-
PDF
- Split View
-
Views
-
Cite
Cite
Leandra Cronjé, Nicole Edmondson, Kathleen D. Eisenach, Liza Bornman, Iron and iron chelating agents modulate Mycobacterium tuberculosis growth and monocyte-macrophage viability and effector functions, FEMS Immunology & Medical Microbiology, Volume 45, Issue 2, August 2005, Pages 103–112, https://doi.org/10.1016/j.femsim.2005.02.007
- Share Icon Share
Abstract
Excess of iron promotes Mycobacterium tuberculosis infection, its replication and progression to clinical disease and death from tuberculosis. Chelation of iron may reduce M. tuberculosis replication, restore host defence mechanisms and it could constitute an application in the prevention and treatment strategies where both iron overload and tuberculosis are prevalent. We investigated the effect of iron and iron chelating agents, like desferrioxamine and silybin, individually and in combination with iron on mycobacterial number, viability in culture and after recovery from monocyte-macrophages, together with monocyte-macrophages viability and oxidative defence. Mycobacterial number and viability in culture were assessed using real-time quantitative PCR of H37Rv IS6110 DNA, 16S rRNA and 85B mRNA, whereas the microplate AlamarBlue™ assay was used to detect viability in culture post-infection. Mitochondrial membrane potential and phosphatidyl serine exposure of monocyte-macrophages, detected using Mitotracker Red fluorescence and Annexin V binding, respectively, served as indicators of host cell viability. Superoxide generation served as marker of monocyte-macrophage effector functions. Extracellular H37Rv showed a significant increase in number and viability in presence of excess iron and, by large, a significant decrease in number and viability in presence of the iron chelating agents, silybin and desferrioxamine, compared to cultivation without supplementation. Intracellularly, excess iron increased H37Rv viability significantly but reduced monocyte-macrophages mitochondrial membrane potential and compromised superoxide production. Desferrioxamine had little influence on intracellular parameters, but consistently prevented effects of excess iron, while silybin significantly altered most intracellular parameters and mostly failed to prevent effects of excess iron. These findings suggest that chelation therapy should be considered in conditions of iron overload and that effective chelating agents like desferrioxamine, with limited intracellular access might need to be used in combination with lypophilic chelating agents.
1 Introduction
Iron is essential for macrophage antimicrobial effector mechanisms, modulating NADPH-dependent oxidative burst, NO production by iNOS, ▪OH generation by the Fenton/Haber–Weiss reaction and cytokine activities [1,2]. However, it is also an obligate requirement for growth and survival of intracellular bacteria. A critical balance in iron levels is therefore required to support optimum protection by immune cells during infection. Iron deficiency reduces the ability of neutrophils to kill bacteria, decreases lymphocyte response to mitogens and antigens, and impairs natural killer (NK) cell activity, while an excess of iron undermines immune protection, promotes free radical tissue damage and facilitates pathogen replication and invasion [2]. The latter is particularly true for Mycobacterium tuberculosis[3] holding an iron-dependent regulator, IdeR, that controls the production of proteins involved in virulence [4], iron metabolism and the M. tuberculosis oxidative stress response [5,6]. Iron is an obligate cofactor for forty different enzymes encoded by the M. tuberculosis genome [7], including enzymes involved in oxygen metabolism and DNA synthesis as well as haem-containing catalase peroxidase (katG), iron-cofactored superoxide dismutase (SOD) and catalase (CAT). These enzymes are secreted into the intraphagosome environment where they protect bacteria from host-produced O;−, H2O2 and ▪OH and inhibit host responses [8].
A role for iron in mycobacterial infections has long been suspected. In the 19th century Trousseau, a Parisian professor in clinical medicine, warned his students that iron supplementation in patients with quiescent tuberculosis (TB) could trigger clinical disease. Several studies confirmed the link between TB and iron, showing that excess in iron markedly increases mycobacterial growth in vitro, in the extra- [9] and intracellular milieu [10], while in vivo it decreases resistance and worsens the outcome in mice [11] and humans [12,13]. The association between iron overload and TB is particularly prominent in Sub-Saharan Africa, illustrated by epidemiological studies [12,13] and reviewed in [14]. African iron overload is supposedly caused by a genetic defect distinct from the HLA locus [15,16] and increased dietary iron intake predisposes to it [17]. A combination of iron overload, which impairs macrophages function, and infection with HIV/AIDS, which hinders T-cell function and causes iron deposition in different tissue, may contribute to TB susceptibility in Africa [14]. Iron overload emphasizes polymorphisms that influence iron-sensitive immune protection. For example, mice carrying susceptible variants of Nramp1, encoding unstable mRNA, succumb more easily to Mycobacterium avium infection during iron overload than resistant animals [18,19]. Nramp1 (natural resistance-associated macrophage protein), a lysosomal membrane protein, targeted to the phagosome supposedly imports iron to catalyze the production of bactericidal ▪OH [18]. A number of associations between NRAMP1 variants and TB have been reported in African populations [20–22].
