a combination treatment of both drugs were administered at 2 h post-infection. Larvae were incubated in Petri dishes at 37°C. At 24 h intervals, five larvae were randomly selected from each treatment group and surface decontaminated and anaesthetized by washing in absolute ethanol. Each larva was then placed in an Eppendorf tube containing 1 mL of sterile PBS and homogenized using a sterile pestle. Bacterial burden from individual caterpillars was then determined by serial dilution of the homogenate in MHB and plating on MacConkey agar (Formedium Ltd, Hunstanton, England). The detection limit for this assay was 100 cfu/mL of larval homogenate.
RESULTS
Two carbapenemase-producing strains of K. pneumoniae are resistant to meropenem but sensitive to the antiviral drug AZT
In comparison to the antibiotic-susceptible Type strain, the two strains producing the carbapenemases displayed resistance to meropenem as expected (Table 1). The MICs of meropenem were the same for both carbapenemase-producing strains but K. pneumoniae NCTC 13438, carrying the KPC-3 carbapenemase, was more resistant to AZT (4 mg/L) than NCTC 13443 with NDM-1 (1–2 mg/L). These results confirmed previous studies with different strains of K. pneumoniae that showed sensitivity to AZT (Elwell et al. 1987; Lewin and Aymes 1989; Peyclit et al. 2018).
Minimum inhibitory concentration (MIC) of zidovudine (AZT) and meropenem (MEM) for the K. pneumoniae Type strain or strains possessing either the NDM-1 or KPC-3 carbapenemases.
Bacterial strains . | MIC (mg/L) . | . |
---|---|---|
AZT | MEM | |
K. pneumoniae NCTC 9633T | - | <0.0625 |
K. pneumoniae NCTC 13443 (NDM-1) | 1.0–2.0 | 128–256 |
K. pneumoniae NCTC 13438 (KPC-3) | 4.0 | 128–256 |
Bacterial strains . | MIC (mg/L) . | . |
---|---|---|
AZT | MEM | |
K. pneumoniae NCTC 9633T | - | <0.0625 |
K. pneumoniae NCTC 13443 (NDM-1) | 1.0–2.0 | 128–256 |
K. pneumoniae NCTC 13438 (KPC-3) | 4.0 | 128–256 |
Minimum inhibitory concentration (MIC) of zidovudine (AZT) and meropenem (MEM) for the K. pneumoniae Type strain or strains possessing either the NDM-1 or KPC-3 carbapenemases.
Bacterial strains . | MIC (mg/L) . | . |
---|---|---|
AZT | MEM | |
K. pneumoniae NCTC 9633T | - | <0.0625 |
K. pneumoniae NCTC 13443 (NDM-1) | 1.0–2.0 | 128–256 |
K. pneumoniae NCTC 13438 (KPC-3) | 4.0 | 128–256 |
Bacterial strains . | MIC (mg/L) . | . |
---|---|---|
AZT | MEM | |
K. pneumoniae NCTC 9633T | - | <0.0625 |
K. pneumoniae NCTC 13443 (NDM-1) | 1.0–2.0 | 128–256 |
K. pneumoniae NCTC 13438 (KPC-3) | 4.0 | 128–256 |
G. mellonella larvae display dose-dependent lethality in response to infection by either strain of MDR K. pneumoniae
The effect of infection with either K. pneumoniae strain on survival of G. mellonella is shown in Fig. 1. In both strains, the heat-killed inoculum had no significant effect on larval survival (P > 0.05). With live inocula, larval survival was affected in a dose-dependent manner during 96 h incubation (Fig. 1). Together, these data indicate that infection with live K. pneumoniae is required to cause larval death and support previous studies that have utilized G. mellonella to study infection by different K. pneumoniae strains (Insua et al. 2013; Wand et al. 2013; McLaughlin et al. 2014; Benthall et al. 2015).
