-
PDF
- Split View
-
Views
-
Cite
Cite
Farah Deeba, Asimul Islam, Syed Naqui Kazim, Irshad Hussain Naqvi, Shobha Broor, Anwar Ahmed, Shama Parveen, Chikungunya virus: recent advances in epidemiology, host pathogen interaction and vaccine strategies, Pathogens and Disease, Volume 74, Issue 3, April 2016, ftv119, https://doi.org/10.1093/femspd/ftv119
- Share Icon Share
The Chikungunya virus is a re-emerging alphavirus that belongs to the family Togaviridae. The symptoms include fever, rashes, nausea and joint pain that may last for months. The laboratory diagnosis of the infection is based on the serologic assays, virus isolation and molecular methods. The pathogenesis of the Chikungunya viral infection is not completely understood. Some of the recent investigations have provided information on replication of the virus in various cells and organs. In addition, some recent reports have indicated that the severity of the disease is correlated with the viral load and cytokines. The Chikungunya virus infection re-emerged as an explosive epidemic during 2004–09 affecting millions of people in the Indian Ocean. Subsequent global attention was given to research on this viral pathogen due to its broad area of geographical distribution during this epidemic. Chikungunya viral infection has become a challenge for the public health system because of the absence of a vaccine as well as antiviral drugs. A number of potential vaccine candidates have been tested on humans and animal models during clinical and preclinical trials. In this review, we mainly discuss the host–pathogen relationship, epidemiology and recent advances in the development of drugs and vaccines for the Chikungunya viral infection.
INTRODUCTION
The name Chikungunya was derived from a ‘Makonde’ word that means ‘one which bends up’; it refers to the posture of the patient due to extreme joint pain. The symptoms of Chikungunya virus (CHIKV) infection are high fever, rashes, nausea, arthralgia, myalgia etc. (Schuffenecker et al. 2006). The Ades aegepti mosquito is the main vector involved in the transmission of the disease. However, the actual burden of Chikungunya viral infection is underestimated because of its overlapping symptoms with dengue fever.
The Chikungunya virus has caused many outbreaks worldwide in the last decade, including the Indian Ocean region, African countries and American continent. During the 2004–09 epidemic the CHIKV was found to infect another species of mosquito, i.e. Aedes albopictus due to a single point mutation in the genome (Schuffenecker et al. 2006). This severe outbreak occurred in 2004 in the Indian Ocean region including India. CHIKV infection is affecting the socioeconomic status of various geographical regions because of the absence of an effective vaccine and antiviral drugs. Chikungunya viral infection is a major health concern in India also due to the presence of a large immunologically naive population. Therefore, regular surveillance for the Chikungunya viral infection should be done in the endemic regions such as India. This will assist in the prediction and control of outbreaks. In addition, the generation of awareness among the general public about the disease is essential for its effective control and management.
GENOME
CHIKV is a member of the genus Alphavirus, in the family Togaviridae (Powers et al. 2000). The CHIKV is a small (60–70 nm in diameter) and enveloped virus. The 11.8 kb genome of CHIKV is a linear, positive sense RNA molecule. Figure 1 shows a schematic representation of the genome of CHIKV and its replication in the host cell. Two-thirds of the genome from the 5′ end has a methyl guanylate cap that consists of a non-structural polyprotein precursor. This precursor is cleaved into non-structural proteins (nsp1, nsp2, nsp3 and nsp4) that are involved in the replication of the virus. The nsp1 protein functions as a cytoplasmic RNA capping enzyme, whereas the nsp2 protein has two separate domains with helicase and protease activity. The nsp3 protein is essential for negative strand RNA synthesis. The nsp4 protein works as an RNA-dependent RNA polymerase (Solignat et al. 2009). One-third of the genome from the 3′ end has a polyadenylate tail that consists of 26S mRNA whose products include the structural proteins. The structural proteins of CHIKV are the surface glycoproteins (E1 and E2), capsid protein and small peptides (E3 and 6K) (Weaver et al. 2005; Powers and Logue 2007; Abere et al. 2012). The viral surface is covered with membrane-anchored spikes which are triplets of a heterodimer of envelope E1 and E2 glycoproteins. The E2 is exposed and has a role in viral attachment, whereas E1 is the fusion protein that facilitates the viral entry. The epitopes in the E2 glycoprotein are recognized by neutralizing antibodies and therefore E2 is the vaccine candidate (Brehin et al. 2008). The lineages of CHIKV are reported with different genotypic characteristics. The genotypes are classified on the basis of E1 gene sequence and the full genome of CHIKV. Three genotypes (East, Central and South African (ECSA), West African and Asian) have been described for the Chikungunya virus (Santhosh et al. 2008).