Removal of iron by chelation or phlebotomy has been shown to improve the clinical outcome in a number of infectious diseases including malaria [23], hepatitis C [24] and AIDS [25]. A similar trend was reported for mycobacterial infections in vitro [26–30] and in mice [31,28,30]. The potential of iron chelation as novel adjunct therapeutic approach to enhance host effector mechanisms and inhibition of pathogen replication is reviewed in [3] and [32]. Correction of iron overload in β-2-microglobulin knockout mice with lactoferrin treatment eliminated their increased TB susceptibility [31]. In vitro studies of M. avium replication showed concentration dependent enhancement or inhibition by deferiprone (L1) and other chelating agents [29], while desferrioxamine (DFO) and N,N′bis(2-hydroxybenzyl)ethylenediamine-N,N′-diacetic acid (HBED) reduced M. avium growth intra- and extracellularly, but was ineffective in vivo [28]. In vivo low efficacy of chelating agents underlines the necessity to identify novel chelating agents or effective combinations of the existent ones. A phytosiderophore was found that inhibits the growth of M. tuberculosis H37Ra in vitro [26]. The flavonoid silybin is another iron-chelating agent from plants [33]. Silybin has diverse characteristics; it has antioxidant capacity [34,35], modulates apoptosis [36–38], is bactericidal [39], inhibits NF-κB and has anti-inflammatory properties [40]. DFO has a high affinity for iron but is lipid insoluble [41] and needs to be administered intravenously to treat iron overload. Silybin, on the otherhand, has a high affinity for ferric iron and a stability constant lower than that for other Fe(III) ligands [33] but is lypophilic and orally actine [35].
In this study, we evaluated the effect of increased levels of iron and exposure to the iron chelating agents, silybin and DFO on M. tuberculosis growth in vitro, extra- and intracellularly, and host cell viability in terms of mitochondrial functionality, membrane integrity and oxidative defence. While iron excess promoted M. tuberculosis growth in media and in monocyte-macrophages (mφ), iron-chelating agents reduced extracellular M. tuberculosis-growth but had limited and variable effects on intracellular parameters. DFO most effectively prevented effects of extracellular iron excess.
2 Materials and methods
All reagents were purchased from Sigma Chemical Company, St Louis, MO unless otherwise indicating.
2.1 M. tuberculosis cultures and treatments
The virulent strain of M. tuberculosis (H37Rv, ATCC Nos. 25618 and 27294) was obtained from the American Type Culture Collection. Frozen stock was thawed and incubated overnight in a shaking water bath at 37 °C. Mycobacteria were sonicated (Bandelin Electronic UW 2070, Berlin, Germany) for 3 cycles of 10 s at 50% power, diluted to a final concentration of 2.5 ×106 CFU ml−1 in Middlebrook 7H9 media and seeded in multi-well plates. Treatments included continuous exposure to FeSO4· 7H2O (500 µM, prepared in dH2O), DFO (500 µM, prepared in dH2O), silybin (500 µM, prepared in absolute ethanol) or iron in combination with either chelating agent in equimolar amounts (500 µM FeSO4· 7H2O mixed with 500 µM of either DFO or silybin). INH was included as a bactericidal control demonstrating growth inhibition (results not shown).
2.2 Real-time quantitative PCR (Q-PCR) to investigate M. tuberculosis growth in culture
Culture aliquots were taken on days 1, 3 and 6 of incubation following treatment, and the quantity of IS6110 DNA, 85B mRNA and 16S rRNA determined. Briefly, mycobacteria were lysed with a FastPrep FP120 cell disrupter in Blue FastRNA tubes (Bio 101, Inc., La Jolla, CA). RNA was prepared using a modified guanidine–phenol extraction method according to [42] and DNA was extracted from the same sample by back-extraction with a basic salt solution according to [43]. Reverse transcriptions (RT) of 85B mRNA and 16S rRNA were performed separately using a GeneAmp PCR System 9600 thermal cycler (Perkin–Elmer, Foster City, CA) according to [42] and [44]. Quantification of IS6110 DNA [20], 85B cDNA [42] and 16S cDNA was performed using an ABI Prism 7700 Sequence Detection System (Applied BioSystems, Perkin–Elmer, Foster City, CA). The number of molecules per sample was calculated by taking into account reverse transcription efficiency, which typically ranged from 1% to 30%. For real-time quantitative-PCR (Q-PCR) of 16S cDNA a dual labeled oligonucleotide detector probe with the sequence 5′-(FAM)-TCCACCACAAGACATGCATCCCGTG-(TAMRA)-3′, forward primer 5′-GAGTGGCGAACGGGTGAGTAACA-3′ and reverse primer 5′-CACCCCACCAACAAGCTGATAGG-3′ (Synthegen, Houston, TX) were used. PCR was performed in a 50-µl reaction mixture as described for 85B Q-PCR by [42]. The number of mycobacteria per ml culture was determined from the quantity of molecules IS6110 DNA; given that single M. tuberculosis H37Rv bacillus contains 16 copies of the IS6110 gene. The quantities of 85B mRNA, which has a low stability and short half-life, were normalized to the quantities of 16S rRNA, which are more stable and remain relatively constant, in order to investigate variation in the proportion of 85B mRNA in the mycobacteria as an indicator of viability.
