-
PDF
- Split View
-
Views
-
Cite
Cite
Graciana Willis, Ivan Nancucheo, Sabrina Hedrich, Alejandra Giaveno, Edgardo Donati, David Barrie Johnson, Enrichment and isolation of acid-tolerant sulfate-reducing microorganisms in the anoxic, acidic hot spring sediments from Copahue volcano, Argentina, FEMS Microbiology Ecology, Volume 95, Issue 12, December 2019, fiz175, https://doi.org/10.1093/femsec/fiz175
- Share Icon Share
ABSTRACT
The geothermal Copahue-Caviahue (GCC) system (Argentina) is an extreme acidic environment, dominated by the activity of Copahue volcano. Environments characterised by low pH values, such as volcanic areas, are of particular interest for the search of acidophilic microorganisms with application in biotechnological processes. In this work, sulfate-reducing microorganisms were investigated in geothermal acidic, anaerobic zones from GCC system. Sediment samples from Agua del Limón (AL1), Las Máquinas (LMa2), Las Maquinitas (LMi) and Baño 9 (B9–2, B9–3) were found to be acidic (pH values 2.1–3.0) to moderate acidic (5.1–5.2), containing small total organic carbon values, and ferric iron precipitates. The organic electron donor added to the enrichment was completely oxidised to CO2. Bacteria related to ‘Desulfobacillus acidavidus’ strain CL4 were found to be dominant (67–83% of the total number of clones) in the enrichment cultures, and their presence was confirmed by their isolation on overlay plates. Other bacteria were also detected with lower abundance (6–20% of the total number of clones), with representatives of the genera Acidithiobacillus, Sulfobacillus, Alicyclobacillus and Athalassotoga/Mesoaciditoga. These enrichment and isolates found at low pH confirm the presence of anaerobic activities in the acidic sediments from the geothermal Copahue-Caviahue system.
INTRODUCTION
SRP include members of the four taxonomic groups: (i) the δ-proteobacteria subdivision, containing diverse genera of Gram-negative, mesophilic sulfate-reducing bacteria (SRB); (ii) Gram-positive, spore-forming SRB, that can resist higher temperatures than the mesophilic group (between 40°C and 60°C; Aüllo et al. 2013); (iii) thermophilic SRB that inhabit high temperature environments, with an optimal growth temperature between 65°C and 70°C; and (iv) thermophilic sulfate-reducing archaea that thrive at temperatures above 80°C, currently represented by the genus Archaeoglobus found only in marine hydrothermal environments (Rabus et al. 2015).
For many years it was known that sulfidogenesis occurred in acidic environments even though the only known SRP were, at the time, extremely intolerant of low pH (e.g. Tuttle, Dugan and Randles 1969). The first acidophilic SRB isolated in pure culture and later described as a novel species was Desulfosporosinus acididurans strain M1T, which was obtained from a geothermal site on the Caribbean island of Montserrat and was shown to grow in pure culture within a pH range from 3.8 to 7.0 using glycerol as the electron donor (Sen and Johnson 1999; Sánchez-Andrea et al. 2015). This SRB is an ‘incomplete oxidiser’ and produces equimolar amounts of acetic acid when growing on glycerol as electron donor, though in co-culture with the type strain of Acidocella aromatica (Jones, Hedrich and Johnson 2013), the acetic acid was oxidised to CO2 and the mixed culture was consequently able to grow at lower pH than pure cultures of D. acididuransT (Kimura et al. 2006). Another acid-tolerant SRB Desulfosporosinus sp. strain GBSRB4.2, able to grow at pH 4.8, was isolated by Senko et al. (2009) in sediments from a coal mine, which could reduce sulfate, iron(III), manganese(IV) and uranium (VI). Also, Alazard et al. (2010) described a novel species, Desulfosporosinus acidiphilus, isolated from an acidic mining effluent decantation pond sediment. This species was demonstrated to be a moderately acidophile, with an optimum at pH 5.2. Rowe et al. (2007) reported that SRB other than Desulfosporosinus species, able to grow at low pH (Johnson et al. 2009), were responsible for precipitation of copper sulfide (CuS) in an abandoned copper mine in south-west Spain (Peptoccocaceae bacterium strain CL4). More recently Ňancucheo and Johnson (2012) described novel consortia of acidophilic SRB (Peptoccocaceae strain CEB3 and D. acididuransT) and other acidophiles, maintained in a sulfidogenic bioreactor that was