-
PDF
- Split View
-
Views
-
Cite
Cite
Jolien Venneman, Caroline De Tender, Jane Debode, Kris Audenaert, Geert Baert, Pieter Vermeir, Pieter Cremelie, Boris Bekaert, Sofie Landschoot, Bert Thienpondt, Benoît Dhed'a Djailo, Danny Vereecke, Geert Haesaert, Sebacinoids within rhizospheric fungal communities associated with subsistence farming in the Congo Basin: a needle in each haystack, FEMS Microbiology Ecology, Volume 95, Issue 8, August 2019, fiz101, https://doi.org/10.1093/femsec/fiz101
- Share Icon Share
ABSTRACT
The unique ecosystem of the Congolese rainforest has only scarcely been explored for its plant–fungal interactions. Here, we characterized the root fungal communities of field-grown maize and of Panicum from adjacent borders in the Congo Basin and assessed parameters that could shape them. The soil properties indicated that comparable poor soil conditions prevailed in fields and borders, illustrating the low input character of local subsistence farming. The rhizosphere fungal communities, dominated by ascomycetous members, were structured by plant species, slash-and-burn practices and soil P, pH and C/N ratio. Examining fungi with potential plant growth-promoting abilities, the glomeromycotan communities appeared to be affected by the same parameters, whereas the inconspicuous symbionts of the order Sebacinales seemed less susceptible to environmental and anthropogenic factors. Notwithstanding the low abundances at which they were detected, sebacinoids occurred in 87% of the field samples, implying that they represent a consistent taxon within indigenous fungal populations across smallholder farm sites. Pending further insight into their ecosystem functionality, these data suggest that Sebacinales are robust root inhabitants that might be relevant for on-farm inoculum development within sustainable soil fertility management in the Sub-Saharan region.
INTRODUCTION
The Democratic Republic of Congo (DRC) belongs to the top five African countries with the highest number of small farms typically occupying less than 2 ha of land (Nagayets 2005). Most of these smallholder households practice predominantly cassava- and maize-based subsistence farming with no or limited access to synthetic fertilizers or other agrochemicals, resulting in poor yields (FAOSTAT 2018). Yield improvement demands sustainable intensification of crop production using alternative approaches that go hand in hand with ecosystem maintenance, for instance the implementation of an integrated soil fertility management (ISFM) (Vanlauwe et al. 2014; Tadele 2017). The latter concept is defined as ‘a set of soil fertility management practices that necessarily include the use of fertilizer, organic inputs and improved germplasm, combined with the knowledge on how to adapt these practices to local conditions, aiming at maximizing agronomic use efficiency of the applied nutrients and improving crop productivity’ (Vanlauwe et al. 2010).
A principle that has received less attention within ISFM, but that fits within the strategy, is the employment of plant growth-promoting (PGP) microorganisms that are indigenously present in sustainably managed fields (Sanginga and Woomer 2009; Bender, Wagg and van der Heijden 2016). In this respect, the diversity and abundance of arbuscular mycorrhizal fungi (AMF), as well as their response to changes in field management intensity, has been the subject of several studies conducted in Sub-Saharan Africa (Muleta et al. 2008; Tchabi et al. 2008; Straker, Hilditch and Rey 2009; Rodríguez-Echeverría et al. 2017). However, AMF are not the only group of root colonizers that might provide benefits to their hosts. The dark septate endophytes (DSE), a diverse complex of ascomycetes known for their ubiquitous presence in the roots of most terrestrial plants, may play an important role as well, especially in stressful environmental conditions (Jumpponen 2001; Rodriguez et al. 2009). Furthermore, extensive sampling during the last decade has shown that fungi from the order Sebacinales (Basidiomycota, Agaricomycetes) are also common endophytes in symptomless plant roots, revealing a previously overlooked ecological niche of this taxon (Selosse, Dubois and Alvarez 2009; Weiß et al. 2011). Additionally, in our recent study, endophytic sebacinoid isolates belonging to the elusive genus Piriformospora, which has recently been merged with the genus Serendipita (Weiß et al. 2016), were found to frequently occur in sudangrass trap cultures that were inoculated with rhizospheric soil from the Kisangani area in the DRC (Venneman et al. 2017).
The order Sebacinales is renowned for its versatile and unparalleled mycorrhizal abilities. Except for arbuscular mycorrhizal (AM) symbiosis, every other mycorrhizal type has been described within the taxon. The Sebacinaceae family (subgroup A) is involved in ectomycorrhizal and ectendomycorrhizal (arbutoid) interactions, as well as in orchid mycorrhizal associations in partially or fully mycoheterotrophic plants. The newly erected family Serendipitaceae (subgroup B) is represented by ericoid and cavendishioid mycorrhizas along with orchid mycorrhizas in green species, mycothalli in Jungermanniales liverworts and ectomycorrhizas in some lineages (Selosse et al. 2007; Oberwinkler et al. 2013; Weiß et al. 2016). Furthermore, it has been demonstrated that several axenically cultured sebacinoid fungi also possess endophytic features. Although originally isolated from South Australian orchids (Warcup and Talbot 1967; Warcup 1988), several strains of the Serendipita ‘vermifera’ species complex, for instance, are able to endophytically colonize the roots of a broad range of herbaceous host plants in which they display particular PGP and biocontrol properties (Barazani et al. 2005; Deshmukh et al. 2006; Barazani, von Dahl and Baldwin 2007; Waller et al. 2008; Ghimire, Charlton and Craven 2009; Ghimire and Craven 2011; Ray and Craven 2016; Sarkar et al. 2019). Additionally, Serendipita ( = Piriformospora) indica and S. ( = P.) williamsii, both derived from trap cultures containing Glomus spores (Williams 1985; Verma et al. 1998; Basiewicz et al. 2012), have been shown to significantly enhance plant growth and confer tolerance to abiotic and biotic stresses when applied as root inoculants under controlled experimental conditions (Varma et al. 1999; Waller et al. 2005, 2008; Deshmukh et al. 2006; Oelmüller et al. 2009; Sharma and Kogel 2009; Franken 2012; Venneman et al. 2017). The most recently accepted species within the enigmatic Serendipita genus, Serendipita herbamans, isolated from field roots of Bistorta vivipara, holds similar promise for stimulating plant growth, as indicated by laboratory inoculation assays (Riess et al. 2014). Over the past decade, in an attempt to bridge the gap between physiological studies, which mainly focus on the application of cultivable strains as endophytic inoculants, and molecular ecology data that initially tended to restrict Sebacinales to mycorrhizal interactions (ectomycorrhizal, orchid mycorrhizal, etc.), several studies have examined the roots of non-mycorrhizal and AM herbaceous plants across a broad spectrum of angiosperm families in diverse geographic regions. Using specific primers for sebacinoid ribosomal DNA combined with either cloning and Sanger sequencing (Selosse, Dubois and Alvarez 2009; Weiß et al. 2011; Garnica et al. 2013; Riess et al. 2014) or metabarcoding technologies (Verbruggen et al. 2014; Wehner et al. 2014), these reports revealed that although sebacinoid endophytes are ubiquitous across several ecosystems, their abundance in plant roots is generally low.
In this context, the present study aimed to investigate the prevalence, composition and taxonomic position of sebacinoid endophytes in smallholder field plots in the northeastern part of the DRC. Plants of interest included maize (Zea mays L.), as one of the country's most important herbaceous subsistence crops, and Panicum spp., which are among the most frequently observed weeds growing adjacent to agricultural sites. Based on the recently obtained collection of 51 new Congolese Serendipita isolates with PGP abilities (Venneman et al. 2017), the city of Kisangani and its surrounding areas were chosen for sampling. We used an Illumina metabarcoding strategy to test two hypotheses: (i) The roots of the agronomically important maize and those of common herbaceous weeds of the species-rich borders surrounding the agricultural fields (Panicum spp.) are characterized by distinct fungal and sebacinoid communities that are driven by specific abiotic and anthropogenic factors. (ii) The largely unexplored sampled Congolese soils are an appropriate source of putatively beneficial fungal taxa that could be implemented in an ISFM approach. For the latter, we evaluated the occurrence of positive and negative correlations between Sebacinales and other fungal groups, and assessed whether Panicum spp. growing in undisturbed weed borders serve as reservoir plants, harboring an actively proliferating sebacinoid population that can provide inoculum to newly installed agricultural crops improving their growth.
MATERIALS AND METHODS
Study sites and sampling
In July–August of 2015, soil and root samples were collected at 52 agricultural sites, distributed along six main roads serving the area of Kisangani (Tshopo Province; 00°31’N, 25°11’E, and 447 m above sea level), indicated as routes BAN (Banalia), IT (Ituri), MAS (Masako), OPA (Opala), SS (Simi-Simi) and YOK (Yoko) (Fig. 1A and Table S1, Supporting Information). Depending on the accessibility of the terrain, a maximal distance of 16 to 60 km from the city center was covered per axis. Kisangani has an equatorial climate, hot and humid all year round, with average monthly temperatures ranging from 27°C up to 34°C, and an average annual precipitation between 1700 and 2000 mm (World Weather Online 2018). However, a distinct dryer period spanning from November to February (< 50 mm per month) is usually recorded. Fields were randomly selected based on the presence of a sole or intercrop stand of maize, one of the most widely cultivated herbaceous crops in the area. After the annual dry period, farmers use the first rains to install new crops in March or April. At the time of sampling, maize plants grown for 2 to 4 months (silking-maturity) were chosen. Furthermore, an attempt was made to find a balance between farmland with and without ‘recent’ exposure to controlled fires, which are part of the common slash-and-burn practice in the region. Burning of the cleared vegetation within a period of four months prior to sampling, i.e. before installation of the maize crop, was considered as ‘recent’.