2.3 Monocyte-macrophage (mφ) cultures and treatments
The premonocytic cell line, U937 (Highveld Biological, Kelvin, South Africa), was grown in RPMI1640 media as stationary suspension culture (Highveld Biological, Kelvin, South Africa) in a humidified atmosphere at 37 °C and 5% CO2. mφ cultures (1 ×105 cells ml−1) were differentiated using Vitamin D3 (10 ng ml−1, 72 h) before various treatments. Cultures were exposed to 500 µM FeSO4· 7H2O for a period of 3 h to induce a state of iron loading marked by ferritin induction [45] or exposed to 500 µM chelating agent (DFO or silybin) to chelate available iron. Chelation was achieved through the addition of 500 µM DFO or silybin to mφ for a period of 3 h. To mimic iron chelation during iron overload, equimolar quantities of iron and chelating agent were added to mφ during 3 h. Extracellular iron or chelating agent were washed off with PBS and intracellular iron levels were quantified using the Sigma Iron and Total iron-binding capacity assays (Procedure No. 565). Briefly, cells were pelleted and digested during 16 h with pepsin (1 mg ml−1) in water acidified with 100 µl 50 mM HCl. After neutralization with 133 mM NaOH, iron levels were determined spectrophotometrically compared to standards. For the infection of mφ, mycobacteria were opsonized in 10% autologous human plasma under agitation at 37 °C for 30 min, washed with PBS and resuspended in RPMI media. mφ were exposed to mycobacteria at mφ to bacillus ratio of 1:1 for 3 h, washed with PBS, and allowed to recover in fresh RPMI media.
2.4 Intracellular mycobacterial viability and replication
The viability and replication of intracellular mycobacteria were detected using an adaption of the microplate Alamar Blue assay (MABA) [46], which detects colorimetric changes due to the oxidation and reduction of the dye. The low inoculation ratio used (1:1 mφ to bacilli ratio) and the minimum number of bacilli required monitoring metabolic activity using MABA, needed 6–7 days incubation of M. tuberculosis recovered from mφ before performing the MABA. A similar incubation period was used for MABA to determine susceptibility to antibiotics [46]. mφ cultures were harvested using trypsin-free EDTA detachment media (Highveld Biologicals, Kelvin, South Africa) and lysed by freeze–thaw cycles after the addition of sterile distilled H2O. Bovine serum albumin (Boehringer Mannheim, Darmstadt, Germany) in PBS (20%) was added to the cell lysate followed by centrifugation (21 194g, 10 min). The pellet was washed, suspended in Middlebrook 7H9 media and sonicated for 10 s before seeding in multiwell plates (200 µl well−1). Middlebrook 7H9 media and 100% reduced AlamarBlue™ (Serotec, Oxford, UK) served as controls. Mycobacteria were cultured at 37 °C and 5% CO2 for an additional seven days to obtain sufficient numbers of mycobacteria before the addition of AlamarBlue™ (25 µl) and Tween 80 (25 µl, Merck, Darmstadt, Germany). Fluorescence was quantified 4 h after the addition of AlamarBlue™ at an excitation wavelength of 544 nm and an emission wavelength of 590 nm (Fluoroskan Ascent, Labsystems, Helsinki, Finland).
2.5 Mitochondrial membrane potential (MMP)
Mitochondrial activity was assessed using MitoTracker Red CMXRos (Molecular Probes, Leiden, Netherlands), a derivative of the red fluorescent X-Rosamine that accumulates in active mitochondria. Seven days after infection, cells were detached, washed and incubated in 250 nM MitoTracker Red (30 min, 37 °C, 5% CO2). Cells were fixed in paraformaldehyde (1%) for 10 min at room temperature, and washed with PBS. MMP was quantified using a Coulter Altra flow cytometer (Coulter, Miami, FL).
2.6 Phosphatidylserine (PS) externalization
Phosphatidylserine (PS) externalization was used as a measure of general cell death without distinguishing apoptosis and necrosis commonly detected with Annexin-PI assays. Fixation, required to comply with safety requirements, nullified the use of PI staining. PS externalization was quantified flow cytometrically through Annexin V binding (Beckton Dickinson, PharMingen, San Jose, CA). Detached cells were washed and incubated in 1 ×binding buffer (95 µl) and Annexin V-Biotin (5 µl) at room temperature (15 min). After washing with 1 ×binding buffer, cells were fixed with cold absolute methanol on ice, washed and incubated in a 1:50 dilution Streptavidin-PerCP (Becton Dickinson PharMingen, San Jose, CA) in the dark (30 min, room temperature). Cells were washed and analyzed using flow cytometry (Coulter Altra Flow Cytometer, Coulter, Miami, FL).