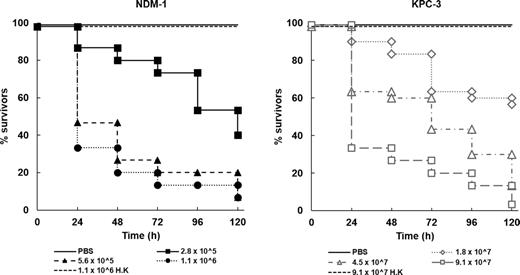
Effect of increasing inoculum dose of live K. pneumoniae NCTC 13443 (NDM-1) or NCTC 13438 (KPC-3) on the survival of G. mellonella larvae during incubation at 37°C for 120 h. Numbers in the legend indicate the inoculum size in bacterial cells per larva. For both strains, the effect of heat-killed (h.k.) bacterial inocula is also shown. No significant mortality was observed in an unmanipulated group (data not shown) or in the uninfected group mock ‘infected’ with sterile PBS. For all infected groups, survival was significantly reduced compared to the mock ‘infected’ group (P < 0.05, log rank test with Holm correction for multiple comparisons); n = 30 (pooled from replicate experiments).
Klebsiella pneumoniae NCTC 13443 (NDM-1) displayed greater virulence than NCTC 13438 (KPC-3), requiring a smaller inoculum of viable bacteria to induce a similar degree of lethality to infected larvae. Infectious doses for each strain were selected for use in subsequent studies on antibiotic efficacy (5.6 × 105 cfu for K. pneumoniae NCTC 13443 and 9.1 × 107 cfu for NCTC 13438) that resulted in the death of approximately 90% of larvae after 96 h incubation at 37°C.
Efficacy of AZT or meropenem monotherapy in G. mellonella larvae infected with either carbapenemase-producing strain of K. pneumoniae is poor
The effect of single doses of AZT or meropenem, 2 h post-infection (p.i) with either K. pneumoniae strain, on survival of G. mellonella larvae is shown in Fig. 2. Both drugs increased larval survival in a dose-dependent manner regardless of the K. pneumoniae strain. However, neither drug conferred high levels of therapeutic benefit, with the exception of AZT versus larvae infected with K. pneumoniae NCTC 13443 (NDM-1) treated with the highest doses of 6.25 or 12.5 mg/kg. In contrast, treatment of larvae infected with the KPC-3-producing strain with the same doses of AZT had no therapeutic benefit after 120 h. As would be expected with these strains, treatment with meropenem conferred only low levels of therapeutic benefit even at the highest doses tested, particularly against infections with K. pneumoniae NCTC 13438 (KPC-3).
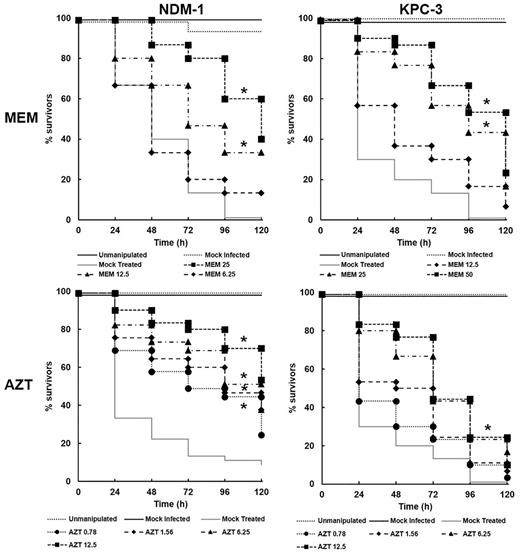
Effect of treatment with zidovudine (AZT) or meropenem (MEM) on survival of G. mellonella larvae infected with K. pneumoniae strains. Groups of larvae were mock ‘infected’ with sterile PBS, or 5.6 × 105 cells of the NDM-1 strain, or 9.1 × 107 cells of the KPC-3 strain. 2 h post-infection (p.i), a single dose of either MEM or AZT was administered (dose in mg/kg is the number shown on the figure). The mock ‘treated’ group represents infected larvae treated with sterile PBS. Larval survival was measured over a period of 120 h at 37°C. * indicates significantly enhanced survival compared to the mock ‘treated’ group (P < 0.05, log rank test with Holm correction for multiple comparisons); n = 30 (pooled from duplicate experiments).
