-
PDF
- Split View
-
Views
-
Cite
Cite
Mette Kolpen, Cecilie F. Appeldorff, Sarah Brandt, Nabi Mousavi, Kasper N. Kragh, Sevtap Aydogan, Haleema A. Uppal, Thomas Bjarnsholt, Oana Ciofu, Niels Høiby, Peter Ø. Jensen, Increased bactericidal activity of colistin on Pseudomonas aeruginosa biofilms in anaerobic conditions, Pathogens and Disease, Volume 74, Issue 1, February 2016, ftv086, https://doi.org/10.1093/femspd/ftv086
- Share Icon Share
Tolerance towards antibiotics of Pseudomonas aeruginosa biofilms is recognized as a major cause of therapeutic failure of chronic lung infection in cystic fibrosis (CF) patients. This lung infection is characterized by antibiotic-tolerant biofilms in mucus with zones of O2 depletion mainly due to polymorphonuclear leukocytic activity. In contrast to the main types of bactericidal antibiotics, it has not been possible to establish an association between the bactericidal effects of colistin and the production of detectable levels of OH ˙ on several strains of planktonic P. aeruginosa. Therefore, we propose that production of OH ˙ may not contribute significantly to the bactericidal activity of colistin on P. aeruginosa biofilm. Thus, we investigated the effect of colistin treatment on biofilm of wild-type PAO1, a catalase-deficient mutant (ΔkatA) and a colistin-resistant CF isolate cultured in microtiter plates in normoxic- or anoxic atmosphere with 1 mM nitrate. The killing of bacteria during colistin treatment was measured by CFU counts, and the OH⋅ formation was measured by 3′-(p-hydroxylphenyl fluorescein) fluorescein (HPF) fluorescence. Validation of the assay was done by hydrogen peroxide treatment. OH⋅ formation was undetectable in aerobic PAO1 biofilms during 3 h of colistin treatment. Interestingly, we demonstrate increased susceptibility of P. aeruginosa biofilms towards colistin during anaerobic conditions. In fact, the maximum enhancement of killing by anaerobic conditions exceeded 2 logs using 4 mg L−1 of colistin compared to killing at aerobic conditions.
INTRODUCTION
Pseudomonas aeruginosa is the major Gram-negative bacterium causing chronic lung infections in cystic fibrosis (CF) patients (Høiby and Koch 2000; Høiby, Ciofu and Bjarnsholt 2010; Høiby et al.2010). The pathogen exists in biofilm aggregates surrounded by polymorponuclear leukocytes (PMNs) in the endobronchial mucus (Baltimore, Christie and Smith 1989; Bjarnsholt et al.2009) that contains anoxic zones (Worlitzsch et al.2002). This depletion of molecular oxygen (O2) is mainly caused by the activity of the accumulated PMNs for the production of superoxide (O2−) (Kolpen et al.2010), nitric oxide (NO) (Kolpen et al.2014a) and by respiration of the lung epithelium (Worlitzsch et al.2002). Even though O2 consumption by bacterial aerobic respiration is diminutive (Kolpen et al.2010), P. aeruginosa is able to proliferate and persist within the endobronchial mucus and sputum from CF patients (Yang et al.2008; Kragh et al.2014). In order to obtain energy in the O2 restricted endobronchial mucus, P. aeruginosa employs anaerobic respiration by denitrification (Kolpen et al.2014b), which enables anoxic growth of P. aeruginosa at physiological levels of nitrate (NO3−) (Hassett et al.2002; Kolpen et al.2014b; Line et al.2014).
