-
PDF
- Split View
-
Views
-
Cite
Cite
Ehsan Ollah Jazaeri, Atiyeh Mahdavi, Asghar Abdoli, Formulation of chitosan with the polyepitope HIV-1 protein candidate vaccine efficiently boosts cellular immune responses in mice, Pathogens and Disease, Volume 75, Issue 8, November 2017, ftx098, https://doi.org/10.1093/femspd/ftx098
- Share Icon Share
Abstract
Human immunodeficiency virus-1 (HIV-1) continues to be a major global public health issue and priority. Despite the variety of antiretroviral therapies, it seems that an effective vaccine against HIV-1 is still very necessary. An ideal HIV-1 vaccine should be able to elicit both humoral and cellular immunities. In this respect, polyepitope vaccines, incorporated from several conserved regions of HIV-1 proteins, have received much attention recently. Herein, the immunogenicity of the HIV-1 polyepitope protein-based candidate vaccines was evaluated in BALB/c mice. Following the plasmid (pET23a-HIV-1-tat/pol/gag/env) preparation and transformation, the recombinant protein expression was optimized in Escherichia coli BL21 (DE3) host cells. After the HIV-1-top4 protein purification, chitosan and alum adjuvants were added to the vaccines formulations to reinforce the immunogenicity of the candidate vaccines. Mice were subcutaneously immunized three times at 2-week intervals with the candidate vaccines and the elicitation of both humoral and cellular immune responses were investigated. Taken together, the results showed that chitosan adjuvanted candidate vaccine conferred a stronger immunogenicity and elicited higher cellular responses than other candidate vaccines (P < 0.05). Thereby, it seems that co-utilizing of potent adjuvants with the HIV-1 polyepitope protein vaccines can help to open new avenues for strategies for HIV/AIDS vaccine design.
INTRODUCTION
Viral infectious diseases are increasing in frequency and lead to high mortality around the world every year (Oyarzun et al.2015). Among them, human immunodeficiency virus-1 (HIV-1) continues to be a major global public health challenge and priority, accounting for nearly 1.2 million acquired immunodeficiency syndrome (AIDS)-related deaths occurring annually worldwide (UNAIDS 2015). By introducing effective antiretroviral drugs since the late 1990s, AIDS-related mortality and morbidity have decreased and the scenario of HIV/AIDS has changed (Cafaro et al.2015). However, developing a safe and effective HIV-1 vaccine seems to be the best solution for achieving the ultimate control of the AIDS pandemic (Wang et al.2016).
Although the great efforts have been made to fight against HIV/AIDS since the discovery of HIV-1 in 1983, there are some important limitations that fail to develop a successful vaccine and must be addressed by new strategies. Some of them include the viral extraordinary mutability and genetic diversity, early establishment of latent viral reservoirs, immune evasion (by quick changing its antigenic structure to new antigenic variants), unsafe use of attenuated viruses for human and lack of a small suitable animal model (Barouch 2008; Koff 2012; Reynell and Trkola 2012; Koff et al.2013). One of the widely used approaches to reduce these problems is employing the recombinant polyepitope DNA technology. In this method, conserved and immunogenic epitopes are arranged together in a small gene construct and either used as a DNA vaccine or their expression product applied as a protein vaccine. One of the paramount advantages of this technology is that the immune response focuses on the critical conserved epitopes and so, provides the possibility to make a simple and universal vaccine that could cover all HIV-1 clades and be intended to induce humoral or cellular immunity (or both of them, at the same time) (Karpenko et al.2014; Orellana-Escobedo et al.2015; Soria-Guerra et al.2015). Also, biosafety (no requirement for in vitro culturing), bioprocessing (rapid large-scale production), selectivity (selected conserved or immunodominant epitopes activate precisely and/or trigger predominantly cellular or humoral responses) and multivalency (multiple determinants from several pathogens) of these vaccines are remarkable (Soria-Guerra et al.2015).
Some criteria that should be considered in polyepitopic vaccine design include epitope conservancy in different variants, epitope immunogenicity and binding affinity of the incorporated epitopes for special major histocompatibility complex molecules (Karpenko et al.2014; Rosa et al.2015). There are reasonable evidences that suggest co-utilizing of the common retroviral structural protein genes (such as gag, pol and env) and regulatory protein genes (such as tat, rev, nef, vpr, vif and vpu) within the structure of polyepitope vaccines can increase the efficacy of vaccines (Mahdavi et al.2010; Mothe et al.2011; Arabi et al.2014; Jafarpour et al.2014). Among the viral structural and regulatory proteins, Gag, Tat, Pol and Env proteins have received considerable attentions due to their critical roles in the viral life cycle and pathogenesis (Mahdavi et al.2009; Mothe et al.2011). The HIV-1 gag gene is expressed in precursor form that is finally broken down to the structural proteins such as p24. This protein is one of the important antigens that is detectable in the sera of HIV-1-infected individuals during the early stages of infection (Mahdavi et al.2009; Mothe et al.2011; Jafarpour et al.2014; Reguzova et al.2015a,b). The gp160 protein, encoded by the HIV-1 env gene, is broken down into the two surface glycoproteins gp120 and gp41. Since the important T-cell and neutralizing antibodies (nAbs) epitopes are located on the envelope proteins and these viral proteins play determinant roles in the viral attachment to the host cells (CD4+ T lymphocytes), and therefore, implicate to the vaccine development (Mahdavi et al.2009; Mothe et al.2011; Jafarpour et al.2014; Reguzova et al.2015a,b). The HIV-1 pol gene codes for the viral vital enzymes including reverse transcriptase, integrase and protease. These enzymes are responsible for turning the single-stranded RNA into a double-stranded DNA, integrating the viral double-stranded DNA into the host genome, and cutting the precursor polypeptides into the functional proteins, respectively (Mahdavi et al.2009, 2010; Jafarpour et al.2014). Finally, the HIV-1 regulatory tat gene synthesizes the Tat protein which activates transcription of all HIV-1 genes. According to the literatures, targeting of the Tat protein can inhibit the virus replication in several stages of the viral life cycle (Alipour, Mahdavi and Abdoli 2017). Taken together, it is emphasized again that incorporating important epitopes from the viral pivotal structural and regulatory proteins in a given recombinant polyepitope protein vaccine can lead to stronger elicitation of immune responses (Mothe et al.2011; Jafarpour et al.2014; Reguzova et al.2015a,b).