2.7 Super oxide (O;−) production
Extracellular O;− generated by mφ was measured directly after exposure to M. tuberculosis. A 100-µl aliquot of harvested cells, suspended in PBS, was incubated (45 min, 37 °C, 5% CO2) in a solution containing 700 µl buffer solution (PBS with 1 mM EDTA and 0.4 mM NaHCO3) containing 1 M cytochrome c (ICN, Aurora, OH) and 30,000 U superoxide dismutase (SOD, Boehringer Mannheim, Darmstadt, Germany). Cell suspensions were centrifuged (12,000g, 5 min) and the absorbance of the supernatants quantified at 550 nm on a Multiskan MS (Labsystems, Helsinki, Finland) against a reference sample for which SOD was omitted.
2.8 Statistical analysis
The least significant difference (LSD) values were calculated from the pooled variance (CoHort Software, Berkeley, CA, 1990). Differences in mean values were considered significant if the LSD (p < 0.05) value was exceeded.
3 Results
An overview of the results obtained in this study is given in Table 1.
3.1 Mycobacterial growth in culture
Real-time Q-PCR has a high sensitivity and was therefore the method of choice to assess mycobacterial numbers and viability. Quantifying M. tuberculosis IS6110 DNA provided a means of enumerating the mycobacteria (Fig. 1A), a technique that compared favorably with CFU counts [44], whereas quantifying M. tuberculosis 85B mRNA served as an indicator of M. tuberculosis viability [42] (Fig. 1B). Messenger RNA is unstable and has a short half-life. When quantities are normalized to that of 16S rRNA, which is more stable and remains relatively constant, the variation in the proportion of 85B mRNA serves as indicator of viability. The overall trend observed with Q-PCR analysis showed that iron excess significantly enhanced the number and viability of M. tuberculosis at both, day 3 and 6 of incubation, compared to the unsupplemented control (Table 1, Figs. 1 A and B) (p < 0.05). Silybin significantly reduced the number of M. tuberculosis at day 1 and 6 compared to the unsupplemented control (Fig. 1A), without affecting viability significantly. DFO significantly reduced the number (Fig. 1A) and viability (Fig. 1B) of M. tuberculosis in culture compared to the unsupplemented control at day 3 and 6. Compared to the unsupplemented control, iron plus silybin significantly increased the number of M. tuberculosis in culture at day 3, reducing it at day 6 (Fig. 1A), while it had the opposite effect on M. tuberculosis viability; i.e., decreasing it at day 3 and increasing it at day 6. Compared to the unsupplemented control, iron plus DFO significantly increased the number of M. tuberculosis in culture at day 3, without any other significant effects on number or viability.
An overview of the effects of excess iron and iron chelation on Mycobacterium tuberculosis (M. tuberculosis) growth and the integrity and effector functions of M. tuberculosis-infected monocyte-macrophages (mφ)
M. tuberculosis (H37Rv) and mφ were treated with iron (FeSO4·7H2O), the chelating agents, silybin and DFO, iron plus silybin or iron plus DFO. Extra- and intracellular number and viability of the M. tuberculosis strain, H37Rv, served as markers of mycobacterial growth, while mitochondrial membrane potential (MMP) and phosphatidylserine (PS) externalization reflected host cell integrity and superoxide (O;−) production an effector function.
Arrows indicate significant increase/decrease, those in italic font show trends of borderline significance, and ‘–’ no influence.
An overview of the effects of excess iron and iron chelation on Mycobacterium tuberculosis (M. tuberculosis) growth and the integrity and effector functions of M. tuberculosis-infected monocyte-macrophages (mφ)
M. tuberculosis (H37Rv) and mφ were treated with iron (FeSO4·7H2O), the chelating agents, silybin and DFO, iron plus silybin or iron plus DFO. Extra- and intracellular number and viability of the M. tuberculosis strain, H37Rv, served as markers of mycobacterial growth, while mitochondrial membrane potential (MMP) and phosphatidylserine (PS) externalization reflected host cell integrity and superoxide (O;−) production an effector function.
Arrows indicate significant increase/decrease, those in italic font show trends of borderline significance, and ‘–’ no influence.
