-
PDF
- Split View
-
Views
-
Cite
Cite
Claudia Vuotto, Ines Moura, Fabrizio Barbanti, Gianfranco Donelli, Patrizia Spigaglia, Subinhibitory concentrations of metronidazole increase biofilm formation in Clostridium difficile strains, Pathogens and Disease, Volume 74, Issue 2, March 2016, ftv114, https://doi.org/10.1093/femspd/ftv114
- Share Icon Share
Resistance mechanism to metronidazole is still poorly understood, even if the number of reports on Clostridium difficile strains with reduced susceptibility to this antibiotic is increasing. In this study, we investigated the ability of the C. difficile strains 7032994, 7032985 and 7032989, showing different susceptibility profiles to metronidazole but all belonging to the PCR ribotype 010, to form biofilm in vitro in presence and absence of subinhibitory concentrations of metronidazole. The quantitative biofilm production assay performed in presence of metronidazole revealed a significant increase in biofilm formation in both the susceptible strain 7032994 and the strain 7032985 exhibiting a reduced susceptibility to this antibiotic, while antibiotic pressure did not affect the biofilm-forming ability of the stable-resistant strain 7032989. Moreover, confocal microscopy analysis showed an abundant biofilm matrix production by the strains 7032994 and 7032885, when grown in presence of metronidazole, but not in the stable-resistant one. These results seem to demonstrate that subinhibitory concentrations of metronidazole are able to enhance the in vitro biofilm production of the above-mentioned PCR ribotype 010 C. difficile strains, susceptible or with reduced susceptibility to this antibiotic, suggesting a possible role of biofilm formation in the multifactorial mechanism of metronidazole resistance developed by C. difficile.
INTRODUCTION
Clostridium difficile has long been recognized as the main cause of infectious diarrhoea occurring in hospitalized patients after prolonged antibiotic treatment (Rupnik, Wilcox and Gerding 2009; Bartlett 2010).
Metronidazole and vancomycin are the classical two recommended drugs for the treatment of C. difficile infection (CDI) (Cohen et al. 2010; Debast, Bauer and Kuijper 2014). In particular, metronidazole is widely used as first-line antibiotic in the treatment of mild-to-moderate CDI (Cohen et al. 2010; Debast, Bauer and Kuijper 2014). Higher treatment failure rates have been recently reported when metronidazole is used for CDI therapy (Vardakas et al. 2012). This clinical finding is even more alarming when CDI is associated to strains with in vitro reduced susceptibility to metronidazole (Musher et al. 2005; Jung et al. 2010; Venugopal et al. 2012), The European Committee on Antimicrobial Susceptibility Testing (EUCAST 2015) epidemiological cut-off (ECOFF) value of >2 μg mL−1 distinguishes wild-type C. difficile isolates from those having a mechanism of resistance to this antibiotic (http://mic.eucast.org/Eucast2/regShow.jsp?Id=21294). Higher MIC values to metronidazole have been observed in many strains belonging to the hypervirulent PCR ribotype 027, known as cause of outbreaks and severe infection worldwide (He et al.2013; Adler et al.2015). Clostridium difficile strains PCR ribotype 027 are characterized by an increased production of spores and both toxin A and B (Warny et al.2005; Akerlund et al.2008). Several studies have also reported a rising incidence with increased metronidazole MICs also in other epidemic C. difficile strains (Peláez et al. 2002; Bishara et al. 2006; Baines et al. 2008; Kuijper and Wilcox 2008).
The metronidazole resistance in C. difficile is due to a multifactorial mechanism not yet fully understood. However, recent studies have suggested the possible involvement of alterations in enzymes/metabolic pathways, particularly those implicated in the electron transport chain (Chong et al. 2014; Moura et al. 2014). In addition, the ability of some clinical and reference strains of C. difficile to form biofilm in vitro has been also recently demonstrated (Dawson et al. 2012; Donelli et al. 2012; Ðapa et al. 2013; Hammond, Donkor and Brown 2014; Semenyuk et al. 2014). In particular, data obtained on a C. difficile strain belonging to the PCR ribotype 027 showed that biofilm-growing cells are more resistant to high concentrations of vancomycin than planktonic cells (Ðapa et al. 2013). Similarly, cells of toxigenic C. difficile strains BI17 (PCR ribotype 027) and J9 grown as biofilm showed tolerance to metronidazole concentrations as high as 100 μg mL−1, while the same strains in liquid culture were inhibited by antibiotic concentrations above 1 μg mL−1, suggesting a 100-fold resistance increase to this drug in the form of biofilm (Semenyuk et al. 2014). This kind of improved resistance to antibiotics has already been described in other biofilm-growing Gram-positive pathogens (Donlan and Costerton 2002; Olson et al. 2011; Pantaléon et al. 2014).
