-
PDF
- Split View
-
Views
-
Cite
Cite
Christopher R. Gourley, Erik Petersen, Jerome Harms, Gary Splitter, Decreased in vivo virulence and altered gene expression by a Brucella melitensis light-sensing histidine kinase mutant, Pathogens and Disease, Volume 73, Issue 2, March 2015, Pages 1–8, https://doi.org/10.1111/2049-632X.12209
- Share Icon Share
Brucella species utilize diverse virulence factors. Previously, Brucella abortus light-sensing histidine kinase was identified as important for cellular infection. Here, we demonstrate that a Brucella melitensisLOV-HK (BM-LOV-HK) mutant strain has strikingly different gene expression than wild type. General stress response genes including the alternative sigma factor rpoE1 and its anti-anti-sigma factor phyR were downregulated, while flagellar, quorum sensing (QS), and type IV secretion system genes were upregulated in the ΔBM-LOV-HK strain vs. wild type. Contextually, expression results agree with other studies of transcriptional regulators involving ΔrpoE1, ΔphyR, ΔvjbR, and ΔblxR (ΔbabR) Brucella strains. Additionally, deletion of BM-LOV-HK decreases virulence in mice. During C57BL/6 mouse infection, the ΔBM-LOV-HK strain had 2 logs less CFUs in the spleen 3 days postinfection, but similar levels 6 days post infection compared to wild type. Infection of IRF-1−/− mice more specifically define ΔBM-LOV-HK strain attenuation with fewer bacteria in spleens and significantly increased survival of mutant vs. wild-type infected IRF-1−/− mice. Upregulation of flagella, QS, and VirB genes, along with downregulation of rpoE1 and related sigma factor, rpoH2 (BMEI0280) suggest that BM-LOV-HK modulates both QS and general stress response regulatory components to control Brucella gene expression on a global level.
INTRODUCTION
Brucella spp. are zoonotic pathogens responsible for the disease brucellosis in animals and humans. Brucellosis in animals can extract a high economic cost due to spontaneous abortions and the need to cull infected herds to prevent disease spread. Although brucellosis is rarely fatal, the global distribution, high economic cost of animal infections, and the length and complexity involved in resolving human infection make research on Brucella virulence mechanisms a priority.
Brucella spp. utilize few of the classic virulence factors employed by other Gram-negative pathogens. Being a facultative intracellular pathogen, brucellae strike a fascinating balance being highly infective, persisting in hosts, and establishing long-term infections. Brucellae exploit a replicative niche within host phagocytic cells. Previous work revealed that the Brucella LOV-HK protein is important to bacterial survival in an in vitro macrophage model of infection (Swartz et al., 2007). An insertional gene knockout of LOV-HK in B. abortus (BA-LOV-HK) resulted in fewer bacteria in macrophages similar to a level observed during infections conducted in the dark (Swartz et al., 2007). LOV-HK contains a light, oxygen, and voltage (LOV) domain that binds a flavin cofactor enabling the protein to sense blue light (Swartz et al., 2007; Rinaldi et al., 2012). Light activates the enzymatic activity of LOV-HK and is necessary for full virulence of the bacterium in macrophages (Swartz et al., 2007). These results provided a clear link between LOV-HK and light-induced virulence in an in vitro cellular infection assay. Whether LOV-HK exerts a similar role in virulence during an in vivo infection is unknown.
In this report, we show deletion of LOV-HK (BM-LOV-HK) from B. melitensis altered expression of the VirB operon, flagella, quorum sensing (QS), and general stress related genes known to be associated with Brucella virulence. We also demonstrate that BM-LOV-HK is necessary for full B. melitensis virulence in immune competent C57BL/6 and immune compromised IRF-1−/− mouse models of infection.
MATERIALS AND METHODS
Bacterial strains, vectors, and culture maintenance
Brucella melitensis strain 16M (ATCC23456) was utilized as the wild-type strain for experiments, as well as for gene deletion studies. Brucella was propagated and maintained in Brucella broth and on Brucella agar (BD). Escherichia coli DH5α was used as the maintenance and propagation strain for vectors. Escherichia coli was propagated and maintained in Luria-Bertani broth and on Luria-Bertani agar. A suicide vector using the p-ZErO-2 backbone containing both kanr and ampr was used to generate the gene deletion strain. Kanamycin was utilized in media at a final concentration of 50 μg mL−1. Ampicillin was used at 100 μg mL−1. See Supporting Information, Table S1 for a list of strains and vectors.
Generation of deletion strain
The ΔBM-LOV-HK (BMEII0679) was produced using homologous recombination as previously described (Rajashekara et al., 2006). Briefly, a suicide vector was constructed containing 1 kb immediately up and downstream of the gene of interest, and a kanamycin resistance cassette was inserted between these two flanking regions. Suicide vectors also contained an ampicillin resistant cassette for counter selection. Suicide vectors were transformed into B. melitensis by electroporation. Desired strains were isolated by resistance to kanamycin and sensitivity to ampicillin. Gene deletion was verified by PCR. See Table S2 for gene deletion primers. Separate growth curves using Brucella broth and minimal media plus glucose showed no significant difference in growth between the BM-LOV-HK mutant and wild type (data not shown). As with wild-type bacteria, colonies of the BM-LOV-HK mutant strain did not stain with crystal violet (data not shown).
Isolation of Brucella RNA for microarray and qRT-PCR
Brucella RNA was isolated from log phase cultures using an adaptation of the Epicentre Master Pure RNA kit/protocol with an additional DNase treatment using Applied Biosystems TurboDNase. Briefly, liquid cultures were grown in Brucella broth (BD) and treated with 1 part stop solution per 10 parts sample (stop solution was 0.5% phenol in ethanol). Treated samples were pelleted at 13 000 g and frozen at −80 °C. During lysis, samples were incubated at 65 °C for 20 min with vortexing every 5 min. RNA was eluted in nuclease free water. A second DNase digestion was conducted using the Applied Biosystems TurboDNase protocol for rigorous digestion. RNA for microarray analysis was assayed for quality and quantity by Thermo NanoDrop and Agilent 2100 Bioanalyzer.