The dramatically increased tolerance of P. aeruginosa during the biofilm mode of growth is considered a major obstacle for eradication by antibiotic treatment in CF patients (Høiby et al.2010). The high tolerance may result from reduced antibiotic penetration, slow growth and expression of specific resistance mechanisms (Høiby et al.2010). However, the actual influences of O2 are far from fully understood. Several major classes of bactericidal antibiotics induce lethal cellular damage by inducing redox-related physiological modifications by target-specific interactions resulting in formation of reactive oxygen species (ROS) (Kohanski et al.2007; Van Acker et al.2013; Dwyer et al.2014). This model proposes interaction between drugs and targets to stimulate superoxide formation that further damages the iron––sulphur cluster releasing ferrous iron for the Fenton reaction resulting in OH⋅ formation during aerobic respiration (Kohanski et al.2007; Dwyer et al.2014). However, the involvement of ROS in antibiotic-mediated killing has recently been questioned (Keren et al.2013; Liu and Imlay 2013), we have shown that the formation of hydroxyl radicals (OH⋅) in planktonic and in biofilm-grown P. aeruginosa contributes to the mechanism of bactericidal killing during ciprofloxacin treatment (Brochmann et al.2014; Jensen et al.2014). In particular, in planktonic cultures the bactericidal activity of ciprofloxacin decreases by removal of available O2 and by reduction of the metabolic activity in aerobic cultures (Brochmann et al.2014; Jensen et al.2014). Accordingly, in biofilm, where the availability of O2 stratifies the metabolism with increased aerobic metabolism in the periphery facing high O2 availability (Stewart 2003), the peripheral subpopulations harbour metabolically active P. aeruginosa with high susceptibility to ciprofloxacin. In contrast, the inner biofilm subpopulations with low metabolic activity are tolerant to ciprofloxacin (Pamp et al.2008). It is therefore suggested that the bacterial metabolic condition and the availability of O2 determines in part the killing contributed by OH ˙ generated during treatment with bactericidal antibiotics (Kohanski et al.2007; Brochmann et al.2014; Dwyer et al.2014; Jensen et al.2014). Accordingly, exclusion of O2 prevents formation of O2− by the one-electron reduction resulting from hyper-oxidation of NADH, which ultimately eliminates formation of bactericidal amounts of OH⋅ resulting in increased tolerance during anaerobic growth to several antibiotics used in clinical settings (Brochmann et al.2014; Dwyer et al.2014).
In contrast to ciprofloxacin, we have demonstrated that the bactericidal activity of colistin on planktonic P. aeruginosa is independent of OH ˙ formation (Brochmann et al.2014). In fact, the bactericidal activity of colistin on planktonic P. aeruginosa is reinforced by anaerobic growth and by reduction of the metabolism in aerobic conditions (Brochmann et al.2014). In addition, colistin targets the inner subpopulation of P. aeruginosa biofilms with low metabolism presumably caused by low O2 tension (Pamp et al.2008), and the effect of colistin on P. aeruginosa biofilms grown under anaerobic conditions has been suggested to lead to decreased susceptibility compared to aerobic growth (Hill et al.2005). This has, however, not yet been associated with the absent contribution of OH ˙ formation to the bactericidal activity of colistin on P. aeruginosa biofilm.
The main purpose of the present study is to investigate the significance of anaerobic conditions resembling zones in endobronchial secretions from CF patients on the activity of colistin on P. aeruginosa biofilms. We have tested the hypotheses: (a) that bacterial killing during colistin treatment is increased during O2 depletion and (b) that the bactericidal effect of colistin is independent on OH ˙ formation. Therefore, we have constructed aerobic and anaerobic bacterial killing curves and estimated aerobic OH ˙ formation during colistin treatment of P. aeruginosa biofilm. By simulating in vivo conditions (Bjarnsholt et al.2009), we will be able to demonstrate the bactericidal activity of colistin in vitro.
MATERIALS AND METHODS
Patients
As defined by the ‘Danish Act on Research Ethics Review of Health Research Projects’ Section 2, the project did not constitute a health research project and was thus initiated without approval from The Committees on Health Research Ethics in the Capital Region of Denmark. The study was carried out with one bacterial isolate from a CF patient with chronic P. aeruginosa lung infection. Chronic infection with the isolate was defined as the presence of bacteria in the lower respiratory tract as detected in each monthly culture from sputum samples for >6 months, or for a shorter time in the presence of increased antibody response (≥2 precipitating antibodies, normal: 0–1) (Høiby 2000).