Furthermore, several studies have been reported that subinhibitory concentrations of antibiotics may be a stress factor able to induce biofilm formation in different bacterial species (Hoffman et al. 2005; Cargill and Upton 2009; Bedran et al. 2014; Wu et al. 2014).
Strains belonging to PCR ribotype 010, even if non-toxigenic, show a mean geometric MIC to metronidazole higher compared to other PCR ribotypes (Moura et al. 2013) and several of these strains with reduced susceptibility to metronidazole in both humans and animals, particularly in dogs, have been described (Spigaglia et al. 2015).
In this study, we report on the ability to produce biofilm, in presence and absence of different subinhibitory concentrations of metronidazole, of three C. difficile clinical strains, belonging to PCR ribotype 010 and showing different antibiotic susceptibility profiles.
MATERIALS AND METHODS
Bacterial strains, typing and antibiotic susceptibility
The three C. difficile isolates 7032994, 7032985 and 7032989 used in this study, kindly provided by E. Bouza (University General Hospital ‘Gregorio Marañón’, Spain), have been previously demonstrated to be non-toxigenic and belonging to the PCR ribotype 010 by agarose gel-based PCR ribotyping method (Moura et al. 2013). All strains are available in the Istituto Superiore di Sanità collection.
The MIC values for metronidazole evaluated by agar dilution method (CLSI 2007) were 2 μg mL−1 for 7032994, 4 μg mL−1 for 7032985 and 32 μg mL−1 for 7032989 (Moura et al. 2013). Reduced susceptibility to metronidazole was defined as MIC > 2 μg mL−1, according to the European Committee on Antimicrobial Susceptibility Testing (EUCAST 2015) ECOFF. As positive control for quantitative biofilm formation assay, the biofilm producer C. difficile strain CdiBs21 was used (Donelli et al. 2012).
Culture conditions
Strains were cultured on brucella agar (Oxoid, UK) plates and maintained in an anaerobic cabinet (Don Whitley Scientific, UK) at 35°C. Few colonies of each strain were inoculated in 10 mL of pre-reduced brain heart infusion (BHI) broth (Oxoid, UK) and incubated for 24 h. Optical density at 600 nm (OD600) of the overnight cultures was measured with a spectrophotometer Ultrospec 2000 (Pharmacia Biotech) and adjusted to OD600 = 0.1. A 10-fold dilution of the adjusted cultures was performed in BHI supplemented with 0.5% yeast extract and 0.1% L-cysteine (BHIs), containing 1% glucose. Cultures were grown in absence and in presence of metronidazole (Sigma-Aldrich) at concentrations corresponding to 1/4 and 1/2 of the MIC value for each strain, namely, 0.5 and 1 μg mL−1 for 7032994, 1 and 2 μg mL−1 for 7032985, 8 and 16 μg mL−1 for 7032989. Approximately 200 μL of each dilution was distributed in triplicate in a 96-well flat bottom polystyrene plate, whereas 2 mL of the same dilutions was inoculated in 24-well polystyrene plates with 10-mm diameter glass slides placed on the bottom of the well. Equal volume of BHIs containing 1% glucose broth was distributed in three wells of the polystyrene plates, as negative control. The plates were incubated anaerobically and biofilm production was assessed after 48 h.
Quantitative biofilm production assay
Quantitative measurement of adherent cells was performed as previously described (Donelli et al. 2012). Briefly, after 48 h incubation, the supernatant was removed and each well of the 96-well plate was washed twice with sterile phosphate-buffered saline (PBS). Following incubation at 60°C for 1 h, dried wells were stained for 15 min with 180 μL of 2% Hucker's crystal violet. Excess stain was washed with distilled water and the 96-well plate was dried at 60°C for 10 min. The assay was performed three times in triplicate.