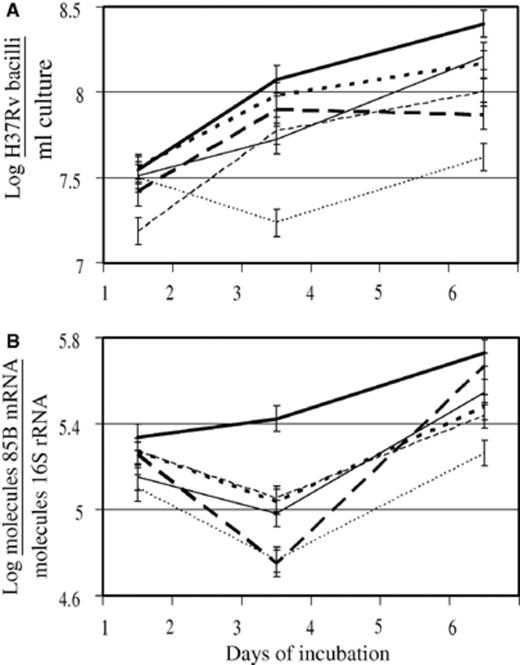
Excess iron enhanced, while iron chelating agents reduced the number (A) and viability (B) of H37Rv in culture, as detected by real time quantitative-PCR (Q-PCR). Mycobacteria were cultured under normal, unsupplemented conditions (′) or treated with iron (500 µM FeSO4· 7H2O; ), silybin (500 µM; — - -), desferrioxamine (DFO, 500 µM; …), iron plus silybin (
) or iron plus DFO (
) and aliquots taken on days 1, 3 and 6 of incubation. Following extraction of DNA and RNA and RT-PCR of 85B mRNA and 16S rRNA, IS6110 DNA, 85B cDNA and 16S cDNA were quantified by the TaqMan assay. IS6110 DNA quantities were used to calculate mycobacterial number (A). Quantities of 85B mRNA, which is unstable and has a short half-life were normalized to the quantities of 16S rRNA, which is more stable and remains relatively constant, in order to investigate variation in the proportion of 85B mRNA in the mycobacteria as an indicator of viability (B). Results represent the mean of two experiments performed in duplicate on days 1, 3 and 6 of incubation. Error bars represent the least significant difference (LSD) calculated from the pooled variance (n= 4; p < 0.05).
3.2 Intracellular M. tuberculosis growth
A 3-h incubation of mφ in the presence of 500 µM FeSO4· 7H2O caused on average a 50-times increase in intracellular iron concentration from 0.05 to 2.5 µM as determined using the Sigma Iron and Total iron binding kit (n= 6; p < 0.05). Phagocytosis efficiency was visually monitored through microscopy and estimated to be on average 1% despite various treatments. Iron excess increased the viability of H37Rv recovered from iron-loaded mφ 2.3-times (p < 0.05) compared to those recovered from unsupplemented, H37Rv-infected cells (Table 1, Fig. 2). Silybin showed a trend towards inhibition of mycobacterial viability but neither silybin nor DFO significantly reduced intracellular mycobacterial viability (Fig. 2). Similarly, intracellular M. tuberculosis viability was not affected by iron plus DFO, compared to unsupplemented controls. In contrast, iron plus silybin significantly enhanced (p < 0.05) the viability of recovered H37Rv (>2.0-times) as compared to unsupplemented controls (Fig. 2).
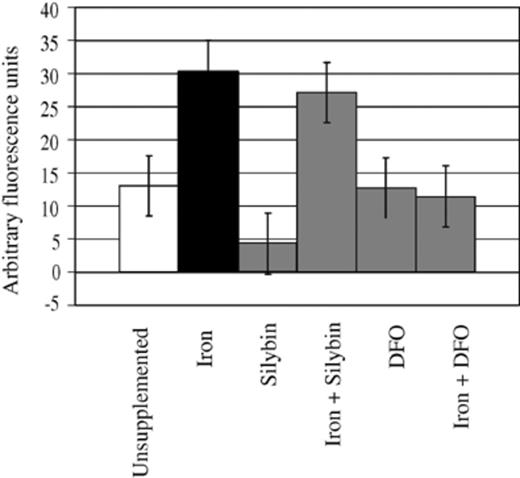
Iron or iron plus silybin supplementation enhanced the viability of H37Rv recovered from monocyte-macrophages (mφ), while silybin, alone, showed borderline suppression and DFO or iron plus DFO had no influence. mφ were incubated in unsupplemented media or media supplemented with iron (500 µM FeSO4· 7H2O), silybin (500 µM), DFO (500 µM), iron plus silybin or iron plus DFO for 3 h. H37Rv was added (1:1 mφ to bacilli ratio) and cultures incubated for another 3 h. mφ were washed and cultured in fresh media for 7 days. mφ were lysed and mycobacteria recovered and cultured in Middlebrook 7H9 media for 7 days before metabolic activity was assayed by the microplate AlamarBlue™ assay (MABA). Results represent the mean of three experiments. Error bars represent the least significant difference (LSD) value calculated from the pooled variance (n= 5; p < 0.05).