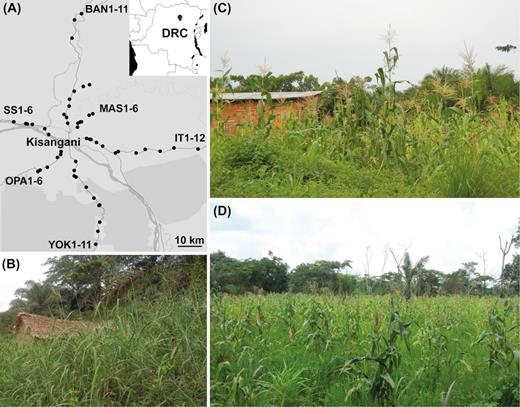
Sampling locations and typical smallholder field plots in Kisangani, DRC. (A) Map showing the 52 agricultural sites that were sampled in this study, starting from the city center of Kisangani and running north to the city of Banalia (samples BAN1-11), northeast to the village of Masako (MAS1-6), east to Ituri province (IT1-12), south through the Yoko forest reserve (YOK1-11), southwest to the city of Opala (OPA1-6) and west through the village of Simi-Simi (SS1-6). (B)Panicum plants in a weed-infested area adjacent to the field. (C) Small garden-like plot installed close to the homestead (near the side of the road) that has not been subjected to a controlled fire (MAS1). (D) Larger site situated deeper inside the forest and managed by slash-and-burn (IT12). In addition to the variety of subsistence crops (Table S1, Supporting Information), perennials like banana, pineapple and oil palm were occasionally located in and around the field. Furthermore, stumps and trunk pieces of cleared trees were also part of the agricultural scene in the (secondary) tropical forest. Map created using R package leaflet (Cheng, Karambelkar and Xie 2018) and maps from © OpenStreetMap.
Proportional to the field size, entire root systems and surrounding soil (upper 5–20 cm, diameter of 15 cm) of two to five representative maize plants, distanced at least 1 m away from each other, were carefully excavated with a shovel. Given that agricultural plots are often characterized by a spatial variability in soil nutrients, plants were carefully selected in areas of the field displaying a homogeneous plant growth. Soil samples were immediately pooled and one subsample per site was retained for determination of soil parameters. At each location, the same procedure was repeated for Panicum plants, which are commonly found as herbaceous weeds adjacent to agricultural sites.
To prevent degradation of fungal DNA, root systems were preserved at 4°C and treated within 24 h after sampling. First, adhering soil particles and organic debris were removed by washing the roots under running tap water for several minutes. Subsequently, dead and foreign root material was discarded and fine healthy-looking maize or Panicum root pieces were detached, followed by three additional rinses in distilled water. For each field, composite samples were prepared for maize and Panicum roots separately, and transferred to 10-mL cryovials containing cetyltrimethylammonium bromide (CTAB) extraction buffer (0.1 M Tris-HCl pH 8.0, 20 mM EDTA pH 8.0, 1.4 M NaCl, 2% CTAB). The tubes were preserved at room temperature for 1 or 2 weeks prior to shipment to Belgium (Faculty of Bioscience Engineering, Ghent University), where they were stored at −20°C until DNA extraction.
Physicochemical analysis of soil samples
Collected soil samples were bulked into one composite sample per site, which was air-dried and passed through a 2-mm-sieve before analysis.
Soil pH was measured in 1:5 (w/v) suspensions of soil in deionized water (pH-H2O) or 1 M KCl (pH-KCl). Total organic carbon (Corg) and total nitrogen (Ntot) were obtained using a Vario MAX elemental analyzer (Elementar, Germany) by measuring CO2 and N2 generated by combustion at 900°C (method Dumas 1831). Available P was analyzed in a Bray-II extract (Bray and Kurtz 1945) with inductively coupled plasma atomic emission spectroscopy (ICP-AES; IRIS Intrepid II, Thermo Scientific, USA). Cation exchange capacity and the exchangeable cations were determined using a 1 M ammonium acetate buffer at pH 7.0 (Van Ranst et al. 1999). In brief, ammonium cations (NH4+) in the buffer were exchanged for Ca2+, K+, Mg2+ and Na+ from the soil adsorption complex, after which the extracted basic cations were quantified with ICP-AES. For determination of cation exchange capacity, the NH4+ ions that had fully saturated the soil colloids were again desorbed through percolation of the substrate with 1 M KCl (exchange with K+ ions), and subsequently quantified in the solution using steam distillation. Particle size distribution, for maize samples only, was assessed by the Robinson-Köhn pipette method (Robinson 1922). When values lower than the analytical detection limit were obtained, they were set to half the value of this limit.
All soil parameters were evaluated according to the reference tables published in the ‘Booker Tropical Soil Manual’ (Landon 1991).
Downstream analysis and statistics of the soil characteristics
All statistical calculations were done in RStudio making use of R version 3.5.1 (R Core Team 2018). The significance level of the statistical tests was set at α = 0.05.
For reasons of comparability between analyses, the 95 samples that were retained in the final dataset of the molecular part below were considered. To visualize general differences in soil data, a principal component analysis with soil properties scaled to unit variance (autoscaling) was carried out through the PCA function in the FactoMineR package (Lê, Josse and Husson 2008). Only the soil characteristics that were available for both sampled plant species were included (all soil properties with the exception of texture data) and a Euclidean distance matrix was computed based on this set of variables, as a measure of environmental distance between sites. To further evaluate the effect of plant species on physicochemical composition, permutational multivariate analysis of variance (PERMANOVA) with 999 permutations was performed on this dissimilarity matrix, using the adonis function in the vegan package (Oksanen et al. 2018). Prior to this test, a permutation-based analysis of multivariate homogeneity of group dispersions (PERMDISP) was run in vegan with the betadisper function (999 permutations). The differences in individual soil parameters between the maize- and Panicum-associated samples were subjected to a closer examination by separate Wilcoxon signed-rank tests, using maize (field) and Panicum (border) data originating from the same location as paired observations (stats package; R Core Team 2018). One-sided tests were carried out under the general assumption that soil nutrient status in the field was higher than in the surrounding areas. Spearman's rank correlations were calculated to test the associations between the different soil variables (cor.test in stats).
To determine whether particular ‘experimental’ groups (e.g. burning versus not burning) varied in their environmental conditions, a global multivariate analysis of variance (MANOVA) was conducted on log2-transformed soil parameters, which had passed a multicollinearity check, using the Wilks’ Lamda test statistic (manova function in stats). Highly collinear soil variables were excluded from the explanatory dataset based on the calculation of correlation coefficients and variance inflation factors. The vifcor algorithm of the usdm package (Naimi et al. 2014) repeatedly looks for the variable pair that has the highest linear correlation above the threshold value (here set at 0.8) and removes the variable with the highest variance inflation factor.
DNA extraction, ITS2 amplification and sequencing
DNA was extracted from two composite samples per location, one consisting of maize roots from the field and the other one of Panicum roots taken from the border, using a CTAB protocol adapted from Doyle and Doyle (1987). Root fragments were ground in liquid nitrogen and 100 mg of the resulting powder was transferred to 500 µL of preheated 2% CTAB buffer, including 0.2% β-mercaptoethanol and 0.3 mg mL−1 proteinase K, added just before use. The mixture was incubated at 65°C for 60 min with gentle shaking every 15 min. The solution was cooled to room temperature prior to extraction of DNA with 500 µL chloroform-isoamyl alcohol (24:1). After continuously inverting the samples for 5 min, they were centrifuged at 12 000 rpm for 10 min. This step was repeated for the supernatant to which an equal amount of chloroform-isoamyl alcohol (24:1) was added. The final extract was treated with 1/100 volume RNase A (10 mg mL−1) for 30 min at room temperature. DNA was precipitated with equal parts of ice-cold isopropanol and incubated overnight at −20°C. A DNA pellet was recovered by cooled centrifugation (4°C) at 13 000 rpm for 30 min, and subsequently swirled in 500 µL wash buffer (80% ethanol with 15 mM ammonium acetate) for 10 min. This was followed by centrifugation at 13 000 rpm for 5 min and an additional washing step with 500 µL of ice-cold 70% ethanol. Finally, DNA pellets were air-dried and resuspended in 50 µL 0.1x TE buffer (1 mM Tris-HCl pH 8.0, 0.1 mM EDTA pH 8.0).
DNA concentrations were determined with a Quantus Fluorometer (double-stranded DNA; Promega, USA), after which all samples were set at a concentration of 5 ng µL−1. The fungal ITS2 rRNA gene region was amplified using an adapted fITS7bis forward primer and the ITS4NGSr reverse primer, both extended with Illumina specific adaptors, which was followed by a second PCR to attach dual indices and sequencing adaptors, as described in Debode et al. (2016). All PCR-products were purified with the CleanPCR reagent kit (MAGBIO, USA). The final libraries were quality controlled via the QIAxcel Advanced system with the QIAxcel DNA High Resolution kit (QIAGEN, USA) and concentrations were measured using a Quantus double-stranded-DNA assay. The final barcoded libraries of each sample were diluted to 10 nM and equally pooled. Resulting libraries were sequenced (2 × 300 bp, paired-end) on an Illumina MiSeq system by Admera (USA), using 30% PhiX DNA as spike-in.
