-
PDF
- Split View
-
Views
-
Cite
Cite
Tirza Doniger, Jonathan M Adams, Eugene Marais, Gillian Maggs-Kölling, Chen Sherman, Dorsaf Kerfahi, Ying Yang, Yosef Steinberger, The ‘fertile island effect’ of Welwitschia plants on soil microbiota is influenced by plant gender, FEMS Microbiology Ecology, Volume 96, Issue 11, November 2020, fiaa186, https://doi.org/10.1093/femsec/fiaa186
- Share Icon Share
ABSTRACT
Desert and semi-desert plants are often associated with a distinct soil biota under the plants and close to the root system. We aimed to understand if similar effects could be found in the taxonomically isolated desert gymnosperm Welwitschia mirabilis in the Namib Desert, and whether this island effect varied with climate and with gender of plants. We took soil cores adjacent to the plants in environments ranging from extreme desert to arid shrubland, and in nearby control sites between the plants. Soil chemistry was analysed, and deoxyribonucleic acid was extracted and sequenced for the bacterial 16s region. Soil under the plants was richer in organic C, N and moisture. Despite the range of climates, the soil around Welwitschia plants was consistently associated with a particular bacterial community composition that was distinct from samples further away. Compared to unvegetated control patches, bacterial diversity close to the plants was reduced. In the plant-associated soil community, there was a clear gender effect across all sites with a distinct community composition and greater diversity under male plants. It is unclear what differences in the soil environment might be producing these gender-associated differences, which provide an additional dimension to the fertile island effect.
INTRODUCTION
In semi-arid environments, the area around plants in semi-arid and arid environments has often been noted as having enhanced soil biotic activity (Sarig, Barness and Steinberger 1994; Callaway 2007; Pen-Mouratov et al. 2010; Rodríguez-Echeverría et al. 2013; Sherman et al. 2019). Plants have been found to influence soil properties in various ways: through input of organic carbon, nitrogen and other nutrients from their litter, root system and fruit and by influencing temperature, soil moisture (SM) content and salinity (Van der Putten et al. 2013; Sherman et al. 2019). This ‘fertile island’ effect in such environments may be associated with increased abundances of fungi, bacteria and small soil animals, and increased respiration and cycling of nutrients (Van der Putten et al. 2013; Sherman et al. 2019).
While the fertile island effect on soil biota is well-established in arid and semi-arid environments (Schlesinger and Pilmanis 1998; Liu and Li 2018; Sherman et al. 2019), it is not clear how this manifests itself in very arid conditions, and how it varies along rainfall gradients (Garner and Steinberger 1989; Stock, Dlamini and Cowling 1999). The earlier study by Sherman et al. (2019) found a strong fertile island effect of the angiosperm Acanthosicyos horridus in the Namib, in terms of microbial biomass and respiration activity.
Our study here was based around Welwitschia mirabilis (Gnetales: Welwitschiaceae), which is a plant endemic to the central and northern Namib Desert along the South Atlantic coast of Namibia and Angola (Fig. 1). It is a gymnosperm with only two broad, strap like leaves that emerge along the margin of a corky stem and grow continuously throughout the year (Rodin 1958; Bornman 1977; von Willert 1993), and a root system dominated by a single large tap root. The plants may reach as much as meter across and possibly live for hundreds of years (Henschel and Seely 2000; Henschel et al. 2019). Welwitschia transpires 0.2–1.4 L of water daily per square metre of leaf (Von Willert and Wagner‐Douglas 1994) and primarily uses the C3 photosynthetic pathway (Von Willert et al. 2005). The extensive fine root system at 5–40 cm depth and absence of very deep roots suggests that plants rely on soil water at relatively shallow depth rather than deep groundwater sources (Kers 1967; Olivier 1995). Throughout the range of Welwitschia, these evergreen, broadleaved, low-growing plants are of considerable importance as they provide rare shade, food, refuge and possibly water to many animal species. This is particularly so where Welwitschia forms the dominant perennial vegetation, as in parts of the Namib Desert with <50 mm mean annual precipitation (MAP) and on gravel fans or gravelly stream beds of ephemeral drainage systems (Seely 1990; Henschel and Seely 2000).
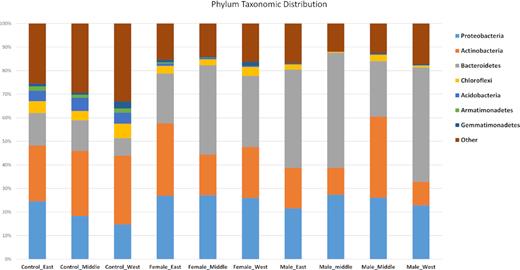
Relative abundance of reads of bacterial phyla. ‘Control’ sites are from bare areas, ‘female’ are from under the leaves of female Welwitschia plants, ‘male’ are from under the leaves of male plants.