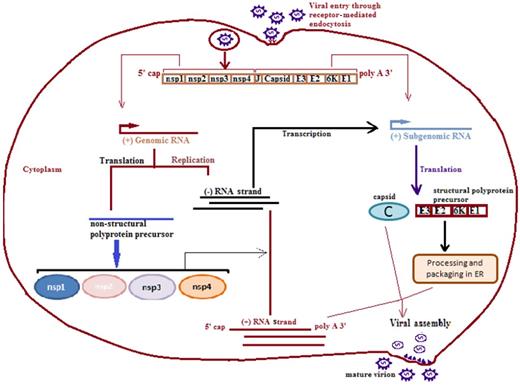
Chikungunya virus replication cycle. The CHIKV enters into the host cell through receptor-mediated endocytosis forming an endosome. The endosome enters the host cell and genome of the virus then replicates in the cytoplasm. The CHIKV genome carries non-translated region (NTR) at both 3′ and 5′ ends and non-coding junction region (J region) between the genes coding for structural and non-structural proteins. The replicated negative strand of RNA is transcribed into positive sense subgenomic RNA, also known as 26S RNA, that serves as mRNA for structural proteins. The genomic and subgenomic RNAs are translated into polyprotein precursors that finally cleave into non-structural proteins (nsp1, nsp2, nsp3 and nsp4) and structural proteins (capsid, E1 and E2). The positive RNA strand, structural proteins and capsid proteins finally assemble into the mature virion. ER, endoplasmic reticulum.
VECTOR AND DISEASE TRANSMISSION
CHIKV is an arbovirus that spreads mainly through Aedes aegypti and Aedes albopictus mosquitoes. The CHIKV life cycle involves: (i) an enzootic sylvatic cycle, and (ii) an epidemic urban cycle. The sylvatic cycle is maintained primarily in African forests involving several arboreal Aedes mosquito species as vectors and non-human primates like monkeys, rodents, birds, etc. as reservoirs (Apandi et al. 2009; Caglioti et al. 2013). It is believed that initially in Africa, the enzootic transmission cycle spilled over and infected nearby human populations (Thonnon et al. 1999; Peyrefitte et al. 2007). The mosquitoes are also involved in inter-human transmission during outbreaks. During epidemics, human beings serve as reservoirs in the urban cycle.
CLINICAL MANIFESTATIONS
The alphaviruses are clinically divided into two subgroups: encephalogenic (e.g. Venezuelan equine encephalitis virus, Western equine encephalitis virus), which infect neurons, and arthritogenic (e.g. Chikungunya virus, Sindbis virus) which are basically associated with polyarthritis and rashes. The infection with CHIKV is followed by a silent period of incubation that lasts 2–4 days. The incubation period is followed by an abrupt onset of symptoms, including fever, headache, nausea, myalgia and rashes (Pialoux et al. 2007). The most prominent and typical clinical feature is the polyarthralgia that can sometimes persist for as long as a few months to years (Schilte et al. 2013). Chikungunya viral infection is commonly not found to be associated with mortality. The severity of disease in the case of CHIKV infection is directly attributed to the severe and prolonged symptoms or effects. In some cases lymphopenia, thrombocytopenia and minor hemorrhagic signs such as epistaxis or gingivorrhagia have also been reported (Schuffenecker et al. 2006). During the 2006 outbreak in Reunion Island neurological complications such as meningoencephilitis were also reported in a small proportion of patients (Quatresous 2006). During this epidemic optic neuritis, anterior uveitis and dendritic lesions occurred as primary acute onset inflammatory reactions (Lalitha et al. 2007; Mittal et al. 2007). Certain follow-up studies of patients with Chikungunya viral infection have shown evidence of cardiac complications such as myocarditis (Obeyesekere and Hermon 1973).
Chikungunya viral infection is generally a self-limiting febrile illness. The resolution of CHIKV infection takes place within the first 15 days of illness after which the disease enters a stage where chronic rheumatism remains as the major feature (Manimunda et al. 2010). The symptoms often mimic those of dengue fever and its spread is also common in dengue endemic regions, therefore the two diseases are commonly indistinguishable (Ravi 2006).