On the other hand, DNA and protein vaccines are often poorly immunogenic alone, due to their high purities, implying the need for an efficient adjuvant and a suitable formulation to boost their immunogenicity (Moingeon, De Taisne and Almond 2002). Moreover, adjuvants not only improve the potency and longevity of immune responses to antigens but also avoid long-lasting immune effects on their own and cause only minimal toxicity. The numerous advantages of adjuvants have increasingly expanded their applications in vaccines formulations and researches (Mohan, Verma and Rao 2013). Chitosan has become a very attractive substance for medical fields due to its biocompatibility, biodegradability and bioactivity. This compound was used in solution form for subcutaneous vaccination using a model antigen and elicited both humoral and cell mediated immune responses (Arca, Günbeyaz and Şenel 2009; Li et al.2013). Although the precise mechanism which it acts is not clear, several studies have shown that Chitosan enhances the accumulation of macrophages, natural killer cells and polymorphonuclear cells. It also results in the increased resistance to infection by inducing cytokines such as IL-2, 10, 12, INF-γ and TNF-α. Positively charged chitosan, as a delivery system, can electrostatically interact with the cell surface negative charges, and consequently, increases antigens’ uptake by antigen-presenting cells (Gupta and Siber 1995; Arca, Günbeyaz and Şenel 2009; Amidi et al.2010; Jabbal-Gill, Watts and Smith 2012; Li et al.2013; Carroll et al.2016). Human-compatible alum adjuvant acts as a depot delivery system, controls antigens’ release and protects them against degradation. It, therefore, increases the duration of the antigen interactions with the immune system, and so, enhances the elicited immune responses against the given antigen. In addition, this adjuvant induces an inflammatory response in tissues. Recent studies have highlighted the importance of interleukin 4 in the antibody response of the animals immunized with alum-adsorbed antigens (O’Hagan 2001; HogenEsch 2002; Gupta and Siber 1995).
In this study, the efficacy of the HIV-1 polyepitopic candidate vaccine (incorporated from the important epitopes of the viral pivotal structural and regulatory proteins Tat, Pol, Gag and Env) was assessed alone and in combination with chitosan and alum adjuvants in mice model. To this end, following the plasmid transformation, optimization of the protein expression and purification, formulation and preparation of the recombinant candidate protein vaccines were carried out. With regard to the importance of the route of vaccine administration in determination of its efficiency and to investigate the efficacy of subcutaneous injection route, BALB/c mice were subcutaneously immunized with the HIV-1 polyepitope candidate vaccines and the frequency and magnitude of both humoral and cellular immune responses from the immunized mice were evaluated and comparatively analyzed.
MATERIALS AND METHODS
Chemicals
The pET23a-HIV-1-tat-pol-gag-env vector was from Pasteur Institute of Iran (Tehran, Iran). The restriction enzymes EcoRI and XhoI were obtained from Fermentas (Vilnius, Lithuania). Chitosan and alum adjuvants, goat anti mouse IgG1 and IgG2a secondary antibodies were purchased from Sigma (St. Louis, MO, USA). Ampicillin and isopropyl-D-thiogalactopyranoside (IPTG) were obtained from Invitrogen (Carlsbad, CA, USA). Ni-NTA agarose was provided by Qiagen (Hilden, Germany). RPMI 1640 was obtained from Gibco (Germany). Cytokine assay kit was purchased from Due Set (R&D Systems, USA). Lactate dehydrogenase (LDH) assay kit and 5-bromo-2-deoxy-uridine (BrdU) kit were purchased from Takara (Tokyo, Japan). All other chemicals were obtained from Merck (Darmstadt, Germany). The data presented in this paper are typical experimental data, and their reproducibility was confirmed by repeating the experiments at least three times.