Here, we set out to investigate in greater detail whether there is a consistent detectable fertile island effect in the soil biota associated with Welwitschia at different points along an aridity gradient in the Namib Desert. Previous studies of soil chemistry found that soils close to Welwitschia plants are richer in nutrients and carbon (Abrams et al. 1997; Henschel and Seely 2000; Mugunga and Mugumo 2013) than soil from nearby barren areas, presumably as a result of steady enrichment by root exudates and decaying leaf material from the plants, although those authors did not study how soil biota might be affected. In an earlier study, Valverde et al. (Valverde et al. 2016) found that the rhizosphere of the Welwitschia has distinctive and diverse communities of bacteria and fungi, compared to the bulk soil 20–30 cm away from the roots. The immediate rhizosphere was enriched in certain commonly mutualistic groups of N fixers, and arbuscular mycorrhiza fungi also occurred. While their work was an important pioneering study of the Welwitschia microbiome, it is important to bear in mind that Valverde et al. sampled only one site, and the bulk (non-rhizosphere) soil they sampled as a control was essentially still under or very close to the plants themselves.
We were also interested in whether the gender of Welwitschia, a dioecious plant, affects the composition of the soil and its bacterial community. About 6% of plant species are dioecious, but there has been little study of how this trait affects the plant and soil microbiome. Wei and Ashmann (2018) found that dioecious wild strawberry plants have gender-specific differences in plant microbiome. Studying a rainforest tree, Rhoades, Sanford and Clark (1994) found increased soil phosphorus under female trees, compared to male trees. Rhoades et al. attributed this to cycling of decomposed fruit back into the soil ecosystem. We also found a gender effect of plants in Sherman et al. (2019) for A. horridus, with greater microbial biomass and activity under female plants—again possibly due to decomposed fruits. Abrams et al. (1997) also found difference in soil properties beneath male and female Welwitschia plants, although there was no investigation of soil biota.
Our main hypothesis was (i) that the area under the leaves and near to the roots of the Welwitschia plants would have a distinct soil biota, dominated by more copiotrophic bacterial groups, with presence of possible mutualistic genera, and much greater connectivity within the bacterial community, than the barren areas between the plants. We also hypothesized (ii) that the plant-associated bacterial community would be fairly constant between sites that had different climates, and much more so than the community associated with unvegetated areas, due to the consistent environment offered by the plant, and (iii) that there would be differences in bacterial community associated with plant gender (a result of possible differences in growth or exudate activity), extending the earlier results on gender-related differences in soil chemistry under Welwitschia found by Abrams et al. (1997). While the microbiome of Welwitschia was investigated by (Valverde et al. 2016), there was no attention paid to possibility of plant gender-related effects.
METHODS
Study region
The Namib Desert in South-western Africa (see map in Fig. S1, Supporting Information) comprises both arid and hyper-arid zones. Rain comes from summer monsoons, but is limited and temporally and spatially unpredictable (Eckardt et al. 2013). Rainfall near the coast is highly variable, unpredictable and rare, but the incidence and the amount of rain increase progressively with distance from the coast (Hachfeld 2000). Mean annual rainfall is inversely related to the occurrence of fog, which is a major source of moisture for plants (Hachfeld 2000). Moisture deposition from fog is more significant towards the coast, but the occurrence and potential of moisture from fog decreases rapidly with distance from the coast to the interior (Hachfeld 2000; Eckardt et al. 2013). Mean annual temperatures vary spatially (Lancaster, Lancaster and Seely 1984), with less variation in mean daily temperatures near the coast (15.7 ± 3.2°C) than 100 km further into the interior (21.9 ± 10.8°C) where seasonal temperature differences are more pronounced.
Sampling sites
In April 2015, we sampled from Welwitschia populations at three different sites in the Central Namib Desert (Fig. S2, Supporting Information, map of site locations). All the sites are gently inclined (<20% slope) desert/semi-desert surface gravel outwash fans, but are situated in very different climates. All the sampling sites were located along the ephemeral Messum River between the Brandberg, Namibia's highest mountain, and the South Atlantic coast (Fig. S1, Supporting Information), which represents a climatic and altitudinal gradient from cool, coastal conditions with very little rainfall to hot interior with seasonal temperature and rainfall variables. The underlying geology of the area consists of Jurassic-age volcanic rocks of the Etendeka Group, comprising diverse basalt, quartz latite and latite types.
The first sampling site was 14 km from the ocean at latitude 21°24.659′ S, longitude 13°56.929′ E at 167 m above sea level (asl) with a mean multiannual precipitation of 17 mm. The plants were located in a shallow outwash gravel fan on the gypsiferous plains west of the Messum Crater, considered as hyperarid desert as the mean annual rainfall in this area is very low, although fog moisture can have a significant ecophysiological impact (Henschel and Seely 2008) in the coastal zone (Juergens et al. 2013). Welwitschia mirabilis was the dominant perennial (Fig. S1, Supporting Information).
The second sampling site was around 46 km from the coast, to the east of the Messum Crater (21°19.881′ S, 14°15.309′ E; 468 m asl) with a mean multiannual rainfall of 60 mm. Large Welwitschia plants were growing on gravel spits within the dry riverbed of the Messum River as well as on the banks outside washes. The distance from the coast suggest that fog may still regularly occur (Hachfeld 2000), supplemented more frequently by rain.
Our third sampling site along the gradient was some 70 km from the coast at the base of the Brandberg (21°15.274′S, 14°28.653′E; 648 m asl), with a mean multiannual rainfall of 110 mm. Scattered W. mirabilis of various sizes were growing among open perennial vegetation on the petrocalcic soils in this area, within gravel fans/outwashes and drainage systems but also widespread on the plains. The surrounding vegetation consisted of a considerable variety of grass, shrub, tree and herbaceous species, along with unvegetated areas, with various Stipagrostis, Commiphora and Acacia species as would typically be considered part of the Arid Savanna Biome (Irish 1994).