DETECTION OF CHIKUNGUNYA VIRUS INFECTION
Diagnosis of Chikungunya viral infection is based upon the clinical symptoms and laboratory diagnosis. Routinely used diagnostic methods include isolation of virus in cell culture, identification of viral RNA by reverse transcriptase (RT)-PCR/real-time PCR and detection of antibodies (IgM/IgG) to the virus. The CHIKV isolation is performed on serum samples collected within the first 7 days of illness. The virus can be isolated in insect and mammalian cell lines (C636, Vero). The cultured virus can be confirmed by RT-PCR or immunofluorescence assay. Virus isolation is time consuming and needs expertise therefore it is no longer being used for the detection of CHIKV infection. The conventional PCR and real-time PCR assays have been developed targeting the envelope and the non-structural genes of CHIKV. RT-PCR can be used for early identification of the CHIKV infection before detection of the antibodies, i.e. within the first 7–10 days of infection. DNA sequencing coupled with the RT-PCR technique can be used to identify the corresponding genotype. Real-time PCR is used to determine the viral load in the clinical samples. The sensitivity of real-time RT-PCR was found to be around 10-fold higher than conventional RT-PCR and as low as 20 copies of RNA transcript can be identified by real-time RT-PCR (Edwards et al. 2007).
The diagnosis of the CHIKV infection can also done by detection of the immune response by serological assays. The immunoassays for IgM/IgG (ELISA) and indirect immunofluorescent assay (IFA) are used for detection of antibodies to the virus. The indirect immunofluorescent assay is also a method for the detection of Chikungunya virus in the clinical samples (Yergolkar et al. 2006). The IgM antibodies develop within 2–3 days after the onset of symptoms of infection and may persist for several months (Sam and AbuBakar 2006; Malvy et al. 2009). The IgG antibodies develop in the convalescent phase and may persist for several months to years (Cavrini et al. 2009). The sensitivity and specificity of serological assays are poorly established because of the possibility of false positive results due to cross reactivity with antibodies to dengue or other arboviruses (Pialoux et al. 2007). Therefore, limitations with detection of the Chikungunya viral infection by IgM capture ELISA exist. Recently a study has tried to improve the ELISA by developing antigen for the assay using Eilat virus instead of the Chikungunya virus (Erasmus et al. 2015). The antigen that was developed does not require biosafety containment facilities and can be used for ELISA without inactivation. However, this assay needs to be tested and evaluated before its implementation on clinical samples.
HOST–PATHOGEN INTERACTIONS
The Chikungunya virus first replicates in the skin fibroblasts after inoculation into the skin of humans through the mosquito bite. Only fibroblasts of the skin have been shown to be susceptible to CHIKV infection amongst various types of cells (Talarmin et al. 2007; Robin et al. 2009). Once replicated in the skin, it further disseminates through blood to liver, muscle tissues, joints, lymph nodes and brain (Fig. 2). On reaching muscles, the Chikungunya virus replicates in muscle satellite and fibroblast cells and not in muscle fibres (Ozden et al. 2007). The CHIKV replicates in joint fibroblasts and the synovial tissues (Hoarau, Jaffar Bandjee and Krejbich Trotot 2010). In addition, the Chikungunya virus RNA and proteins have been identified in the synovial tissues during severe and persistent arthralgia (Parola et al. 2006; Manimunda et al. 2007; Ozden et al. 2007; Hoarau, Jaffar Bandjee and Krejbich Trotot 2010).
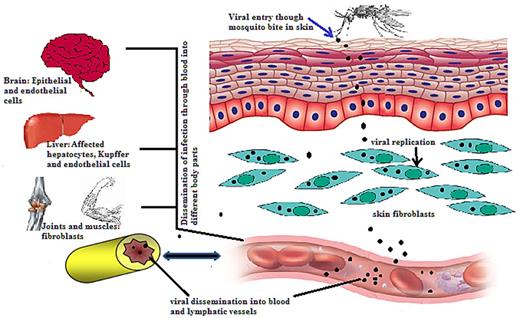
A schematic representation of the systemic Chikungunya virus dissemination. The Chikungunya virus enters the host cell by the mosquito bite. The virus then replicates into fibroblasts of skin and enters nearby lymph nodes through which it disseminates into the blood. The Chikungunya virus further migrates to different organs and tissues through blood vessels.