In summary, neither AZT or meropenem monotherapy offered high levels of therapeutic benefit versus infections with either strain of carbapenemase-producing K. pneumoniae, although both drugs were moderately more effective against the NMD-1-producing strain than the KPC-3 strain.
Combination therapy with AZT plus meropenem shows enhanced efficacy compared to monotherapy versus G. mellonella infections with carbapenemase-producing MDR strains of K. pneumoniae
The effect of monotherapy, with AZT or meropenem, compared with combination therapy on survival of G. mellonella larvae, and burden of infecting bacteria, for both carbapenemase-producing strains of K. pneumoniae is shown in Fig. 3. Pilot experiments were carried out testing many different doses of AZT or meropenem in combination to identify the most effective doses to employ against larval infections with either strain of K. pneumoniae (data not shown). From these initial studies, optimal combination dosing regimens were selected that offered the best therapeutic benefit and subsequently studied in detail—NCTC 13443 (AZT − 0.78 mk/kg + meropenem − 6.25 mg/kg) and NCTC 13438 (AZT − 6.25 mg/kg + meropenem − 12.5 mg/kg) (Fig. 3).
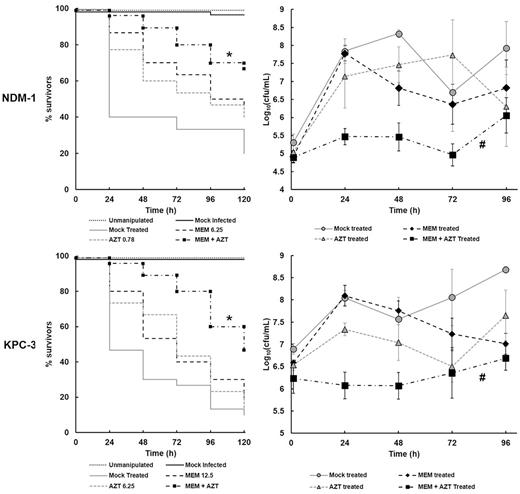
Effect of treatment with combinations of AZT and MEM on the survival and internal bacterial burden of G. mellonella larvae infected with K. pneumoniae strains. Larvae were infected with PBS (mock ‘infected’), K. pneumoniae NCTC 13443 (NDM-1) − 5.6 × 105 cells, or NCTC 13438 (KPC-3) − 9.1 × 107 cells and treated with either PBS (mock ‘treated’), or a single dose of each drug individually, or a combination of MEM and AZT at 2 h p.i. (dose in mg/kg is the number shown on the figure). Larvae were incubated at 37°C for 120 h and survival recorded every 24 h. The larval burden of K. pneumoniae was determined from five individual larvae per treatment group every 24 h for 96 h at 37°C. Error bars indicate ± SEM. * combination treatment group with significantly enhanced survival compared with any of the constituent monotherapies (P < 0.05, log-rank test with Holm's correction for multiple comparisons). n = 30 (pooled from duplicate experiments). # significant difference in larval burden between groups treated with the combination of AZT + MEM compared with each monotherapy (P < 0.05, the Mann–Whitney U-test compared the combination therapy with each monotherapy). n = 5.
As shown previously (Fig. 2), a single dose 2 h p.i of either AZT or meropenem had minimal therapeutic benefit on larvae infected with either strain of K. pneumoniae (Fig. 3). This was reflected in large increases over 24 h in the internal burden of either infecting K. pneumoniae strain within individual larvae. For larvae infected with NCTC 13443 (NDM-1), the increase in bacterial numbers after treatment with either meropenem or AZT was the same as larvae mock treated with PBS. With strain NCTC 13438 (KPC-3), larvae treated with either meropenem or PBS alone also displayed an identical increase in bacterial burden after 24 h. Notably, the increase in bacterial numbers after treatment with AZT was reduced (approximately 0.5 log10 cfu/mL), indicating some inhibitory effect of the drug on proliferation of strain NCTC 13438 within the larvae. However, this smaller increase in bacterial numbers post AZT therapy was not reflected in any significant reduction in larval death compared to treatment with meropenem or PBS (Fig. 3).
