-
PDF
- Split View
-
Views
-
Cite
Cite
Hong Kuan Deng, Danielle Le Rhun, Benoit Lecuelle, Evelyne Le Naour, Muriel Vayssier-Taussat, Role of the spleen in Bartonella spp. infection, FEMS Immunology & Medical Microbiology, Volume 64, Issue 1, February 2012, Pages 143–145, https://doi.org/10.1111/j.1574-695X.2011.00908.x
- Share Icon Share
Abstract
Bartonella spp. are intra-erythrocytic pathogens of mammals. In this study, we investigated the role of the spleen, and other tissue and organs in Bartonella infection. Using an in vivo model of mice infection by Bartonella birtlesii, we detected accumulation of bacteria in the spleen, with transient infection of the liver, but failed to detect any bacteria in brain or lymph nodes. We then compared bacteraemia in normal Balb/C mice and in splenectomized mice. Bacteraemia in splenectomized mice was 10-fold higher than in normal mice and lasted 2 weeks longer. In conclusion, the spleen seems to retain and filter infected erythrocytes rather than to be a sanctuary for chronic Bartonella infection.
The bacterial genus Bartonella comprises at least 24 species or subspecies of facultative bacteria that use arthropod transmission and haemotropism as a mammalian parasitism strategy. Each species is highly adapted to infect one or few reservoir hosts in which they induce asymptomatic intra-erythrocytic bacteraemia (Vayssier-Taussat et al., 2009). When they are introduced into an accidental host or into immuno-compromised individuals, infection can result in diverse clinical manifestations. The mode of infection of the reservoir hosts is common to all Bartonella species. Following injection, the bacterial inoculum is cleared from circulating blood within a few hours but bacteraemia reappears approximately 5 days later. Bacteria can be observed, adhering to and invading mature erythrocytes in the blood within 2 days. An erythrocyte is usually invaded by a single bacterium, which, once inside, replicates in a membrane-bound compartment over a period of several days until eight daughter cells, on average, are created. Replication then ceases, and the infected erythrocytes have been shown to persist in the circulation for several weeks (Schulein et al., 2001; Rolain et al., 2003).
The stages of infection that precede the arrival of bartonellae in the bloodstream have been far less well characterized. The current opinion is that the vascular endothelial cells may serve as a primary niche for Bartonella spp. before they enter the bloodstream (Dehio, 1999, 2001; Dehio, et al., 2001; Schulein et al., 2001; Schmid et al., 2004, 2006; Pulliainen & Dehio, 2009). However, other putative cells, such as erythrocytic precursors, have also been proposed as putative niches of Bartonella spp. (Rolain et al., 2003; Mandle et al., 2005).
The aim in this study was to explore the different possible locations of Bartonella spp. in the first week following infection, using Balb/C mice infected with a rodent-adapted Bartonella birtlesii (Boulouis et al., 2001). For this purpose, an inoculum of 5 × 107 colony forming units (CFU) of B. birtlesii (IBS 325T strain) was inoculated into the caudal vein of Balb/C mice. At 0.5, 1, 2, 3 and 7 days post-infection, the mice were euthanized and the lymph nodes, bone marrow, spleen, liver, brain and blood were sampled. Organ samples were mechanically crushed using a Dounce potter in 1 mL of F12 medium before being grown for 5 days on Columbia agar containing 5% defibrinated sheep blood (CBA) in a humidified atmosphere with 5% CO2 at 35 °C. Cells from lymph nodes (axillary, inguinal and mesenteral lymph nodes) and bone marrow were isolated by disrupting the lymph nodes, and flushing medium through the bone. The recovered cells and erythrocytes were then lysed by hypotonic shock and the released bacteria were grown on CBA in a humidified atmosphere with 5% CO2 at 35 °C.
As shown in Fig. 1a, no bacteria were recovered from the lymph nodes, bone marrow or brain, indicating that these tissues or organs were not targets for B. birtlesii in this animal model of Bartonella sp. infection. In contrast, B. birtlesii colonized the liver and, to a much greater extent, the spleen during the first 7 days following infection, and before seeding the bloodstream. Whereas liver colonization stopped after this first step, the spleen remained highly infected throughout the duration of bacteraemia (data not shown).