When the virus disseminates to brain it seems to infect the lining of the choroid plexus of stromal cells of the nervous system. The CHIKV tissue tropism studied in a mouse model was found to match with the tissue tropism reported in the in vitro system. The onset of symptoms coincides with a rise in viral titer. Thus activation of the innate immune response occurs, which results in the production of type I interferons as the primary response. The type I interferons are mainly involved in the innate immune responses against viral infections. These interferons are known to induce the expression of interferon-stimulated genes (ISGs) having antiviral, antiproliferative and immune-modulatory functions (Kumar et al. 2012). The immune response elicited by CHIKV involves the production of many cytokines, chemokines and growth factors. The levels of many cytokines (INF α, CXCL9, CXCL10, CCL2 (MCP-1), IL6 and INF γ) were found to be higher in the acute phase and their level decreased with the convalescent phase (Fig. 3) (Rulli et al. 2011; Wauquier et al. 2011). Interferon α (INF α) is detectable even on the first day of infection and its level correlates with the plasma viral load (Dupuis-Maguiraga et al. 2012). The high level of CXCL9, CXCL10, CCL2 and IL6 in the acute phase indicates inflammation initiating the adaptive T cell immune response. The CXCL9 and CXCL10 are also part of the chemokine program regulating the migration of monocytes/macrophages, natural killer cells and memory T cells (Deshmane et al. 2009). The chemokines are crucial mediators of innate as well as adaptive immune responses against various viral infections (Rot and von Andrian 2004). Both CXCL9 and CXCL10 are induced by INF γ and work as a chemo-attractant for T cells. Both these chemokines signal through a common receptor, i.e. CXCR3. Therefore, they may have a role in activation of T cells also, as CXCR3 identifies the T cell subsets (Qin et al. 1998). The CCL2 is also referred to as monocyte chemotactic protein 1 (MCP-1) that leads monocytes, memory T cells and dendritic cells to the sites of inflammation.
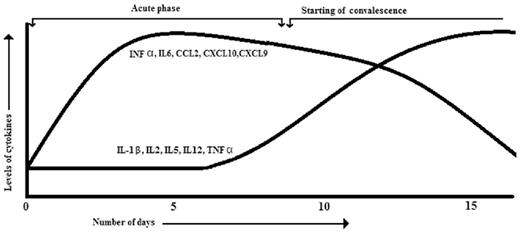
A graphical representation showing levels of various cytokines and chemokines after CHIKV infection. The graph shows an increase and decrease in levels of different cytokines with respect to the onset of symptoms and its resolution.
IL6 is an immune mediator of fever that activates muscle metabolism so as to increase body temperature (Cronstein 2007). The levels of granulocyte macrophage colony-stimulating factor (GM-CSF) receptor and IL6 levels in plasma were significantly higher in patients with persistent arthralgia (Chow et al. 2011). IL6 is a pleotropic cytokine and has a destructive role in rheumatoid arthritis due to which joint inflammation and pain occur (Cronstein 2007; Schaible et al. 2010). The increased levels of IL6 may also contribute to the severe joint pain in the CHIKV infection. The IL6 is also a major B cell growth factor (Hirano et al. 1985) and a known inducer of IgG3 (Kawano, Noma and Yata 1994). The high IgG3 titer is induced by high viremia and is also correlated with the higher IL6 level. An early increase of IgG3 levels was found to be associated with efficient viral clearance in vivo (Kam et al. 2012). The higher level of C-reactive protein (CRP) is activated by higher IL6 levels. Higher CRP levels, in turn, activate macrophage-mediated phagocytosis, lymphopenia (very low levels of lymphocytes), lower monocyte level, neutrophilia and high viral load (Chow et al. 2011). Eighty percent of the individuals infected with CHIKV were found to have lymphopenia. These patients experienced a significantly lower level of circulating B and T lymphocytes (Borgherini et al. 2007). Such low levels of lymphocytes cannot be directly due to the infection, but it is the higher levels of type I INFs that induce cell death in lymphocytes (Schwartz and Albert 2010). The elevated level of RANTES (Regulated on activation, normal T cell expressed and secreted) is observed during many viral infections. Likewise, the elevated level of RANTES is also reported during dengue viral infection. An investigation by Ng and colleagues observed that the level of RANTES decreased significantly during the Chikungunya virus infection. Therefore, a decrease in the level of RANTES can be considered a biomarker to differentiate between the Chikungunya and dengue infections since both these viral infections have overlapping symptoms (Kelvin et al. 2011).
The onset of the adaptive immune response through activation and proliferation of CD8+ T cells occurs in the early stage of infection. The percentages of CD3+ and CD8+ lymphocytes were found to be significantly higher in patients infected with CHIKV on days 0, 1 and 2 in comparison with healthy controls (Wauquier et al. 2011). The level of cytokines such as TNF-α, IL-1β, IL2, IL5 and IL12 were found to increase with patient recovery (Fig. 3) (Ng et al. 2009; Chow et al. 2011). TNFα and IL-1β play a major role in inflammatory diseases and contribute mainly to the joint pain in diseases like rheumatoid arthritis. However, in Chikungunya viral infection the levels of TNFα and IL-1β were found to increase in the convalescent phase and did not significantly differ in patients reporting mild or severe conditions (Ng et al. 2009). This indicates no or a mild contribution of TNFα and IL-1β in persistent arthralgia in CHIKV patients.