In direct contrast to monotherapies, a single dose of a combination of AZT + meropenem resulted in significantly (P ≤ 0.05) enhanced survival of larvae infected with either MDR strain of K. pneumoniae (Fig. 3). For example, 120 h p.i with NCTC 13443 (NDM-1), populations treated with PBS, AZT alone or meropenem alone showed survival of 20, 40 and 47% respectively. In contrast, 67% of larvae treated with the combination survived. At the same time p.i with NCTC 13438 (KPC-3), treatment with PBS, AZT alone or meropenem alone showed survival of 10, 13 and 17% respectively compared to 47% for the combination. Correlating with this enhanced survival, the burden of bacteria within the infected larvae did not show the large increase after 24 h observed previously with the monotherapies, and, bacterial numbers remained significantly lower throughout the duration of the experiment (P ≤ 0.05). Whilst the combination treatment halted the proliferation of infecting bacteria of either strain, numbers did not fall below the initial infecting inoculum size. Thus, the therapeutic benefit conferred by AZT + meropenem combination treatment appears to be due to a bacteriostatic effect in vivo.
In summary, a combination treatment of AZT + meropenem offers a potential novel therapy for treatment of MDR, carbapenemase-producing strains of K. pneumoniae.
The inhibitory action of the combination of AZT plus meropenem versus K. pneumoniae is not significantly synergistic in vitro
To help understand the nature of the inhibitory action of the combination of AZT with meropenem that conferred enhanced efficacy in vivo, checkerboard and time-kill experiments were conducted in vitro (Fig. 4). A checkerboard assay showing the effect of different AZT and meropenem combinations on growth of both carbapenemase-producing strains of K. pneumoniae is shown in Fig. 4A. For NCTC 13443 (NDM-1), there was some evidence of minor synergy (FICI ≤ 0.5) at only two combinations tested. The majority of the other combinations that inhibited growth did so in an indifferent or additive fashion. For strain NCTC 13438 (KPC-3), none of the inhibitory combinations tested were synergistic, with all displaying indifference or additivity.
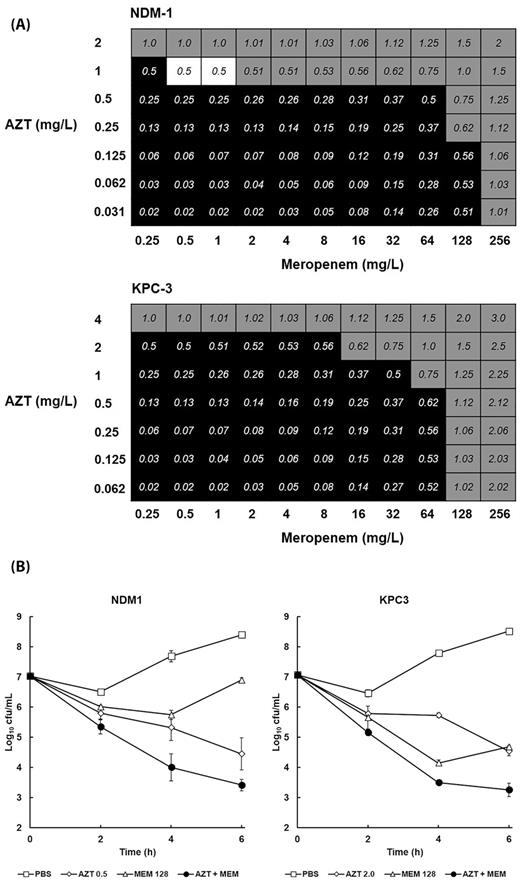
The effect of combination of AZT with MEM on the growth and viability of K. pneumoniae NCTC 13443 (NDM-1) or NCTC 13438 (KPC-3) in vitro. Fractional inhibitory concentration indices (FICI) of AZT combined with MEM versus NDM-1 and KPC-3 after 24 h in MHB at 37°C (A). Black squares indicate FICI values where bacterial growth occurred. Grey squares indicate wells where the FICI values were ≥0.5 (indicating inhibition was not synergistic). White squares show FICI values of 0.5 or less where bacterial growth was inhibited and thus indicate synergistic inhibition of growth. The experiment was performed in duplicate and a representative result is shown. Time-kill curves of the effect of 6 h exposure to PBS or MIC50 of; MEM alone (128 mg/L for both NDM-1 and KPC-3); AZT alone (0.5 mg/L NDM-1 and 2 mg/L KPC-3) or AZT + MEM (NDM-1 − 128 mg/L MEM + 0.5 mg/L AZT; KPC-3 − 128mg/L MEM + 2 mg/L AZT). Error bars indicate ± SEM from duplicate experiments (B).