Brucella cDNA production, labeling, microarray hybridization
All microarray analysis (three biological replicates per strain) was conducted according to Roche NimbleGen Arrays User's Guide for Gene Expression Analysis for 385K arrays. Briefly, cDNA was produced using the NimbleGen protocol and Invitrogen Superscript II. cDNA labeling was conducted using the Nimblegen One-Color DNA Labeling Kit and protocol. Array hybridization was conducted for c. 18 h at 42 °C on a Maui hybridization system. Washed and dried arrays were scanned on a GenePix 400B Scanner. Data were extracted and normalized from scanned array images using roche nimblescan Software. The Bioconductor ebarray package was used to determine significance (LNN > 0.5) (Newton et al., 2001; Kendziorski et al., 2003), where fold change calculation was ΔBM-LOV-HK/WT for positive fold change and −1/(ΔBM-LOV-HK/WT) for negative fold change.
Raw microarray data
Raw microarray data have been deposited with the Gene Expression Omnibus (GEO) (http://www.ncbi.nlm.nih.gov/geo/), series record GSE42599.
qRT-PCR of Brucella genes
RNA was isolated three times per bacterial strain as described above. First strand cDNA synthesis conducted with the High Capacity cDNA Reverse Transcription Kit from Applied Biosystems. A control reaction without reverse transcriptase was conducted for each biological replicate to ensure the absence of gDNA contamination. cDNA produced was used at a final dilution of 1 : 1000 per qRT-PCR. Final primer concentration was 400 nM per primer, per reaction. qRT-PCR was conducted for 40 cycles of 95 °C for 15 s, 60 °C for 30 s, and 72 °C for 30 s. Samples were subjected to 95 °C for 2.5 min before cycling began. Bio-Rad iQ™ SYBR® Green Supermix chemistry was used. Values were calculated by the delta-delta CT method and normalized to expression of the IF-1 (BMEI1671) gene (Eskra et al., 2001). qRT-PCR primers are identified in Table S3.
Measurement of spleen bacterial colony-forming units (CFUs) and mouse survival
C57BL/6 or IRF-1−/− mice (n = 4 per strain, per time point) were infected intraperitoneally with 1 × 106 CFUs and then housed until euthanized. Spleens were harvested and macerated with disposable tissue grinders (Fisher) in 3 mL of phosphate buffered saline (PBS). Liquid product was 10-fold serially diluted in PBS and replica plated to determine spleen CFUs. Plates were grown for three days before counting. Results were analyzed using the Mann-Whitney test performed with graphpad prism version 5.00 for Windows, GraphPad Software, San Diego, CA.
IRF-1−/− mice were infected [B. melitensis 16M (n = 11) and ΔBM-LOV-HK (n = 12)] intraperitoneally with 1 × 106 CFUs and then housed until they either succumbed to Brucella infection or were euthanized (Matsuyama et al., 1993; Kamijo et al., 1994; Taki et al., 1997; Salkowski et al., 2000; Ko et al., 2002, b; Rajashekara et al., 2005, b). Results were analyzed using the Mantel–Cox test performed with graphpad prism version 5.
All animal protocols and experiments were approved and conducted in accordance with the University of Wisconsin-Madison Research Animal Care and Use Committee.
RESULTS
Differences in global gene expression between the ΔBM-LOV-HK strain and B. melitensis 16M grown in broth culture
To identify the genes directly and indirectly regulated by BM-LOV-HK, a whole genome microarray expression analysis was conducted with three biological replicates comparing the ΔBM-LOV-HK mutant strain to B. melitensis 16M wild type. RNA was isolated from log phase broth cultures to access growing bacteria. Microarray results were verified using qRT-PCR to analyze separately isolated mRNA samples. Importantly, several virulence related groups of significantly upregulated genes including flagellar genes and the Brucella type IV secretion system (T4SS) VirB operon were identified. Additionally, vjbR and blxR (babR), two luxR homologues and probable Brucella QS regulators were also upregulated in the mutant strain compared to wild type. The Brucella general stress response system (GSR), regulated through the RpoE1 alternate sigma factor and the anti-anti-sigma factor PhyR, was among the groups of notably downregulated genes. These flagellar, type IV secretory system, QS, and general stress response genes are known contributors to Brucella virulence (Rambow-Larsen et al., 2008; Uzureau et al., 2010; Weeks et al., 2010). See Table 1 for a selection of notable differentially regulated genes. Of the 3198 arrayed genes, 243 (c. 7.6%) were significantly differently expressed in the mutant vs. wild-type strain. Figure 1 provides a graphical overview of important genes, while expression data for all significantly differentially expressed genes are presented in Tables S4 and S5 with fold changes and log normal-normal values (LNN > 0.5 significant). Besides COGs of poorly characterized and genes of unknown function, the largest group of differentially regulated genes were those involved in metabolism. Other categories of genes that were up or downregulated include information storage and processing, and cellular processes and signaling.
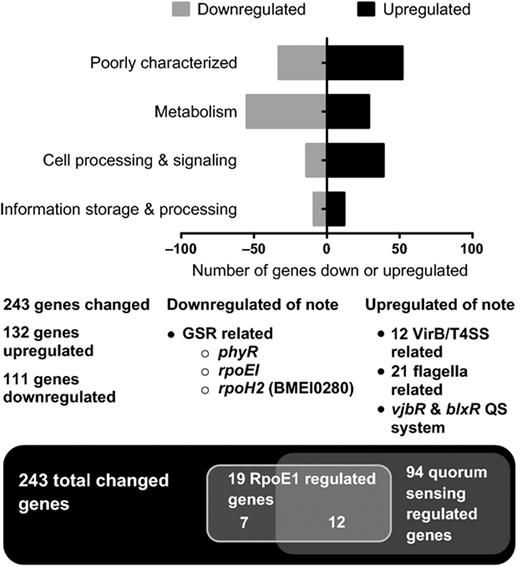
Differentially regulated genes in ΔBM-LOV-HK strain as compared to WT 16M strain from microarray data. One-hundred and thirty two total genes upregulated and 111 downregulated in mutant strain vs. WT. Numbers of genes are organized by COG functional category and represented by different black or grey bars. Of the 243 changed genes, 94 are known to be regulated by QS. Nineteen are regulated by RpoEI and 12 of the 19 are also regulated by QS. See Table S6 for additional detail. T4SS is type IV secretion. QS is quorum sensing. GSR is general stress response.