Bacterial strains, media and antibiotics
Wild-type P. aeruginosa strain PAO1 used for the experiments was obtained from the Pseudomonas Genetic Stock Centre (http://www.pseudomonas.med.ecu.edu; strain PAO0001). A catalase A negative PAO1 (ΔkatA) mutant (Hassett et al.1999). Three clinical P. aeruginosa strains isolated from CF patients with chronic lung infection were also tested for their response to colistin: 9B, a non-mucoid isolate; 9A, a mucoid isolate and one clinical non-mucoid colistin-resistant P. aeruginosa strain (CF 1021) (Miller et al.2011; Brochmann et al.2014). The MIC of colistin to 9A, 9B and CF 1021 was 0.125 mg L−1, 0.094 mg L−1 and 256 mg L−1, respectively, as measured by E-test. Strains were grown in Luria–Bertani (LB) broth [5 g L−1 yeast extract (Oxoid, Roskilde, Denmark), 10 g L−1 tryptone (Oxoid) and 10 g L−1 NaCl (Merck, Rahway, NJ), pH 7.5], incubated overnight at 37°C and shaken at 170 rpm.
The bactericidal antibiotic colistin sulphate salt (Sigma–Aldrich, Brøndby, Denmark) was used. For determination of bacterial colony forming units (CFU) counts, solid lactose agar plates [‘Blue plates’ a modified Conradi-Drigalski medium containing 10 g L−1 detergent, 1 g L−1 Na2S2O3·H2O, 0.1 g L−1 bromothymol blue, 9 g L−1 lactose, 0.4 g L−1 glucose and pH 8.0. All plates were incubated overnight at 37°C.
Susceptibility testing of young and mature biofilm
Survival curves and OH· formation were assayed to investigate the effect of O2 on P. aeruginosa treated with colistin or hydrogen peroxide (H2O2).
Two hundred microliter of overnight cultures of PAO1, ΔkatA and a colistin-resistant CF isolate of P. aeruginosa with an OD600 adjusted to 0.4 before 100-fold dilution in AB minimal media supplemented with trace metals and 0.5% (v/w) glucose (ABTG) (Clark 1967) to achieve ca. 106 cells mL−1 were added to each well of black microtiter plates with transparent flat bottom (16503, Thermo Fisher Scientific, Rochester, NY). To ensure metabolic activity during the study of the effect of oxygen, all media were supplemented with NaNO3 (1 mM) (Sigma) to allow anaerobic respiration. All oxygen-depleted cultures were prepared in an anaerobic bench using equilibrated media with a partial pressure of <0.02% O2 as determined with a multiparameter metre HQ40d (HACH Company, Loveland, CO). All microtiter plates were covered with parafilm and lid and incubated at 37°C for 1 (young biofilm) or 3 days (mature biofilm). The density of young untreated PAO1 biofilms was 5 × 107 CFU ml−1 and 2 × 107 CFU ml−1 in aerobic and anaerobic conditions, respectively. The density of mature untreated PAO1 biofilms was 6 × 106 CFU ml−1 and 2 × 108 CFU ml−1 in aerobic and anaerobic conditions, respectively. Furthermore, the density of young and mature untreated ΔkatA biofilms was 3 × 107 and 2 × 107 CFU ml−1 in aerobic conditions, respectively. The density of young and mature untreated CF1012 biofilms was 6 × 107 and 5 × 107 CFU ml−1 in aerobic conditions, respectively. Finally, the density of mature untreated 9A and 9B was 1 × 107 and 9 × 107 CFU ml−1 in aerobic conditions and during anaerobic conditions 8 × 105 and 5 × 106 CFU ml−1, respectively. Supernatant was replaced by 200 μl of a colistin or a H2O2 solution in ABTG [with and without 100 mM thiourea (Sigma Aldrich, Brøndby, Denmark)] in 2-fold dilutions from 0.25 to 64 mg L−1. Final concentrations in the wells of 5 μM 3′-(p-hydroxylphenyl fluorescein) fluorescein (HPF) (Molecular Probes, Eugene, OR) were added for detection of OH ˙ (Kohanski et al.2007), and the plates were further incubated at 37°C for 60 and 180 min. After incubation, the fluorescence from the OH ˙ indicator was recorded in a plate reader (Wallac 1420, Victor X3, Perkin Elmer, MA). The excitation/emission wavelengths were 485/535 for HPF.