EPIDEMIOLOGY
The CHIKV is believed to have originated in Africa and then spread to all over the world, causing many epidemics and affecting millions of people (Powers et al. 2000). The geographical distribution of CHIKV commonly includes tropical countries, but the viral infection also extended into the temperate regions after the 2004 epidemic (Robinson 1955). It was first reported from East Africa around Tanzania during an epidemic of fever in 1952–53. This fever was later described in 1955 as a ‘dengue like disease’ (Lumsden 1955; Sergon et al. 2008). After the first report, the Chikungunya virus infection was documented worldwide involving many countries including Tanzania, Ghana, Mozambique, Burkina Faso, Comoros, Kenya, Central African Republic, Benin, Uganda, Nigeria, Zimbabwe, Senegal, South Africa, Cameroon, Congo, Guinea, Mayotte, Burundi, Sudan, Malawi, Namibia, Gabon, Sri Lanka, Myanmar, Thailand, Vietnam, Indonesia, Malaysia, Taiwan, Laos, Philippines, Pakistan, Singapore, Cambodia, India, China, Sri Lanka, etc. Indian Ocean regions with reported cases are La Reunion, Madagascar, Mauritius, Seychelles etc. (Hammon, Rudnick and Sather 1960; Shah, Gibbs and Banerjee 1964; Thaung et al. 1975; Thonnon et al. 1999; Lam et al. 2001; Muyembe-Tamfum et al. 2003; Laras et al. 2005; Schuffenecker et al. 2006; Lanciotti et al. 2007; Renault et al. 2007; Cavrini et al. 2009). Chikungunya has been reported to re-emerge unexpectedly in many countries with a gap of 7–20 years (Powers et al. 2000). The first Asian outbreak was documented in 1958 in Bangkok and Thailand (Jupp and McIntosh 1988), after which there were outbreaks in many parts of India in 1963–65. Later on re-emerging epidemics were documented in 1999–2000 in Gabon affecting around 50 000 people (Pastorino et al. 2004). A subsequent epidemic was reported from Indonesia in 2001–03 (Laras et al. 2005).
The most recent re-emerging epidemic was reported from the Indian Ocean region, including India, from 2004 to 2009 that affected millions of people in various countries. This epidemic in the Indian Ocean region was believed to have originated from Kenya. Strains of this outbreak formed a separate clade in the ECSA genotype that is known as Indian Ocean Lineage (IOL) (Yergolkar et al. 2006; Volk et al. 2010; Weaver and Forrester 2015). Thus, this epidemic provided a genotypic shift of the CHIKV from Asian to ECSA genotype in the Indian Ocean region. This ECSA genotype has been reported in the subsequent epidemiological studies from this region (Vashishtha et al. 1998; Arankalle et al. 2007). A unique molecular signature of this outbreak was the E1 A226V mutation that was absent in the initial phase of the epidemic, but later on was found in almost 90% of the isolates (Schuffenecker et al. 2006). Due to this mutation the virus was able to infect A. albopictus, which was not the usual host of CHIKV until this epidemic. The mutation A226V in the E1 protein was shown to provide cholesterol independence to a Semliki Forest virus population (Tsetsarkin et al. 2007). The ability of a virus to infect insect cells by providing a better adaptation to the lipid composition of these cells could be improved by the mutation that affects cholesterol dependency. The experimental demonstrations showed that viruses without the E1 A226V mutation were not as successful at replicating in A. albopictus (Vazeille et al. 2007). On the other hand, the mutated ones had a higher efficiency of replication and dissemination in A. albopictus (Parola et al. 2006). Therefore, this mutation was attributed to the higher epidemic potential of the virus during this epidemic. Since A. albopictus has a long life span and is active all day long, the spread of CHIKV infection became rapid and reached many more geographical regions than expected. The overall geographical distribution of the Aedes mosquitoes has changed since this outbreak. The Aedes albopictus is now spreading to regions that were earlier inhabited by the Aedes aegypti. Consequently, the circulation patterns of the Chikungunya virus, as well as the dynamics of the epidemics caused by this virus, are expected to be modified in the future.