BME# . | Gene . | Fold change* . | LNN† . | Description . |
---|---|---|---|---|
General stress response | ||||
BMEI0371 | rpoE1 | −3.8 | 1.00 | RNA polymerase sigma-70 factor |
BMEI0372 | phyR | −2.4 | 1.00 | Two-component response regulator |
BMEI0373 | - | −4.2 | 1.00 | Hypothetical protein |
BMEI0374 | phyK | −5.9 | 1.00 | Sensory transduction histidine kinase |
BMEI0280 | rpoH2 | −3.0 | 1.00 | RNA polymerase sigma factor |
Quorum sensing | ||||
BMEII1116 | vjbR | 2.7 | 1.00 | Quorum sensing regulator protein |
BMEI1758 | blxR | 5.3 | 1.00 | Quorum sensing regulator protein |
Type IV secretion (virB) | ||||
BMEII0025 | virB1 | 3.3 | 1.00 | Lytic transglycosylase |
BMEII0026 | virB2 | 9.9 | 1.00 | Pilus |
BMEII0027 | virB3 | 9.4 | 1.00 | Channel protein |
BMEII0028 | virB4 | 5.4 | 1.00 | ATPase |
BMEII0029 | virB5 | 7.0 | 1.00 | Attachment mediating protein |
BMEII0030 | virB6 | 4.1 | 1.00 | IM Channel protein |
BMEII0031 | virB7 | 2.8 | 1.00 | Channel protein |
BMEII0032 | virB8 | 3.7 | 1.00 | Channel protein |
BMEII0033 | virB9 | 3.4 | 1.00 | Channel protein |
BMEII0034 | virB10 | 3.8 | 1.00 | Channel protein |
BMEII0035 | virB11 | 4.0 | 1.00 | ATPase |
BMEII0036 | virB12 | 2.8 | 1.00 | Outer membrane protein |
Flagella | ||||
BMEII0150 | fliC | 2.3 | 1.00 | Flagellin |
BMEII0151 | fliF | 2.4 | 1.00 | M-ring protein |
BMEII0159 | flgE | 1.9 | 0.89 | Hook protein |
BMEII0160 | flgK | 1.7 | 0.60 | Hook-associated protein (junction) |
BMEII0161 | flgL | 2.0 | 0.98 | Hook-associated protein (junction) |
BMEII0162 | flaF | 1.8 | 0.79 | Biosynthesis regulatory factor |
BMEII0170 | flgJ | 1.9 | 0.93 | Peptidoglycan hydrolyzing protein |
BMEII0171 | flgN | 2.0 | 0.98 | Type III secretion chaperone |
BMEII0172 | fliL | 1.9 | 0.95 | Basal body-associated protein |
BMEII1085 | flgA | 1.8 | 0.88 | P-ring biosynthesis protein |
BMEII1086 | flgG | 2.0 | 0.98 | Basal body rod protein |
BMEII1087 | fliE | 1.8 | 0.83 | Hook-basal body rod protein |
BMEII1088 | flgC | 2.0 | 0.95 | Basal body rod protein |
BMEII1089 | flgB | 1.8 | 0.79 | Basal body rod protein |
BMEII1107 | flgF | 2.5 | 1.00 | Basal body rod protein |
BMEII1108 | flgF | 2.6 | 1.00 | Basal body rod protein |
BMEII1110 | fliM | 1.7 | 0.67 | Motor switch protein (C-ring) |
BMEII1111 | - | 1.9 | 0.94 | Hypothetical protein |
BMEII1113 | fliG | 1.7 | 0.69 | Motor switch protein |
BMEII1114 | flhB | 7.0 | 1.00 | Biosynthesis protein - export apparatus |
BMEII1115 | - | 4.9 | 1.00 | Hypothetical protein |
BME# . | Gene . | Fold change* . | LNN† . | Description . |
---|---|---|---|---|
General stress response | ||||
BMEI0371 | rpoE1 | −3.8 | 1.00 | RNA polymerase sigma-70 factor |
BMEI0372 | phyR | −2.4 | 1.00 | Two-component response regulator |
BMEI0373 | - | −4.2 | 1.00 | Hypothetical protein |
BMEI0374 | phyK | −5.9 | 1.00 | Sensory transduction histidine kinase |
BMEI0280 | rpoH2 | −3.0 | 1.00 | RNA polymerase sigma factor |
Quorum sensing | ||||
BMEII1116 | vjbR | 2.7 | 1.00 | Quorum sensing regulator protein |
BMEI1758 | blxR | 5.3 | 1.00 | Quorum sensing regulator protein |
Type IV secretion (virB) | ||||
BMEII0025 | virB1 | 3.3 | 1.00 | Lytic transglycosylase |
BMEII0026 | virB2 | 9.9 | 1.00 | Pilus |
BMEII0027 | virB3 | 9.4 | 1.00 | Channel protein |
BMEII0028 | virB4 | 5.4 | 1.00 | ATPase |
BMEII0029 | virB5 | 7.0 | 1.00 | Attachment mediating protein |
BMEII0030 | virB6 | 4.1 | 1.00 | IM Channel protein |
BMEII0031 | virB7 | 2.8 | 1.00 | Channel protein |
BMEII0032 | virB8 | 3.7 | 1.00 | Channel protein |
BMEII0033 | virB9 | 3.4 | 1.00 | Channel protein |
BMEII0034 | virB10 | 3.8 | 1.00 | Channel protein |
BMEII0035 | virB11 | 4.0 | 1.00 | ATPase |
BMEII0036 | virB12 | 2.8 | 1.00 | Outer membrane protein |
Flagella | ||||
BMEII0150 | fliC | 2.3 | 1.00 | Flagellin |
BMEII0151 | fliF | 2.4 | 1.00 | M-ring protein |
BMEII0159 | flgE | 1.9 | 0.89 | Hook protein |
BMEII0160 | flgK | 1.7 | 0.60 | Hook-associated protein (junction) |
BMEII0161 | flgL | 2.0 | 0.98 | Hook-associated protein (junction) |
BMEII0162 | flaF | 1.8 | 0.79 | Biosynthesis regulatory factor |
BMEII0170 | flgJ | 1.9 | 0.93 | Peptidoglycan hydrolyzing protein |
BMEII0171 | flgN | 2.0 | 0.98 | Type III secretion chaperone |
BMEII0172 | fliL | 1.9 | 0.95 | Basal body-associated protein |
BMEII1085 | flgA | 1.8 | 0.88 | P-ring biosynthesis protein |
BMEII1086 | flgG | 2.0 | 0.98 | Basal body rod protein |
BMEII1087 | fliE | 1.8 | 0.83 | Hook-basal body rod protein |
BMEII1088 | flgC | 2.0 | 0.95 | Basal body rod protein |
BMEII1089 | flgB | 1.8 | 0.79 | Basal body rod protein |
BMEII1107 | flgF | 2.5 | 1.00 | Basal body rod protein |
BMEII1108 | flgF | 2.6 | 1.00 | Basal body rod protein |
BMEII1110 | fliM | 1.7 | 0.67 | Motor switch protein (C-ring) |
BMEII1111 | - | 1.9 | 0.94 | Hypothetical protein |
BMEII1113 | fliG | 1.7 | 0.69 | Motor switch protein |
BMEII1114 | flhB | 7.0 | 1.00 | Biosynthesis protein - export apparatus |
BMEII1115 | - | 4.9 | 1.00 | Hypothetical protein |
*Fold Change is ΔBM-LOV-HK/WT for positive fold change and −1/(ΔBM-LOV-HK/WT) for negative fold change.