operated at pH as low as 2.2, that were used to remove transition metals (copper, nickel, cobalt and zinc) from synthetic acidic mine waters. On the other hand, the first thermophilic acid-tolerant SRB was isolated by Mori et al. 2003 from a hot spring. This isolate, described as Thermodesulfobium narugenseT, was found to be an autotroph with an optimum growth pH between 5.5 and 6.0. Elsewhere, novel thermophilic and acid-tolerant SRB, Desulfothermobacter acidiphilusT and Thermodesulfobium acidiphilumT, were isolated from terrestrial hot springs at Kamchatka peninsula, and were reported to be autotrophic and use hydrogen as energy source (Frolov et al. 2017, 2018). Other thermophilic acid-tolerant SRB of the genus Thermodesulfobium (Thermodesulfobium sp. strain 3baa) was recently isolated from sediments from an acidic mine pit lake in Germany (Ruffel et al. 2018).
SRB are versatile in terms of growth conditions and they can develop in a range of different environmental conditions (Muyzer and Stams 2008). Extreme environments such as hot water pools, acidic hot springs, hydrothermal systems in shallow and deep sea, and mining-impacted areas are a source of sulfate reducers that can grow at elevated temperatures and low pH values. Many studies have focused on the geochemistry and microbiology of these environments not only because of their ecological implications, but also because indigenous microorganisms have potential application in many biotechnological processes, such as bioprecipitation of transition metals from industrial and mine-impacted wastewaters (Johnson 2012; Ňancucheo and Johnson 2012; Santos and Johnson 2018; González et al. 2019) and the use of microbial electrochemical systems (MESs, Pozo et al. 2017; Ni et al. 2018). Many studies where acidophilic/acid-tolerant SRB were detected, using both culture-dependent and -independent approaches, have been carried out with AMD streams, microbial mats, pit lakes (e.g. Rowe et al. 2007; Falagán et al. 2014) and acidic rivers (e.g. Sánchez-Andrea et al. 2011, 2013). Regarding volcanic environments with extreme temperature and/or pH conditions, several hot spring from the Kamchatka peninsula (Russia) and from the Yellowstone National Park were widely characterised in terms of microbial ecology (e.g. Johnson et al. 2001; Fishbain et al. 2003; Meyer-Dombard 2005; Burgess et al. 2012; Chernyh et al. 2015; Mardanov et al. 2018) and isolation of acidophilic/acid-tolerant SRB (Frolov et al. 2016, 2017, 2018). Other studies related to volcanic areas were conducted at the Tans-Mexican volcanic belt (Souza Brito et al. 2014; Medrano-Santillana et al. 2017; Prieto-Barajas et al. 2018), Narugo hot spring in Japan (Mori et al. 2003) and geothermal water from a gold mine in Japan (Hirayama et al. 2005) among others.
The geothermal Copahue-Caviahue (GCC) system is a volcanic extreme environment located in the north west of Neuquén province, Argentina (Fig. 1). Copahue volcano (37°51′S, 71°10.2′ W), 2977 m.a.s.l., is an andesitic stratovolcano with a small acidic crater lake. Its activity dominates the biogeochemistry of the environment, which is characterised by wide ranges of temperature and pH (<1–8) and the presence of heavy metals (Chiacchiarini et al. 2010). This volcanic area has been subject to studies of geology, geochemistry, volcanism and hydrotherapy over the last decades (Varekamp 2004; Pedrozo et al. 2008; Agusto et al. 2013; Chiodini et al. 2015; Roulleau et al. 2016). Most investigations on ecology, biodiversity and microbiology in this geothermal environment are relatively recent. Some of these have been focused on the isolation and characterization of the aerobic, acidophilic iron- and sulfur- oxidizing microorganisms (e.g. Acidithiobacillus ferrooxidans, Acidithiobacillus thiooxidans) due to their potential applications in biomining processes (Lavalle et al. 2005; Chiacchiarini et al. 2010; Giaveno et al. 2013; Urbieta et al. 2014, 2015; Castro and Donati 2016). However, few studies have been previously carried out with anaerobic sediments from Copahue volcano and these have tended to be restricted to water and sediments from the Rio Agrio and the recipient lake Caviahue (Wendt-Potthoff and Koschorreck 2002; Koschorreck et al. 2003) and neutrophilic SRP (Chiacchiarinni et al. 2010), even though most of the geothermal ponds have extremely low pH values.