Protein expression and purification
The presence of the HIV-1-tat-pol-gag-env fragment in pET-23a plasmid was confirmed using double digestion of the recombinant vector by the restriction enzymes EcoRI and XhoI (Fermentas, Germany) followed by gel electrophoresis and DNA sequencing. The confirmed recombinant plasmid was then introduced into the competent cells Escherichia coli DH5α, in order to increase the copy number of the plasmid, and finally transformed to E. coli BL21 (DE3) host cells by a chemical method (Sambrook and Russell 2006). To detect the best colonies producing the recombinant protein, some of them were separately selected, cultured and induced at mid-log phase (OD600 = 0.5–0.8). For optimization of the recombinant protein expression, the impact of various factors including different temperatures (19–37°C, 1.0°C and 2.0°C unit intervals from 19–24°C and 27–37°C, respectively), various concentrations of IPTG (0.1, 0.5 and 1 mM) and different culture media (LB (Luria-Bertani), TB (Terrific broth) and 2XYT) on the protein production was examined by incubating the selected colonies at mentioned conditions for 1, 2, 3, 4 and 6 h after induction. At different steps of the optimization, 1 ml of each culture was harvested and the cells were lysed by heat denaturation and resolved in 12.5% sodium dodecyl sulfate polyacrylamide gel electrophoresis (SDS-PAGE) in order to evaluate the recombinant protein expression (Laemmli 1970). Following expression optimization, large-scale production and purification of the recombinant HIV-1-top4 protein was carried out. Briefly, the transformed E. coli BL21 (DE3) host cells were cultivated in 5 ml of 2XYT medium with ampicillin (50–100 μg ml−1) at 37°C with vigorous shaking (250 rpm) for 12.0–14.0 h. The precultured cells were then used to inoculate production medium at a concentration of 1% (v/v) and added to 250 ml of 2XYT medium in 1 L Erlenmeyer flasks and induced with 0.1 mM IPTG when the culture reached an OD600 of 0.5–0.8. After addition of IPTG, the cultivation was continued for 6 h at 20°C with shaking (180 rpm min−1). Finally, the medium was cooled down and the cells were harvested by centrifugation at 5000 g for 20 min. The supernatant was discarded and the pellet was stored at –20°C overnight. For the protein purification, bacterial pellets were resuspended in the lysis buffer containing 50 mM Tris-base (pH 8.0), 300 mM NaCl, 10 mM imidazole, 1 mM PMSF and 10% (v/v) glycerol. The cells were lysed by sonication on ice and the cellular fractions were cleared by centrifugation at 12 000 rpm for 20 min at 4°C (Sambrook and Russell 2006). Since the recombinant HIV-1-top4 protein included a His6-tag at its C-terminus, purification of the recombinant protein was carried out using a Ni-NTA agarose column and the resultant supernatants were applied onto the column equilibrated with 50 mM NaH2PO4 (pH 8.0), 300 mM NaCl and 10 mM imidazole. After gentle shaking on ice for 1 h, the column was washed as step gradients with the required volume of the same buffer containing 2% Triton X-100 and increasing concentrations of imidazole (20, 30 and 50 mM imidazole, respectively, in different washing steps). Finally, the pure protein was eluted by elution buffer containing 50 mM NaH2PO4 (pH 8.0), 600 mM NaCl and 250 mM imidazole. The purity of the protein was confirmed by 12.5% SDS-PAGE analysis and total protein concentration was estimated by the Bradford method using Coomassie blue with bovine serum albumin (BSA) as the standard protein (Bradford 1976). After SDS-PAGE verification, the collected pure fractions were dialyzed against 50 mM phosphate-buffered saline (PBS) (pH 7.2–7.4) by gentle stirring for overnight at 4°C and finally stored at –40°C till used. The endotoxin content of the different pure fractions was also measured by Limulus amoebocyte lysate (Lonza, Switzerland) according to the manufacturer's instructions.
Mice immunization protocol
The immunogenicity of the HIV-1-top4 recombinant protein alone and formulated with chitosan and alum adjuvants was evaluated in BALB/c mice. Inbred female BALB/c mice (6–8 weeks old) were obtained from Pasteur Institute of Iran (Karaj, Iran). National Institutes of Health (NIH) guidelines for the care and use of laboratory animals were carried out. The mice were divided into six groups (six mice/group) and were subcutaneously immunized three times (on days 0, 14 and 28) at 2-week intervals with the candidate vaccines according to the schedule (Table 1). The control mice were subcutaneously immunized with each of adjuvants alone and sterile PBS.
Group . | Immunogen . | Adjuvant . | Injection . |
---|---|---|---|
HIV-1-top4 protein alone | HIV-1-top4 protein (10 μg/100 μl) | —- | Subcutaneous |
HIV-1-top4 + alum | HIV-1-top4 protein (10 μg/100 μl) | Alum (15 μg/100 μl) | Subcutaneous |
HIV-1-top4 + chitosan | HIV-1-top4 protein (10 μg/100 μl) | Chitosan (10 μg/100 μl) | Subcutaneous |
Control | PBS (100 μl) | —- | Subcutaneous |
Group . | Immunogen . | Adjuvant . | Injection . |
---|---|---|---|
HIV-1-top4 protein alone | HIV-1-top4 protein (10 μg/100 μl) | —- | Subcutaneous |
HIV-1-top4 + alum | HIV-1-top4 protein (10 μg/100 μl) | Alum (15 μg/100 μl) | Subcutaneous |
HIV-1-top4 + chitosan | HIV-1-top4 protein (10 μg/100 μl) | Chitosan (10 μg/100 μl) | Subcutaneous |
Control | PBS (100 μl) | —- | Subcutaneous |
Group . | Immunogen . | Adjuvant . | Injection . |
---|---|---|---|
HIV-1-top4 protein alone | HIV-1-top4 protein (10 μg/100 μl) | —- | Subcutaneous |
HIV-1-top4 + alum | HIV-1-top4 protein (10 μg/100 μl) | Alum (15 μg/100 μl) | Subcutaneous |
HIV-1-top4 + chitosan | HIV-1-top4 protein (10 μg/100 μl) | Chitosan (10 μg/100 μl) | Subcutaneous |
Control | PBS (100 μl) | —- | Subcutaneous |
Group . | Immunogen . | Adjuvant . | Injection . |
---|---|---|---|
HIV-1-top4 protein alone | HIV-1-top4 protein (10 μg/100 μl) | —- | Subcutaneous |
HIV-1-top4 + alum | HIV-1-top4 protein (10 μg/100 μl) | Alum (15 μg/100 μl) | Subcutaneous |
HIV-1-top4 + chitosan | HIV-1-top4 protein (10 μg/100 μl) | Chitosan (10 μg/100 μl) | Subcutaneous |
Control | PBS (100 μl) | —- | Subcutaneous |
Lymphocyte proliferation assay
Two weeks after the final immunization, spleens from each group of the immunized mice were removed under sterile conditions and suspended in sterile cold PBS containing 2% fetal bovine serum (FBS). Red blood cells were lysed with lysis buffer (containing 90 ml of 0.16 M NH4Cl and 10 ml of 0.17 M Tris, pH 7.2), and single-cell suspension was then adjusted to 3 × 106 cells/ml in RPMI 1640 (Gibco) supplemented with 10% FBS, 4 mM L-glutamine, 100 μg/ml streptomycin and 100 IU/ml penicillin. Cell suspension (100 μl) was dispensed into 96-well flat-bottom culture plates and stimulated with 10 μg/ml of the HIV-1-top4 protein as antigen recall. Cancanavalin-A (5 μg/ml) (Gibco) was used as a positive control. Unstimulated wells were used as the negative controls and complete culture medium was used as a blank. After 72 h of incubation at 37°C with 5% CO2, 100 μl of BrdU labeling solution was added into each well and incubation continued for 18 h. The culture medium was then removed by centrifugation (300 g at 4°C) and the wells were dried and fixed with 100 μl of fixation/permeabilization buffer. Subsequently, 100 μl of anti-BrdU antibody was added to each well and the plates were washed five times with wash buffer (PBS, pH 7.2) and then tetramethylbenzidine (TMB) substrate solution was added. The reaction was finally stopped by adding 100 μl of 2 N H2SO4. The optical density (OD) for each well was measured at 450 nm using a Universal Microplate Reader (Biohit 8000, USA). The cell proliferation was reported as the stimulation index (SI) which was calculated according to the formula: OD of stimulated wells/OD of unstimulated wells.