†LNN value > 0.5 considered significant and calculated using ebarray package for Bioconductor.
BME# . | Gene . | Fold change* . | LNN† . | Description . |
---|---|---|---|---|
General stress response | ||||
BMEI0371 | rpoE1 | −3.8 | 1.00 | RNA polymerase sigma-70 factor |
BMEI0372 | phyR | −2.4 | 1.00 | Two-component response regulator |
BMEI0373 | - | −4.2 | 1.00 | Hypothetical protein |
BMEI0374 | phyK | −5.9 | 1.00 | Sensory transduction histidine kinase |
BMEI0280 | rpoH2 | −3.0 | 1.00 | RNA polymerase sigma factor |
Quorum sensing | ||||
BMEII1116 | vjbR | 2.7 | 1.00 | Quorum sensing regulator protein |
BMEI1758 | blxR | 5.3 | 1.00 | Quorum sensing regulator protein |
Type IV secretion (virB) | ||||
BMEII0025 | virB1 | 3.3 | 1.00 | Lytic transglycosylase |
BMEII0026 | virB2 | 9.9 | 1.00 | Pilus |
BMEII0027 | virB3 | 9.4 | 1.00 | Channel protein |
BMEII0028 | virB4 | 5.4 | 1.00 | ATPase |
BMEII0029 | virB5 | 7.0 | 1.00 | Attachment mediating protein |
BMEII0030 | virB6 | 4.1 | 1.00 | IM Channel protein |
BMEII0031 | virB7 | 2.8 | 1.00 | Channel protein |
BMEII0032 | virB8 | 3.7 | 1.00 | Channel protein |
BMEII0033 | virB9 | 3.4 | 1.00 | Channel protein |
BMEII0034 | virB10 | 3.8 | 1.00 | Channel protein |
BMEII0035 | virB11 | 4.0 | 1.00 | ATPase |
BMEII0036 | virB12 | 2.8 | 1.00 | Outer membrane protein |
Flagella | ||||
BMEII0150 | fliC | 2.3 | 1.00 | Flagellin |
BMEII0151 | fliF | 2.4 | 1.00 | M-ring protein |
BMEII0159 | flgE | 1.9 | 0.89 | Hook protein |
BMEII0160 | flgK | 1.7 | 0.60 | Hook-associated protein (junction) |
BMEII0161 | flgL | 2.0 | 0.98 | Hook-associated protein (junction) |
BMEII0162 | flaF | 1.8 | 0.79 | Biosynthesis regulatory factor |
BMEII0170 | flgJ | 1.9 | 0.93 | Peptidoglycan hydrolyzing protein |
BMEII0171 | flgN | 2.0 | 0.98 | Type III secretion chaperone |
BMEII0172 | fliL | 1.9 | 0.95 | Basal body-associated protein |
BMEII1085 | flgA | 1.8 | 0.88 | P-ring biosynthesis protein |
BMEII1086 | flgG | 2.0 | 0.98 | Basal body rod protein |
BMEII1087 | fliE | 1.8 | 0.83 | Hook-basal body rod protein |
BMEII1088 | flgC | 2.0 | 0.95 | Basal body rod protein |
BMEII1089 | flgB | 1.8 | 0.79 | Basal body rod protein |
BMEII1107 | flgF | 2.5 | 1.00 | Basal body rod protein |
BMEII1108 | flgF | 2.6 | 1.00 | Basal body rod protein |
BMEII1110 | fliM | 1.7 | 0.67 | Motor switch protein (C-ring) |
BMEII1111 | - | 1.9 | 0.94 | Hypothetical protein |
BMEII1113 | fliG | 1.7 | 0.69 | Motor switch protein |
BMEII1114 | flhB | 7.0 | 1.00 | Biosynthesis protein - export apparatus |
BMEII1115 | - | 4.9 | 1.00 | Hypothetical protein |
BME# . | Gene . | Fold change* . | LNN† . | Description . |
---|---|---|---|---|
General stress response | ||||
BMEI0371 | rpoE1 | −3.8 | 1.00 | RNA polymerase sigma-70 factor |
BMEI0372 | phyR | −2.4 | 1.00 | Two-component response regulator |
BMEI0373 | - | −4.2 | 1.00 | Hypothetical protein |
BMEI0374 | phyK | −5.9 | 1.00 | Sensory transduction histidine kinase |
BMEI0280 | rpoH2 | −3.0 | 1.00 | RNA polymerase sigma factor |
Quorum sensing | ||||
BMEII1116 | vjbR | 2.7 | 1.00 | Quorum sensing regulator protein |
BMEI1758 | blxR | 5.3 | 1.00 | Quorum sensing regulator protein |
Type IV secretion (virB) | ||||
BMEII0025 | virB1 | 3.3 | 1.00 | Lytic transglycosylase |
BMEII0026 | virB2 | 9.9 | 1.00 | Pilus |
BMEII0027 | virB3 | 9.4 | 1.00 | Channel protein |
BMEII0028 | virB4 | 5.4 | 1.00 | ATPase |
BMEII0029 | virB5 | 7.0 | 1.00 | Attachment mediating protein |
BMEII0030 | virB6 | 4.1 | 1.00 | IM Channel protein |
BMEII0031 | virB7 | 2.8 | 1.00 | Channel protein |