At each site, we collected sets of soil samples in the vicinity of randomly selected plants of varying sizes that were growing on gravel fans or other stable gravelly surfaces. For each site, three soil samples were collected beneath male plants, three below female plants and three in barren control areas. Within each site, we sampled neighbouring pairs of male and female plants, to avoid spatial clustering by gender. Each sample was in fact combined from four subsamples collected from cores of 5 cm diameter of the upper 0–10 cm of the soil that was covered by leaves of either a male or a female W. mirabilis plant, with each core approximately 10 cm from the main tap root and reaching to the zone of horizontal roots that emerge from the tap root. In the case of the controls, we took four subsamples from open and completely unvegetated spaces (also on the same or similar gravel areas) between the plants that were at least 40 m from any Welwitschia. The different samples of bare areas were spaced at least a 100 m apart. We observed varying quantities of organic debris on the soil surface at all the Welwitschia plants, but did not observe appreciable leaf or other organic debris underneath any of the other species of plants at the sites.
Each soil sample was placed in an individual plastic bag and transported to the laboratory in a cooler to prevent overheating.
Soil analysis for each sample was carried out as follows: SM content was determined gravimetrically by drying soil samples for 72 h at 105°C. Organic matter content was determined by igniting samples for 8 h at 490°C. Soil pH was measured with a glass electrode using a 1:2 soil:water ratio. Conductivity was determined by measuring electrical conductivity in a soil–water suspension (soil:double distilled water = 2:5) using an autoranging EC/temp meter (TH2400, EI-Hamma). Nitrogen was determined by using the modified Kjeldahl method, ISO 11261:1995 (E) N mg/kg. Soil physiochemical data is presented in Table S1 (Supporting Information).
Deoxyribonucleic acid (DNA) was extracted from 0.5 g soil using an Exgene soil SV kit from GeneAll (Songpa-gu, Korea), according to manufacturer's instructions. DNA concentration was measured on a Thermo Scientific NanoDrop™ 1000 Spectrophotometer (Waltham, MA).
Polymerase chain reaction and agarose gel verification
Bacterial community analysis was carried out using a 16s rRNA gene primer for the V1-V2 hypervariable region of bacterial 16s rDNA. Initially, the bacterial 16s rDNA was amplified using the standard primers, 27F (5′-AGAGTTTGATCCTGGCTCAG-3′) and 1100R (5′-GGGTTNCGNTCGTTG-3′) (Turner et al. 1999). This was followed by nested amplification using the same forward primer but barcoded at the 5′ end, and the 338R reverse primer (5′-TGCTGCCTCCCGTAGGAGT-3′) (Amann, Ludwig and Schleifer 1995). Polymerase chain reaction was carried out on an Applied Biosystems Veriti™ 96-Well Thermal Cycler as described in our recent publication (Unc et al. 2019).
Sequencing was performed at the Bar-Ilan University sequencing facility using the Ion Torrent Personal Genome Machine (PGM, Life Technologies). Sequences were processed and filtered, and operational taxonomic units (OTUs) were generated at 97% similarity using Usearch v9.0 (Edgar 2010). We picked a representative sequence for each OTU and used the RDP classifier (Wang et al. 2007) in QIIME, version 1.7.0 (Caporaso et al. 2010), with the GreenGenes_13_8 (DeSantis et al. 2006) reference database to annotate taxonomic information for each representative sequence. For those sequences that we were unable to classify using the GreenGenes database, we used the Silva v132 (Quast et al. 2013) database.
Statistical analysis
All statistical analyses were conducted in R v 3.6.1 (R Core Team 2019 2019) using the vegan v2.5–6 (Oksanen et al. 2013), and Ampvis2 (Andersen et al. 2018) R packages. A permutational multivariate analysis of variance (PERMANOVA) was performed with 999 permutations using the Adonis function in Vegan R package to test which parameter has a significant effect on bacterial community composition. Redundancy analysis was performed in VEGAN using the significant environmental variables from the PERMANOVA (< = 0.01) as explanatory variables. The analysis of the Shannon-Diversity index was performed using the Ampvis2 package and significance was determined using the Wilcoxon Rank Sum test in R. Using the Ampvis2 package, the constrained principal component analysis was performed using the Hellinger transformation and constrained to plant gender. Plots were generated with the ggplot2 R package.
Network analysis
To understand the co-occurrence patterns of OTUs in different localities, we performed network analysis (Faust and Raes 2012). To make a clear interpretation of the microbial connectivity and decrease the complexity of the networks, we chose the family level. Prior to performing Spearman's correlation test between relative abundance of OTUs, we removed OTUs that were present only once in one sample. We aggregated OTUs based on family level and counted the number of connections (Spearman's rho > 0.85) between families To adjust P values, the Benjamini–Hochberg method was used (P < 0.01). The network topology was described based on set of measures, average clustering coefficients, average path length and modularity. The interactive platform Gephi was used to explore and visualize the structure of network. We generated 1000 random networks of equal size (same number of nodes and edges) as each of the original networks and compared their topological properties with the observed network to determine that the generated networks are not random. This analysis was performed in R software.