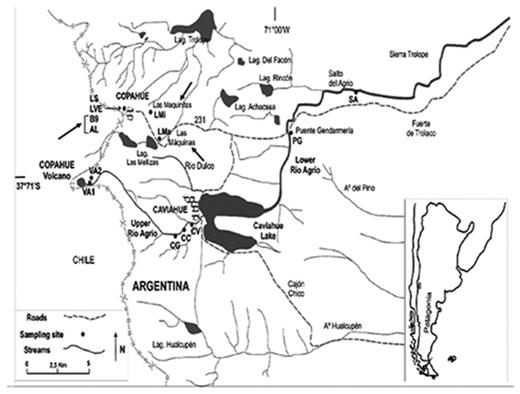
Locations of the geothermal fields within the Copahue geothermal system and sampling points (indicated with black arrows): Las Máquinas (LMa), Las Maquinitas (LMi), Baño 9 (B9–2 and B9–3) and Agua del Limón (AL). Other sample points indicated in the map, belonging to the Rio Agrio, were not included in this study (Chiacchiarini et al. 2010).
The general aim of this study was to demonstrate the presence of SRB with the ability to grow at low pH values. The current study focused on the enrichment, isolation and identification by 16S rRNA sequencing of SRB from the anaerobic zones of Copahue-Caviahue areas.
MATERIALS AND METHODS
Site description and sampling
Three sampling points were chosen for this study, representing different geothermal fields within the Copahue system (indicated by arrows in Fig. 1): Las Máquinas, Las Maquinitas and Copahue village. Las Máquinas (LMa) presents a temperature ∼40 ± 2°C and pH ∼3 and was selected for its physicochemical conditions but also for being the site less affected by infrastructure and anthropogenic activities. Las Maquinitas (LMi) is the smallest thermal spring at Copahue system and has elevated temperatures around 92 ± 2°C in the thermal ponds. Some visible sulfur deposits were observed in the coldest walls of the ponds. In recent years, this area has suffered several human impacts as its sediments are used for health treatments. Copahue village is the biggest thermal spring at Copahue system and has been greatly affected by infrastructure works during the construction of the village. The area comprises the therapeutic thermal complex, the section called Baño 9 (B9) and Agua del Limon (AL), among other thermal springs. The area is of interest due to its wide range of pH (2–7) and temperature (15–90°C), and CO2 and H2S emanate from several ponds and hot springs within it (Varekamp 2004; Pedrozo et al. 2008; Chiacchiarini et al. 2010; Chiodini et al. 2015).
At these sites, sediment samples were collected from five different acidic hot springs (Table 1), AL1, LMa2, LMi, B9–2 and B9–3, referred to in the geothermal location described above. In general, the water layer in the hot springs was approximately 10 cm deep and sediments below were collected in sterile polypropylene bottles to avoid evaporation and the entry of oxygen. Samples were kept at 4°C until processing on return to the laboratory. Water samples were also collected and filtered through 0.22 µm (pore size) cellulose acetate membrane filters (Millipore) to determine sulfate and metal concentrations.
Physicochemical parameters of Copahue samples determined in situ, dissolved iron concentration (mM) and in sediments (mg/g of dry sample).