BMEII0032 | virB8 | 3.7 | 1.00 | Channel protein |
BMEII0033 | virB9 | 3.4 | 1.00 | Channel protein |
BMEII0034 | virB10 | 3.8 | 1.00 | Channel protein |
BMEII0035 | virB11 | 4.0 | 1.00 | ATPase |
BMEII0036 | virB12 | 2.8 | 1.00 | Outer membrane protein |
Flagella | ||||
BMEII0150 | fliC | 2.3 | 1.00 | Flagellin |
BMEII0151 | fliF | 2.4 | 1.00 | M-ring protein |
BMEII0159 | flgE | 1.9 | 0.89 | Hook protein |
BMEII0160 | flgK | 1.7 | 0.60 | Hook-associated protein (junction) |
BMEII0161 | flgL | 2.0 | 0.98 | Hook-associated protein (junction) |
BMEII0162 | flaF | 1.8 | 0.79 | Biosynthesis regulatory factor |
BMEII0170 | flgJ | 1.9 | 0.93 | Peptidoglycan hydrolyzing protein |
BMEII0171 | flgN | 2.0 | 0.98 | Type III secretion chaperone |
BMEII0172 | fliL | 1.9 | 0.95 | Basal body-associated protein |
BMEII1085 | flgA | 1.8 | 0.88 | P-ring biosynthesis protein |
BMEII1086 | flgG | 2.0 | 0.98 | Basal body rod protein |
BMEII1087 | fliE | 1.8 | 0.83 | Hook-basal body rod protein |
BMEII1088 | flgC | 2.0 | 0.95 | Basal body rod protein |
BMEII1089 | flgB | 1.8 | 0.79 | Basal body rod protein |
BMEII1107 | flgF | 2.5 | 1.00 | Basal body rod protein |
BMEII1108 | flgF | 2.6 | 1.00 | Basal body rod protein |
BMEII1110 | fliM | 1.7 | 0.67 | Motor switch protein (C-ring) |
BMEII1111 | - | 1.9 | 0.94 | Hypothetical protein |
BMEII1113 | fliG | 1.7 | 0.69 | Motor switch protein |
BMEII1114 | flhB | 7.0 | 1.00 | Biosynthesis protein - export apparatus |
BMEII1115 | - | 4.9 | 1.00 | Hypothetical protein |
*Fold Change is ΔBM-LOV-HK/WT for positive fold change and −1/(ΔBM-LOV-HK/WT) for negative fold change.
†LNN value > 0.5 considered significant and calculated using ebarray package for Bioconductor.
A selection of 15 genes was chosen for expression analysis by qRT-PCR to verify the microarray expression findings. Three biological replicates for each strain were isolated from log phase cultures and analyzed, and these RNA isolations were separate from isolations used to generate microarray expression data. Gene expression was normalized to the expression of the IF-1 (BMEI1671) gene. Trends for gene expression were consistent with microarray data for selected genes, Table 2.
BME# . | Name . | Fold change . | Fold change . |
---|---|---|---|
. | . | qRT-PCR . | microarray . |
BMEI0280 | rpoH2 | −9.2 | −3.0 |
BMEI0371 | rpoE1 | −8.0 | −3.8 |
BMEI0372 | phyR | −8.5 | −2.4 |
BMEI0374 | phyK | −9.2 | −5.9 |
BMEI0884 | gryA | −1.2 | 1.1 |
BMEI1758 | blxR/babR | 2.1 | 5.4 |
BMEII0026 | virB2 | 2.7 | 9.9 |
BMEII0029 | virB5 | 1.7 | 7.0 |
BMEII0035 | virB11 | 1.2 | 4.0 |
BMEII0105 | frpB | −1.6 | −3.4 |
BMEII0150 | fliC | 2.0 | 2.3 |
BMEII0151 | fliF | 5.1 | 2.4 |
BMEII0159 | flgE | 3.2 | 1.9 |
BMEII1113 | fliG | 3.4 | 1.7 |
BMEII1116 | vjbR | 3.4 | 2.7 |
BME# . | Name . | Fold change . | Fold change . |
---|---|---|---|
. | . | qRT-PCR . | microarray . |
BMEI0280 | rpoH2 | −9.2 | −3.0 |
BMEI0371 | rpoE1 | −8.0 | −3.8 |
BMEI0372 | phyR | −8.5 | −2.4 |
BMEI0374 | phyK | −9.2 | −5.9 |
BMEI0884 | gryA | −1.2 | 1.1 |
BMEI1758 | blxR/babR | 2.1 | 5.4 |
BMEII0026 | virB2 | 2.7 | 9.9 |
BMEII0029 | virB5 | 1.7 | 7.0 |
BMEII0035 | virB11 | 1.2 | 4.0 |
BMEII0105 | frpB | −1.6 | −3.4 |
BMEII0150 | fliC | 2.0 | 2.3 |
BMEII0151 | fliF | 5.1 | 2.4 |
BMEII0159 | flgE | 3.2 | 1.9 |
BMEII1113 | fliG | 3.4 | 1.7 |
BMEII1116 | vjbR | 3.4 | 2.7 |
Brucella melitensis 16M number (BME#), NCBI searchable. Name: Short gene name. Fold Change is ΔBM-LOV-HK/WT for positive fold change and −1/(ΔBM-LOV-HK/WT) for negative fold change. Expression data for qRT-PCR were calculated with ΔΔCT method.