The effect of exposure to the single drugs (at MIC50) and a combination (also at MIC50 for each drug) on viability of both strains is shown in Fig. 4B. Bacterial viability was measured over a period of 6 h at 37°C. Control populations of both strains, mock treated with PBS increased in cell number over the duration of the experiment. Exposure to AZT alone resulted in a loss of viability of both strains of approximately 3 log10 cfu/mL after 6 h. In contrast, exposure to meropenem also resulted in loss of viability, but after 6 h exposure, there was evidence from both strains that the surviving population of bacteria was recovering and growth resuming. With both strains, the combination treatment resulted in a steady decline in viability of approximately 4 log10 cfu/mL after 6 h. Despite the greater bactericidal effect of the combination compared to the individual drugs, the loss of viability induced by the combination was only approximately 1 log10 cfu/mL more than AZT alone. This supports the checkerboard results by showing that the inhibition of K. pneumoniae induced by exposure to the combination of AZT with meropenem is not strongly synergistic. Notably, despite being bactericidal, the combination did not eliminate all bacteria over the duration of the experiment. This observation is supported by the G. mellonella larval burden assays (Fig. 3) where infecting K. pneumoniae were never eliminated and a reduced number of bacteria were always detectable.
DISCUSSION
The antibacterial properties of AZT have been well documented but the drug has never been formally approved to treat bacterial infections (Elwell et al. 1987; Lewin and Aymes 1989; Ng et al. 2018). With the emergence of untreatable infections by MDR, or XDR, Gram-negative bacteria, there has been renewed interest in exploiting these properties and ‘repurposing’ the drug. In fact, a recent study proposed using AZT alone as a salvage therapy for colistin-resistant infections (Peyclit et al. 2018). In this study, AZT was shown to inhibit two MDR, carbapenemase-producing strains of K. pneumoniae with MICs between 1.0 and 4.0 mg/L, confirming the known inhibitory effect of AZT on this pathogen (Elwell et al. 1987; Lewin and Aymes 1989; Peyclit et al. 2018). In addition, G. mellonella larvae infected with the same strains displayed enhanced survival after monotherapy with AZT.
Despite many studies showing that AZT is antibacterial, one reason why the drug may not be highly effective as a monotherapy is the induction of resistance after short-term exposure (Lewin et al. 1990). AZT only inhibits bacteria that possess a thymidine kinase that phosphorylates the AZT such that it can then be incorporated into DNA and arrest DNA replication (Doleans-Jordheim et al. 2011). In E. coli, mutations, or the presence of insertion sequences, in the gene encoding thymidine kinase (that could result in impaired function of the enzyme) correlated with AZT resistance (Doleans-Jordheim et al. 2011). Furthermore, E. coli strains resistant to AZT have been isolated from patients undergoing therapy with the drug and the activity of thymidine kinase in these strains was reduced (Lewin et al. 1990). Thus, because of the issue of resistance to AZT, the most likely application of the drug as an antibacterial therapy for MDR Enterobacteriaceae is in combination therapies with antibiotics that could help reduce the onset of resistance.