Site . | Sample . | Location . | pH . | T [°C] . | E’ [mV] . | SO42- (mM) . | Sulfide (mM) . | DOa . | TOC (%)b . | Humidity (%) . | Fe Sediment (mg/g dry sample) . | Water (mM) . |
---|---|---|---|---|---|---|---|---|---|---|---|---|
Baño 9 | B9 (2) | 37°49’0.20’’S 71°5’50.41’’W | 5.1 | 57 | -131 | 16.87 | ND | ND | 0.8 | 63 | 16.8 | 0.26 |
B9 (3) | 37°49’0.87’’S 71°5’50.46’’W | 5.1 | 67 | -290 | 15.10 | ND | ND | 1.0 | 42 | 40.0 | 0.23 | |
Las Máquinitas | LMi | 37°49’0.9’’S 71°5’12’’W | 5.2 | 90 | -126 | 31.35 | 0.020 | 0.60 | 0.7 | 47 | 26.3 | 2.14 |
Las Máquinas | LMa2 | 37°50’2.61’’S 71°5’3.20’’W | 3.0 | 38 | -70 | 15.31 | 0.028 | 1.0 | 0.8 | 69 | 22.1 | 0.31 |
Agua del Limón | AL1 | 37°49’0.13’’S 71°5’36.18’’W | 2.1 | 41 | -200 | 27.39 | 0.011 | 0.30 | 2.2 | 53 | 25.3 | 0.25 |
Site . | Sample . | Location . | pH . | T [°C] . | E’ [mV] . | SO42- (mM) . | Sulfide (mM) . | DOa . | TOC (%)b . | Humidity (%) . | Fe Sediment (mg/g dry sample) . | Water (mM) . |
---|---|---|---|---|---|---|---|---|---|---|---|---|
Baño 9 | B9 (2) | 37°49’0.20’’S 71°5’50.41’’W | 5.1 | 57 | -131 | 16.87 | ND | ND | 0.8 | 63 | 16.8 | 0.26 |
B9 (3) | 37°49’0.87’’S 71°5’50.46’’W | 5.1 | 67 | -290 | 15.10 | ND | ND | 1.0 | 42 | 40.0 | 0.23 | |
Las Máquinitas | LMi | 37°49’0.9’’S 71°5’12’’W | 5.2 | 90 | -126 | 31.35 | 0.020 | 0.60 | 0.7 | 47 | 26.3 | 2.14 |
Las Máquinas | LMa2 | 37°50’2.61’’S 71°5’3.20’’W | 3.0 | 38 | -70 | 15.31 | 0.028 | 1.0 | 0.8 | 69 | 22.1 | 0.31 |
Agua del Limón | AL1 | 37°49’0.13’’S 71°5’36.18’’W | 2.1 | 41 | -200 | 27.39 | 0.011 | 0.30 | 2.2 | 53 | 25.3 | 0.25 |
ND: not determined
DO: Dissolved Oxygen
TOC: Total Organic Carbon (determined in 0.1 g of sediment corrected with humidity)
Physicochemical parameters of Copahue samples determined in situ, dissolved iron concentration (mM) and in sediments (mg/g of dry sample).