BME# . | Name . | Fold change . | Fold change . |
---|---|---|---|
. | . | qRT-PCR . | microarray . |
BMEI0280 | rpoH2 | −9.2 | −3.0 |
BMEI0371 | rpoE1 | −8.0 | −3.8 |
BMEI0372 | phyR | −8.5 | −2.4 |
BMEI0374 | phyK | −9.2 | −5.9 |
BMEI0884 | gryA | −1.2 | 1.1 |
BMEI1758 | blxR/babR | 2.1 | 5.4 |
BMEII0026 | virB2 | 2.7 | 9.9 |
BMEII0029 | virB5 | 1.7 | 7.0 |
BMEII0035 | virB11 | 1.2 | 4.0 |
BMEII0105 | frpB | −1.6 | −3.4 |
BMEII0150 | fliC | 2.0 | 2.3 |
BMEII0151 | fliF | 5.1 | 2.4 |
BMEII0159 | flgE | 3.2 | 1.9 |
BMEII1113 | fliG | 3.4 | 1.7 |
BMEII1116 | vjbR | 3.4 | 2.7 |
BME# . | Name . | Fold change . | Fold change . |
---|---|---|---|
. | . | qRT-PCR . | microarray . |
BMEI0280 | rpoH2 | −9.2 | −3.0 |
BMEI0371 | rpoE1 | −8.0 | −3.8 |
BMEI0372 | phyR | −8.5 | −2.4 |
BMEI0374 | phyK | −9.2 | −5.9 |
BMEI0884 | gryA | −1.2 | 1.1 |
BMEI1758 | blxR/babR | 2.1 | 5.4 |
BMEII0026 | virB2 | 2.7 | 9.9 |
BMEII0029 | virB5 | 1.7 | 7.0 |
BMEII0035 | virB11 | 1.2 | 4.0 |
BMEII0105 | frpB | −1.6 | −3.4 |
BMEII0150 | fliC | 2.0 | 2.3 |
BMEII0151 | fliF | 5.1 | 2.4 |
BMEII0159 | flgE | 3.2 | 1.9 |
BMEII1113 | fliG | 3.4 | 1.7 |
BMEII1116 | vjbR | 3.4 | 2.7 |
Brucella melitensis 16M number (BME#), NCBI searchable. Name: Short gene name. Fold Change is ΔBM-LOV-HK/WT for positive fold change and −1/(ΔBM-LOV-HK/WT) for negative fold change. Expression data for qRT-PCR were calculated with ΔΔCT method.
Many genes differentially expressed in the ΔBM-LOV-HK strain are regulated by Brucella QS and general GSRs
Several previous studies have identified genes differentially expressed when QS regulator genes vjbR and blxR (babR) are separately deleted from parent strains (Rambow-Larsen et al., 2008; Uzureau et al., 2010; Weeks et al., 2010). Because expression of both vjbR and blxR was upregulated in the ΔBM-LOV-HK strain, we conducted an analysis of previous studies to identify QS regulated components also differentially regulated in the ΔBM-LOV-HK strain compared to B. melitensis. Of the 243 differentially expressed genes in the ΔBM-LOV-HK strain, 94 of these genes overlapped with genes differentially regulated by at least one QS regulator deletion. Additionally, the downregulation of several GSR regulatory components in the ΔBM-LOV-HK strain led us to expand our analysis to include genes differentially regulated in a recent study comparing gene expression in a B. abortus rpoE1 deletion mutant to wild type under oxidative stress (Kim et al., 2013). Nineteen genes were differentially regulated in both the ΔBM-LOV-HK strain and the B. abortus rpoE1 deletion strain. Of those 19 overlapping genes, 12 are also differentially regulated in QS studies, Fig. 1. An expanded analysis including data specific to individual genes is available in Table S6.
Both C567BL/6 and IRF-1−/− mice infected with the ΔBM-LOV-HK strain had decreased spleen CFUs and IRF-1−/− mice infected with the ΔBM-LOV-HK strain had increased survival compared to IRF-1−/− mice infected with B. melitensis
C57BL/6 mice were infected with the ΔBM-LOV-HK strain and wild-type B. melitensis, and CFUs per gram of spleen tissue were determined at 3 and 6 days postinfection. At day 3, the ΔBM-LOV-HK strain possessed decreased CFUs compared to wild type; however, by day 6, CFUs for mutant and wild-type bacteria were similar (Fig. 2a). These results suggest a delay in growth or greater initial killing for the ΔBM-LOV-HK strain during initial C57BL/6 mouse infection. By day 6, mutant and wild-type CFUs were similar, therefore, to better investigate in vivo attenuation of the ΔBM-LOV-HK strain, IRF-1−/− mice were infected with the ΔBM-LOV-HK and wild-type B. melitensis strains.
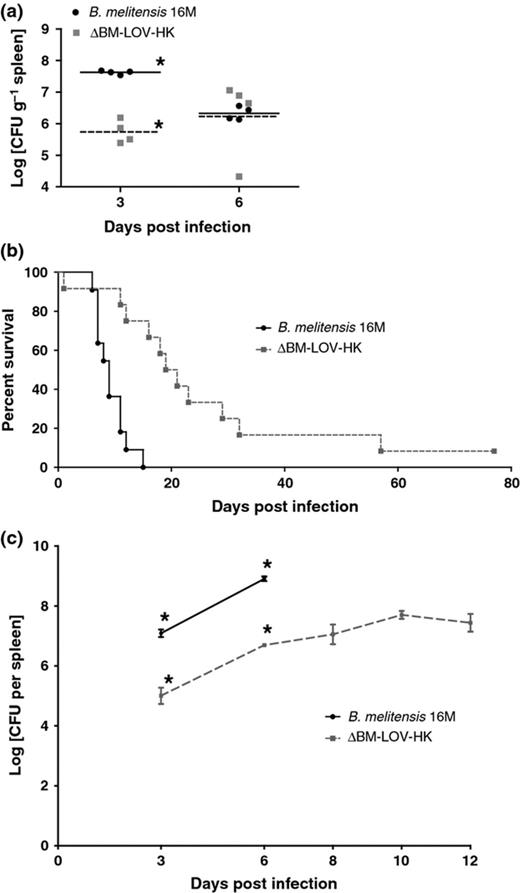
Decreased virulence of ΔBM-LOV-HK in mice. (a) C57BL/6 mouse spleen CFUs (n = 4 each per time point) infected with 16M or ΔBM-LOV-HK. ΔBM-LOV-HK infected spleens contain significantly less bacteria at 3 days postinfection (*P = 0.0286, Mann-Whitney) but not at 6 days postinfection. 16M & ΔBM-LOV-HK strains used in part (a) contain empty pBBRmcs4 plasmid. (b) Percent survival of IRF-1−/− mice infected with Brucella melitensis 16M (n = 11) or ΔBM-LOV-HK (n = 12). ΔBM-LOV-HK infected mice survive significantly longer than 16M infected mice (P = 0.0001, Mantel-Cox). (c) IRF-1−/− mouse spleen CFUs (n = 4 each per time point) infected with 16M or ΔBM-LOV-HK. ΔBM-LOV-HK infected spleens contain significantly less bacteria at 3 and 6 days postinfection (*P = 0.0286, Mann-Whitney) for both time points. 16M infected IRF-1−/− mice begin to succumb to infection 6 days postinfection. Means shown by horizontal lines in (a) standard error of mean shown by error bars in (c) and infectious dose of 1 × 106 CFUs for a-c.