Measurement of IFN-γ production
A total number of 1.5 × 106 cells/ml from single-cell suspension of each mouse spleen were distributed in the wells of a 24-well plate, stimulated in vitro with 10 μg/ml of the HIV-1-top4 recombinant protein and incubated at 37°C in 5% CO2 for 72 h. The plates were then centrifuged at 300 g and 4°C for 10 min, and the supernatants were collected for cytokine analysis. IFN-γ production was measured using a commercial kit from R&D Systems (Duo Set, USA) according to the manufacturer's instructions. The quantity of IFN-γ cytokine was reported as pg/ml according to the plotted standard curve.
Cytotoxic T-lymphocyte activity assay
Splenocyte suspensions from the immunized mice were prepared as the effector cells. Briefly, 14 days after the final immunization, single-cell suspensions were prepared and adjusted to 2 × 106 cells/100 μl in RPMI 1640 (Gibco) supplemented with 10% FBS, 4 mM L-glutamine, 100 μg/ml streptomycin and 100 IU/ml penicillin and dispensed into 96-well flat-bottom culture plates. Mouse SP2/0 cell line (the tumor cells derived from the syngeneic BALB/c mouse myeloma) was selected as the target cells. The target cells were pulsed overnight with 10 μg/ml of the HIV-1-top4 antigen. The target cells in amount 20 000 cells/100 μl were incubated with 2 × 106 cells/100 μl of the effector cells at ratio 100/1 effector/target (E/T) for 5 h in RPMI 1640 containing 2% BSA at 37°C and 5% CO2. After centrifugation at 300 g and 4°C for 10 min, 100 μl supernatants of each well were transferred to the equivalent 96-well flat-bottom plates, and the cytotoxicity was determined by assaying LDH release at 450 nm by the infected cells. The lysis percentage (cytotoxicity) was calculated by the formula:
Specific cytolysis (%) = (OD of experimental LDH release—OD of spontaneous LDH release of effector cells)—OD of spontaneous LDH release from target cells)/(maximum LDH release of target cells—OD of spontaneous LDH release of target cells) × 100.
Meanwhile, the maximum lysis was determined from the supernatants of the cells that were lysed by 1% Triton X-100 and the spontaneous release was determined from the target cells incubated with RPMI 1640 containing 2% BSA.
Measurement of IgG subclasses
Bleeding was carried out 1 week after the last injection. The sera were separated and specific antibodies (IgG1 and IgG2a) were determined by an optimized indirect ELISA method. Briefly, 100 μl of 10 μg/ml of the recombinant HIV-1-top4 protein in PBS was coated into 96-well ELISA Maxisorp plates (Nunc, Naperville, IL) for overnight at 4°C. The wells were washed with PBS containing 0.05% Tween 20 (TPBS) and blocked with 1% BSA in PBS, for 1 h at 37°C. After washing, 100 μl of the diluted sera (1/400 in 1% BSA/TBS/Tween 20) were added to each well and the plate was incubated at 37°C for 2 h. The wells were then washed five times with TPBS (0.1%) and incubated for 2 h with 100 μl diluted horseradish peroxidase-conjugated anti mouse IgG1 and IgG2a (1/7000) (Sigma). The plates were finally washed five times with wash buffer and incubated for 30 min with 100 μl of TMB substrate in dark place. The reaction was stopped with 2 N H2SO4 (50 μl/well) and color intensity was measured at 450 nm with an ELISA plate reader (Biohit 8000, USA).
Th1:Th2 index calculation
Following measurement of IgG1 and IgG2a antibodies, the Th1:Th2 indexes were calculated according to the formula of IgG2a/IgG1. A value of the index above 1 means a shift of the immune response toward the Th1 type (cellular immunity), whereas the value of the index below 1 shows a shift of the immune response toward the Th2 type (humoral immunity) (Liu et al.2013).
Statistical analysis
All experiments were carried out in triplicates, and the data were compared as means ± SD of each experiment. All statistical analyses were performed using the Graphpad Prism 6.01 software (GraphPad Software Inc, San Diego, CA, USA) by one-way ANOVA followed by Tukey's test. In all cases, the mean differences showing P ≤ 0.05 were considered as significant.