Sequence processing
Demultiplexing of the amplicon dataset and barcode removal was done by the sequencing provider. The raw demultiplexed sequence data are freely available in the NCBI Sequence Read Archive under the accession number PRJNA529773 (National Center for Biotechnology Information; www.ncbi.nlm.nih.gov).
A detailed description of the sequence read processing can be found in De Tender et al. (2016). Briefly, primers were removed with Trimmomatic v0.32 (Bolger, Lohse and Usadel 2014), after which Illumina forward and reverse reads were merged by the program PEAR v0.9.8 (Zhang et al. 2014). Extraction of the ITS2 gene sequences from the complete amplicon sequence, which can still contain parts of the neighboring, highly conserved ribosomal genes, was performed by the ITSx program v1.0.11 (Bengtsson-Palme et al. 2013). The Usearch software v7.0.1090 (Edgar 2014) was used for quality filtering, merging of all sequences, dereplication and sorting by size. Further on, sequences were clustered into operational taxonomic units (OTUs) with an identity level of 98.5% using Uparse (Edgar 2014). Finally, sequences of individual samples were mapped back to the representative OTUs by implementation of the usearch_global algorithm at 97% identity, and converted into an OTU table (McDonald et al. 2012).
Taxon assignment of the fungal OTU sequences and downsizing of the dataset
Taxon assignments of fungal OTU sequences were done using the UNITE database (version 7.1, release November 20, 2016; Kõljalg et al. 2013). Rarefaction analysis carried out by implementation of the alpha_rarefaction.py script showed that rarefaction depth was reached at 25 000 sequences. Illumina sequencing failed with four samples (three samples of maize and one sample of Panicum) and five additional samples (three maize samples and two Panicum samples) contained a lower number of sequences than the established rarefaction depth; these samples were not considered for further analysis. The resulting dataset was characterized by a read count that varied across samples from 28 262 to 157 119. Accordingly, the number of retrieved OTUs ranged between 732 and 1870 per root system, adding up to a total of 12 399 different OTUs across the entire dataset. For subsequent data evaluation, this was further downsized to 3023 OTUs under the arbitrary prerequisite that OTUs were to be included for downstream analysis only if they had a minimal count of four in at least five different samples, taking both maize and Panicum samples into consideration. In addition, non-target organisms (Protista, eight OTUs) were removed while unassigned sequences were retained. In this final quality-filtered dataset of 3015 OTUs with a total sum of 6 598 913 reads, the read count per sample ranged between 26 609 and 155 351, with an average of 73 062 reads for maize and 66 083 in case of Panicum. This corresponded to a number of OTUs that varied from 458 to 1266 per examined root system (348–1073 when excluding singleton OTUs within particular samples), with an average count of 807 (632) and 764 (575) OTUs for maize and Panicum, respectively.
Phylogenetic analysis of Sebacinales OTUs
The ITS2 sequences of all detected Sebacinales OTUs were subjected to a BLAST search against the GenBank nucleotide database (NCBI), after which they were aligned together with their highest BLAST hits using the online service of MAFFT v7 with default settings (Katoh, Rozewicki and Yamada 2017). To position the OTUs within the Sebacinales order, representative sequences selected from the datasets of Oberwinkler et al. (2014), Garnica et al. (2016) and Weiβ et al. (2016) were also included in the analysis. The resulting alignment matrix, obtained via the FFT-NS-i iterative refinement method, was manually edited in Jalview (Waterhouse et al. 2009). In order to estimate phylogenetic relationships, a maximum likelihood (ML) tree was inferred from the final alignment via PhyML v3.1 (Guindon et al. 2010), implementing the TVM+I+G substitution model with corresponding parameters as suggested by the Akaike's information criterion in JModelTest v2.1.10 (Darriba et al. 2012). The ML heuristic search started with the construction of a BioNJ tree (Gascuel 1997), which was further improved through a combination of nearest-neighbor interchange and subtree pruning-regrafting branch swapping algorithms. Branch support for the ML tree was assessed based on the Shimodaira–Hasegawa approximate likelihood ratio test (SH-aLRT; Anisimova and Gascuel 2006). The phylogeny was rooted with Auricularia auricula-judae (DQ520099) as outgroup and visualized using iTOL v4.3 (Letunic and Bork 2016).
Downstream analysis and statistics of the fungal community composition
Statistical analyses were done in RStudio version 3.5.1 (R Core Team 2018). The significance level of each statistical test was set at α = 0.05.
For each sample of the final dataset, richness (Chao1 estimator) and diversity (Shannon–Wiener) indices were determined via the estimate_richness function of the phyloseq package (McMurdie and Holmes 2013). In addition, Pielou's evenness measure was calculated (J’ = H’/lnS; with H’ = Shannon diversity and S = total number of OTUs within sample). Differences in these alpha diversity metrics between defined ‘treatment’ groups were evaluated with t-tests.
Fungal community composition was studied by converting Hellinger-transformed OTU tables (Legendre and Gallagher 2001) into Bray–Curtis distance matrices. These dissimilarities in community structure were visualized through ordination of a principal coordinates analysis (PCoA; Gower 1966), using the cmdscale function of the stats package. PERMDISP and PERMANOVA statistics (999 permutations) were conducted on the Bray–Curtis distances to test biological differences due to grouping variables. For both the entire (maize + Panicum) and maize dataset, total as well as sebacinoid and glomeromycotan OTU composition was examined. When analyzing sebacinoid communities, samples without Sebacinales were removed from the dataset prior to multivariate analyses. To assess which OTUs (or orders) contributed most to the overall dissimilarity between the ‘treatment’ levels of the grouping variable, similarity percentage (simper) analysis was performed with the simper function in vegan. A supplemental multi-level pattern analysis (multipatt function) on absolute count data was conducted in the indicspecies package (De Cáceres and Legendre 2009) to identify OTUs that could be significantly associated with maize or Panicum (999 permutations). Indicator value indices (IndVal) are defined by two components, A and B: component A gives information about how restricted an OTU is to a specific group (specificity or positive predictive value), while component B is related to the probability of finding the OTU in samples belonging to that specific group (fidelity or sensitivity).
Finally, relative read abundances of specific fungal taxa (Sebacinales, AMF) were compared between ‘experimental’ groups by means of non-parametric Mann–Whitney U tests and correlated with other fungal taxa. For the latter, Pearson's correlation coefficients at the OTU, family and order level were determined based on Hellinger-transformed data.
Statistical analysis of the effects of soil and geographic parameters on fungal community composition
A first indication of the overall impact of soil and geographic properties on the OTU structure of the set of maize samples was provided by a partial Mantel test (mantel.partial function in vegan). Therefore, a separate Euclidean distance matrix was created for the soil variables and for the geographic coordinates, which had first been converted into (x,y) km-based cartesian coordinates (latlong2grid function in SpatialEpi package; Kim and Wakefield 2018). A Spearman's correlation coefficient was then calculated by comparing soil distances with the Bray–Curtis dissimilarity matrix of the fungal community data, while controlling for the effect of the spatial matrix, and vice versa (analyzing spatial distances while controlling for soil matrix). To link individual soil parameters with fungal OTU composition in maize roots, an unconstrained analysis using the envfit function in vegan was conducted, after excluding highly collinear soil variables (with vifcor, see higher). Correlations with a P-value below 0.050, based on 999 permutations, were fitted onto the PCoA ordination space, and the strength of the interactions was expressed through the goodness-of-fit statistic or squared correlation coefficient (r2).
To evaluate the influence of abiotic soil properties on the occurrence of specific fungal groups, Spearman's correlation coefficients were computed between individual soil parameters and relative read abundances of fungal taxa.
RESULTS
Study sites in the Kisangani area and sampling criteria
Along each of six road axes serving the Kisangani region in the tropical woodlands of the DRC (Fig. 1A), smallholder maize fields either recently exposed to a slash-and-burn event or not (Table S1, Supporting Information) were randomly selected for sampling. In addition to excavating entire maize root systems and collecting the adhering soil, samples were also taken from Panicum plants found as ubiquitous herbaceous weeds in the undisturbed borders adjacent to the agricultural sites (Fig. 1B). All field plots ranged in size from a few square meters near the hut, usually installed at the side of the road (Fig. 1C), up to 1 hectare deeper inside the forest or in more remote areas (Fig. 1D). Maize stands were primarily associated with cassava (Manihot esculenta Crantz) but other intercrops were encountered as well (Table S1, Supporting Information).
Physicochemical characteristics evidence poor soil conditions reflecting the low-input character of subsistence farming
All sampled soils in the study area were deeply weathered and had previously been classified as Ferralsols (van Engelen et al. 2006) according to the international WRB standard (World Reference Base for Soil Resources) of the Food and Agriculture Organization (FAO; IUSS Working Group WRB 2015). Soil particles were mainly composed of sand (median value: 79.62 ± 6.82%) and to a lesser extent of silt (8.26 ± 4.98%) and clay (11.83 ± 4.46%). The cation exchange capacity was very low, defined by median values of 6.2 (± 2.1) and 4.9 (± 2.1) cmol(+) kg−1 of soil in maize- and Panicum-associated samples, respectively. Further details on the physicochemical characteristics of the 95 evaluated soil samples are given in Table S2 (Supporting Information).