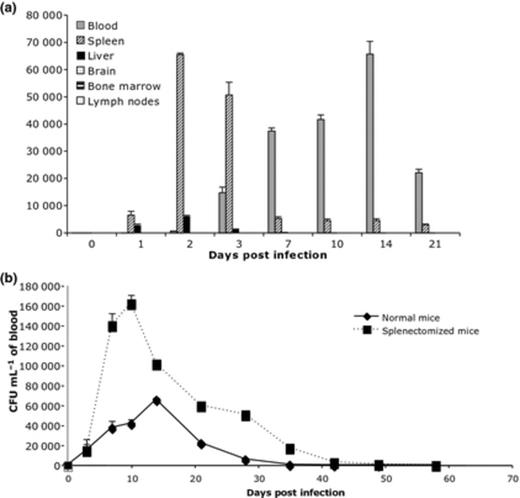
(a) Detection of bacteria in different organs, tissues, and in blood during the first 7 days of Balb/C mice infection with Bartonella birtlesii. The recovered bacteria are expressed in CFU mL−1 of blood; CFU mg−1 of organs, and CFU per 104 of cells recovered from lymph nodes and bone marrow. n = 5 (per day). (b) Bacteraemia in normal or splenectomized Balb/C mice infected with B. birtlesii. n = 10.
To investigate whether the spleen could provide a possible sanctuary for chronic Bartonella infection, as demonstrated for other bacteria (Trulzsch et al., 2007; Watson & Holden, 2010), and whether the spleen filters infected erythrocytes, as shown for Plasmodium falciparum (Safeukui et al., 2008), bacteraemia induced by B. birtlesii infection was compared in normal Balb/C mice and in splenectomized mice. During the first 7 days, bacteraemia was the same in both normal and splenectomized mice. Thereafter, bacteraemia was 10-fold higher in splenectomized mice than in normal mice and lasted 2 weeks longer (Fig. 1b).
The spleen is an important site for host response to bacterial infection (Junt et al., 2008). Within the spleen, free bacteria may encounter various tissue-resident phagocytes, such as macrophages, dendritic cells and neutrophils, which could eliminate them. In our model, during the first week following infection (when B. birtlesii was not yet inside the erythrocytes), having a spleen or not had no effect on the time of appearance of bacteria in blood or the level of bacteraemia. This suggests that the spleen does not have a fundamental role in the first step of infection, based on the model used. Once the bacteria were intra-erythrocytic, the spleen appeared to strangle the infection, as bacteraemia was 10-fold higher in splenectomized mice. This data suggest that the spleen has a role in clearing Bartonella-infected erythrocytes.
The elimination of microorganisms in the spleen is mainly triggered by the clearance by the splenic macrophages of opsonized encapsulated bacteria (such as pneumococci, meningococci and Escherichia coli) and intra-erythrocytic parasites (such as those causing malaria and babesiosis). However, in the specific case of intra-erythrocytic parasites such as Plasmodium sp., the spleen may also retain Plasmodium-infected erythrocytes through mechanical sensing of deformed or rigid erythrocytes, which explains the fulminant nature of these infections in persons with anatomic or functional asplenia (Safeukui et al., 2008). In our study, we have shown that the spleen is able to retain Bartonella spp., although the mechanisms of retention have still to be elucidated. First, we have to confirm that Bartonella is localized within the spleen erythrocytes. If this is so, we will then have to determine the mechanism by which the infected erythrocytes are retained. Indeed, it is widely accepted that the infection of erythrocytes by Bartonella has no effect on their physiology (no changes in life span or in membrane structures, etc.) (Schulein et al., 2001). However, a deformation factor, called ‘deformin’, has been identified in Bartonella bacilliformis and Bartonella henselae which causes marked physiological changes (pitting and invagination) of the erythrocyte membranes (Xu et al., 1995; Iwaki-Egawa & Ihler, 1997). This factor could insert itself into the erythrocyte membrane as a pore-like structure, leading to deformation of the erythrocytes. This would explain the differences in clearance between infected and uninfected erythrocytes, the altered erythrocytes being filtered out by spleen as demonstrated, for example, for Plasmodium sp. (Buffet et al., 2011).
It would be interesting to elucidate whether retention of poorly deformable red blood cells also has a crucial impact on Bartonella spp. infections.
References