RESULTS
Expression optimization and the protein purification
After verifying the presence of the HIV-1-top4 DNA fragment in pET-23a plasmid and transformation into the Escherichia coli BL21 (DH5α) competent cells and then E. coli BL21 (DE3) expression cells, expression optimization was carried out in E. coli BL21 (DE3) host cells by incubation of the selected colonies in different expression conditions, as mentioned in Materials and methods section. Optimum expression was obtained at 20°C, 2XYT medium, 0.1 mM IPTG and 6 h after induction. The His6-tagged HIV-1-top4 protein was efficiently purified by affinity chromatography using a Ni-NTA agarose column. To eliminate the lipopolysaccharide contaminations from the recombinant HIV-1-top4 protein expressed in E. coli cells, 2% Triton X-100 was used to extensively wash the column as the recombinant protein was immobilized on the column. It has been proven that endotoxins are efficiently separated from the aqueous phase in this concentration of the detergent (Siddappa et al.2006). However, for greater certainty, we also determined the endotoxin content of the pure fractions. The results showed that the final concentration of endotoxin in the eluted solutions was 0.04 EU/ml. The pure eluted fractions were collected for SDS-PAGE analysis. On SDS-PAGE under heat denatured conditions, the purified recombinant protein showed a purity of 95% and migrated to around 24 kDa. Finally, the pure HIV-1-top4 protein was dialyzed against PBS (pH 7.2–7.4) and its concentration was determined by the Bradford method (Fig. 1).
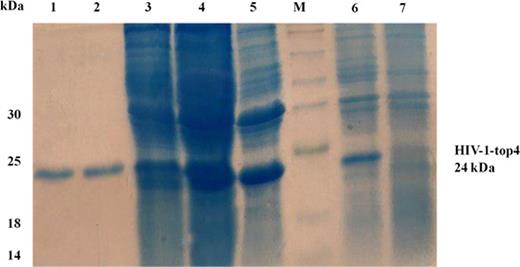
SDS-PAGE analysis of the purified HIV-1-top4 protein and its expression in 2XYT medium at 20°C. Lane M, molecular size marker; lanes 1 and 2, the purified recombinant protein after purification via affinity Ni-NTA column chromatography; lane 3, supernatant of cell lysates of E. coli cells after sonication and centrifugation; lane 4, pellet of cell lysates of E. coli cells after sonication and centrifugation; lane 5, whole cell lysates of E. coli cells after sonication; lane 6, whole cell lysates of E. coli cells 6 h after addition of 0.1 mM IPTG and lane 7, whole cell lysates of E. coli cells before IPTG addition.
Lymphocyte proliferation assay
For measuring lymphocyte proliferative responses, BALB/c mice were subcutaneously immunized with the HIV-1-top4 protein alone, the HIV-1-top4 adjuvanted with alum and the HIV-1-top4 adjuvanted with chitosan on days 0, 14 and 28, respectively. The cellular immune responses induced by vaccination were finally evaluated by BrdU method and the SI values were calculated. According to the results, chitosan adjuvanted HIV-1-top4 vaccine showed the significant SI value compared to the other groups (Fig. 2) (P < 0.05).
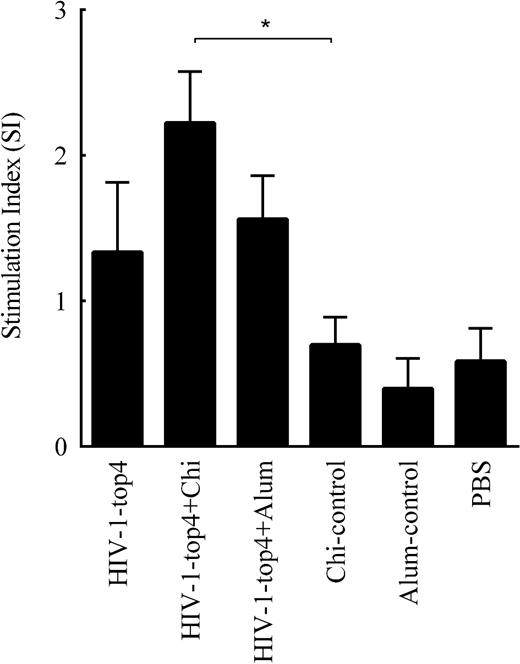
Lymphocyte proliferative responses of the immunized mice. Mice were subcutaneously immunized three times at 2-week intervals with the candidate vaccines. Spleen lymphocytes from individual mice were stimulated with the HIV-1-top4 recombinant protein and then prepared from the immunized mice, 14 days after the third and final immunization. Their subsequent proliferation responses were analyzed, using the BrdU test, and presented as the SI values. The results were expressed as the mean of SI values ± SD of triplicate experiments (P values < 0.05 were considered to be statistically significant).
Evaluation of IFN-γ production
To measure the cellular immune responses elicited by the candidate vaccines, the production of the IFN-γ cytokine was evaluated by the ELISA method. As depicted in Fig. 3, the production of IFN-γ cytokine has dramatically increased in response to the HIV-1-top4 formulated with chitosan adjuvant (P < 0.05). There was no significant difference between IFN-γ cytokine production in the mice vaccinated with the HIV-1-top4 protein alone and the HIV-1-top4 formulated with alum adjuvant.