According to a modified quantification test (Stepanovic et al. 2000), strains were classified into different levels of adhesiveness on the basis of the measured optical density at 570 nm (OD570). The cut-off OD570 (ODC) was defined as three standard deviations above the mean OD of the negative control and the strains were classified as non-adherent (OD570 ≤ ODc), weakly adherent (ODc < OD570 ≤ 2 × ODc), moderately adherent (2ODc < OD570 ≤ 4 × ODc) and strongly adherent (OD570 > 4 × ODc).
The supernatant content of the 24-well plate was removed after 48 h incubation and each well with round glass slides placed on the bottom was washed twice with PBS. The biofilms grown on the top of the glass slides were observed by field emission scanning electron microscopy (FESEM) and confocal laser scanning microscopy (CLSM) as described below.
Field emission scanning electron microscopy
Clostridium difficile biofilms, grown on the round glass slides, were fixed with 2.5% glutaraldehyde in sodium cacodylate 0.1 M buffer (pH 7.4) at room temperature. After 30 min, the glutaraldehyde solution was removed and the sample washed three times with the same buffer and postfixed with OsO4 solution (1%) for 20 min at room temperature in the dark. The removal of the OsO4 solution was then followed by washing samples three times for 2–3 min with sodium cacodylate 0.1 M buffer and subsequent dehydration process through graded ethanol (30%, 50%, 70%, 85%, 95% and 100%), 10 min each step and three repetitions with 100% ethanol. Alcohol dehydration was followed by critical point drying and gold coating by sputtering. Biofilm samples were examined by a field emission scanning electron microscope (Sigma-Zeiss) at an accelerating voltage of 5 kV.
Confocal laser scanning microscopy
Biofilms were fixed with 3.7% paraformaldehyde at room temperature for 30 min. After washing with PBS, the C. difficile biofilms were visualized by fluorescent staining with 8 μM propidium iodide (Invitrogen) at room temperature for 10 min, in order to detect in red all bacterial cells. After being washed in distillate water, the samples were incubated with 50 μg mL–1 of concanavalin A-conjugated fluorescein isothiocyanate (Con A-FITC) (Sigma-Aldrich) for 5 min at room temperature to stain the glycocalyx matrix in green. The stain was aspirated, and the coverslips were gently washed twice with distilled water. All these procedures were performed in the dark.
The excitation/emission maxima for propidium iodide and Con A-FITC was 490/635 and 495/525 nm, respectively. Biofilms were examined by using a CLSM (Nikon C1si) with 20× lens and the images were acquired at a resolution of 512 × 512 pixels.
Statistical analyses
Three independent assays of biofilm production were performed. Two-tailed, Mann Whitney test with GraphPad Prism software (GraphPad Software Inc., La Jolla, CA, USA) was used to determine whether the differences observed in the growth of each strain in the presence or absence of antibiotic were significant. Differences were considered statistically significant for P values < 0.05.
RESULTS
Clostridium difficile adhesiveness in presence and absence of metronidazole
Based on the obtained OD570 values, the C. difficile strains 7032994, 7032985, 7032989 and CdiBs21 were defined as non-adherent for OD570 ≤ 0.235, weekly adherent for 0.235 < OD570 ≤ 0.471, moderately adherent for 0.471 < OD570 ≤ 0.941 and strongly adherent for OD570 > 0.941.
In absence of metronidazole, strains 7032994 and 7032985 were classified as moderately adherent (OD570 values 0.673 ± 0.16 and 0.742 ± 0.10, respectively) while strain 7032989 was considered as strongly adherent, although with an OD570 = 0.967 ± 0.26, close to the 4× ODc (0.941) (Fig. 1).