We performed a Random Forest analysis to explore the influence of environmental factors on network connectivity. Random Forest is a powerful machine learning tool that offers high prediction accuracy by using an ensemble of decision trees based on bootstrapped samples from a dataset (Breiman L. 2001). Random Forest was performed with 999 permutations using the ‘randomForest’ and ‘rfPermute’ packages in R. The best predictors were identified based on their importance using the ‘importance’ and ‘varImpPlot’ functions. Increase in node purity and mean square error values were used to determine the significance of the predictors using the random Forest Explainer package (Paluszynska and Biecek 2017). The factors significant at P < 0.01 were selected as the predictors of network connectivity.
Data deposition
The datasets generated in this study were deposited in the NCBI Sequence Read Archive database under accession number PRJNA526428.
RESULTS
The sequencing stage produced 260 072 sequences. After quality-filtering and chimera detection a total of 171 928 sequences were obtained from all samples and these could be clustered into 4687 OTUs at 97% similarity. Samples were analysed at a cutoff level of 1400 reads. Rarefaction (Fig. S3, Supporting Information) shows that no curves reached asymptote, as is typical for soil bacterial communities, but that the plant-associated communities were less diverse than the soils between plants.
A phylum breakdown of the bacterial community results is shown in Fig. 1. The community was dominated by several phyla, with Proteobacteria, Actinomycetes and Bacteriodetes being most abundant. Bacteriodetes were consistently much more abundant in the samples close to plants than in the unvegetated areas between plants.
A heat map diagram (Fig. 2) shows the major OTUs at genus or nearest known taxon level. An unclassified member of the Actinomycetales (Actinobacteria) and an unclassified member of the Bacteriodetes are particularly abundant under the plants, compared to control areas. Conversely, the plant-associated areas are depleted in several OTUs, including several unclassified Actinobacteria, that are abundant in the unvegetated control areas. Putative plant growth-promoting bacteria based on literature reviews are presented as a heat map in Fig. S4 (Supporting Information), comparing abundances between the soil under plants and bare soil.

Heat map generated based on the z-scores of relative abundance major bacterial OTUs identified to genus level.
A constrained principal component analysis (Fig. 3) shows that the unvegetated control sites have a discrete bacterial community from that existing under the plants, and also confirms a difference between the bacterial communities under male and female plants.
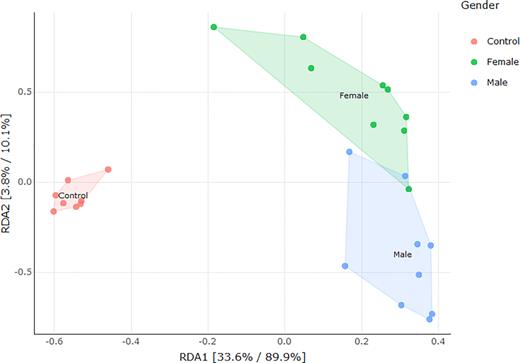
Principal component analysis constrained plot of soil bacterial community composition of control unvegetated soil, vs soil under male and female plants respectively.
PERMANOVA results (Table 1) confirm the dominant role of plant gender in affecting bacterial community composition. The analysis shows that bacterial community composition was affected by SM (F 1,2 = 10.66, P = 0.0001), explaining 25% of bacterial variation, pH explaining 7% of bacterial variation (F 1,2 = 3.13, P = 0.029), plant gender (F 2,2 = 3.64, P = 0.006) explaining 17% of bacterial variation and MAP (F 1,2 = 2.56, P = 0.04), explaining 6% of bacterial variation. However, the interaction between these parameters was not significant (Table 1).
Results of multivariate PERMANOVA showing significant differences in bacterial community composition.
. | Bacterial community composition . | ||||
---|---|---|---|---|---|