Ordination of the scaled soil properties by principal component analysis, preserving more than 60% of the original variance in the dataset, illustrated that the total physicochemical composition slightly differed between maize and Panicum rhizospheric soil samples (PERMANOVA, R2 = 0.050, P = 0.013). Differentiation between field and border seemed to be especially driven by pH-H2O (5.94 ± 0.59 in field soil versus 5.40 ± 0.42 in border soil), Bray-II P (11.85 ± 7.41 versus 5.26 ± 2.31 mg kg−1), C/N ratio (13.06 ± 1.97 versus 11.73 ± 1.67) and exchangeable Ca2+ (2.35 ± 1.49 versus 1.35 ± 0.86 cmol(+) kg−1), as indicated by the respective soil vectors that were directed towards the group of maize soil samples (Fig. S1, Supporting Information). This finding was confirmed by Wilcoxon signed-rank tests, demonstrating that most of the evaluated soil characteristics tended to have consistently higher values in the field than in the adjacent undisturbed border (P < 0.050), with the four above-mentioned parameters showing the most pronounced differences (P < 0.002).
Additionally, plots with and without recent exposure to slash-and-burn practices significantly differed from each other in their overall soil condition (MANOVA, Wilks’ λ, P = 0.043). The main contributor to this group difference appeared to be pH-H2O (univariate test, P = 0.044): sites that had been subjected to a controlled fire were defined by lower median pH-H2O values (5.84 ± 0.56) than those obtained from non-burned fields (6.14 ± 0.43). Fertilization effects that are usually linked with fire events, such as elevated levels of plant available nitrogen, phosphorus and basic cations, could not be detected at the time of sampling.
Similarly, when two ‘experimental’ groups were created for agricultural sites that were located at a distance of either more or less than 50 m from the road, pH-H2O was again found to be the most distinctive soil parameter, although not at a statistically significant level (MANOVA, Wilks’ λ, P = 0.156; univariate test, P = 0.052). In this case, median pH-H2O values were slightly higher in plots that were situated in proximity of the road (6.13 ± 0.62) than in fields that were installed deeper inside the forest (5.82 ± 0.42).
Taxonomic coverage of the rhizospheric fungal communities and phylogeny of Sebacinales OTUs
Each of the 3015 OTUs, retained after metabarcoding of the fungal ITS2 rRNA gene in 95 plant root DNA samples, occurred in 5 to 95 root systems (mean value: 24.73) and contained 25 up to 186 529 reads across all samples. The 75 most numerous OTUs covered 52% of the total read count (Table S3, Supporting Information).
The root-associated fungal communities were dominated by OTUs belonging to the Ascomycota, representing on average 46.30% (± 5.62%) of all retrieved OTUs per maize sample and 50.70% (± 7.50%) in case of Panicum (56.22 ± 11.77% versus 46.44 ± 18.15% of all reads). Glomeromycota and Basidiomycota were the second and third most abundant fungal phyla, respectively, accounting for 12.20% (± 2.51%) and 7.02% (± 1.43%) of the OTUs in maize versus 5.53% (± 2.53%) and 5.72% (± 1.23%) in Panicum (Fig. 2A and Table S4, Supporting Information). However, in terms of read abundance, Basidiomycota (maize: 16.02 ± 8.09%, Panicum: 14.17 ± 9.93%) appeared to be more strongly represented than Glomeromycota (maize: 4.63 ± 3.54%, Panicum: 0.64 ± 0.84%) (Fig. S2A and Table S4, Supporting Information). The remaining OTUs were mainly ‘unassigned’ and could not be identified at kingdom level (UNITE database), comprising on average 33.16% (± 5.14%) and 37.12% (± 6.28%) of all OTUs detected per maize and Panicum root system, respectively (22.24 ± 10.28% versus 37.24 ± 18.02% of all reads). Furthermore, a small proportion of the OTUs and reads (less than 2%) belonged to Chytridiomycota, Mortierellomycota, Mucoromycota and fungi with an unidentified or uncertain (‘other’, ‘incertae sedis’) taxonomic position (Fig. 2A, Fig. S2A and Table S4, Supporting Information).
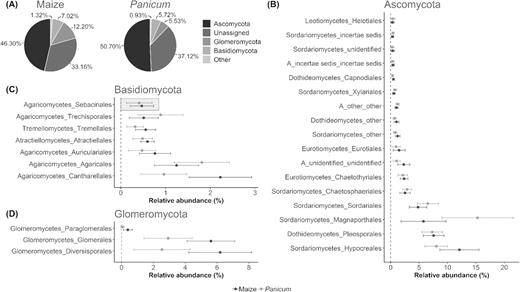
Taxonomic distribution of the 3015 OTUs (97% identity) according to the UNITE database. (A) Average relative OTU abundance of fungal phyla across root systems of the sampled plant hosts maize and Panicum. ‘Other’ comprises Chytridiomycota, Mortierellomycota, Mucoromycota and fungi with an unidentified or uncertain (‘other’, ‘incertae sedis’) taxonomic position, altogether accounting for less than 2% of all OTUs. (B, C, D) Dot plots of average abundance (percentage of total OTU count per sample) with standard deviation for the most frequently encountered orders, having a maximum relative OTU abundance of at least 1% per sample, grouped according to the dominant phyla Ascomycota (B), Basidiomycota (C) and Glomeromycota (D) and separated by plant host. Orders are ranked in ascending order from top to bottom by abundance values in maize. The target group of this study, the Sebacinales, is found among the top seven basidiomycetous orders and is framed in (C).
All OTUs, except for those classified as ‘unassigned’, could be allocated to 57 order groups. In maize roots, the Hypocreales (12.11 ± 3.44% of all OTUs), Pleosporales (7.57 ± 1.83%) and Magnaporthales (5.78 ± 3.95%) were the three ascomycetous orders with the highest relative OTU abundance (Fig. 2B). The dominant orders among the Basidiomycota were Cantharellales (2.23 ± 0.70%), Agaricales (1.25 ± 0.49%) and Auriculariales (0.76 ± 0.35%) (Fig. 2C). In Panicum, the most commonly occurring ascomycetous order was Magnaporthales (15.30 ± 6.29%), followed by Hypocreales (8.03 ± 2.00%) and Pleosporales (7.32 ± 1.84%) (Fig. 2B), whereas for the Basidiomycota, Agaricales (1.81 ± 0.62%), Cantharellales (0.96 ± 0.51%) and Trechisporales (0.89 ± 0.51%) appeared most frequently (Fig. 2C). Additionally, Diversisporales and Glomerales accounted for the largest fraction of Glomeromycota OTUs in the root fungal communities of both sampled plant species, with respective mean values of 6.20% (± 1.97%) and 5.61% (± 1.50%) in maize versus 2.54% (± 1.78%) and 2.92% (± 1.51%) in Panicum (Fig. 2D). Similar findings were obtained when ranking of the orders was based on relative read count instead of OTU-richness (Fig. S2, Supporting Information).
Sebacinoid fungi, which are the target group of this study, could be detected in the majority of the examined root systems, where they encompassed between 0.00% and 0.87% of all OTUs (3.62% of all reads) recovered per maize sample, and up to 1.02% of all OTUs (3.53% of all reads) in Panicum roots. Notwithstanding this low abundance, the Sebacinales taxon was listed among the 50% most important basidiomycetous orders (Fig. 2C, Fig. S2C and Table S4, Supporting Information) and consisted of 34 OTUs, 12 of which were retained in the final dataset (Table S5, Supporting Information). To analyze their phylogeny based on the ITS2 data, a heuristic ML approach was taken in which the 34 new sebacinoid OTUs were supplemented with a comprehensive set of publicly available reference sequences. This analysis clearly separated the Sebacinales into the known subgroups A and B (Weiβ et al. 2004; Fig. S3, Supporting Information), which have been recently appointed as the Sebacinaceae (Oberwinkler et al. 2014) and Serendipitaceae (Weiβ et al. 2016) families, respectively. The new Congolese sequences all clustered within the Serendipitaceae and spanned different phylogenetic groups. The 12 most dominant OTUs, with the exception of SEB31, were distributed across two well-supported clades (SH-aLRT values > 0.70), indicated as ‘cluster 1’ and ‘cluster 2’ (Fig. 3 and Table S5, Supporting Information). This taxonomic coverage was expanded with three additional clusters when the 22 minor OTUs (and the dominant SEB31) were considered as well (Fig. S3, Supporting Information).
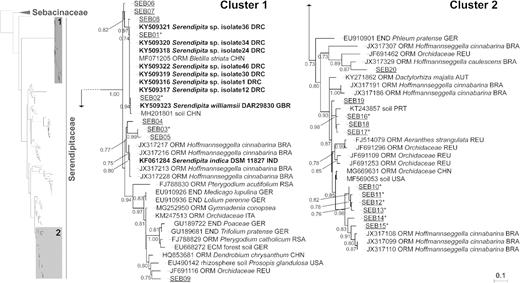
Serendipitaceae phylogeny with emphasis on the distribution of the most dominant sebacinoid OTUs associated with maize and Panicum roots from agricultural sites in the Congo Basin and their relationships with publicly available reference sequences. Tree topology was inferred from 170 sequences spanning the ITS2 rRNA gene region by a ML approach using the TVM+I+G substitution model; Auricularia auricula-judae (DQ520099) was chosen as outgroup. Labels include accession numbers from GenBank (NCBI), interaction types, host plants or other environmental sources (soil) and geographic origin. The sebacinoid OTUs from this study are designated as ‘SEB’ (underlined) and sequences derived from identified fungal specimens or strains are indicated in bold. SH-aLRT branch supports (≥ 0.70) are displayed at the nodes. All but one of the 12 most commonly retrieved Congolese OTUs, marked here with an asterisk (Table S5, Supporting Information), are grouped into two well-supported clades, designated as ‘cluster 1’ and ‘cluster 2’. The OTUs that were putatively identified as ‘Piriformospora’ spp. could be allocated to the first cluster, which contains the reference species Serendipita ( = Piriformospora) indica and S. ( = P.) williamsii, as well as members of the Congolese S. ‘williamsii’ species complex. Phylogenetic placement of all 34 recovered OTUs within the Serendipitaceae family is shown in the full tree available in Fig. S3 (Supporting Information). Association types: ECM, ectomycorrhiza; END, endophyte; ORM, orchid mycorrhiza. Country codes: AUT, Austria; BRA, Brazil; CHN, China; DRC, Democratic Republic of Congo; GBR, Great Britain; GER, Germany; IND, India; ITA, Italy; PRT, Puerto Rico; REU, Reunion Island (France); RSA, Republic of South Africa; and USA, United States of America.