The IRF-1−/− mouse provides an informative in vivo model for Brucella infection because this mouse can distinguish various levels of mutant Brucella strain attenuation. The ability to kill IRF-1−/− mice has been shown to be a strong indicator of virulence for Brucella mutants, and some highly attenuated bacterial mutants do not readily kill IRF-1−/− mice (Matsuyama et al., 1993; Ko et al., 2002a,b; Baek et al., 2004; Rajashekara et al., 2005a,b, 2006, 2008; Miyoshi et al., 2007; Rambow-Larsen et al., 2008; Trant et al., 2010; Petersen et al., 2011; Arenas-Gamboa et al., 2012). To determine the importance of BM-LOV-HK to in vivo virulence, survival of IRF-1−/− mice was determined after infection with either wild-type B. melitensis or the ΔBM-LOV-HK strain. ΔBM-LOV-HK infected IRF-1−/− mice survived significantly longer than wild-type Brucella infected mice (Fig. 2b), and significantly fewer CFUs were recovered from ΔBM-LOV-HK infected spleens than wild-type infected spleens at three and six days postinfection (Fig. 2c). Wild-type Brucella infected IRF-1−/− mice began to succumb 6 days after infection (Fig. 2b). Wild-type infected mice reached an average bacterial spleen load of 1 × 109 CFUs at 6 days of infection, while the average bacterial load of ΔBM-LOV-HK infected spleens was 1 × 108 CFUs or less up to twelve days postinfection. These results suggest deletion of BM-LOV-HK contributes to a reduced B. melitensis virulence phenotype but does not prevent eventual death of IRF-1−/− mice as do some B. melitensis gene deletions (Matsuyama et al., 1993; Ko et al., 2002a,b; Baek et al., 2004; Rajashekara et al., 2005a,b, 2006, 2008; Miyoshi et al., 2007; Rambow-Larsen et al., 2008; Trant et al., 2010; Petersen et al., 2011; Arenas-Gamboa et al., 2012). Both IRF-1−/− and C57BL/6 mouse infections show decreased ΔBM-LOV-HK strain virulence compared to wild type. These results suggest BM-LOV-HK is necessary for full B. melitensis in vivo virulence.
DISCUSSION
The deletion of BM-LOV-HK demonstrates that this light-sensing kinase affects expression of vital Brucella virulence genes that may alter murine infection. Sensing of environmental signals is essential for bacterial survival. However, the extent to which a light-sensing kinase may exert an effect on gene expression in a facultative intracellular pathogen had been unclear. A genome scale microarray expression comparison between the ΔBM-LOV-HK strain and B. melitensis 16M identified 243 genes directly or indirectly regulated by BM-LOV-HK expression, and many of these genes are involved in control and regulation of virulence and general stress response. Of specific interest was upregulation of the T4SS/VirB operon and QS system. Our analysis of previous studies identified 94 differentially expressed genes in ΔvjbR and ΔblxR strains that were also differentially expressed in the ΔBM-LOV-HK strain. The direct signaling relationship between BM-LOV-HK and the QS and T4SS remains to be identified, but virB genes and a functional T4SS are essential for full Brucella virulence and proper trafficking within cells (Hong et al., 2000; Comerci et al., 2001; Boschiroli et al., 2002; Watarai et al., 2002; Rouot et al., 2003; Zygmunt et al., 2006; Roux et al., 2007; Nijskens et al., 2008; Starr et al., 2008; Paixão et al., 2009; de Jong and Tsolis, 2011). Consistent with VirB operon upregulation, mutant strain expression of QS components vjbR and blxR were also significantly increased. VjbR and BlxR are known regulators of VirB expression and an increase in VjbR would increase VirB expression (Delrue et al., 2005; Arocena et al., 2010). There is debate as to the role of BlxR expression on VirB. BlxR could be acting as a repressor, activator, or both depending on circumstances (Rambow-Larsen et al., 2008; Uzureau et al., 2010; Caswell et al., 2012). In this case, both QS regulators and the T4SS are upregulated. Abnormal expression of Brucella QS and T4SS components may cause unknown pleiotropic effects, such as deleterious secretion of bacterial proteins (de Jong et al., 2008; den Hartigh et al., 2008). Two proteins, VceA and BPE123, are transported by the Brucella T4SS and are differentially regulated in the ΔBM-LOV-HK strain (de Jong et al., 2008; Marchesini et al., 2011). VceA was downregulated, while BPE123 was upregulated and is encoded near flagella genes. A large collection of flagellar genes were also upregulated in the ΔBM-LOV-HK strain. This observation is consistent with previous work showing a strong overlap in flagellar genes, including fliC, fliF, flgE, and flaF, being upregulated after the deletion of the RpoE1 sigma factor, which was significantly downregulated in the ΔBM-LOV-HK strain (Ferooz et al., 2011). Also, regulation of expression of flagellar genes can be controlled by an additional RpoH sigma factor and the two-component regulator FtcR, and neither of these genes were differentially regulated in our array (Delory et al., 2006; Leonard et al., 2007). Although the presence of certain flagellar genes is important for Brucella virulence, the exact mechanisms by which flagellar genes contribute to Brucella virulence are unknown.