Estimation of the bacterial survival was done by counting CFU after sonication of the biofilms according to (Hengzhuang et al.2011). CFU mL−1 was estimated by plating of serial dilutions. In this assay, the lower level of detection for bacterial counts was 1 colony/plate, corresponding to 10 CFU ml−1. Consequently, all samples with ≤1 colony/plate were recorded as 10 CFU ml−1 (Brochmann et al.2014).
Microsensor measurement of pH
The pH was measured in both the aerobic and anaerobic treated PAO1 samples after treatment with 2-fold dilutions of colistin from 0.25 to 64 mg L−1 in ABTG supplemented with 1 mM NO3− with a pH microelectrode (pH-25, Unisense A/S, Århus, DK) and a reference electrode, which are used to establish a reference potential against the pH microelectrode, (ref-100, Unisense A/S, Århus, DK) mounted in a motorized PC-controlled profiling setup (MM33 and MC-232, Unisense A/S). Positioning and data acquisition were controlled by dedicated software (Sensortrace Pro 2.0, Unisense A/S). The pH- and reference electrode was calibrated with buffers of pH 4 and 7 at the experimental temperature of 37°C (Thomas 1979). The electrode has a detection limit of 0.1 pH unit.
Statistical methods
Statistical significance was evaluated by ordinary one-way ANOVA analysis using Dunnet's multiple comparison test and by two-way ANOVA analysis followed by Bonferroni's multiple comparison test. A P value ≤ 0.05 was considered statistically significant. The tests were performed with Prism 6.1 (GraphPad software, La Jolla, California, USA) and EXCEL (Microsoft Office 2007, Redmond, WA).
RESULTS
Anaerobic conditions increase the susceptibility of P. aeruginosa biofilms to colistin
Bactericidal activity of colistin treatment on both aerobically and anaerobically grown P. aeruginosa biofilms was indicated by the decreased fraction of surviving cells with increasing concentrations of colistin. Significantly fewer bacteria survived during colistin treatment of mature (3 days old) PAO1 biofilms during anaerobic conditions compared to aerobic conditions (Fig. 1). In fact, the maximum decrease of survival by anaerobic conditions required half the concentration of colistin compared to the survival at aerobic conditions. However, the fraction of surviving cells was not affected by the presence of O2 during colistin treatment in the one-day young biofilms (Fig. 1). To ensure that these results are not only applicable to PAO1, we have compared the effect of colistin on aerobically and anaerobically grown biofilms of P. aeruginosa sputum isolates from chronically infected CF patients. The activity of colistin against both susceptible CF isolates was increased by anaerobic conditions as indicated by the 2- to 8-fold increase of colistin needed to decrease the aerobically bacterial survival significantly (Fig 2). Furthermore, we can exclude that the bactericidal activity of colistin was due to changes in pH since the pH of the buffered medium was not affected by the treatment as shown in Fig. S1 (Supporting Information).