The first outbreak of Chikungunya virus infection in India took place in 1963–64 in Kolkata (Shah, Gibbs and Banerjee 1964). Subsequent epidemics were reported from many parts of India, including Chennai, Pondicherry, Vellore, Visakhapatnam, Rajmundry, Kakinada and Nagpur in 1964–65 (Rao 1966; Rodrigues et al. 1972) and Barsi in 1973 (Padbidri and Gnaneswar 1979) affecting millions of people. The virus was postulated to have disappeared from India and South East Asia after 1973 (Burke, Nisalak and Nimmannitya 1985; Neogi et al. 1995; Bhatia and Narain 2009), but during 2004 it suddenly re-emerged as an explosive epidemic in India affecting around 1.3 million people in 13 different states after a gap of almost 32 years (Wauquier et al. 2011). Several states in India experienced massive outbreaks of Chikungunya viral infection during 2004–09, including Andhra Pradesh, Karnataka, Maharashtra, Rajasthan, Gujarat, Tamilnadu, Orissa and Madhya Pradesh (Cavrini et al. 2009). Subsequent smaller outbreaks of Chikungunya viral infection have been reported from many parts of India after the massive 2004–09 epidemic. The main affected regions were Delhi, Haryana, Kolkata, Kerala, Andaman and Nicobar Island (Pialoux et al. 2007; Niyas et al. 2010; Singh et al. 2010; Shrinet et al. 2012; Afreen et al. 2014). These studies have reported 8–45% CHIKV positivity using different diagnostic methods, including PCR, real-time PCR and serological assays.
A detailed investigation of the evolution of Chikungunya virus strains in India from 1963 to 2006 was carried out during the massive outbreak. The phylogenetic characterization showed that the strains identified during 1963–73 clustered into the Asian genotype. All the isolates identified during the 2004–09 epidemic clustered into the ECSA genotype making a separate group, IOL (Indian Ocean Lineage) (Arankalle et al. 2007). Another investigation clustered the CHIKV strains in the ECSA genotype by phylogenetic analysis of the E2 protein gene during another small outbreak in Odisha in 2010–11 (Sahu et al. 2013). Various mutations were also identified in the E2 protein. The T cell and B cell epitopes were also predicted for the E protein and two of them were found to be associated with antigenicity. Structural characterization by homology modeling revealed three domains (A, B and C) in the E2 protein. Most of the mutations were identified in the domain B, which modulates the binding of virion with the host cell, and domain C is the transmembrane domain. The most probable B and T cell epitopes were identified in the conserved domain A.
An investigation of full genome analysis of five isolates from Pune during the 2004–09 epidemic identified numerous mutations throughout the genome along with the typical A226V mutation (Arankalle et al. 2007). Comprehensive full genomic analysis of two outbreak strains from Gwalior revealed the presence of three unique mutations, two in the E1 protein and one in the nsp1 protein (Santhosh et al. 2008). These unique molecular features, including the A226V mutation in the E1 protein, were found to be associated with high evolutionary and epidemic potential of these CHIKV strains. Full genome analysis of the six isolates of these outbreak strains from Kerala revealed the presence of 37 novel mutations (Sreekumar et al. 2010). At least 20 mutations mapped to the functionally significant domains of the corresponding viral proteins. They also concluded that 18 mutations in the structural proteins affected the T cell epitope immunogenicity. Another investigation from Pune studied the evolution of the CHIKV during the massive epidemic using 27 full genomes and partial E1 gene datasets. The mean substitution rate of the CHIKV was calculated to be 8.8 × 104 substitutions site−1 year−1 (Cherian et al. 2009). The evolutionary time scale of the CHIK viruses was estimated to be 300 years old. In addition, the progenitor of the 2005–07 viruses was identified 9 years ago that originated in Central Africa.
Numerous phylogenetic studies have also provided insights into the molecular evolution of Chikungunya virus in India (Santhosh et al. 2008). Comparison of synonymous and non-synonymous substitution rates in different genomic regions suggested purifying selection pressures (Arankalle et al. 2007; Sahu et al. 2013). This analysis also revealed the presence of many positively selected sites in the genome. An investigation from Pune identified 10 positively selected sites throughout the genome (Arankalle et al. 2007). Five positively selected sites were identified in the E2 region in most of the isolates in a study from Odisha (Sahu et al. 2013). However, the affect of these positively selected sites on the pathogenesis of Chikungunya virus infections should be investigated further. The phylogenetic investigations from different parts of India have thus contributed to the understanding of the molecular evolution and epidemiology of this re-emerging viral pathogen. Therefore, regular surveillance for the Chikungunya viral infection should be carried out in endemic regions like India. The data generated from the surveillance will assist in the formulation of control measures to prevent outbreaks in this region.
PREVENTION AND CONTROL
Vector control is the only way to reduce CHIKV infections due to the absence of an effective vaccine and antiviral drugs. Prevention of mosquito breeding can be achieved by elimination of stagnant water in drains, room air coolers, broken bottles, tin cans, etc. Insecticides should be used to control the adult and larval mosquito population. Protection from mosquito bites can be achieved by using mosquito-repellent creams, wearing clothes which cover the body, use of mosquito nets over the beds and the use of wire mesh on doors and windows. Therefore, vector surveillance should be carried out in the endemic areas for effective control of infection. In addition, the general public should be educated on various aspects of the disease that will assist in better management of this viral infection.