3.3 Host cell viability
MMP and PS externalization were used as indicators of host cell viability. MMP in H37Rv-infected mφ was significantly reduced by iron, silybin and DFO (p < 0.05), as well as iron plus silybin compared to unsupplemented, infected cells (Table 1, Fig. 3A). Iron plus DFO did not influence the MMP of infected mφ compared to unsupplemented, infected cells. The contribution of intracellular M. tuberculosis to the measurement of host cell MMP was determined to be non-significant (data not shown). Silybin significantly increased PS exposure (p < 0.05) in H37Rv-infected cells, while iron, DFO and iron plus silybin or DFO did not alter PS externalization when compared to unsupplemented, infected cells (Table 1, Fig. 3B). Unsupplemented, H37Rv-infected cells together with those treated with iron, DFO and iron plus silybin or DFO, showed PS exposures similar to unsupplemented, uninfected cells (data not shown) (p < 0.05).
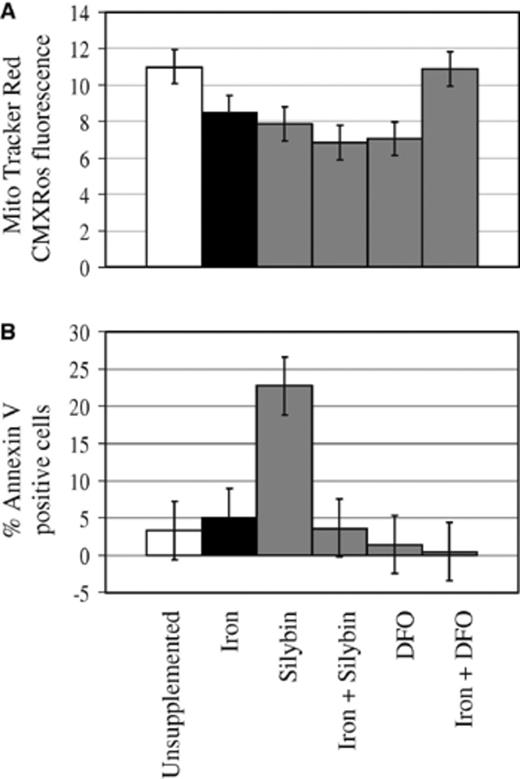
Excess iron, iron chelating agents and iron plus silybin reduced mitochondrial membrane potential (MMP) (A), while silybin increased phosphatidylserine (PS) externalization of H37Rv-infected monocyte-macrophages (mφ) (B). mφ were incubated in unsupplemented media or media supplemented with iron (500 µM FeSO4· 7H2O), silybin (500 µM), DFO (500 µM), iron plus silybin or iron plus DFO for 3 h. H37Rv was added (1:1 mφ to bacilli ratio) and cultures incubated for another 3 h. mφ were washed, cultured in fresh media for 7 days and viability measured in terms of MMP based on MitoTracker Red CMXRos accumulation and cell membrane integrity reflected by PS externalization as detected by Annexin V binding. Results represent the mean of three experiments performed in duplicate. Error bars represent the least significant difference (LSD) value calculated from the pooled variance (n= 6; p < 0.05).
3.4 O;− production
Macrophages produce O;− through the NADPH oxidase complex upon activation as an early defence mechanism. The oxidative burst was used as an indicator of host cell effector function. Prior treatment of mφ with iron, silybin and iron plus silybin, significantly reduced mφ (O;−) production (p < 0.05) upon infection, while DFO, alone or plus iron had no significant influence on O;− levels compared to unsupplemented, H37Rv-infected cells (Table 1, Fig. 4).
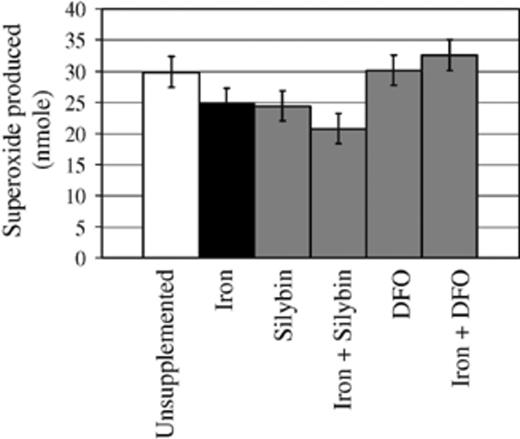
Iron, silybin and iron plus silybin supplementation reduced superoxide O;− production by monocyte-macrophages (mφ) after infection with H37Rv, while DFO, alone or plus iron, had no influence. mφ were incubated in unsupplemented media or media supplemented with iron (500 µM FeSO4· 7H2O), silybin (500 µM), DFO (500 µM), iron plus silybin or iron plus DFO for 3 h. H37Rv was added (1:1 mφ to bacilli ratio) and cultures were incubated for another 3 h before O;− levels were quantified. Results represent the mean of three experiments performed in duplicate. Error bars represent the least significant difference (LSD) calculated from the pooled variance (n= 6; p < 0.05).