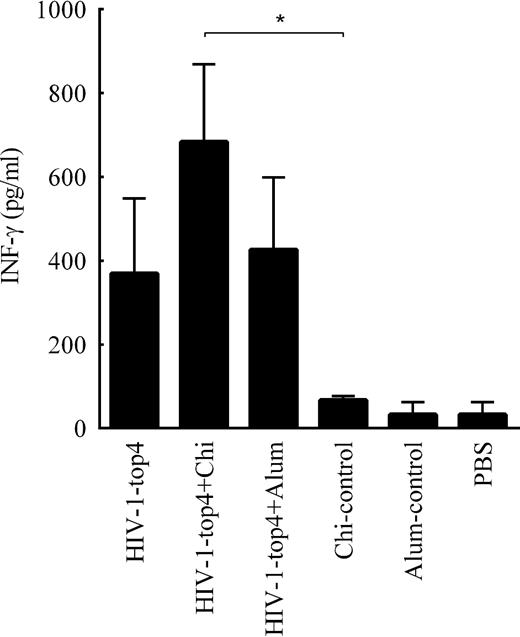
Evaluation of the cellular immune responses against the HIV-1-top4 based candidate vaccines. IFN-γ production in isolated splenocytes from the mice immunized with the recombinant HIV-1-top4 protein, alone and formulated whit adjuvants chitosan and alum, was assayed in the cell culture supernatants using cytokine assay (ELISA). Data are mean of triplicate samples (P < 0.05).
T-Lymphocyte cytotoxicity assay
Measuring of the HIV-1-top4-specific cytotoxic T-lymphocytes (CTL) induced by the candidate vaccines was carried out by LDH releasing evaluation. Two weeks after the last immunization, the single cell splenocytes from the vaccinated mice were co-cultured with the target cells (SP2) for 5 h. As shown in Fig. 4, immunization with the HIV-1-top4 + chitosan candidate vaccine has significantly increased CTL activities in the vaccinated mice compared to other groups (P < 0.05).
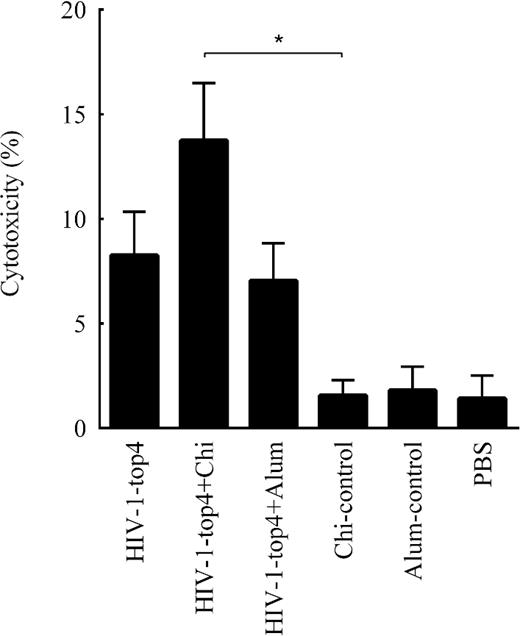
Cytotoxic responses elicited by the HIV-1-top4-based candidate vaccines (HIV-1-top4 alone, HIV-1-top4 + chitosan and HIV-1-top4 + alum) as measured by the LDH release after 5 h incubation using the LDH assay. The results were calculated as percentage of the specific cytolysis as mentioned in ‘Materials and Methods’ section, while Triton X-100 lysed cells served as the positive control (P < 0.05).
Determination of the specific IgG1 and IgG2a isotypes
Determination of the specific IgG isotypes (IgG1 and IgG2a) against the HIV-1-top4 antigen was carried out by the ELISA method. For this end, mice were subcutaneously immunized with the HIV-1-top4 protein alone, HIV-1-top4 + chitosan and HIV-1-top4 + alum on days 0, 14 and 28, respectively. Elicited humoral immune responses were evaluated 1 week after the last injection. Assessment of the specific IgG1 and IgG2a antibody titers in the sera of experimental groups revealed that the immunization of mice with the candidate HIV-1-top4-based vaccines resulted in significant increases in both antibody titers in the vaccinated mice against the control groups (P < 0.05) (Fig. 5A and B). There were no significant differences in the antibodies levels between the vaccinated mice with the HIV-1-top4 protein alone, HIV-1-top4 + alum and HIV-1-top4 + chitosan.
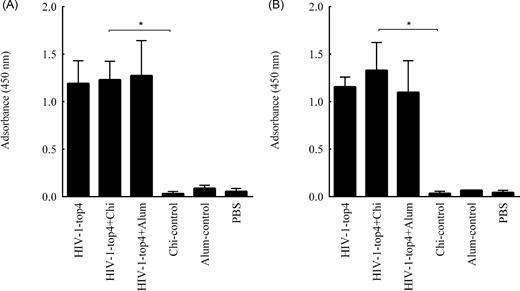
Evaluation of humoral immune responses against the HIV-1-top4-based candidate vaccines. Serum samples from individual immunized mice were collected 1 week after the third and final immunization, and the presence of IgG subclasses (including IgG1 (A) and IgG2a (B)) antibodies was detected with an optimized indirect ELISA as mentioned in ‘Materials and Methods’ section (P < 0.05).
Assessment of Th polarization
The IgG1 (Th2 hallmark) and IgG2a (Th1 hallmark) subclass responses generated by the candidate vaccines were used to calculate the Th1:Th2 indexes according to the formula of ‘IgG2a/IgG1’ (Liu et al.2013). The results revealed that the mice vaccinated with the HIV-1-top4 protein alone, HIV-1-top4 + chitosan and HIV-1-top4 + alum had the Th1:Th2 indexes 0.969, 1.044 and 0.891, respectively (Table 2). This indicates that the candidate vaccines HIV-1-top4 alone and HIV-1-top4 + alum induced Th2-polarized antibody responses in the vaccinated mice (humoral immunity) while the HIV-1-top4 candidate vaccine adjuvanted with chitosan induced Th1-polarized antibody responses in the immunized mice (cellular immunity). Therefore, the results suggest that chitosan adjuvant may have effects on shaping Th1 polarization induced by the HIV-1-top4 protein vaccines which is in a good agreement with CTL, lymphocyte proliferation and INF-γ production responses.