Site . | Sample . | Location . | pH . | T [°C] . | E’ [mV] . | SO42- (mM) . | Sulfide (mM) . | DOa . | TOC (%)b . | Humidity (%) . | Fe Sediment (mg/g dry sample) . | Water (mM) . |
---|---|---|---|---|---|---|---|---|---|---|---|---|
Baño 9 | B9 (2) | 37°49’0.20’’S 71°5’50.41’’W | 5.1 | 57 | -131 | 16.87 | ND | ND | 0.8 | 63 | 16.8 | 0.26 |
B9 (3) | 37°49’0.87’’S 71°5’50.46’’W | 5.1 | 67 | -290 | 15.10 | ND | ND | 1.0 | 42 | 40.0 | 0.23 | |
Las Máquinitas | LMi | 37°49’0.9’’S 71°5’12’’W | 5.2 | 90 | -126 | 31.35 | 0.020 | 0.60 | 0.7 | 47 | 26.3 | 2.14 |
Las Máquinas | LMa2 | 37°50’2.61’’S 71°5’3.20’’W | 3.0 | 38 | -70 | 15.31 | 0.028 | 1.0 | 0.8 | 69 | 22.1 | 0.31 |
Agua del Limón | AL1 | 37°49’0.13’’S 71°5’36.18’’W | 2.1 | 41 | -200 | 27.39 | 0.011 | 0.30 | 2.2 | 53 | 25.3 | 0.25 |
Site . | Sample . | Location . | pH . | T [°C] . | E’ [mV] . | SO42- (mM) . | Sulfide (mM) . | DOa . | TOC (%)b . | Humidity (%) . | Fe Sediment (mg/g dry sample) . | Water (mM) . |
---|---|---|---|---|---|---|---|---|---|---|---|---|
Baño 9 | B9 (2) | 37°49’0.20’’S 71°5’50.41’’W | 5.1 | 57 | -131 | 16.87 | ND | ND | 0.8 | 63 | 16.8 | 0.26 |
B9 (3) | 37°49’0.87’’S 71°5’50.46’’W | 5.1 | 67 | -290 | 15.10 | ND | ND | 1.0 | 42 | 40.0 | 0.23 | |
Las Máquinitas | LMi | 37°49’0.9’’S 71°5’12’’W | 5.2 | 90 | -126 | 31.35 | 0.020 | 0.60 | 0.7 | 47 | 26.3 | 2.14 |
Las Máquinas | LMa2 | 37°50’2.61’’S 71°5’3.20’’W | 3.0 | 38 | -70 | 15.31 | 0.028 | 1.0 | 0.8 | 69 | 22.1 | 0.31 |
Agua del Limón | AL1 | 37°49’0.13’’S 71°5’36.18’’W | 2.1 | 41 | -200 | 27.39 | 0.011 | 0.30 | 2.2 | 53 | 25.3 | 0.25 |
ND: not determined
DO: Dissolved Oxygen
TOC: Total Organic Carbon (determined in 0.1 g of sediment corrected with humidity)
Enrichment and isolation
To obtain active sulfidogenic consortia at acidic pH conditions, five sediment samples from Copahue volcano were inoculated into specific liquid medium. The growth medium was prepared and sparged with nitrogen to displace oxygen, autoclaved at 121°C for 20 minutes and dispensed into sterile 50 mL flasks. Sediment samples were added to the flasks (approximately in a 1:3 ratio), which were then sealed. Cultures were incubated at 30°C for 30 days using the AnaeroGen system (Oxoid, UK) to create an anaerobic environment. The growth medium contained: 3 mM glycerol (as electron donor), 0.1 g/L yeast extract, 4 mM ZnSO4, 100 µM FeSO4, 5 mM K2SO4, basal salts and trace elements (Ñancucheo et al. 2016), and was adjusted to pH 3.0. FeSO4 and ZnSO4 were added from 1M stock solutions prepared from the heptahydrate salts. Sulfate reducing activity was determined by measuring differences in concentrations of glycerol, sulfate and acetic acid at the beginning and after 1 month of incubation. The formation of off-white precipitates (ZnS), changes to pH and microscopic confirmation of the presence of microorganisms were also used as indicators of SRB activity.
Isolation of acidophilic SRB from the enrichment cultures was performed by streaking liquid samples from enrichment onto the overlay medium described by Ñancucheo et al. (2016) and incubated anaerobically (AnaeroGen system) at 30°C. Colonies were purified by repeated single colony isolation. Cells were resuspended in 0.05 M NaOH and 0.25% SDS at 90°C for 20 minutes, and 16S rRNA genes amplified and sequenced. Colonies were inoculated into 3 mL liquid medium and analysed for glycerol and sulfate consumption and acetate production.