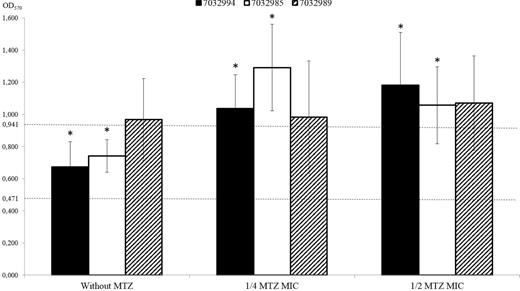
The effect of metronidazole (MTZ) in C. difficile strains adhesiveness. OD570 values of 7032994, 7032985 and 7032989 C. difficile strains (black, white and diagonally striped bars, respectively) growth without metronidazole or in presence of a concentration corresponding to 1/4 or 1/2 of the metronidazole MIC value for each strain. The values are means ± s.d. of three independent experiments. An asterisk (*) indicates a statistically significant difference in OD570 value when the same strain was grown in presence or absence of metronidazole (P-value < 0.05, two-tailed, Mann Whitney test). Dotted lines indicate the cut-off OD570 (ODC) values. In vitro levels of adherence were defined as moderately adherent for 0.471 < OD570 ≤ 0.941 and strongly adherent for OD570 > 0.941.
On the basis of the OD570 values, the susceptible strain 7032994 and the strain 7032985 with reduced susceptibility to metronidazole were classified as moderately adherent in the absence of metronidazole. On the contrary, a statistically significant variation in OD570 values (P value comprised between 0.00013 and 0.011) was observed for both strains when grown in the presence of subinhibitory concentrations of metronidazole, consequently classifying both 7032994 and 7032985 as strongly adherent.
The average OD570 value of the strain 7032994 slightly increased from 1.037 ± 0.21 to 1.183 ± 0.33 in presence of metronidazole at 1/4 and 1/2 of the MIC value, respectively. On the contrary, a non-significant reduction of the average OD570 value was observed for the strain 7032985 (from 1.057 ± 0.24 to 1.291 ± 0.27) when metronidazole concentration rose from 1/4 to 1/2 MIC.
Differently, the biofilm-forming ability of the metronidazole stable-resistant strain 7032989 was not further increased by the antibiotic pressure, without any statistically significant difference between the OD570 values observed in absence or in presence of metronidazole.
FESEM and CLSM analysis of C. difficile biofilms
In accordance with data obtained by the quantitative biofilm production assay, FESEM analysis revealed a significant increase in biomass under antibiotic pressure for the strains 7032994 and 7032985 (Fig. 2). Particularly, in presence of metronidazole at 1/2 of the MIC value (1 and 2 μg mL−1, respectively), both 7032994 and 7032985 strains showed an increased biofilm-forming ability, characterized by the production of a thick biofilm formed by layered aggregates (Fig. 2B and D, respectively), not observable in the stable-resistant strain (Fig. 2F).
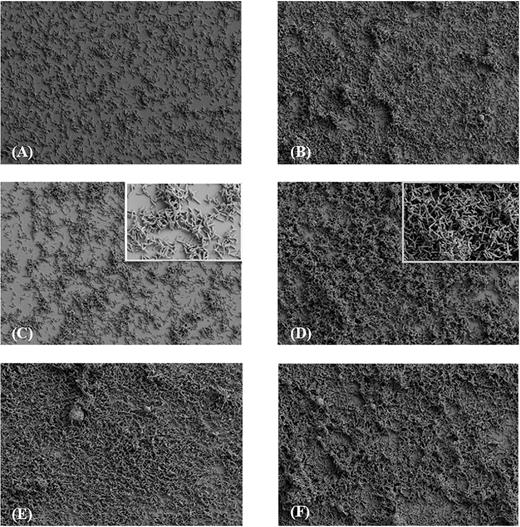
FESEM analysis of C. difficile strains biofilms. A metronidazole concentration of 1/2 of the MIC value for each strain was used. Biofilms were obtained at an accelerating voltage of 2 kV and a magnification of 1000×. (A) 7032994 strain, (B) 7032994 in presence of metronidazole; (C) 7032985 strain, including a 5000× magnification photo; (D) 7032985 in presence of metronidazole, including a 5000× magnification; (E) 7032989 strain; (F) 7032989 in presence of metronidazole.