4 Discussion
This study investigated the effect of iron excess and iron chelation on the replication and viability of H37Rv M. tuberculosis, extra- and intracellularly, and on host cell survival and oxidative defence during infection. An overview of the results obtained is given in Table 1. Extracellular H37Rv showed a significant increase in number and viability in the presence of excess iron and, by large, a significant decrease in number and viability in the presence of the iron chelating agents, silybin and DFO, compared to cultivation without supplementation. Excess iron or chelating agents reduced viability of host cells based on MMP, while iron and silybin, alone or in combination, compromised O;− production. DFO had little influence on intracellular parameters, but most consistently prevented effects of excess iron, while silybin significantly changed most intracellular parameters, while it mostly failed to prevent effects of excess iron.
Based on the abundance of nucleic acids, excess iron enhanced numbers and viability of extracellular H37Rv, while silybin and DFO, alone, mostly reduced extracellular numbers and viability (Table 1, Fig. 1). These results support the recognized role of iron in mycobacterial growth and virulence in culture [5,9] and the suppression thereof when sequestered [26,28]. DFO given together with excess iron largely prevented the growth-promoting effects of iron (Table 1, Fig. 1), supporting its efficacy as chelating agent, preventing the uptake of iron. In contrast, silybin given with excess iron had significant, opposite and time-dependent effects on number and viability of extracellular M. tuberculosis. Viability increasing over time at the cost of numbers in the presence of iron and silybin, possibly relates to a combination of lower stability of the silybin–iron complexes [33] compared to DFO–iron complexes and bactericidal activity [39]. The potential benefit of reducing iron availability, however, needs to be evaluated in the context of the host-pathogen milieu since iron deficiency in the host is just as detrimental as iron overload [2].
Iron enhanced the viability of H37Rv in mφ compared to unsupplemented controls. This was prevented when iron was given together with DFO but not with silybin, while neither silybin nor DFO alone influenced viability of M. tuberculosis significantly, (Table 1, Fig. 2). The enhancement of M. tuberculosis intracellular viability by iron is in agreement with the observed extracellular effect (Fig. 1), relates to similar findings for growth in vitro [9], intracellular [10] and in vivo [11] and agrees with the importance of iron in M. tuberculosis virulence [3,4,6]. Silybin, alone, decreased M. tuberculosis viability at borderline significance compared to DFO that had no effect, which may relate to better intracellular access as lypophilic chelating agent [33] and bactericidal activity [39]. Silybin's chelation ability appears limited, in contrast to DFO, since it did not reduce excess iron-enhanced intracellular viability of M. tuberculosis. The difference in the ability of silybin and DFO to counteract the effects of excess iron on M. tuberculosis viability (Fig. 2, Iron + Silybin vs. Iron + DFO) may reflect the high affinity of DFO for iron (1031), while iron plus silybin, which did not differ from iron alone, suggested a low iron affinity, availability of silybin-bound iron for mycobacterial metabolism, similar to holotransferrin that enhances M. avium growth [27], or toxic iron complexes hampering host responses [23]. Besides potentially host-‘friendly’ characteristics, silybin has traits, which could favor mycobacterial viability, such as antioxidant capacity [34,35] and the ability to inhibit NF-κB [40]. Decreased binding of NF-κB to the iNOS promoter region could reduce NO production and thereby increase M. tuberculosis viability [47]. Differences between silybin and DFO may also relate to concentration dependent effects of different chelating agents as observed by Douvas et al. [29]. The observation for DFO plus iron is in agreement with DFO inhibition of intramacrophagic growth of M. avium[28], while lack of effect, on its own, likely results from its limited membrane permeability [41].
Supplementation with iron and/or silybin as well as DFO significantly reduced MMP in H37Rv-infected mφ, compared to H37Rv-infected mφ grown in unsupplemented media (Fig. 3A), which in itself had a significantly reduced MMP compared to uninfected, unsupplemented cells that showed a MitoTracker Red CMXRos fluorescence of approximately 14 (Results not shown). Thus, infection with H37Rv significantly reduced mitochondrial activity, which was further reduced by iron loading or deprivation. MMP is a key indicator of mitochondrial activity, which in turn controls cell survival or death by apoptosis or necrosis, depending on the MMP status. Apoptosis is a defence mechanism of macrophages against mycobacterial infection and is prevented by H37Rv as a virulence strategy [48]. Radical metabolism is partner to energy metabolism in mitochondria and modulation of iron homeostasis may influence mitochondrial activity [49]. Excess iron facilitates hydroxyl radical production and oxidative injury, while limited iron may hamper heam–protein function which compromises mitochondrial function. The critical balance required for iron homeostasis in mitochondrial activity makes deviations from the norm intolerable and may favor mycobacterial growth. MMP was the only intracellular parameter influenced by DFO, which may relate to the high sensitivity of MMP to reflect early compromises in cell viability. Iron plus silybin, in contrast to iron plus DFO, did not abrogate the effects of iron on MMP. This corresponds with the iron plus silybin-related increase in intracellular viability of M. tuberculosis (Table 1, Fig. 2) and may relate to the same factors as described above. As with intracellular M. tuberculosis viability, DFO was able to neutralize the effects of excess iron on MMP.