Each of the nine OTUs that were putatively identified as ‘Piriformospora’ spp. (SEB01-09) could be allocated to ‘cluster 1’. With regard to the most frequently encountered OTUs, SEB03 was affiliated with S. indica and mycorrhizal sequences from Brazilian Hoffmannseggella cinnabarina orchids (Oliveira et al. 2014), whereas SEB01 and SEB02 were more closely related to the recently proposed S. ‘williamsii’ species complex consisting of previously isolated Congolese relatives from the Kisangani region (Venneman et al. 2017). The less frequently amplified SEB04-05 and SEB06-08 were also closely linked with S. indica and S. williamsii, respectively, in contrast to SEB09 that had a rather distant connection with the reference strains.
The more complex ‘cluster 2’ harbored 8 of the 12 dominant Sebacinales OTUs (SEB10-17) and 3 of the minor OTUs (SEB18-20). Whereas SEB10-15 clustered together with sequences derived from mycorrhizas of the orchid H. cinnabarina (Oliveira et al. 2014), sequences obtained from other specific orchid mycorrhizal associations (Martos et al. 2012; Yang et al. 2017) and from soil (Urbina et al. 2016; Beck and Yannarell 2017) constituted the highest BLAST hits to SEB16-19. Additionally, SEB20 seemed to be most closely related to ORMs detected in Hoffmannseggella caulescens (Oliveira et al. 2014).
Host plant, agricultural management within a tropical forest landscape and particular soil characteristics shape the total fungal communities
For a detailed analysis of the fungal OTU tables, we focused on (i) differences in fungal richness, diversity and evenness; (ii) differences in community composition; and (iii) influence of environmental parameters, including geographic location and soil properties. The grouping variables of interest were plant species (maize, Panicum), cropping systems (vegetation type in previous season), burning practices (subjected to slash-and-burn or not) and distance between field and road (less or more than 50 m).
The number of OTUs obtained from the root systems of maize and Panicum was comparable (range 564–1266 and 458–1133, respectively). Consequently, the estimated OTU richness, defined by respective average Chao1 values of 980 (± 187) and 953 (± 188), did not show a significant difference between both plant hosts (t-test, P = 0.493). Fungal Shannon diversity, on the other hand, was significantly higher in maize than in Panicum (P < 0.001), with respective mean indices of 4.27 (± 0.36) and 3.70 (± 0.47), already indicating differences in microbial evenness. The latter was confirmed by Pielou's evenness values, which averaged 0.64 (± 0.05) in maize roots and 0.56 (± 0.06) in Panicum roots, suggesting a more even fungal species distribution in the field as compared to the border (P < 0.001).
Furthermore, ordination analysis demonstrated that fungal OTU composition was clearly structured by host plant species. Maize and Panicum samples could visually be separated from each other along the PCoA plot's first axis, which explained 15.70% of the variation in the original dissimilarity matrix (Fig. 4). Homogeneity of dispersion between both plant groups was fulfilled (PERMDISP, P = 0.672) and observations could therefore be validated by PERMANOVA (R2 = 0.127, P = 0.001). At the order level, a similar separation between maize and Panicum was found (PERMANOVA, R2 = 0.260, P = 0.001; Fig. S4A, Supporting Information), with up to 79% of the detected dissimilarity between both plant species determined by a set of 20 order groups (simper analysis, footnote Table S4, Supporting Information). Magnaporthales, Hypocreales and Cantharellales appeared to be the main drivers behind the observed patterns in fungal community structure, with respective estimated contributions of 8.30%, 7.48% and 6.86%.
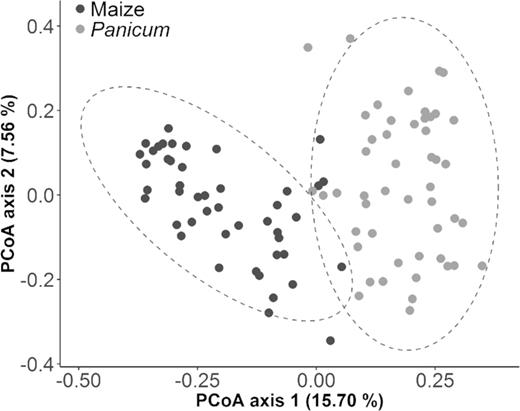
PCoA ordination (first two dimensions) based on Bray–Curtis dissimilarities of Hellinger-transformed OTU abundance data from 46 maize root systems (dark-colored dots) and 49 Panicum root samples (light-colored), collected in agricultural fields and adjacent weed-infested areas, respectively. Distinct patterns of fungal community composition are observed according to the sampled plant type. Simper analysis revealed an overall Bray–Curtis dissimilarity between plant groups of 81% with the 75 most discriminative OTUs collectively accounting for 20% of this observed dissimilarity. The majority of these top contributors are also included in the list of the 75 most abundant OTUs and contain several important indicator species of each host (IndVal > 0.800) (Table S3, Supporting Information). Dashed lines represent 95% confidence ellipses.
Concerning the impact of agricultural management on fungal community composition, we analyzed the effect of crop rotation schemes, slash-and-burn practices and location of the field relative to the forest. Because the Panicum samples were taken from undisturbed areas adjacent to the agricultural field, these were not considered here. Bray–Curtis dissimilarities among the OTUs of the different maize samples were plotted in a reduced ordination space. As illustrated in Fig. 5, maize fungal communities seemed to be moderately clustered into two groups, being partly separated from each other along the PCoA plot's first axis, which together with the second axis accounted for 22.84% of the total variance in the original dissimilarity matrix.
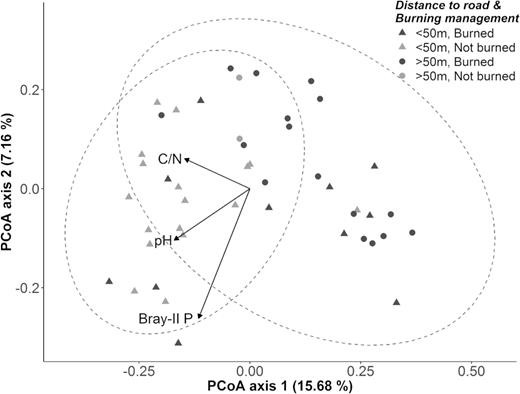
PCoA ordination based on Bray–Curtis dissimilarities of Hellinger-transformed OTU abundance data from 46 maize root systems, with soil variables fitted onto the two-dimensional space as vectors. Colors represent the two burning treatments (slash-and-burn or not) and symbols refer to the location of the field in relation to the forest (distance between field and road more or less than 50 m). Note that both grouping variables largely coincide since slash-and-burn management is generally practiced at a safe distance from the homestead, usually somewhat deeper inside the forest. When controlled fires did occur near the side of the road, this mainly happened before installation of the family hut or at larger areas that were not managed as family gardens. The soil vectors Bray-II P, pH and C/N ratio are significantly correlated with the ordination configuration; their arrows point in the direction of maximum increase. Dashed lines represent 95% confidence ellipses based on the two burning treatments.
Vegetation type of the previous season could not be linked to the observed clustering pattern (PERMANOVA, P > 0.050) and type of intercrop could not be evaluated due to the dominance of cassava. However, burning management did seem to have a notable impact on the fungal communities as their composition differed significantly between the two burning treatments (PERMANOVA, R2 = 0.061, P = 0.001). Furthermore, Chao1 values were significantly lower (t-test, P = 0.015) in fields with slash-and-burn management, as opposed to sites that had not been subjected to a controlled fire (924 ± 172 versus 1060 ± 183, respectively).
Notwithstanding these findings, the differentiation among maize root-associated communities in PCoA ordination could equally be explained by the location of the field in relation to the forest. Indeed, grouping according to this spatial variable largely corresponded to the one based on burning management, suggesting that the choice of burning practice depends on how far the field is situated from the road and homestead (Fig. 5). Together with a number of burned plots, all but three of the non-burned sites in the PCoA ordination space clearly coincided with the cluster of fields that were found close to the road and family hut (at less than 50 m), in a rather small garden-like setting. On the other hand, sampling sites that were located at a distance of more than 50 m from the road were usually characterized by a recent slash-and-burn event (Fig. 5, and Table S1, Supporting Information). This indication of a difference in fungal community composition between the two described spatial groups was confirmed by a significant PERMANOVA test (R2 = 0.059, P = 0.001), although differences in group dispersion (PERMDISP, P = 0.002) might have contributed to the latter result as well. In addition, the fields that were installed deeper inside the forest were defined by lower Chao1 indices (906 ± 133) as compared to the plots that were closely connected with the homestead (1028 ± 203) (t-test, P = 0.018).