Additional evidence on the enhanced biofilm development in presence of metronidazole was provided by the CLSM analysis. In fact, compared to the FESEM analysis, that requires a dehydration treatment strongly reducing the chance to observe the exopolysaccharide (EPS) matrix, CLSM imaging is able to provide a full visualization of the EPS matrix. Thus, it has been possible to observe a rich biofilm matrix in the susceptible strain 7032994 (Fig. 3B) and in the strain 7032885 with reduced susceptibility (Fig. 3D and E). The latter, as already shown by FESEM analysis, appears to be more responsive to the antibiotic pressure in terms of biofilm production. The green-staining Con A-FITC binds to the EPS residues of the matrix. The high predominance of green fluorescence in Fig. 3B and D compared to the red stain (bacterial cells) highlights the larger amount of EPS produced by both 7032994 and 7032885 strains in presence of the antibiotic. On the contrary, the resistant strain 7032989 did not show any increase in the matrix production (data not shown).
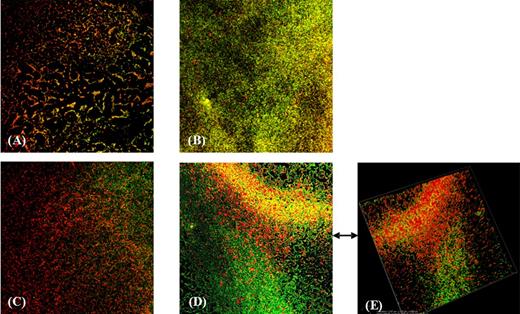
CLSM analysis of biofilms formed by C. difficile strains in presence and absence of metronidazole. The red-fluorescent propidium iodide stain labels bacteria, while the lectin Concanavalin A binds to residues of the exopolysaccharides matrix. The concentration of metronidazole used was 1/2 of the MIC value for each strain. (A) 7032994 strain, (B) 7032994 in presence of metronidazole, (C) 7032985 strain, (D) 7032985 in presence of metronidazole and (E) three-dimensional image of 7032985 biofilm in presence of metronidazole.
DISCUSSION
Biofilm formation allows many pathogens to survive in presence of limiting environmental conditions or damaging agents. Most of the hospital-acquired infections are biofilm based and, for this reason, are difficult to treat (Archibald and Gaynes 1997; Costerton 2001; Donlan and Costerton 2002; Ha, Chung and Ryoo 2005). In fact, sessile bacterial communities can survive to antibiotic concentrations 1000-fold higher if compared to those able to kill the respective planktonic cells (Gilbert, Das and Foley 1997). An association between biofilm production and bacterial persistence in the presence of antibiotics has already been observed for several pathogens (Anderl, Franklin and Stewart 2000; Mah and O'Toole 2001; Donlan and Costerton 2002; Olson et al. 2011; Pantaléon et al. 2014). Furthermore, antibiotic pressure has been demonstrated to enhance biofilm formation in different bacterial species (Hoffman et al. 2005; Cargill and Upton 2009; Bedran et al. 2014; Wu et al. 2014).
The increased antibiotic resistance reported for C. difficile clinical isolates and the recurrences of CDI are challenges in the disease management. It has been estimated that approximately 22% and 14% of CDI treatment failures, as well as 27% and 24% of recurrences, are associated to treatment with metronidazole or vancomycin, respectively (Vardakas et al. 2012).
Recent studies on C. difficile toxigenic strains have demonstrated that the sessile phase of investigated strains is more resistant to these antibiotics than the planktonic one (Ðapa et al. 2013; Semenyuk et al. 2014), thus contributing to the persistence of C. difficile in the intestinal tract and enhancing the occurrence of recurrent infections (Semenyuk et al. 2014). Although investigations on human bioptic samples have not yet been performed to directly detect the presence of biofilm-growing C. difficile, studies carried out on animal models have reported the presence of C. difficile cell aggregates associated with intestinal mucus (Buckley et al.2011; Semenyuk et al.2015). Biofilm-growing C. difficile cells show specific features that may facilitate infection, such as abundant spores and major toxins A and/or B production (Dawson et al.2012; Crowther et al.2014; Semenyuk et al.2014).