Chemical analysis
At each sampling point, redox potential (E’) and pH values were measured in situ using a multiparameter Hanna HI 8424 meter coupled to a HI 1043 pH electrode and a combination redox potential electrode with an integral Ag/AgCl reference. Dissolved oxygen (DO) concentrations in the water in contact with sediments was determined using a JPB-607A portable DO analyser (Hinotek, China), and sulfide was measured with a Hach DR-850 equipment using the methylene blue technique-equivalent to USEPA method 376.2 or Standard Method 4500-S2− D (Greenberg et al. 1985). Total Organic Carbon (TOC), heavy metals and water content in sediments were determined in duplicate. Water content was determined by weight loss at 105°C when drying 1 g of sample. TOC was determined by oxidation of 6 g of sediment with potassium dichromate in acidic conditions according to the Walkley–Black procedure (Schumacher 2002). Heavy metals were measured according to the USEPA method 3050 B, using approximately 2 g of wet sediment. The amount of transition metals in sediments, expressed in milligram of metal per grams of dry sample, was calculated then using the water content determine for each sample.
For all analytical measurements, samples were filtered through 0.22 μm (pore size) cellulose acetate membranes. Sulfate was determined by turbidimetry using a Beckman DU 640 spectrophotometer (Fullerton, CA, USA) at 450 nm (Greenberg et al.1985). Glycerol was determined by an enzymatic colorimetric method using a commercial kit (TG color, Wiener Lab) following the manufacturer's instructions. Concentrations of acetic acid were measured by high performance liquid chromatography (Waters 1525) equipped with a Diode Array Detector (Waters 2996) at 210 nm and a PRP-X300 column (Hamilton) at room temperature. Sulfuric acid (0.5 mM) was used as mobile phase (2.0 mL/min). Concentration of transitions metals (in both acid digestion and water samples) were measured using an atomic absorption spectrophotometer (Shimadzu AA-6650, Shimadzu Corporation, Kyoto, Japan).
Microscopic observations
Bacterial morphology in enrichment cultures was investigated by using an optical microscope with phase contrast. Fluorescence in situ hybridisation (FISH) was performed for rapid identification of cultures at domain level. For this, 1 mL of each enrichment culture was centrifuged at 2000–3000 rpm to remove metal sulfides. Fixation of samples was performed as described by Urbieta et al. (2015) and hybridizations as described by Amann (1995) with CY3-labelled probes for the Bacteria domain EUB338 (5′ GCTGCCTCCCGTAGGAGT 3′; Amann et al. 1990) and Archaea domain (5′ GTGCTCCCCCGCCAATTCCT 3′; Stahl and Amann 1991). Pure cultures of Desulfovibrio vulgaris DSM 644 and Acidianus copahuensis DSM 29038 were used as positive controls for Bacteria and Archaea, respectively. Samples were observed with an epifluorescence microscope (Leica 2500) and images were taken using a Leica DFC 300 FX camera and its corresponding software (Leica Microscopy Systems Ltd., Heerbrugg, Switzerland).
16S rRNA gene analysis
DNA was extracted from each of the sulfidogenic cultures using the Ultra–CleanTM Soil DNA Isolation Kit (MoBio Laboratories, USA) according to the manufacturer's instruction. The starting material was prepared by filtering 5.0 mL of each culture through 0.22 um cellulose acetate membranes and the filters used for DNA extraction. The 16S rRNA genes were amplified by PCR as previously described (Okibe et al. 2003) using the universal bacterial primers 27F-CM (5′ AGAGTTTGATCMTGGCTCAG 3′) and 1387R (5′GGGCGGWGTGTACAAGGC 3′) and archaeal primers A-21F (5′ TTCCGGTTGATCCYGCCGGA 3′) and 915R (5′GTGCTCCCCCGCCAATTCCT 3′). The PCR products were checked by electrophoresis on 1% agarose gel.