To account for the influence of site-specific environmental parameters on OTU distribution in maize root systems, a partial Mantel test was carried out comparing fungal community dissimilarity with the distance matrices of the geographic coordinates and the soil characteristics. Whereas no significant correlation was found between geographic location and community structure (Mantel r = 0.091, P = 0.071; controlling for the effect of soil properties), the OTU dissimilarity matrix did seem to be linked with differences in soil properties among the sampled sites (Mantel r = 0.160, P = 0.012; controlling for the spatial matrix). For that reason, all soil variables were overlaid onto the ordination space in an unconstrained analysis (envfit) to identify the main edaphic drivers behind fungal community composition in maize roots; three variables with collinearity problems were excluded prior to analysis (Corg, sand and Ca2+). Among the remaining set of eleven parameters (variance inflation factor < 7), Bray-II P (r2 = 0.404, P = 0.001), pH-H2O (r2 = 0.197, P = 0.009) and C/N ratio (r2 = 0.125, P = 0.049) were moderately but significantly correlated with OTU composition (Fig. 5).
Sebacinoid communities respond distinctly to different environmental conditions compared to the total and glomeromycotan populations
Sebacinoid sequences could be retrieved from the majority of the examined root samples, with detection of at least two reads per root system in 87% of the maize samples and 80% of the Panicum samples. Notwithstanding this widespread occurrence, their abundance values were very low, with mean values of 0.40% of all reads in maize and 0.23% in Panicum (Fig. S2C, Supporting Information), constituting a statistically significant difference (Mann–Whitney U test, P = 0.033).
The sampled plant species also seemed to have a significant effect on the occurrence of individual OTUs, resulting in distinct sebacinoid communities in both hosts (PERMANOVA, R2 = 0.076, P = 0.001; Fig. 6A). SEB10, SEB01 and SEB02 dominated the set of 12 Sebacinales OTUs and covered more than 70% of all sebacinoid reads across samples (Table S5, Supporting Information). Consequently, host plant-driven differential abundances among these three OTUs were mainly responsible for structuring the Sebacinales communities. SEB01 was present in as much as 32 of the 46 analyzed maize samples, compared to 12 out of 49 for Panicum, and also SEB02 was more strongly associated with maize root systems than with those of Panicum (present in 25 versus 9 samples, respectively; Table S5, Supporting Information). Interestingly, both SEB01 and SEB02, as well as SEB03, were tentatively identified as ‘Piriformospora’ spp. closely related to the recently described S. ‘williamsii’ species complex (Venneman et al. 2017) (‘cluster 1’ in Fig. 3). Collectively, they represented on average 0.233% (range: 0.000–3.603%) and 0.025% (range: 0.000–0.841%) of the total read count per maize and Panicum root sample, respectively. This higher prevalence of ‘cluster 1’ spp. in the fungal communities of maize was found to be statistically significant (Mann–Whitney U test, P < 0.001). Also for the unidentified Serendipitaceae OTU SEB10 (‘cluster 2’ in Fig. 3) more reads were recovered from maize than from Panicum roots with respective mean values of 0.114% and 0.069% (range: 0.000–0.949% versus 0.000–0.683%), which, however, did not constitute a significant difference (Mann–Whitney U test, P > 0.050). Furthermore, the reads of SEB10, as well as those of the closely related SEB11-14, were distributed over a more even number of maize and Panicum samples as compared to the figures obtained for SEB01 and SEB02 (Table S5, Supporting Information).
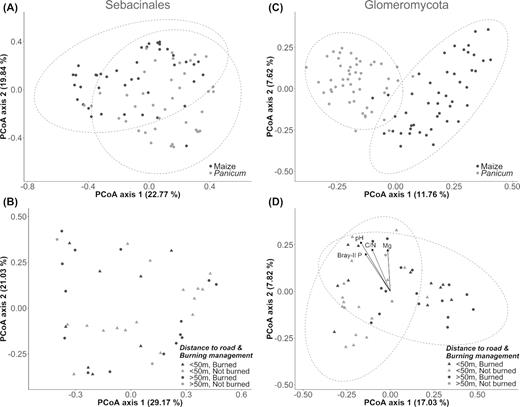
PCoA ordination of sebacinoid and glomeromycotan (AMF) communities (based on Hellinger-transformed OTU abundances) showing the effects of sampled plant type and management or location related factors. (A, C) Ordination configuration of all samples demonstrating a separation of the symbiotic communities according to plant host (maize versus Panicum), which is more pronounced in case of AMF. (B, D) PCoA diagram of maize samples only, displaying the influence of burning management (colors) and field location relative to the forest (distanced more or less than 50 m from road; symbols). Whereas the 12 considered Sebacinales OTUs are not significantly clustered by the grouping variables or correlated with specific soil variables (B), a moderate clustering is observed among the 362 AMF OTUs and their composition seems to be linked with the soil vectors pH, C/N ratio, Bray-II P and Mg (D). Dashed lines represent 95% confidence ellipses based on sampled plant host (A, C) or the two burning treatments (B, D).
Then, the impact of agricultural and environmental factors on the sebacinoid communities was evaluated by ordination analysis. Sebacinoid OTU composition in the maize root systems did not seem to be clustered according to specific grouping variables, including burning management (PERMANOVA, P > 0.050; Fig. 6B), nor was it driven by particular soil chemical properties (envfit, P > 0.050). Similarly, neither the total Sebacinales read abundances nor those of the putative ‘Piriformospora’ spp. (SEB01-03) were influenced by management practices (Mann–Whitney U tests, P > 0.050) or correlated with soil variables or alpha diversity indices (Spearman, −0.250 < r < 0.250).
Next, in order to assess the specificity of this behavior of Sebacinales in the studied ecosystem, we analyzed the community composition of the Glomeromycota, as a well-studied symbiotic phylum known for its AM associations. This taxon was mainly composed of fungi belonging to the orders Glomerales and Diversisporales (class Glomeromycetes), which collectively accounted for 97% of the 362 considered glomeromycotan OTUs, and for more than 98% of the AMF reads. Both groups were listed among the top 20 orders that contributed most to the overall dissimilarity between the root-associated total fungal communities of maize and Panicum, unlike the Sebacinales order that could not be identified as an important discriminative order (simper analysis, Table S4, Supporting Information). The Glomerales were detected at an average relative read abundance of 2.84% in the roots of maize (range: 0.14–14.87%) compared to 0.50% in those of Panicum (range: 0.01–4.87%). A similar strong host plant effect was demonstrated among the Diversisporales, which were characterized by respective mean abundance values of 1.70% (range: 0.07–6.53%) and 0.13% (range: 0.00–0.62%) (Fig. S2D, Supporting Information). Consequently, the composition of the 362 mycorrhizal OTUs was distinctly clustered by sampled plant species in PCoA ordination (Fig. 6C). This observation was further supported by a significant PERMANOVA test (R2 = 0.092, P = 0.001), although the result might have been influenced by differences in group dispersion as well (PERMDISP, P = 0.002). Additionally, simper analysis revealed that a set of 20 OTUs explained approximately 20% of the overall 89% dissimilarity between both plant groups (Table S6, Supporting Information); with the exception of one OTU, all of them were more strongly associated with maize than with Panicum. Furthermore, comparable to the total fungal community composition but in contrast to that of the sebacinoids, a moderate clustering in maize AMF communities was observed, which appeared to coincide with burning management (PERMANOVA, R2 = 0.055, P = 0.002) and location of the field relative to the forest (PERMANOVA, R2 = 0.060, P = 0.001) (Fig. 6D). Glomerales and Diversisporales read abundances, however, did not seem to be affected by these grouping variables (Mann–Whitney U tests, P > 0.050), whereas glomeromycotan Chao1 and Shannon indices (t-test, P < 0.050) appeared to be lower in fields with a recent fire exposure (105 ± 32 and 3.37 ± 0.49, respectively) as compared to those without (131 ± 33 and 3.68 ± 0.36). Concerning the effect of soil characteristics, AMF community composition in maize was generally linked with the variables pH-H2O (r2 = 0.332, P = 0.001), C/N ratio (r2 = 0.209, P = 0.004), Bray-II P (r2 = 0.207, P = 0.002) and exchangeable Mg2+ (r2 = 0.171, P = 0.013), as demonstrated by envfit vector fitting (Fig. 6D).
The Sebacinales exhibit moderate (in)compatibilities with other fungal groups
To uncover potential interactions between sebacinoid fungi and other fungal groups in maize samples, Pearson's correlation coefficients at the family level were calculated. Groups with unidentified or uncertain family positions and with less than 1% maximum relative abundance across samples were not considered. Although none of the remaining 29 families appeared to be strongly correlated with the Serendipitaceae (|Pearson's r| < 0.500), some interesting trends could be observed (Table S7, Supporting Information). The most pronounced and negative relationship seemed to be established with the Hypocreaceae family (r = −0.362, P = 0.013), whose members could mainly be identified as Trichoderma spp. (65% of all OTUs; 82% when including the Hypocrea teleomorphs). At the OTU level, this negative association was not clearly present when looking at the most abundant Sebacinales OTU (SEB10), though it did stand out in case of the most important OTU from Serendipitaceae ‘cluster 1’ (SEB01). For the latter, a significant and nearly significant negative correlation was found with a Hypocrea species (r = −0.309, P = 0.036) and with three Trichoderma OTUs, respectively (r = ±−0.280, P < 0.064). Therefore, co-occurrence of these two types of mutualistic endophytes appeared to be uncommon.