Th polarization. Th-polarization responses in the vaccinated mice were determined according to the value of IgG2a/IgG1 (Th1:Th2). All experiments were repeated three times.
. | HIV-1-top4 protein alone . | HIV-1-top4 protein + chitosan . | HIV-1-top4 protein + alum . |
---|---|---|---|
IgG1 | 1.192 | 1.231 | 1.274 |
IgG2a | 1.155 | 1.33 | 1.097 |
Th1/Th2a | 0.969 | 1.080 | 0.861 |
. | HIV-1-top4 protein alone . | HIV-1-top4 protein + chitosan . | HIV-1-top4 protein + alum . |
---|---|---|---|
IgG1 | 1.192 | 1.231 | 1.274 |
IgG2a | 1.155 | 1.33 | 1.097 |
Th1/Th2a | 0.969 | 1.080 | 0.861 |
A value of the index above 1 shows a shift of the immune response toward the Th1 type (cellular immunity), whereas the value of the index below 1 means a shift of the immune response toward the Th2 type (humoral immunity).
Th polarization. Th-polarization responses in the vaccinated mice were determined according to the value of IgG2a/IgG1 (Th1:Th2). All experiments were repeated three times.
. | HIV-1-top4 protein alone . | HIV-1-top4 protein + chitosan . | HIV-1-top4 protein + alum . |
---|---|---|---|
IgG1 | 1.192 | 1.231 | 1.274 |
IgG2a | 1.155 | 1.33 | 1.097 |
Th1/Th2a | 0.969 | 1.080 | 0.861 |
. | HIV-1-top4 protein alone . | HIV-1-top4 protein + chitosan . | HIV-1-top4 protein + alum . |
---|---|---|---|
IgG1 | 1.192 | 1.231 | 1.274 |
IgG2a | 1.155 | 1.33 | 1.097 |
Th1/Th2a | 0.969 | 1.080 | 0.861 |
A value of the index above 1 shows a shift of the immune response toward the Th1 type (cellular immunity), whereas the value of the index below 1 means a shift of the immune response toward the Th2 type (humoral immunity).
DISCUSSION
One of the new approaches to produce more effective HIV-1 vaccines is designing and producing of synthetic polyepitope HIV-1 immunogens, using conserved epitopes and improving of their delivery strategies. According to the literature, multiepitopic DNA vaccines could induce T-cell responses in immunized mice (Cafaro et al.2015; Fernández-Tejada, Haynes and Danishefsky 2015). Mothe et al. (2011) designed a novel HIV-1 vaccine and showed that conserved but subdominant epitopes induced strong cellular responses that could inhibit the replication of multiple HIV-1 variants in viral inhibition assay. Reguzova et al. (2015a,b) also demonstrated that DNA vaccine constructs expressing artificial HIV-1 polyepitopic T-cell immunogens induced cellular responses following BALB/c mice immunization. This approach theoretically enables overcoming high antigenic variation of HIV-1. Moreover, it focuses immune responses on protective determinants and extends the range of production of neutralizing antibodies due to mammalian polyclonal responses. However, there may be variety of epitopes that can be protective and overlooked. Besides, using of some immunodominant epitopes could convey the immune responses to themselves which maybe decreases the efficacy of polyepitope vaccine (Karpenko et al.2014).
Several studies revealed that vaccines incorporated from the viral structural and regulatory proteins show more immunogenicity compared to those composed of either HIV-1 structural or regulatory proteins alone (Mahdavi et al.2009). So far, different structural and regulatory proteins of HIV-1 have been used as target antigens to create polyepitope vaccines. For example, Mothe et al. (2011) produced a polytopic HIV-1 vaccine including epitopes of Gag, Pol, Vif and Nef proteins and accounted this candidate vaccine decreased the viral loads (Mothe et al.2011). According to the literature, the HIV-1 proteins Tat, Pol, Gag and Env are among the most suitable candidates to develop safe and effective HIV/ADIS vaccines (Jafarpour et al.2014; Alipour, Mahdavi and Abdoli 2017). Jafarpour et al. (2014) showed that the HIV-1 polyepitope DNA vaccine (HIV-1-tat-pol-gag-env) could efficiently induce both immune system arms in the vaccinated BALB/c mice. These proteins play critical roles in the HIV-1 life cycle and pathogenesis. Therefore, their inhibition would considerably decrease the virus infectivity at different steps (Jafarpour et al.2014). Blocking the HIV-1 Tat and Pol proteins greatly reduce the viral pathogenesis process and targeting the Gag protein has been effective in controlling the progression of AIDS (Jafarpour et al.2014). The HIV-1 Env protein has a key role in the attachment of the virus to T-lymphocytes and the initiation of infection; hence, the induction of immune responses against this viral protein may be important for HIV-1 neutralization (Jafarpour et al.2014).
On the other hand, it has been documented that adjuvants can reinforce immunogenicity and efficacy of recombinant vaccines and addition of potent adjuvants to vaccines’ formulations may also boost and shape antigen-specific antibody responses and help to antigen dose sparing (O’Hagan 2001). Mahdavi et al. employed the p24-Nef fusion peptide with complete and incomplete Freund's adjuvants. They showed that the vaccines formulated with the adjuvant elicited higher both humoral and cellular immune responses compared to the non-adjuvanted vaccines (Mahdavi et al.2010).
Some adjuvants, such as alum and chitosan, due to their particular nature, can be associated with antigens and protect them against degradation, and therefore, allow them to be present for extended periods of time (O’Hagan 2001). It seems that the formulation of novel polyepitope vaccines containing vital epitopes from the major structural and regulatory proteins of HIV-1 with potent adjuvants can be a suitable approach to increase the HIV-1 vaccines efficacies and elicit stronger immune responses in vaccinated mice (Arabi et al.2014). Abdoli et al. (2017) reported that conjugated anionic PEG-citrate G2 dendrimer with multiepitope HIV-1 candidate vaccine elicited the efficient cellular immune responses in the immunized mice.