16S rRNA gene clone libraries were constructed to analyse the microbial composition of the sulfidogenic consortia. Positive PCR products from each enrichment culture (pooled from three independent reactions) were purified using the Ultra Clean® PCR Clean-Up Kit (MOBIO Laboratories) and ligated using the pGEM-T Easy Vector System (Promega, USA). Before cloning DNA concentration of PCR products was determined using the NanoDrop 2000 (Thermo Fisher Scientific). The resulting ligation products were transformed into Escherichia coli strain DH5α according to the manufacturer's instructions. For each library, 32 white colonies were randomly picked and selected for the correct insert size by colony PCR with vector primers, resulting in 30 clones with the correct insert size per sample. The plasmid from each of these clones was purified using the Concert Rapid miniprep system (Life Technologies) and DNA inserts were subjected to restriction fragment length polymorphism (RFLP) analysis, using the restriction enzymes MspI and CfoI to determine the number of different 16S rRNA genes that had been cloned. The restriction fragment length polymorphism analysis revealed five restriction pattern groups, and 14 clones were sequenced in total. Plasmids of at least one or two representative clones for each restriction fragment length polymorphism pattern were sequenced using the primers SP6 and T7 from the p-GEM-T Easy Vector System. The sequencing reactions were performed by Macrogen Inc. (Seoul, Korea). Sequences were assembled with preGap and Gap 4 software from Staden Package (Bonfield, Smith and Staden 1995) and plasmid sequences were manually removed using the NCBI VecScreen tool. All sequences were checked for chimeras using the online analysis Bellerophon (Huber et al. 2004). The taxonomic identification of the sequences was performed using the public databases SILVA, EzBioCloud and GenBank (Altschul et al. 1997; Quast et al. 2013; Yoon et al. 2017). MEGA 6 was used to establish the phylogenetic affiliations of the 16S rRNA gene sequences from SRB isolates (Tamura et al. 2013). A consensus tree was generated, and bootstrap analysis was performed.
RESULTS
Characteristics and physicochemical analysis of Copahue hot springs
The main physicochemical characteristics of sediment and water samples, and concentrations of transition metals in water samples, are shown in Table 1. Total soluble Cu, Pb, Ni and Cr were measured in water in contact with sediments, but their concentrations were below the detection limits (0.02; 0.5; 0.05; 0.06 mg/L, respectively) of atomic absorption spectrophotometry. The samples were found to be acidic (AL1 and LMa2) to moderately acidic (LMi, B9–2 and B9–3) suggesting that the microbial communities present might be resistant or tolerant to acidic conditions. Temperatures varied from mesophilic (LMa2 and AL1 samples) to moderately thermophilic and hyperthermophilic conditions (B9–2, B9–3 and LMi samples). DO measurements revealed anoxic environments and a highly reducing conditions indicated by negative redox potentials. All samples contained relatively high sulfate and low dissolved sulfide concentrations in the water in contact with sediments. Furthermore, TOC results showed low values of organic matter (in general lower than 2%) compared to those reported for other volcanic environments with similar characteristics (Lantican et al. 2011). Iron was the only metal present in both water and sediments in relatively high concentrations (16–40 mg/g dry sample).
Sulfate reduction at acidic pH in enrichment from Copahue
Stoichiometry of glycerol oxidised and sulfate reduced in low pH enrichment cultures (acetic acid was not detected in any of the experiments).
Enrichment culture . | Glycerol oxidised (mM) . | Sulfate reduced (mM) . | ZnS/ H2S generation . |
---|---|---|---|
AL1 | 2.3 | 4.2 | + |
B9–2 | 2.5 | 4.4 | + |
B9–3 | 2.2 | 3.9 | + |
LMa2 | 2.2 | 3.7 | + |
LMi | 2.4 | 4.2 | + |
Enrichment culture . | Glycerol oxidised (mM) . | Sulfate reduced (mM) . | ZnS/ H2S generation . |
---|---|---|---|
AL1 | 2.3 | 4.2 | + |
B9–2 | 2.5 | 4.4 | + |
B9–3 | 2.2 | 3.9 | + |
LMa2 | 2.2 | 3.7 | + |
LMi | 2.4 | 4.2 | + |
Stoichiometry of glycerol oxidised and sulfate reduced in low pH enrichment cultures (acetic acid was not detected in any of the experiments).