THERAPEUTICS AND CURE
No antiviral drugs are available for CHIKV infections, therefore the treatment is completely symptomatic. Antipyretics, analgesics and anti-inflammatory drugs are usually given for the treatment of fever, pain, etc. Attempts are being made to develop therapeutics and drugs for the treatment of CHIKV infection by various scientists all over the world. Chloroquinone has antiviral activity against many viruses, including CHIKV, but a recent study from India showed that chloroquinone was not effective in the treatment of early musculoskeletal pain and arthritis in acute CHIKV infection compared with the non-steroidal anti-inflammatory drug (NSAID) e.g. meloxicam (Chopra, Saluja and Venugopalan 2014). Ribavarin also has antiviral activity against Chikungunya virus. Ribavarin in combination with INF-α2b showed a synergistical antiviral effect against Chikungunya viral infection in in vitro conditions (Briolant et al. 2004). Another study of the combination of ribavarin with doxycycline showed a decrease in CHIKV replication in cell culture and decreased viral load and inflammation in mice models (Rothan et al. 2015). Another broad spectrum antiviral drug, favipiravir, was shown to inhibit replication of different Chikungunya virus strains in cell cultures in a recent investigation (Delang et al. 2014). The mice experiments with favipiravir protected mice from severe neurological disease and a decrease in mortality rate was also observed.
The use of human immunoglobulins purified from convalescent phase CHIKV patients proved to have a good prophylactic and therapeutic efficacy against CHIKV infection in mouse models (Couderc et al. 2008). In one particular study two different monoclonal antibodies, 5F10 and 8B10, were prepared against CHIKV. These antibodies were formulated by activation, immortalization, amplification and cloning of B cells from a donor with a history of CHIKV infection. Both these antibodies specifically neutralize CHIKV infection strongly in in vitro conditions (Warter et al. 2011). The level of MCP-1 was found to be elevated during the CHIKV infection. Therefore, one particular study demonstrated that treatment of infected mice with bindarit, which is an inhibitor of MCP-1 synthesis, showed symptoms that were mild and of short duration. The histology of joint and muscle tissue of infected mice treated with bindarit also showed very low inflammation compared with the untreated mice (Hoarau, Jaffar Bandjee and Krejbich Trotot 2010). Trials of antiviral therapy using RNA interference-mediated inhibition of viral replication are promising but sequence dependent. A reduction of CHIKV titer up to 99.6% in specially designed small interfering (si)RNA for conserved regions of nsp3 and E1 gene was reported in an in vitro study. Complete inhibition of viral replication targeting ns1 and E2 protein genes in in vivo conditions was reported in another investigation (Dash et al. 2008; Parashar et al. 2013).
VACCINES
The recurring epidemics, re-emergence and widespread global distribution of CHIKV have necessitated the development of an effective vaccine. Challenges to vaccine development for the CHIKV infection are enormous. The vaccine should induce high levels of antibodies to completely protect against the CHIKV infection. In addition, the vaccine should be economical so that developing countries can easily afford them since most of the epidemics occur in these regions. Many clinical and preclinical trials for development of an effective and economical vaccine are being carried out across the globe (Singh et al. 2013).
WHOLE VIRUS-INACTIVATED VACCINES
The initial vaccine candidate that was developed was a whole virus grown in a green monkey kidney cell line inactivated by formalin (Harrison et al. 1971). The preliminary clinical trials showed good immune response with few side effects. Three different CHIKV strains grown in cell culture that were inactivated by Tween–ether showed good immune response in preclinical trials (Eckels, Harrison and Hetrick 1970).
The formalin-inactivated whole virus vaccine was generated from the ECSA genotype of the 2006 Indian outbreak strain. This vaccine had a very good immunogenic potential to neutralize the infectivity of the virus by enhancing both the humoral and cell-mediated immune response in a mouse model (Tiwari et al. 2009). Another vaccine candidate was also derived from the ECSA genotype of CHIKV. The whole virus was inactivated by β-propiolactone (BPL)–formalin, which proved to be a promising vaccine candidate in the mouse model (Kumar, Sudeep and Arankalle 2012). The inactivated whole virus vaccine derived from an Asian isolate completely protected against mice viremia and arthritis in another study (Gardner et al. 2010).