Along with other ongoing efforts to evaluate HIV/AIDS vaccines, in this study we investigated the induction of both cellular and humoral immune responses elicited by the HIV-1-top4 candidate vaccines formulated with chitosan and alum adjuvants in BALB/c mice and the results were compared to each other and those obtained from the mice vaccinated with the HIV-1-top4 recombinant protein alone. Since the route of vaccine administration is determinant to its efficacy and it has been reported that subcutaneous injection can stimulate both cellular and humoral immune responses (Mann et al.2014), we vaccinated mice subcutaneously with the candidate vaccines.
As mentioned before, induction of cellular immune responses is an important factor that increases candidate vaccine efficiency against HIV-1 (Fouts et al.2015). To evaluate the elicitation of cellular arm of the immune system, induction of lymphocyte proliferative responses, secretion of cytokine IFN-γ and the cytotoxic activity of CTLs were measured as critical aspects of cellular immune responses. The results of lymphocyte proliferation responses revealed while all candidate vaccines (HIV-1-top4 alone, HIV-1-top4 + alum and HIV-1-top4 + chitosan) efficiently elicited T-cell proliferative responses, chitosan adjuvanted HIV-1-top4 vaccine induced higher proliferative responses than the HIV-1-top4 protein alone or HIV-1-top4 adjuvanted with alum (Fig. 2). Secretion of the IFN-γ cytokine is considered as a hallmark of Th1 cell-mediated immune response. It has been shown that the reduced levels of this cytokine are highly relevant to the disease progression to AIDS (Mahdavi et al.2009, 2010). Our results showed that the responses induced by the chitosan adjuvanted HIV-1-top4 vaccine were higher than the other candidate vaccines and the mean difference was also significant (Fig. 3). Finally, the ability of a candidate vaccine to induce strong CTL responses is another important feature of an ideal vaccine against HIV-1 (Mahdavi et al.2010). Indeed measuring the activity of LDH released from the HIV-1 infected cells is considered as one of the suitable procedures to evaluate CTL cytotoxic responses since they are specially recognized and killed by CTLs. According to our results, although all of the HIV-1-top4-based candidate vaccines (with or without adjuvant) were efficiently able to induce cytotoxic responses, the HIV-1-top4-based antigens formulated with chitosan adjuvant elicited significantly stronger CTL activity compared to the other groups (Fig. 4).
Induction of humoral responses is another important characteristic of an efficacious vaccine against HIV-1 (Fouts et al.2015). Specific serum antibodies (especially against gp120 and gp41) could be effective in preventing HIV-1 infection (Mahdavi et al.2010; Jafarpour et al.2014). According to our results, the mice immunized with HIV-1-top4 candidate vaccines (HIV-1-top4 + chitosan, HIV-1-top4 + alum and HIV-1-top4 alone) induced the secretion of both IgG1 (as the hallmark of Th2 and humoral response) and IgG2a (as the hallmark of Th1 and cellular response) antibodies (Fig.5A and B) that there is no significant difference between them.
Taken together, our results showed while the HIV-1-top4 + chitosan candidate vaccine has elicited similar humoral immune responses to those obtained with the naked protein, it could efficiently boost cellular immune responses in the immunize mice compared to the naked protein. These results are consistent with other studies that reported high immunogenicity of the chitosan adjuvanted vaccines (Arabi et al.2014; Eshghjoo et al.2015; Abdoli et al.2017). It has been revealed that the particle nature of chitosan may culminate the harvesting, processing and presentation of antigens by antigen-presenting cells. Moreover, its positive charge could increase antigens’ uptake that finally increase candidate vaccines’ immunogenicity.
Finally, the shift of the cytokine pattern is also considered as an important factor in the induction of protective responses to HIV-1 infection (Riou et al.2014). Cytokine profile analysis of our candidate vaccines (Table 2) showed that the chitosan adjuvanted HIV-1-top4 vaccine could shift immune responses to Th1 immune response that plays an important role in viral infections such as HIV-1, and this profile facilitates the induction of cellular immunity against viral pathogens (Liu et al.2013).
CONCLUSION
Since HIV/AIDS continues to be a major global public health issue and priority, it is necessary to develop new efficient and alternative approaches for overcoming or reducing the limitations of the current vaccines. One of the new approaches to elicit stronger immune responses is design and construction of synthetic polyepitope HIV-1 vaccines by incorporating important conserved T- and B-cell epitopes that can totally elicit broader responses of neutralizing antibodies as well as cytotoxic and helper T-lymphocytes. The addition of various potent adjuvants to vaccines’ formulations is also considered as another suitable approach to reinforce the immune responses induced by the administered antigens. Introducing new generation of adjuvants may also help to develop a new generation of vaccines. The combination of these approaches, thus, may have efficient impacts on the elicitation of stronger cellular and humoral immune responses. In this regard, the results of this study revealed that while all recombinant polytope candidate vaccines could simultaneously induce efficient humoral and cellular immune responses compared to the control groups, chitosan adjuvant could efficiently boost the cellular immunity responses against the HIV-1-top4 + chitosan candidate vaccine and help to shift the immune responses toward cellular immunity in the vaccinated mice indicating the adjuanticity of this compound.
Further studies considering the cellular and humoral aspects of this novel HIV-1 candidate vaccine formulation could be held to further pursue the evaluation of its efficiency and the findings can provide guidance for future studies.
Acknowledgements
The authors deeply thank the research council of the Institute for Advanced Studies in Basic Sciences for its support during the course of this project.
Conflict of interest. None declared.