Unsupplemented controls, iron, DFO and iron in combination with silybin or DFO showed similar levels of Annexin V binding (Table 1, Fig. 3B), corresponding to that of unsupplemented, uninfected cells (data not shown). This observation supports cellular well-being and the lack of cell death, in particular apoptosis for which Annexin V is often used as a marker. In our hands, H37Ra, known to induce apoptosis in host cells [50,51], gave approximately 12% Annexin V positive cells (data not shown). Close to 23% Annexin V positive cells were observed for silybin-supplemented, H37Rv-infected cultures (Fig. 3B). Since iron supplementation given together with silybin showed no effect on Annexin V binding (Fig. 3B, Iron + Silybin), induction of PS exposure is likely regulated through a chelation-mediated mechanism. High levels of Annexin V binding may also reflect destruction of the cell membrane in necrotic cells. However, silybin has been shown to induce apoptosis in various cell types [36–38]. From the data available, it is difficult to know whether the increase of Annexin V positive cells in silybin-supplemented, H37Rv-infected cultures indicates apoptosis or necrosis. Two observations may argue against necrosis. Firstly, MMP levels, that are normally lower in necrotic cells than apoptotic cells, are not significantly different between iron, silybin and DFO (Fig. 3A), while iron and DFO treated cells, as discussed above, do not appear to be necrotic, based on Annexin V binding and compare to unsupplemented, H37Rv-infected cells (Fig. 3B) and unsupplemented, uninfected cells (data not shown). Secondly, silybin almost significantly reduced viability of M. tuberculosis intracellularly (Fig. 2), an observation that is in agreement with the reported effect of apoptosis on intracellular mycobacteria [50]. Furthermore, the antioxidant capacity of silybin [34,35] may ensure ROS levels sufficient to signal apoptosis, but insufficient to promote oxidative injury and necrosis. Considering the retainment of cell membrane symmetry by the iron plus silybin treatment together with the increased viability of intracellular M. tuberculosis after iron plus silybin treatment (Fig. 2), and the decreased MMP (Fig. 3A), it might suggest that M. tuberculosis-related suppression of apoptosis is supporting mycobacterial growth in an environment where silybin reduces NO and O;− production (Fig. 4) through its NF-κB inhibiting [40] and anti-oxidant properties [34,35], respectively. The inability of DFO, alone or in combination with iron, to influence PS exposure confirms its limited intracellular access and supports intracellular signaling of membrane PS exposure.
Unsupplemented, H37Rv-infected cells showed no induction of an oxidative burst (Fig. 4) compared to unsupplemented, uninfected cells (data not shown). This is in contrast with the induction of NADPH oxidase during infection of mφ with the attenuated H37Ra strain, supported by the literature and results from our laboratory. Iron and silybin, alone or combined suppressed constitutive levels of O;−. Enhanced viability of M. tuberculosis supported by iron (Fig. 2, iron and iron + silybin) may contribute to the significant suppression of O;− in host cells treated under the same conditions (Fig. 4). Large quantities of iron-cofactored SOD secreted by M. tuberculosis may decrease host O;− levels in iron-loaded, H37Rv-infected mφ[8]. Silybin is a diverse molecule with anti-oxidant properties [34,35] and the ability to inhibit NF-κB [40], besides chelating iron [33]. Our results support the anti-oxidant capacity of silybin [34,35], decreasing O;− levels most likely through inhibition of NADPH oxidase [37] (Fig. 4, Silybin vs. Unsupplemented). DFO, alone or in combination with iron did not influence O;− levels. The observation that DFO, alone, does not interfere with constitutive O;− levels is difficult to explain in the light of the suppression of mitochondrial activity by DFO (Fig. 3A), the key basal producer of oxidants. The lack of influence of DFO is again most likely due to the difficulty with which it crosses cell membranes, while unaltered levels of O;− with iron + DFO support effective chelation of extracellular iron.
Results described in this paper present a case for iron chelation as a novel adjunct therapy for iron-burdened individuals with TB or prophylaxis for those at risk of infection or activation of latent M. tuberculosis infection. The co-occurrence of TB, HIV, iron overload and possible genetic predispositions in populations from Sub-Sahara Africa make individuals from this region particularly tailored for the proposed therapeutic approach. Silybin showed characteristics with potential but DFO was more effective as chelating agent than silybin though it had limited intracellular access. Future studies need to identify more appropriate candidates for iron chelation therapy or combination of existent chelating agents that could effectively sequester extra- and intracellular iron without jeopardizing host cell viability and defence. Knowledge on possible interactions between chelating agents and the current TB drug arsenal is also essential.
Acknowledgements
This research was supported by the National Research Foundation (NRF) and the Medical Research Council (MRC) of South Africa. The work on quantitative PCR was supported by the Tuberculosis Prevention and Control Research Unit (NIH contract N01-AI-95383). We thank Shima Patel for her technical assistance with the quantitative PCR assays.
References