LIVE ATTENUATED VACCINES
The first live attenuated vaccine strain for CHIKV was developed by Levitt and colleagues in 1986 by passaging a Thailand strain of CHIKV in cell culture (Levitt et al. 1986). The vaccine was able to replicate in both species of the vector A. aegepti and A. albopictus. The live attenuated vaccine was developed by serially passaging plaque-purified CHIKV in the MRC-5 (Human fetal lung fibroblast) cell line. This vaccine was safe and highly immunogenic with side effects well within the tolerable limits. In the phase II trial, investigators observed sero-conversion in 98% of the vaccines by day 28 and the presence of neutralizing antibodies in 85% of volunteers for 12 months (Edelman et al. 2000). CHIKV with point mutations at amino acid numbers 12 and 82 in the E2 glycoprotein proved to be a good candidate for development of live attenuated vaccine in another investigation (Gorchakov et al. 2012).
SYNTHETIC VACCINES
Three different chimeric alphavirus vaccines were developed by using naturally attenuated strains of Venezuelan equine encephalitis virus, Eastern equine encephalitis virus and Sindbis virus as a backbone expressing structural protein genes of CHIKV (Mallilankaraman et al. 2011). An efficient replication of these vaccine candidates was achieved in the cell cultures. In addition, all the vaccine candidates generated a robust immune response in mice. Wang and colleagues developed another recombinant vaccine candidate comprising the non-replicating adenovirus vector expressing the structural proteins of CHIKV (Wang et al. 2011). The vaccine induced a good humoral immune response in mice. In an effort to develop a vaccine against CHIKV infection, Plante and colleagues replaced the subgenomic promoter in a CHIKV clone by internal ribosome entry site (IRES) from encephalomyocarditis virus and thus altered the levels and host-specific mechanism of structural protein gene expression (Muthumania et al. 2008). This vaccine was found to be highly immunogenic and efficacious in mice. In addition, the vaccine was found to be incapable of replicating in the mosquito cells. Another potential vaccine candidate is MVA-CHIKV that is based on modified vaccinia virus Ankara (MVA) expressing all the structural genes of Chikungunya virus (capsid, E3, E2, 6K and E1) (Plante et al. 2011). The trials of the above-mentioned vaccine candidate were carried out on mice that showed both potent and broad cellular immune responses even on administration of high viral doses.
DNA VACCINES
Another approach for the design of a vaccine against Chikungunya virus includes the development of DNA vaccines. During an investigation on DNA-based vaccine, several modifications were made in the consensus sequences of capsid and envelope protein genes of CHIKV that included codon optimization, addition of a Kozak sequence, and a substituted immunoglobulin E leader sequence inserted in a plasmid (Wanga et al. 2008). The vaccine was able to induce both humoral and cellular immune response in mice. Vaccination using envelope-based synthetic DNA vaccine using a combined E1, E2 and E3 gene construct was found to generate a humoral as well as a cell-mediated immune response in rhesus macaques, each of which is capable of providing protection against the CHIKV infection (Garcia-Arriaza et al. 2014).
VIRUS-LIKE PARTICLES
The selective expression of structural proteins (C-E3-E2-6K-E1) of the West African strain of CHIKV forms non-infectious virus-like particles (VLPs) in vitro and showed high titers of neutralizing antibodies both in monkeys and immunodeficient mice (Akahata et al. 2010). The recombinant baculoviruses were used to generate CHIKV VLPs in insect cells in another study (Metz et al. 2013). The VLPs provided protection against viremia and arthritis in the mouse model. The VLPs generated in another recent investigation proved to be a most promising vaccine candidate as the phase I clinical trials showed immunogenic, well-tolerated and safe effects (Chang et al. 2014).
CONCLUSION
The Chikungunya virus is a re-emerging infection affecting millions of people worldwide. The Chikungunya viral infection is associated with a number of challenges such as overlapping clinical symptoms with dengue virus, recurring epidemics, persistent arthralgia and mutations in viral genome leading to enhanced geographical spread and inability to control the vector-mediated transmission of the disease. The recurring and persistent epidemics in different geographical regions have enhanced efforts to develop drugs and vaccines to combat Chikungunya virus infection.
The Chikungunya viral infection is a major health concern in India. Therefore, continuous viral surveillance should be carried out in the regions reporting regular outbreaks in the monsoon season. This surveillance will describe the circulating genotypes of Chikungunya virus in these regions. The information on the circulating strains of Chikungunya virus will assist efforts to develop vaccines and antiviral drugs. These data will also facilitate the prediction and control of the epidemics. In addition, an increase in the general awareness of the disease by educating the general public can be beneficial for a better management of this emerging viral infection.
FUNDING
Work in our laboratory is supported by grants from the University Grants Commission and the Council of Scientific and Industrial Research, Government of India.
Conflict of interest. None declared.
REFERENCES