. | Df . | Mean square . | F model . | R2 . | P value . |
SM | 1 | 1.40 | 10.66 | 0.25 | 0.0001 |
pH | 1 | 0.41 | 3.13 | 0.07 | 0.029 |
Gender | 2 | 0.48 | 3.64 | 0.17 | 0.006 |
MAP | 1 | 0.33 | 2.56 | 0.06 | 0.04 |
SM*pH | 1 | 0.15 | 1.20 | 0.02 | 0.31 |
SM*gender | 2 | 0.11 | 0.87 | 0.04 | 0.58 |
pH*gender | 2 | 0.14 | 1.11 | 0.05 | 0.38 |
SM*MAP | 1 | 0.13 | 1.05 | 0.02 | 0.40 |
pH*MAP | 1 | 0.20 | 1.52 | 0.03 | 0.19 |
Gender*MAP | 2 | 0.12 | 0.96 | 0.04 | 0.50 |
SM*pH*gender | 2 | 0.10 | 0.80 | 0.03 | 0.65 |
SM*pH*MAP | 1 | 0.10 | 0.80 | 0.01 | 0.58 |
SM*gender*MAP | 2 | 0.06 | 0.52 | 0.02 | 0.93 |
pH*gender*MAP | 2 | 0.15 | 1.17 | 0.05 | 0.35 |
SM*pH*gender*MAP | 2 | 0.08 | 0.66 | 0.03 | 0.80 |
SM*pH*gender | 2 | 0.18 | 1.11 | 0.05 | 0.09 |
Residuals | 2 | 5.60 | 0.30 | ||
Total | 25 | 1 |
. | Bacterial community composition . | ||||
---|---|---|---|---|---|
. | Df . | Mean square . | F model . | R2 . | P value . |
SM | 1 | 1.40 | 10.66 | 0.25 | 0.0001 |
pH | 1 | 0.41 | 3.13 | 0.07 | 0.029 |
Gender | 2 | 0.48 | 3.64 | 0.17 | 0.006 |
MAP | 1 | 0.33 | 2.56 | 0.06 | 0.04 |
SM*pH | 1 | 0.15 | 1.20 | 0.02 | 0.31 |
SM*gender | 2 | 0.11 | 0.87 | 0.04 | 0.58 |
pH*gender | 2 | 0.14 | 1.11 | 0.05 | 0.38 |
SM*MAP | 1 | 0.13 | 1.05 | 0.02 | 0.40 |
pH*MAP | 1 | 0.20 | 1.52 | 0.03 | 0.19 |
Gender*MAP | 2 | 0.12 | 0.96 | 0.04 | 0.50 |
SM*pH*gender | 2 | 0.10 | 0.80 | 0.03 | 0.65 |
SM*pH*MAP | 1 | 0.10 | 0.80 | 0.01 | 0.58 |
SM*gender*MAP | 2 | 0.06 | 0.52 | 0.02 | 0.93 |
pH*gender*MAP | 2 | 0.15 | 1.17 | 0.05 | 0.35 |
SM*pH*gender*MAP | 2 | 0.08 | 0.66 | 0.03 | 0.80 |
SM*pH*gender | 2 | 0.18 | 1.11 | 0.05 | 0.09 |
Residuals | 2 | 5.60 | 0.30 | ||
Total | 25 | 1 |
Results of multivariate PERMANOVA showing significant differences in bacterial community composition.
. | Bacterial community composition . | ||||
---|---|---|---|---|---|
. | Df . | Mean square . | F model . | R2 . | P value . |
SM | 1 | 1.40 | 10.66 | 0.25 | 0.0001 |
pH | 1 | 0.41 | 3.13 | 0.07 | 0.029 |
Gender | 2 | 0.48 | 3.64 | 0.17 | 0.006 |
MAP | 1 | 0.33 | 2.56 | 0.06 | 0.04 |
SM*pH | 1 | 0.15 | 1.20 | 0.02 | 0.31 |
SM*gender | 2 | 0.11 | 0.87 | 0.04 | 0.58 |
pH*gender | 2 | 0.14 | 1.11 | 0.05 | 0.38 |
SM*MAP | 1 | 0.13 | 1.05 | 0.02 | 0.40 |
pH*MAP | 1 | 0.20 | 1.52 | 0.03 | 0.19 |
Gender*MAP | 2 | 0.12 | 0.96 | 0.04 | 0.50 |
SM*pH*gender | 2 | 0.10 | 0.80 | 0.03 | 0.65 |
SM*pH*MAP | 1 | 0.10 | 0.80 | 0.01 | 0.58 |
SM*gender*MAP | 2 | 0.06 | 0.52 | 0.02 | 0.93 |
pH*gender*MAP | 2 | 0.15 | 1.17 | 0.05 | 0.35 |
SM*pH*gender*MAP | 2 | 0.08 | 0.66 | 0.03 | 0.80 |
SM*pH*gender | 2 | 0.18 | 1.11 | 0.05 | 0.09 |
Residuals | 2 | 5.60 | 0.30 | ||
Total | 25 | 1 |
. | Bacterial community composition . | ||||
---|---|---|---|---|---|
. | Df . | Mean square . | F model . | R2 . | P value . |
SM | 1 | 1.40 | 10.66 | 0.25 | 0.0001 |
pH | 1 | 0.41 | 3.13 | 0.07 | 0.029 |
Gender | 2 | 0.48 | 3.64 | 0.17 | 0.006 |
MAP | 1 | 0.33 | 2.56 | 0.06 | 0.04 |
SM*pH | 1 | 0.15 | 1.20 | 0.02 | 0.31 |
SM*gender | 2 | 0.11 | 0.87 | 0.04 | 0.58 |
pH*gender | 2 | 0.14 | 1.11 | 0.05 | 0.38 |
SM*MAP | 1 | 0.13 | 1.05 | 0.02 | 0.40 |
pH*MAP | 1 | 0.20 | 1.52 | 0.03 | 0.19 |
Gender*MAP | 2 | 0.12 | 0.96 | 0.04 | 0.50 |
SM*pH*gender | 2 | 0.10 | 0.80 | 0.03 | 0.65 |
SM*pH*MAP | 1 | 0.10 | 0.80 | 0.01 | 0.58 |
SM*gender*MAP | 2 | 0.06 | 0.52 | 0.02 | 0.93 |
pH*gender*MAP | 2 | 0.15 | 1.17 | 0.05 | 0.35 |
SM*pH*gender*MAP | 2 | 0.08 | 0.66 | 0.03 | 0.80 |
SM*pH*gender | 2 | 0.18 | 1.11 | 0.05 | 0.09 |
Residuals | 2 | 5.60 | 0.30 | ||
Total | 25 | 1 |
Using the significant variables from the PERMANOVA (SM and gender (< = 0.1), we ran the redundancy analysis showing the variables effect on variation in soil bacterial community composition (Fig. 4).