In addition to regulating flagellar genes, the RpoE1 sigma factor has homologues in other bacteria that regulate genes responsible for bacterial general stress response (GSR) to stressors such as heat, salt concentration, and nutrient limitation (Alvarez-Martinez et al., 2006, 2007; Gourion et al., 2006, 2008; Sauviac et al., 2007; Martínez-Salazar et al., 2009). Both the rpoE1 gene and the phyR gene, a known GSR partner, are downregulated in the ΔBM-LOV-HK strain. Nineteen genes differentially regulated in a B. abortus ΔrpoE1 strain were also differentially regulated in the ΔBM-LOV-HK strain (Kim et al., 2013). Twelve of these genes overlapped with genes differentially regulated in the above mentioned QS studies. Recently, ΔrpoE1 and ΔphyR B. abortus mutants were reported as more sensitive to oxidative stress than wild-type B. abortus (Kim et al., 2013). Also, Brucella rpoE1 mutants have decreased virulence in mouse models of infection, but this attenuation is much later than what we have currently demonstrated for ΔBM-LOV-HK strain infections (Delory et al., 2006; Kim et al., 2013). We had separately deleted phyR and a neighboring kinase we term phyK (BMEI0374) from B. melitensis. Consistent with Kim et al., 2013, we noted no growth difference (in culture or RAW 264.7 macrophages) or difference in survival in IRF-1−/− mice for either mutant strain compared to wild-type B. melitensis (data not shown). Clearly, biochemical interaction analyses, stress condition testing, and animal experiments to directly compare persistence of the ΔBM-LOV-HK strain and a B. melitensis rpoE1 deletion mutant, in wild-type mice, are warranted for future studies. More than a third of all differentially regulated genes in the ΔBM-LOV-HK strain appear to be controlled at a level downstream from BM-LOV-HK by QS, GSR, or in tandem by both systems. Together, these analyses reveal two possible arms of BM-LOV-HK signaling, QS and GSR. Our results suggest BM-LOV-HK may act upstream to modulate both QS and GSR regulatory components to control Brucella gene expression on a global level.
Data are emerging on direct signaling partners for BM-LOV-HK. Others have indicated LOV-HK binds and phosphorylates PhyR in vitro (Rinaldi et al., 2012) providing a direct link between LOV-HK and the RpoE1 sigma factor. This link could explain many of the changes in gene expression observed in our ΔBM-LOV-HK strain, specifically with stress response and flagellar genes. Further, at least two uncharacterized kinases surround the phyR operon. One of these kinases, PhyK, shares significant homology to BM-LOV-HK but does not have a LOV domain and likely reacts to other environmental signals (not light). A picture of regulation is developing in which BM-LOV-HK may activate the RpoE1 pathway under certain stimuli, while another kinase such as PhyK may subsume that role or even modulate PhyR phosphorylation at a later point in growth.
Taken together, both C57BL/6 and IRF-1−/− mouse infection results point to a possible important role for BM-LOV-HK during the early stage of mouse infection. The delayed death and decreased spleen CFUs in mutant infected IRF-1−/− mice may be explained by impaired intracellular replication or pathogenesis, increased initial killing of mutant bacteria, or improper trafficking within the host. Further work to determine trafficking of the ΔBM-LOV-HK strain within mice may shed light on whether the mutant is abrogated in dissemination. Future animal experiments to directly compare persistence of the ΔBM-LOV-HK strain and a B. melitensis rpoE1 deletion mutant, in wild-type mice, may also be informative. Attempts to restore ΔBM-LOV-HK to wild-type virulence in mice have yielded unexpected findings of further attenuation beyond the ΔBM-LOV-HK phenotype (Fig. S1). An interpretation is that improper expression of the BM-LOV-HK gene leads to further ΔBM-LOV-HK strain attenuation compared to wild-type B. melitensis as suggested by Fig. S1a and b. Others have shown that improper expression of virB components including the overexpression of VirB5 can lead to B. suis 1330 attenuation (Sprynski et al., 2012). Also, as discussed above, the expression of the VirB operon is significantly altered in the ΔBM-LOV-HK strain compared to wild type.
Results have shown that BM-LOV-HK can sense and be phosphorylated in the presence of a blue light signal (Swartz et al., 2007). Future work remains to identify and confirm the direct BM-LOV-HK interaction partners and network as well as the importance of extra organismal light activation of BM-LOV-HK for in vivo Brucella virulence. Is a light-sensing kinase at the apex of the regulatory hierarchy for expression of these genes? Is extra organismal blue light the only activation signal to which BM-LOV-HK responds, or could there be an internal host signal that activates the protein? In the present study, we have shown that BM-LOV-HK is important for B. melitensis virulence in an animal model of infection. Deletion of BM-LOV-HK decreases virulence of B. melitensis during C57BL/6 and IRF-1−/− mouse infections. Brucella melitensis gene expression was altered by deletion of BM-LOV-HK. Our results suggest that BM-LOV-HK directly or indirectly regulates members of the Brucella QS and general stress systems.
This work was supported by NIH grants AI073558, RO3AI101611, and BARD grant US-4378-11.
Conflict of interest statement. None declared.
SUPPLEMENTARY DATA
Figure S1. Spleen CFUs and IRF-1−/− mouse survival failed to show ΔBM-LOV-HK strain restored to WT virulence with lac promoter plasmid expression of BM-LOV-HK.
Table S1. Bacterial strains and vectors utilized.
Table S2. Deletion primers.
Table S3. qRT-PCR primers.
Table S4. Genes upregulated in δBM-LOV-HK as compared to WT 16M.
Table S5. Genes downregulated in δBM-LOV-HK as compared to WT 16M.
Table S6. Genes regulated By BM-LOV-HK, VjbR, BlxR/BabR, and RpoE1.
References