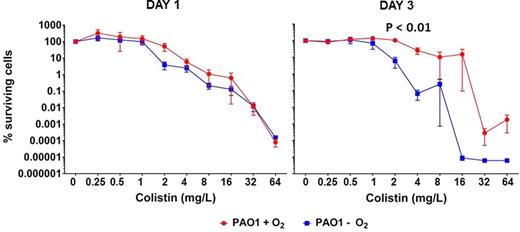
Percentages surviving cells demonstrating the effect of oxygen on colistin (0.25–64 mg L−1) treatment of P. aeruginosa biofilms. Effect of aerobic (red curve) and anaerobic (blue curve) conditions on surviving cells of 1- (left graph) and 3-day-old PAO1 (right graph) biofilms. Mean ± SEM of 4–6 individual experimental set-ups with quadruplicates are shown. Statistical significance was determined using two-way ANOVA followed by Bonferroni's multiple comparison tests.
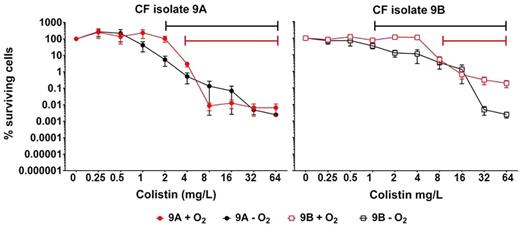
Percentages surviving cells demonstrating the effect of oxygen on colistin (0.25–64 mg L−1) treatment of clinical sputum P. aeruginosa isolates biofilms. Effect of aerobic (red curves) and anaerobic (black curves) conditions on surviving 3-day-old 9A (a mucoid CF isolate) (filled circles) and 9B (a non-mucoid CF isolate) (open square) biofilms. Intervals of significant changes by colistin treatment are indicated by the solid lines. Mean ± SEM of 4–7 individual experimental set-ups with quadruplicates are shown. Statistical significance was determined using one-way ANOVA followed by Dunnett's multiple comparison tests.
To assay whether young and mature biofilm cells were killed by colistin through the mechanism of ROS in aerobically grown biofilms, ΔkatA mutant biofilms, and the effect of addition of thiourea (a ROS inhibitor) to PAO1 biofilms were investigated. Colistin induced killing could not be prevented by scavenging of OH ˙ with thiourea on young and mature PAO1 biofilms. Furthermore, the young and mature ΔkatA mutant biofilms had not increased susceptibility to colistin (Fig. 3), indicating that antioxidant defence does not protect against colistin. Even at high concentrations of colistin the killing was impaired in the colistin-resistant mutant (Fig. 3). In addition, increased concentrations of colistin in aerobically growing biofilms did not induce a significant increase in OH ˙ formation by HPF fluorescence during 180 min of incubation, and HPF fluorescence was not higher in the kata mutant as during ciprofloxacin treatment (Jensen et al.2014) (Fig. S2, Supporting Information). HPF was used because it is a more specific indicator for OH ˙ radicals who in part mediate the bactericidal effect of several antibiotics (Kohanski et al.2007). That is why DCHF-DA was omitted from the experiment as it detects a broader range of ROS including OH ˙, H2O2 and O2− (Setsukinai et al.2003).
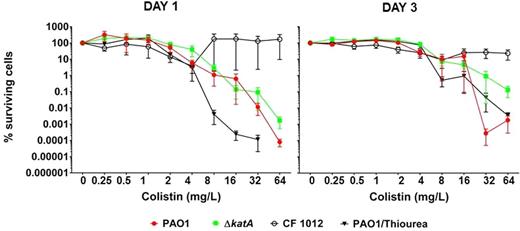
Percentages surviving cells demonstrating the effect of colistin (0.25–64 mg L−1) treatment of aerobic P. aeruginosa biofilms. Effect of colistin treatment on 1- and 3-day-old biofilms of PAO1 (red curve), ΔkatA (green curve), colistin-resistant CF isolate (black curve with open circle) and PAO1 with scavenging of OH ˙ by thiourea (black curve with triangles). Mean ± SEM of 4–6 individual experimental set-ups with quadruplicates are shown. Statistical significance was determined using one-way ANOVA followed by Dunnett's multiple comparison tests.