As far as the AMF families were concerned, Glomeraceae and Claroideoglomeraceae showed the strongest, however non-significant (P = 0.070), interaction with Serendipitaceae (r = 0.270 for both). At the order level, a significant Pearson's correlation coefficient of 0.327 was obtained between Sebacinales and Glomerales (P = 0.026), implying that both symbiotic groups co-exist in maize root fungal communities. In Panicum samples, no noteworthy associations between particular fungal taxa and Sebacinales or Serendipitaceae were found at the order or family level, respectively. Nevertheless, there was a trend of co-occurrence for Sebacinales and Glomerales (r = 0.261, P = 0.070).
When considering all 34 detected OTUs with putative Sebacinales identity, approximately 83% of the maize samples and 65% of the Panicum samples contained more than one OTU per root system (with at least two reads per OTU). For maize, a maximum number of 11 different OTUs per sample was obtained, with a median value of 4 OTUs. With regard to Panicum, the maximum and median values were 10 and 2, respectively. Therefore, strong competition amongst sebacinoid strains seems unlikely.
DISCUSSION
The distinct fungal communities supported by maize and Panicum roots are mainly driven by host plant and environmental factors, rather than by agricultural practices
Notwithstanding that Ascomycota represented the most abundant phylum in both maize and Panicum root systems, our data clearly showed a host-driven clustering in root-associated fungal community structure. The Hypocreales constituted the most important order in maize samples, with strong representations of Fusarium (Nectriaceae), Myrothecium (incertae sedis), Sarocladium (incertae sedis) and Trichoderma (Hypocreaceae; teleomorph Hypocrea), while Panicum roots appeared to be dominated by Magnaporthales reads, with Pseudophialophora as most abundant genus.
Given that AMF diversity and colonization rate generally decline in response to increased land-use intensity (Jansa et al. 2003; Oehl et al. 2004; Tchabi et al. 2008; Lumini et al. 2010) and that maize samples were harvested in cultivated fields as opposed to the Panicum samples from the undisturbed borders, it was not unexpected to find a contribution of the AMF orders Glomerales and Diversisporales to the overall dissimilarity among the fungal communities of both hosts. However, in contrast to the prediction, both orders were more abundant in the cultivated maize plants than in the neighboring Panicum plants. Although soil analysis pointed out that some soil variables were slightly elevated in the field as compared to the borders, within an agricultural context, the overall soil nutrient status at the farm sites was not substantially different from that of their surrounding ‘microhabitat’, and it appeared to be too depleted to support high crop yields. Moreover, in addition to the restricted application of external inputs, the dense weed cover and the many intercrops that are usually found in smallholder field plots may have positively affected root-associated fungal communities in maize, as was suggested before in wheat (Verbruggen et al. 2014). Consequently, subsistence agriculture in the Congo Basin can actually be considered as unintended organic farming, favoring the maintenance of a high soil microbial diversity in the field. In line with this reasoning and consistent with previous studies reporting a diversity peak of most fungal groups in tropical soil ecosystems (Tedersoo et al. 2014), the average Shannon index in both Panicum and maize roots was extremely high (3.70 ± 0.47 and 4.27 ± 0.36, respectively). As a reference, Shannon diversity typically varies between 1.5 and 3.5, and rarely surpasses 4.0 (Magurran 2004). Taken together, the compositional differences in the fungal communities of the analyzed roots could mainly be attributed to the plant effect, rather than the impact of agricultural management.
However, when analyzing the impact of slash-and-burn practices in the sampled region, the total fungal community grouped into two distinct clusters coinciding with the applied burning treatments. Despite this observation, none of the examined soil parameters pointed at significant changes in soil fertility due to field burning, which is in conflict with most studies describing specific fertilization effects (Certini 2005). Thus, it seems likely that burning management was not carried out to an extent that it could have seriously altered soil chemical and microbial composition. Interestingly, a similar clustering pattern as with burned and non-burned fields was obtained when grouping in the PCoA ordination space was based on distance between field and road, implying that the environmental setting of the field in relation to the forest is a more plausible explanation for the observed pattern in fungal community structure.
Finally, soil pH was identified as the main discriminative soil characteristic between the ‘treatment levels’ of the above-discussed grouping variables and it was also found to exert a significant impact on total fungal OTU composition in the rhizosphere of the examined plants. This finding is consistent with several other studies where pH has been reported as a key edaphic factor shaping microbial community structure (Högberg, Högberg and Myrold 2007; Dumbrell et al. 2010; Rousk et al. 2010; Tedersoo et al. 2014; Zhang et al. 2016; Maghnia et al. 2017; Zhang et al. 2019). Furthermore, available (Bray-II) P and soil C/N ratio also proved to be significantly correlated with the community distance matrix. The C/N ratio is a useful indicator of the decomposability of soil organic matter and it frequently recurs as a determining factor in fungal population studies (Högberg, Högberg and Myrold 2007; Dumbrell et al. 2010; Wan et al. 2015; Maghnia et al. 2017; Zhang et al. 2019). Available P, on the other hand, is less generally documented (Siciliano et al. 2014; Maghnia et al. 2017), although it is an important parameter for AMF communities (Dumbrell et al. 2010).
The sebacinoid community is a minor but robust component of the fungal microbiome and largely unaffected by abiotic factors
Based on our previous research in the Kisangani region (Venneman et al. 2017), we assumed that endophytic Sebacinales would represent a substantial part of the fungal community in field-sampled roots originating from the same area. Indeed, in the current study, as much as 34 sebacinoid OTUs could be retrieved, all of which belonged to the Serendipitaceae family (subgroup B). This is in concordance with previous reports that placed the majority of the recovered endophytic sequences in the same family (Selosse, Dubois and Alvarez 2009; Weiß et al. 2011; Riess et al. 2014; Verbruggen et al. 2014; López-García et al. 2016). All but one of the 12 most dominant OTUs appeared to be distributed across two well-supported clades. The putative ‘Piriformospora’ sequences could be assigned to ‘cluster 1’, which contained several endophytic sequences reported by earlier DNA-based studies (Weiß et al. 2011; Riess et al. 2014) or obtained from axenically cultured strains with endophytic properties, including S. indica, S. williamsii and the recently isolated Congolese collection (Venneman et al. 2017). On the other hand, the OTUs of ‘cluster 2’ seemed to be more strongly associated with mycorrhizal interaction types in orchids (Martos et al. 2012; Oliveira et al. 2014) than with endophytic sequences.
In contrast to the relatively large number of detected Sebacinales OTUs, the percentage share of sebacinoids in the total read count per maize and Panicum sample revealed that these endophytes naturally occur at very low abundances, ranging between 0.00% and 3.62%. This finding corroborates previous environmental studies that tend to agree on the low abundance of Sebacinales in plant roots based on the laborious molecular and microscopical detection of these endophytes in field samples (Selosse, Dubois and Alvarez 2009; Weiß et al. 2011; Garnica et al. 2013; Riess et al. 2014). More specifically, a metabarcoding approach enabled Verbruggen et al. (2014) to uncover that Sebacinales encompassed less than 1% of all reads associated with wheat roots from organically managed fields in Switzerland (0.01–0.44% per individual OTU). Similarly, Detheridge et al. (2016) recovered a Sebacina taxon from soil following a three-year cultivation of forage crops at an average abundance of 0.8% (0.4–1.6%). On the other hand, Wehner et al. (2014) listed an uncultured Serendipitaceae fungus among the top ten most frequently encountered OTUs within Asteraceae from semi-arid grassland in Germany, comprising 2.65% of all reads.
Notwithstanding their low abundance, Sebacinales appeared to be widely spread in the Congo Basin, since they could be detected in 83% of the evaluated maize and Panicum root systems, which is in line with several other reports describing that sebacinoid endophytes are ubiquitously present in diverse natural environments. For instance, Selosse, Dubois and Alvarez (2009) and Weiß et al. (2011) examined the roots of a broad spectrum of herbaceous angiosperms in temperate, tropical and subarctic regions and were able to obtain sebacinoid amplicons from 84% and 100% of the 25 and 27 plant families tested, respectively. Similarly, Riess et al. (2014) sampled economically important and co-occurring herbaceous plants under different land uses in Germany and retrieved sebacinoid sequences from approximately 65% of the examined plant families. However, at sample level, Selosse, Dubois and Alvarez (2009) and Riess et al. (2014) detected Sebacinales in only 29% and 16% of the investigated root systems, respectively, which is much lower than our detection level of 83% across maize and Panicum samples. These contrasting figures might be attributed to the fact that our sampling focused on two plant species from the same family (Poaceae), whereas in the other studies a variety of plant species was considered. Moreover, the evaluated fields in Kisangani were defined by comparable low-input management systems as opposed to sites under variable land use intensity in the other reports. Indeed, when focusing on just one plant species, Verbruggen et al. (2014) recorded sebacinoid OTUs in 73% of the investigated wheat plots under organic management and López-García et al. (2016) recovered sebacinoid sequences from 71% of the analyzed root samples of Festuca brevipila R. Tracey growing in the Mallnow natural reserve in Germany.
Interestingly, in sharp contrast to the total and the glomeromycotan communities, the sebacinoid community did not display obvious signs of sensitivity to general land use in the region. Only a minor difference in community composition was observed between both sampled plant hosts and no influence of the slash-and-burn management, the location of the field in relation to the forest, or a legacy effect of the preceding vegetation could be detected. These results are in conflict with other reports suggesting that the presence of endophytic Sebacinales might be affected by land use intensity. For instance, Garnica et al. (2013) showed that recently disturbed ecosystems in the Bavarian Alps were characterized by significant phylogenetic clustering of sebacinoid communities. Compared to conventional agriculture, Verbruggen et al. (2014) reported a higher prevalence of Sebacinales in wheat roots from organically managed fields and a strong relationship between the occurrence of these fungi and weed diversity.