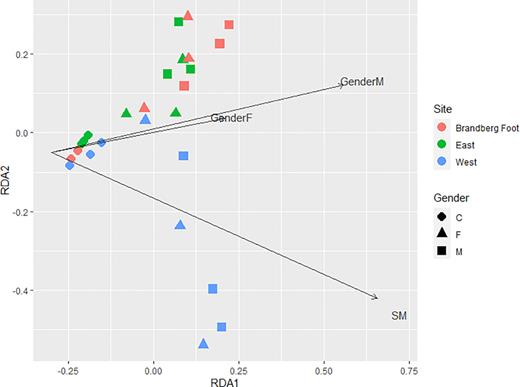
Redundancy analysis plot of soil bacterial community composition with biplot of significant factors found in the PERMANOVA.
Network analysis
Correlation-based network analysis was conducted to study the connectedness of the system (Faust and Raes 2012), based on the relationship between the bacterial families. In the connectivity analysis, based on strong significant positive correlations (r > 0.85 and P < 0.01). The greatest number of correlations was observed in the unvegetated areas (47 nodes and 600 edges) compared to Welwitschia plants (25 nodes and 139 edges). When comparing female plants with male plants, female plants (43 nodes and 81 edges) showed higher correlations compared to males (37 nodes and 44 edges) (Fig. 6 and Table S2 and Fig. S5, Supporting Information). Thus, connectivity analysis showed that the unvegetated control soil sites had much greater connectivity—in terms of both numbers of nodes and especially edges—than the soils under plants, with females having higher connectivity than males. Random Forest analysis reveal that SM is main factor influencing variation in network connectivity among samples, and that plant gender is the second most important factor (Fig. S6, Supporting Information).
DISCUSSION
Hypothesis 1
As expected, the soil associated with the Welwitschia plants has a distinct bacterial community, compared to unvegetated control soils away from any plants. The plant-associated biota was particularly rich in phylum Bacteriodetes, at around 30–40% of total reads, in contrast to the open spaces between plants that had relatively greater abundance of Proteobacteria and Actinobacteria. Bacteriodetes are known as a copiotrophic group which often utilizes complex but readily hydrolysable polysaccharides, as well as oligosaccharides (Berlemont and Martiny 2015) and their high abundance under Welwitschia may be due to some form of labile carbon derived from the plant. Rhizosphere soils of wild angiosperms have sometimes been found to have an abundance of Bacteriodetes—for example, in a semi-arid grassland in North America, three species of forbs had around 19% Bacteriodetes in the rhizosphere (Aleklett et al. 2015). Many studies from plants of more mesic and plant nutrient-rich environments (e.g. in maize, Yang et al. 2017) have found Bacteriodetes to be abundant in the rhizosphere, although this is not always the case. Our sampling under the plants was not strictly speaking within the rhizosphere of the main root, but is within the zone of finer lateral roots that fan out under the plant. Under the surface cover of the large leaves of the plant it is also possible that the soil biota was influenced by substances from above ground parts of the plant.
Despite some resemblances to the rhizosphere biota of some other plants, the relative abundance of Bacteriodetes under Welwitschia plants is higher than in any of the other examples of rhizosphere or soil biota that we could find in the literature. It is also interesting that the earlier study by Valverde et al. (2016) found much lower relative abundance of Bacteriodetes around Welwitschia plants—less than 6% of reads in the rhizosphere soil. This might be due to some sort of site effect in the single population sampled by Valverde et al. (2016), as opposed the three populations we sampled, or some other difference in methodology. Our survey was not the inner rhizosphere soil directly off the root surface, but instead the top 10 cm of bulk soil under the plant—which might make a substantial difference to the supply of plant-derived carbon from the roots. Since we sampled the whole soil mass and did not only sample directly off the root surfaces, it would be expected that root-derived carbon in our samples was actually less than in the study of Valverde et al, yet our recorded abundance of Bacteriodetes is much greater.
At a finer taxonomic level, in our results there are two dominant OTUs that are more abundant under the Welwitschia plants compared to unvegetated control areas—an unclassified member of the Actinomycetales (Actinobacteria) and an unclassified member of the Bacteriodetes (Fig. 2). Due to a lack of any relevant literature on these uncultured OTUs (which do not appear to have any close cultured relatives), the role that these may play in the system is unclear.
The OTU and genus level composition does not apparently reveal the presence of bacteria which have been seen as growth-promoting or mutualistic in plants in more mesic environments. For example, Rhizobium, Bradyrhizobium, PseudomonasandBurkholderia are not among the most abundant genera associated with the Welwitschia plants, despite their common associations with plant roots in many environments (Aleklett et al. 2018) and their apparent growth promoting effects in mesic agricultural environments (Hartmann et al. 2017). In contrast, Valverde et al. found Rhizobiales—including Rhizobium, Bradyrhizobium—as among the commonest groups of bacteria associated with Welwitschia plants. Again, it is possible that the difference is in some way a site-specific effect. We did not find any difference in relative abundance of the ‘typical’ plant mutualist genera Pseudomonas, Rhizobium, Bradyrhizobium, PseudomonasandSphingobacterium between soils from under plants and the control soils (Fig. S4, Supporting Information).