Specificity of OH• formation
Measurement of HPF fluorescence to detect OH ˙ formation and the following OH ˙ mediated cytotoxicity was confirmed by controlling the effect of H2O2 treatment, which leads to intracellular formation of OH ˙ (Imlay, Chin and Linn 1988). Treatment of aerobically growing ΔkatA biofilms with increasing H2O2 concentrations demonstrated an increased HPF fluorescence (Fig. S3, Supporting Information) and concomitantly increased bacterial killing (Fig. S4, Supporting Information). As expected, removal of OH ˙ by scavenging with thiourea prevented HPF fluorescence and rescued ΔkatA biofilms (Fig. S3, Supporting Information). Therefore, our assay is able to detect formation of cytotoxic levels of OH ˙ as increased HPF fluorescence. Treatment with H2O2 is in accordance with the validation of the specificity of HPF fluorescence upon H2O2 treatment as previously demonstrated (Brochmann et al.2014), and the need for high concentrations of H2O2 is in accordance with the tolerance of mature PAO1 biofilm against H2O2 (Hassett et al.1999). Though thiourea rescued ΔkatA and reduced HPF fluorescence during H2O2 treatment additional effects of thiourea is suggested by the inexplicably uniform effect of thiourea in lowering HPF fluorescence at all concentrations of H2O2, including the untreated well. This underlines that thiourea may have other effects than scavenging of OH. In order to control the role of autofluorescence, we subtracted the background fluorescence of unstained biofilms from the fluorescence of HPF stained biofilms. In our experiment, the background fluorescence represented less than 10% of the specific HPF fluorescence, and the variation of the background fluorescence was also less 10%. In addition, our method is sensitive enough to detect OH formation that contributes to the bacterial activity of ciprofloxacin (Jensen et al.2014).
DISCUSSION
The stratified physiological mode of growth by subpopulations in P. aeruginosa biofilm confers tolerance to several antibiotics commonly used by clinicians. This is in part caused by the metabolic changes resulting from the limited O2 availability and nutrient penetration through the biofilm due to bacterial consumption (Walters et al.2003; Pamp et al.2008). In endobronchial secretions from chronically infected CF mucus, however, the intense O2 depletion by the PMNs (Kolpen et al.2010) results in redistribution of the chemical microenvironment with the potential to stratify the physiological mode of growth by P. aeruginosa biofilms. In fact, the growth rate of P. aeruginosa in biofilm in infected CF lungs is determined by the number of surrounding PMNs rather than by the position of the bacteria in the biofilm (Kragh et al.2014), and anaerobic respiration is induced (Kolpen et al.2014b). Therefore, the lung mucus in chronically infected CF patients may be considered as stratified by the PMNs with zones of oxygen depletion harbouring entire biofilm growing without aerobic respiration, which emphasizes the relevance of the contribution of O2 to the activity of antibiotics against biofilm. In this regard, we have demonstrated that the bactericidal action of colistin on P. aeruginosa biofilm does not involve formation of OH ˙ resulting from reduction of O2 during aerobic respiration. This is opposed to the contribution of OH ˙ formation to the bactericidal activity of ciprofloxacin on P. aeruginosa biofilm (Jensen et al.2014) and to the bactericidal activity of major types of antibiotics in biofilms and planktonic cultures of several species (Kohanski et al.2007; Van Acker et al.2013; Dwyer et al.2014). Furthermore, we found that the lack of O2 increases the bactericidal activity of colistin on mature biofilm, which is in contrast to antibiotics involving OH ˙ formation as a bactericidal agent, where O2 depletion attenuates the bactericidal activity (Brochmann et al.2014; Dwyer et al.2014). Thus, our results points to the absence of protection provided by anaerobic conditions of P. aeruginosa biofilm during colistin treatment since the bactericidal activity of colistin on P. aeruginosa does not involve formation of cytotoxic levels of OH ˙ during aerobic respiration. Preventing ROS formation by removing O2 did not protect biofilms against colistin as would have been expected if OH ˙ is involved in bacterial killing by colistin. In fact, anaerobic conditions increased the killing of P. aeruginosa biofilm by colistin. This finding is supported by biofilm subpopulations exhibiting low metabolic activity (Haagensen et al.2007; Pamp et al.2008).