Moreover, unlike the general observation that the total and the AM fungal community structures were driven by Bray-II P, soil pH and C/N ratio, neither the sebacinoid community composition nor the abundance of Sebacinales and ‘Piriformospora’ spp. appeared to be correlated with any of the examined soil variables. This finding contradicts the results of López-García et al. (2016) who demonstrated that pH is a major driver controlling the distribution of sebacinoid fungi and those of Detheridge et al. (2016) who detected a negative correlation between sebacinoid abundance and soil nitrate-N levels. However, also Garnica et al. (2013) and Verbruggen et al. (2014) were not able to link specific soil characteristics to sebacinoid composition or occurrence, respectively, and the former study hypothesized that soil parameters may only play a role in extreme habitats.
Although we should consider the possibility that Sebacinales have benefited from the prevailing low-impact agricultural conditions, the data altogether imply that sebacinoid endophytes in the sampled area are a robust group of fungi in terms of responsiveness to environmental gradients and external disturbance. This finding supports the work of Sarkar et al. (2019), who came to the same conclusion via a different approach, by showing that S. vermifera (MAFF 305830) is very resilient to biotic stress and dynamically adapts to changes in the plant host environment.
Congolese soils as a rich source of plant beneficial fungal taxa for on-farm inoculum development within an ISFM context
With a number of OTUs that varied from 458 to 1266 per examined root system, the studied agricultural area appeared to harbor an extremely rich rhizospheric community. However, approximately one third of these OTUs could not be assigned to a fungal taxon and within several orders a vast proportion of the OTUs could not be identified at the family level. The presence of such a large unspecified fraction suggests that there is a high number of microorganisms still to be described within this largely unexplored ecosystem, including strains with potentially plant growth-stimulating abilities.
From our analyses it is clear that the sampled soils contain a large pool of putatively useful fungi, which could be stimulated by proper agricultural practices to generate a taxonomically diverse inoculum of locally adapted strains for implementation within an ISFM approach. This method of on-farm inoculum production using indigenous fungi has already proven its applicability in case of AMF (Douds et al. 2005; Douds, Nagahashi and Hepperly 2010).
Besides Sebacinales, Glomeromycota strains, which are well known to engage in beneficial interactions with their host, constituted an important part of the examined fungal community, as has been discussed above. Furthermore, Trichoderma spp. were also identified as putatively relevant plant symbionts especially in the roots of maize. These organisms are among the most frequently isolated soil fungi from both temperate and tropical regions and contain several species with biocontrol and/or PGP activities (Harman et al. 2004).
Regarding other fungal taxa that could possibly be beneficial to plant growth, the presence of DSE was more difficult to evaluate as they belong to different ascomycetous orders (Jumpponen and Trappe 1998; Newsham 2011; Andrade-Linares and Franken 2013). Nevertheless, the majority of the Capnodiales OTUs was found to be allocated to Cladosporium, which has been reported as a DSE group in earlier studies (Ban et al. 2012). Within Sordariales, approximately a quarter of the reads was affiliated with Chaetomium species, presumably C. cupreum, which has known applications in plant disease control, for example as a component of the commercial broad-spectrum biological fungicide Ketomium® (Soytong et al. 2001).
In view of inoculum development, not only presence but also compatibility of different groups of beneficial organisms needs to be considered. However, as a consequence of the scarce detection of endophytic sebacinoids in natural ecosystems, information on the interaction between Sebacinales and other endophytic taxa in plant roots is largely fragmentary. Nevertheless, it has been speculated that there might be an intriguing interplay between AM and sebacinoid species. Williams (1985) frequently observed an association between multinucleate, sterile, septate fungi and AMF in pot cultures, which seemed to affect the relation between the host plant and the AM endophyte. Three of these multinucleate rhizoctonias had a teleomorph closely resembling S. vermifera Oberwinkler and one of them, DAR 29830 isolated from a Glomus fasciculatum trap culture with clover roots, was later described as S. williamsii (Basiewicz et al. 2012). Additionally, S. indica was recovered by chance from a fresh spore of the AM fungus Glomus mosseae, which had been multiplied within a maize trap culture with Indian desert soil (Verma et al. 1998). Furthermore, in our previous study we retrieved isolates, collectively designated as the S. ‘williamsii’ species complex, from trap cultures that were initially set up to maintain AMF. Root staining indicated that the presence of AM associations did not prevent Serendipita spp. from colonizing the same root piece of the sudangrass trap plant (Venneman et al. 2017). Here, using Illumina metabarcoding, we confirmed that this phenomenon also occurred in natural ecosystems: a significant positive correlation was detected between Sebacinales and members of the dominant AMF order Glomerales, especially in maize samples (Pearson's r = 0.327) and less so in Panicum roots (Pearson's r = 0.261).
In contrast, a negative interaction was apparent in case of the Hypocreaceae family, which mainly harbored Trichoderma/Hypocrea sequences, suggesting that its species are not likely to associate with sebacinoid endophytes in nature. The Trichoderma genus is well known for its biocontrol potential, which involves mycoparasitism, antibiosis, competition for nutrients and space and induction of defense responses in the plant (Harman et al. 2004). As they are important antagonists of pathogenic fungi, it might well be that they negatively interfere with some beneficial fungi, including Sebacinales. This presumption is reinforced by the work of Anith et al. (2011), who observed a negative influence of T. harzianum on the mycelial growth and the root colonization rate of S. indica in a dual culture plate assay and an in vitro dual inoculation experiment with black pepper plantlets, respectively. On the other hand, application of Trichoderma spp. seems to be compatible with AMF establishment as it has been demonstrated that Trichoderma inoculation either has a neutral (Vázquez et al. 2000) or a synergistic (Martínez-Medina, Roldán and Pascual 2011) effect on AMF colonization. Here, no significant correlations were found between Hypocreaceae and AMF families, corroborating the theory of a neutral effect.
Finally, previous studies have stated that sebacinoid diversity in roots is generally very low with a unique OTU detected in the majority of the root samples, enabling direct Sanger sequencing without preceding cloning efforts (Selosse, Dubois and Alvarez 2009; Verbruggen et al. 2014; López-García et al. 2016). However, when taking all 34 Sebacinales OTUs into account and only considering those with a minimum of two reads, we observed that the roots of maize and Panicum were regularly occupied by more than one OTU at the same time. In fact, a maximum number as high as 11 sebacinoid OTUs per evaluated root system was recorded.
Taken together, when exploring the perspectives of on-farm inoculum development, the observed competition between Trichoderma and Serendipitaceae spp. might impede positive results, whereas the combination of AMF with Serendipitaceae spp. might have significant potential.
CONCLUSIONS
Here we examined rhizospheric sebacinoids within the context of subsistence farming in the Congo Basin and demonstrated that they were widely present across smallholder farmer plots in Kisangani albeit in low abundance. In this respect, they can literally be considered as a needle in each haystack, which, in turn, raises questions regarding their ecological role. Due to difficulties in isolating and cultivating Sebacinales, insights into the ecology of sebacinoid endophytes are currently limited. Nevertheless, their demonstrated extensive diversity, associated with their symbiotic versatility, lack of host specificity and widespread distribution across diverse habitats, provides a large resource of unexplored strains that can potentially help to improve our understanding of their ecosystem functionality. Furthermore, the sebacinoid community seemed to be less susceptible to environmental and anthropogenic factors than the total fungal and glomeromycotan community, suggesting that they are robust inhabitants of plants roots. Additionally, the frequently observed association between sebacinoid and AM fungi suggests that Sebacinales might impact fundamental plant processes through interaction with other fungal groups.
In view of their implementation in an ISFM approach, we could not validate whether Panicum borders might function as a natural and undisturbed repository of sebacinoid fungi, providing inoculum to the adjacent agricultural field. The reason thereof is that soil conditions in the sampled fields and the adjacent borders turned out not to be substantially different within an agricultural context due to the predominantly low-impact management practices. An important question to be answered for ISFM application purposes is whether the low quantities of Sebacinales present in natural ecosystems possess the same PGP effects as demonstrated by cultivable strains under artificial conditions. Typically, greenhouse and in vitro inoculations use relatively large amounts of inoculum, which, in the absence of competition with other root colonizers, usually leads to a high degree of colonization. Nevertheless, preliminary data suggest that a dense colonization is not imperative for a successful symbiotic association, as significant plant growth promotion has been observed at low colonization levels and even before obvious colonization was apparent (our unpublished data).
Although more research is needed to elucidate their function and behavior in natural ecosystems, based on our data we believe that Sebacinales are promising fungi for applications within ISFM approaches in Sub-Saharan Africa, where they could assist in reducing the reliance on high-cost chemical inputs.
ACKNOWLEDGEMENTS
We thank the staff of the Faculty of Renewable Natural Resources at the University of Kisangani for their cooperation and assistance in the field. We are also grateful to the local farmers for allowing us to sample their crops. Further, we acknowledge VLIR-UOS for supervising the P1 project that focuses on the contribution of sustainable agriculture to food security in the northeastern basin of the Democratic Republic of Congo.
FUNDING
This work was supported by the Flemish Interuniversity Council–University Cooperation for Development (VLIR-UOS) within the framework of the P1 project in the Congo Basin and by a postdoctoral grant from the Research Foundation Flanders (FWO) [project 12S9418N] to CDT. The Center for International Forestry Research (CIFOR) provided additional laboratory equipment.
Conflicts of interests. None declared.