The Welwitschia plant-associated soil biota has lower richness and diversity of bacteria at the OTU and phylum level (Fig. 5) compared to the unvegetated control areas. This reduction in diversity in copiotrophic conditions is commonly observed in soils (Hartmann et al. 2017). A Wilcox non-parametric significance test was performed to compare Shannon diversity indices between the different genders. Significant differences were found between all three gender states (bare ground, male and female) (Fig. 5).
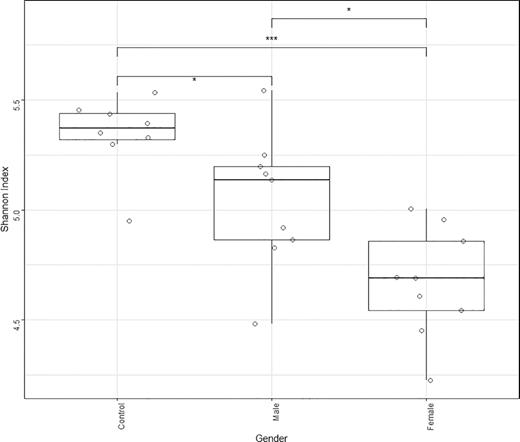
Shannon diversity of bacterial community, of control unvegetated sites, soil under female plants and under male plants, compared. *P < 0.05, **P < 0.01 and ***P < 0.001.
It is clear from both soil chemical analysis and bacterial community analysis, that the plant-associated soil—which has greatly elevated organic carbon and nitrogen concentrations—represents a distinct ecological system from the unvegetated soil from control areas away from the plants. It is interesting that despite the quite different climate and overall vegetation conditions between the three sites, varying from extreme desert to semi-desert scrub, the soil bacterial communities associated with the plants—and from unvegetated areas between plants—are very similar between the sites.
Unexpectedly, the bacterial community in unvegetated soil areas between Welwitschia plants had far greater network complexity than the soil under plants (Fig. 6), apparently related largely to differences in SM (Fig. S6, Supporting Information). This contrasts with the general observations of soils which form a stable, productive and nutrient rich system (Morriën et al. 2017). It is unclear what it is about the unvegetated soils that produces such high connectivity. While the unvegetated soil areas have slightly greater bacterial richness (Fig. S3, Supporting Information), the contrast is not great enough to produce such fundamentally different patterns. Explaining the high network connectivity of the unvegetated control soils thus remains a challenge for now, and it might be regarded as challenging the ecological paradigm that stable and biologically productive soils produce high connectivity. Organisms in extreme and biologically unproductive environments often depend more strongly upon mutualistic relationships, and it is possible that this is the cause for the greater connectivity of the unvegetated areas (Odum 1971). For example, desert crust, algal mats and lichens are all examples of consortia important on desert surfaces (Evans and Johansen 1999), and it is possible that the more interconnected bacterial community we observe is partly due to this.
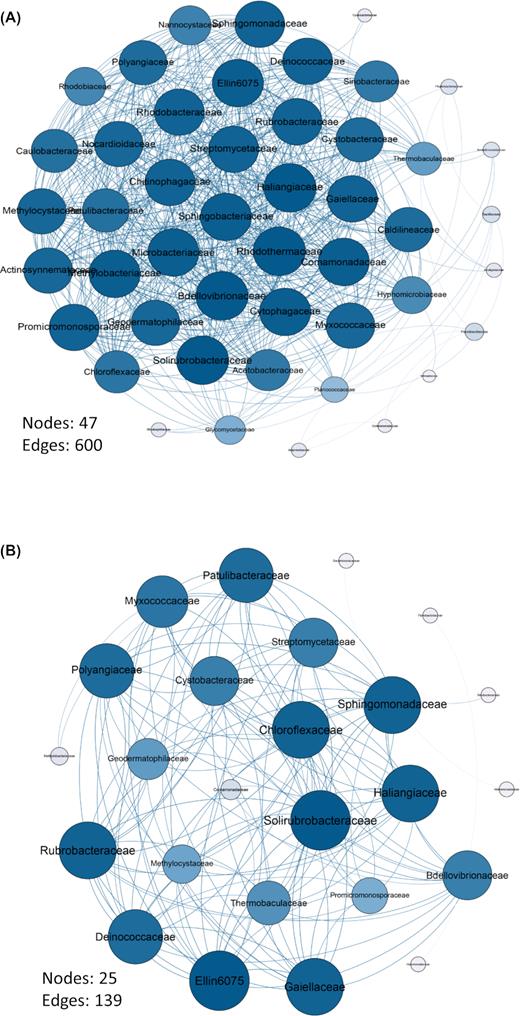
Connectivity diagrams of (A) unvegetated control soil areas (B) under all plants (male and female).
As we were not able to observe plants at a variety of different growth stages, it is to some extent unclear whether the ‘plant-associated’ effect on bacterial community structure is a result of the growth and activity of the plant, or whether pre-existing spatial differences in soil chemistry and soil texture (both known to influence soil microbial communities (Tripathi et al. 2012)) produced the differences in bacterial communities and in parallel also allowed the Welwitschia plants to establish and grow. It is plausible in fact that both factors are to some extent at work. Microsite differences are known to be very important in desert plant early establishment (Elller et al. 1983; Henschel et al. 2019), whereas feedback effects from plant growth that amplify spatial heterogeneity in the environment are known from many vegetation systems (Adams 2009). Judging by the strong differences in soil chemistry and biota between samples under the plants and those in bare areas, as well as their consistency across sites, it seems likely to us that the influence of the established plant on its surrounding soil dominates this effect.