Pseudomonas aeruginosa is able to grow and sustain under anoxic conditions by performing denitrification in the endobronchial mucus from CF patients (Kolpen et al.2014b; Line et al.2014). During anaerobic respiration by denitrification, where the citric acid cycle is lowered, less energy is preserved (Chen and Strous 2013) with lower growth rates to follow. Consequentially, bacteria may be dormant or slow growing. This low anaerobic metabolism is supported by physiological concentrations of nitrate (NO3−) (Line et al.2014). We therefore added 1 mM NO3− to sustain anaerobic growing biofilm cells. It has previously been demonstrated that the effect of colistin on planktonic P. aeruginosa is increased when grown under anaerobic conditions (Hill et al.2005). Here, we substantiate that addition of 1 mM NO3− to sustain strictly anaerobic growing biofilm cells increases bactericidal activity of colistin, highlighting the importance of the type of electron acceptors employed by respiration supporting growth. In addition, more susceptibility to colistin under anaerobic conditions in clinical CF isolates has also been demonstrated (Zemke, Gladwin and Bomberger 2015). Interestingly, bacterial respiration can be inhibited by colistin due to cytoplasmic membrane disruption as demonstrated by oxygen consumption (LaPorte, Rosenthal and Storm 1977; Zemke, Gladwin and Bomberger 2015). Whether colistin also inhibits anaerobic respiration, however, remains to be documented. When colistin targets lipid A, which is located in the core of the lipopolysaccharide (LPS) within the outer membrane of Gram-negative bacteria, disruption of this membrane is induced (Wu et al.1999; Zhang et al.2000). This leads to increased bacterial killing by colistin on metabolic inactive bacteria located in the inner core of biofilms (Pamp et al.2008; Chiang et al.2012).
These results confirm that bactericidal antibiotics such as colistin that are independent on OH ˙-mediated killing have an increased bactericidal effect on anaerobically grown P. aeruginosa biofilms. The decreased tolerance of anaerobic mature biofilm against colistin has emphasized the importance of antimicrobial peptides as bactericidal drugs active during low O2 conditions. Our finding of increased bactericidal activity of colistin against anaerobic compared to aerobic biofilms is supported by recent findings of decreased MIC-values of colistin under ‘CF like’ conditions (Pompilio et al.2015). We suggest that the increased bactericidal activity of colistin during anaerobic growth of P. aeruginosa biofilms may result from a low ability to establish tolerance by modifying the LPS molecule (Miller et al.2011; Moskowitz et al.2012; Gutu et al.2013), due to low metabolism accompanying anaerobic growth.
In conclusion, our results show that the bactericidal activity of colistin on P. aeruginosa biofilms does not follow the common OH ˙-mediated killing (Kohanski et al.2007). This indicates that P. aeruginosa biofilms present in environments with O2 depletion is susceptible to antibacterial peptides as colistin targeting the subpopulations of biofilms with low metabolism (Pamp et al.2008). Therefore, these data support the use of colistin as a bactericidal drug, where low O2 availability is common in the endobronchial mucus (Worlitzsch et al.2002), CF sinuses (Aanaes et al.2011), abscesses (Hays and Mandell 1974) and other infectious biofilms (Xu et al.1998; Høiby et al.2010).
SUPPLEMENTARY DATA
MK has been supported by a UC-CARE (University of Copenhagen- Center for Antimicrobial Research) grant. KNK was supported by the Human Frontiers Science Program.
Conflict of interest. None declared.
REFERENCES