A number of studies have highlighted effective combinations of AZT with various approved antibiotics in vitro including: tigecycline against MDR E. coli and K. pneumoniae (Ng et al. 2018); colistin versus colistin-resistant K. pneumoniae (Falagas et al. 2019) or E. coli (Loose et al. 2018; Peyclit et al. 2018); trimethoprim and/or sulfamethizole against trimethoprim-resistant E. coli and K. pneumoniae clinical isolates (Wambaugh et al. 2017); and the aminoglycosides, gentamicin and amikacin against E. coli (Doleans-Jordheim et al. 2011). Notably, two studies demonstrate enhanced efficacy in vivo of AZT in combination with colistin (Hu, Liu and Coates 2019) or polymyxin B (Lin et al. 2019), compared to their constituent monotherapies, in murine infection models with NDM-producing K. pneumoniae and/or colistin-resistant E. coli. In this study, a combination therapy consisting of AZT with meropenem resulted in enhanced efficacy against infections by two MDR, carbapenemase-producing strains of K. pneumoniae in G. mellonella larvae compared to each monotherapy. Supporting these findings, a recent screen of Food and Drug Administration-approved drugs identified that AZT acted as an antibiotic-resistance breaker (ARB) when combined with meropenem and potentiated the inhibitory effect of the antibiotic against MDR K. pneumoniae in vitro (Hind et al. 2019). Clearly, only two NCTC carbapenemase-producing strains were used in this study and additional studies using a range of carbapenemase-producing clinical isolates will be required to confirm the enhanced efficacy of this combination.
Despite the enhanced efficacy of the combination, the larval populations treated with the combinations still suffered mortality for the duration of the experiments albeit less than those treated with the monotherapies. This observation could be explained by the effect the different treatments had on the burden of infecting bacteria within the larvae. For example, monotherapies had no detrimental effect on the infecting bacteria of either strain because numbers increased rapidly over the first 24 h in the same fashion as larvae sham-treated with PBS. However, combination therapy had the effect of preventing infecting bacteria of either strain from proliferating in the larvae but, notably, did not reduce the initial bacterial burden. The fact that combination-treated larvae still contained viable bacteria could account for these populations suffering mortality at a reduced rate compared to populations treated with either monotherapy. These observations in vivo were supported by the results from the in vitro time-kill experiments. For example, despite the combination of AZT with meropenem showing a bactericidal effect, lethality slowed over a 6 h period of exposure and low numbers of either strain survived. Furthermore, the enhanced efficacy of the combination treatment in vivo was unlikely to be due to a synergistic interaction between AZT and meropenem because the in vitro experiments largely showed an indifferent or additive effect. The lack of potent synergy between AZT and meropenem versus the two K. pneumoniae strains could account for the observed survival kinetics of infected larvae and the failure of the combination treatment to confer full survival or eliminate all infecting bacteria at the doses tested. It is likely that the enhanced efficacy of the combination observed in vivo can be explained by the FICI values observed in vitro. For example, the best FICI value obtained for the NDM-1 strain was 0.5 and for the KPC-3 strain 0.62. These values represent a weak synergistic effect or, at the very least, an indifferent or additive effect of the combination versus both strains. An additive effect is supported by the time-kill assay whereby a 6 h exposure to the combination resulted in only approximately 1 log10 cfu/mL greater reduction in cell numbers than either of the constituent drugs alone.
For therapy of Human Immunodeficiency Virus (HIV), AZT is administered orally at 300 mg twice a day indicating that blood plasma concentrations above the MIC for K. pneumoniae could be reached (Peyclit et al. 2018; Falagas et al. 2019). Furthermore, AZT is well-tolerated, and toxicity generally only manifests after long-term use of the drug—a scenario that would be unlikely if antibiotic/AZT combinations were used to treat acute bacterial infections.
In summary, this work has identified that combination of AZT with meropenem represents a plausible alternative therapy to treat infections with MDR, carbapenemase-producing strains of K. pneumoniae and merits further investigation.
FUNDING
This research was funded by the University of St Andrews.
Conflicts of interest
None declared.