It seems that there is a general consistency and stability in the associated community of Welwitschia, illustrating the strength of the ‘fertile island effect’ enforced by the plant. It would interesting to compare the fertile island effect on soil biota of another species from the same landscape in the Namib, A. spinosa (Sherman et al. 2019). Unfortunately, as their paper did not include any amplicon or other microbial community analysis, no taxonomic information is available.
Hypothesis 2
We found that as expected, location (under the plants vs unvegetated areas), and plant gender had a much greater effect on the composition of soil biota than the climate zone in which they were located. This seems to emphasise the constancy of the biotic and physical environment provided by the plant. However, unexpectedly, the bacterial community of the unvegetated control areas was also unaffected by climate zone—which is surprising considering the large rainfall gradient. This perhaps relates more to the microsite conditions of the locations where Welwitschia tends to grow, which are free draining gravelly substrates. While soil chemistry is generally considered a predominant influence on soil bacterial communities, soil texture and drainage conditions can also be important (Caporaso et al. 2011).
It may be worth considering that the high connectivity of the bacterial community of the unvegetated control areas is both a result of and a cause of the lack of response of the unvegetated soil bacterial community to differences in climate.
Hypothesis 3
We found clear statistically significant evidence (Fig. 3) of a difference in the associated soil biota between male and female Welwitschia plants, in agreement with our hypothesis. It is unclear what might cause this, and what its implications are for the soil system. Although our study did not find any difference in soil chemistry associated with plant gender, previous studies (Rhoades, Sanford and Clark 1994; Sherman et al. 2019) that found a male–female difference in soil chemistry invoke an effect of rotting fruit under the female plants. A previous study (Abrams et al. 1997) also found a gender-associated soil chemistry difference for Welwitschia. Although Welwitschia does not produce fruit, it does produce nectar drops from its cones to attract pollinating insects (Wetschnig and Depisch 1999) which could—in such a long lived plant—affect the soil underneath. It is also interesting that the soil under male plants has higher bacterial diversity than under female plants. If the soil environment under female plants is more copiotrophic, it is not surprising that there is lower bacterial diversity as copiotrophic environments commonly have lower bacterial diversity due to more intense competition and overgrowth by stronger competitors (Xia et al. 2020). Gender-related differences in input to soil of secondary metabolites—for example, from differences in nectar input—might also potentially affect soil bacteria: microbes are known to participate in metabolisms of soil allelochemicals (Zhu, Zhang and Ma 2011).
Another interesting contrast in the bacterial community connectivity is a difference in the number of connecting edges between male and female Welwitschia plants. Female plants had around twice as many edges, and different nodes, compared to male plants, despite their lower bacterial species richness (Fig. 6). Again, it is a challenge to explain why such a strong difference exists.
Surprisingly, in contrast with the earlier study by Henschel and Seely (2000), there was no difference in associated soil parameters between male and female plants. This contradiction is puzzling and suggests that the soil association may be strongly site dependent. It is also possible that it varies seasonally or is altered by the occasional rain events, even if it leaves a longer lasting legacy in the microbial community. However, the difference in bacterial community composition suggests that there must be real chemical differences in the soil between the plant genders, even if we were unable to identify them. What exactly these differences are, whether relating to root turnover, leaf turnover or root exhudates, is unclear, and no such gender-dependent differences have ever been reported before in the fertile island effect. As mentioned above, one plausible cause for the gender-related difference in soil biota is the production of drops of nectar secreted by the female cones to attract pollinating insects (Wetschnig and Depisch 1999). Secretion of secondary compounds to the soil is another possible mechanism by which plants may alter soil biota (Stinson et al. 2006) although no gender-specific effects have been reported. One possibility that may be considered is that the supply of carbon from fallen nectar also enhances nitrogen fixation or phosphorus mobilization by microbes under female plants, providing extra resources for seed production.
CONCLUSIONS
Our study revealed that a consistent fertile island effect on soil bacterial biota occurs in the case of the gymnosperm Welwitschia, with the ecology of the fertile island apparently differing little despite the range of climates sampled. It is unclear whether there are any mutualisms that help sustain in Welwitschia in this environment, but none of the ‘standard’ rhizosphere-associated mutualists (e.g. Rhizobium, BurkholderiaandPseudomonas) are abundant or even enriched under the plants. It may be of benefit to study in more detail those bacterial groups which are actually present in the Welwitschia rooting zone to provide potential novel mutualists for use in land rehabilitation in arid environments—although Welwitschia is so isolated taxonomically that its mutualists may have little relevance for other plants. It is intriguing that a strong effect of plant gender on bacterial community was detected: it would also be interesting to understand whether the distinctiveness of the root-associated community, and the differences between plant genders, also occurs across other groups such as archaea, fungi and small metazoans—and how these differences may translate into soil functional properties such as metabolic diversity and resilience. It would also be interesting to consider whether the differences in soil microbiome might in some way reflect functional niche differences between the two plant genders, perhaps playing an adaptational role.
Conflicts of interest
None declared.