In the present study, we have shown that (i) the three investigated C. difficile strains, belonging to the PCR ribotype 010 and showing different metronidazole susceptibility profiles, are able to form biofilm in vitro; (ii) subinhibitory concentrations of metronidazole enhance biofilm formation in two of the three strains.
Particularly, the quantitative biofilm production assay performed in presence of metronidazole has revealed a significant increase in biofilm formation in the susceptible strain 7032994 and in the strain 7032985 with reduced antibiotic susceptibility. On the contrary, the ability of the stable-resistant strain 7032989 to produce biofilm did not change when the antibiotic was in the medium.
A recent proteomic analysis on the strains used in this study has demonstrated that C. difficile 7032989, with high level of resistance to metronidazole, has an altered pyruvate-ferredoxin oxidoreductase (PFO) production and a less efficient electron transfer required for metronidazole activation (Moura et al. 2014). These peculiar characteristics could in part explain the behaviour of strain 7032989 in presence of subinhibitory concentrations of metronidazole. In fact, it is known that metronidazole is activated by reduction and it has been demonstrated that electrons required for this activation are released from pyruvate by the activity of PFO and transferred to the drug by the low-redox-potential carrier ferredoxin (Land and Johnson 1997).
On the other hand, even if the increase of biofilm formation in both 7032994 and 7032985 strains is presumably due to a global cellular response to stress and not to a single mechanism, CLSM images seem to suggest that the subinhibitory concentration of metronidazole could influence specific genes involved in matrix production, inducing their overexpression.
Interestingly, it has been recently demonstrated that in vitro biofilm formation in a C. difficile strain PCR ribotype 027 is stimulated by subinhibitory concentrations of vancomycin (Ðapa et al. 2013), suggesting that both the antibiotics of choice for CDI treatment could be able to enhance in vivo biofilm production.
Additionally, a genetic heterology in C. difficile strains, causing both hospital- and community-acquired infections, has been demonstrated, pointing out also the environment (water, soil), food and animals as possible sources Freeman et al. 2010; Dubberke et al. 2011; Hensgens et al. 2012; Hoover and Rodriguez-Palacios 2013). Furthermore, recent studies have reported a genetic correlation between the strains isolated from humans and those collected from animals or environment (Bakker et al. 2010; Keessen, Gaastra and Lipman 2011; Janezic et al. 2012, 2014). In this regard, it is interesting to note that C. difficile strains PCR ribotype 010, either resistant or with reduced susceptibility to metronidazole, have been recently detected in dogs (Spigaglia et al. 2015), suggesting a potential role of these pets as reservoir of C. difficile strains. In fact, even if PCR ribotype 010 is non-toxigenic, the possibility that strains in vivo acquire the PaLoc cannot be excluded, especially because PaLoc has been recently transferred in vitro to non-toxigenic isolates by genetic recombination (Brouwer et al. 2013).
As the concentrations of this antibiotic in watery stools following oral therapy range between 0.8 and 24.2 mg g−1 (Bolton and Culshaw 1986), it is possible that the low concentrations of antibiotic present in the gut in some phases of the CDI treatment (particularly at the beginning and at the end) could stimulate C. difficile to grow as biofilm. Given the persistence of biofilm-growing bacterial cells in the human intestine and the extended protection provided by the biofilm phenotype during infections (Macfarlane and Dillon 2007), the production of an increased, dense biofilm could have an important clinical relevance in the treatment failure and recurrence of infections associated with C. difficile strains displaying susceptibility or reduced susceptibility to metronidazole in vitro.
According to the results of this study, subinhibitory concentrations of metronidazole seem to play a pivotal role in the enhancement of biofilm production by C. difficile strains PCR ribotype 010, either susceptible or with reduced susceptibility to this antibiotic. The protective barrier provided by biofilm could partially explain the treatment failures of CDI caused by strains with an in vitro susceptible phenotype, as already reported (Musher et al. 2005; Jung et al. 2010; Venugopal et al. 2012). Further investigations will be needed to shine more light on the different aspects of this complex mechanism of resistance.
FUNDING
This work was partially supported by the European Community's Seventh Framework Programme FP7/2007-2013 [grant agreement No. 237942].
Conflict of interest. None declared.
REFERENCES