Enrichment culture . | Glycerol oxidised (mM) . | Sulfate reduced (mM) . | ZnS/ H2S generation . |
---|---|---|---|
AL1 | 2.3 | 4.2 | + |
B9–2 | 2.5 | 4.4 | + |
B9–3 | 2.2 | 3.9 | + |
LMa2 | 2.2 | 3.7 | + |
LMi | 2.4 | 4.2 | + |
Enrichment culture . | Glycerol oxidised (mM) . | Sulfate reduced (mM) . | ZnS/ H2S generation . |
---|---|---|---|
AL1 | 2.3 | 4.2 | + |
B9–2 | 2.5 | 4.4 | + |
B9–3 | 2.2 | 3.9 | + |
LMa2 | 2.2 | 3.7 | + |
LMi | 2.4 | 4.2 | + |
Microscopic observations showed that enrichment cultures consisted mostly of rod-shaped and spore-forming bacteria with ellipsoidal spores causing the swelling of the cells, as common features of the enrichment cultures. Other bacterial morphologies, including smaller bacilli, were also detected in smaller numbers in all cultures, indicating the existence of mixed populations. FISH analysis revealed that in all enrichment cultures all cells stained with DAPI showed positive hybridisation with the Bacteria-specific probe. In contrast, no hybridisation was detected when the Archaea-specific probe was used, indicating that enrichment cultures where dominated by Bacteria (see Supplementary Material Figure S1, Supporting Information). Other cultures showed similar hybridisation efficiency.
Phylogenetic identification of clones from Copahue sulfidogenic enrichment cultures
None of the enrichment cultures showed positive results when Archaea-specific primers were used, which correlates with negative Archaea FISH hybridisation. On the other hand, positive amplification with Bacteria-specific primers was achieved with DNA extracted from enrichment cultures. The distribution and percentage of taxonomic units of each clone library were also calculated (Fig. 2). The 16S rRNA gene sequences from all sulfidogenic cultures were classified into three different phyla: Firmicutes (Bacilli and Clostridia classes); Thermotogae (Thermotogae class) and Proteobacteria (Gammaproteobacteria class). Although the method (cloning/sequencing) used to describe the bacterial structure and composition provides lower diversity coverage compared to high-throughput sequencing (HTS) methods, it was possible to identify the sulfate-reducing microorganisms in the enrichment cultures and other bacteria present in minor numbers. Moreover, cloning/sequencing approach generates longer sequences than the high-throughput sequencing's short reads, thereby providing a more robust phylogenetic affiliation (Medrano-Santillana et al. 2017). Biomolecular analysis of the enrichment cultures showed that members of genera reported as spore-forming sulfate reducers were found as the dominant bacteria in all enrichment cultures.
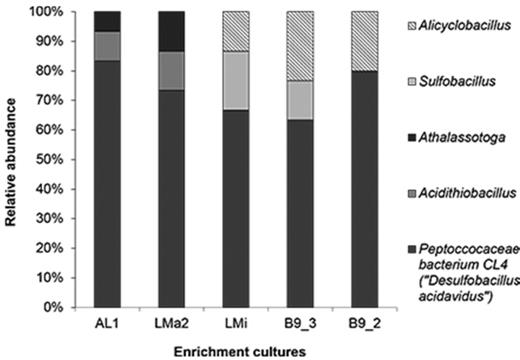
Relative abundance of 16S rRNA genes from enrichment cultures originated from Agua del Limón (AL1), Las Máquinas (LMa2), Las Maquinitas (LMi) and Baño 9 (B9–2, B9–3) hot spring sediments. Light grey bars correspond to Sulfobacillus clones; dark grey bars correspond to Peptococcaceae bacterium CL4 clones and lined bars corresponds to Alicyclobacillus clones. The affiliation is based on EZBioCloud database.
Sequences distantly related to the genus Desulfitobacterium dominated the low pH enrichment cultures AL1(83%), LMa2 (73%), LMi (67%), B9–3 (63%) and B9–2 (80%) (Fig. 2). The closest relative (98% sequence identity) was Peptoccocaceae bacterium strain CL4 (EF061086, ‘Desulfobacillus acidavidus’ strain CL4) (Table 3). ‘D. acidavidus’ was described as a mesophilic, strictly anaerobic, spore-forming bacterium able to metabolise glycerol completely to CO2 coupled to sulfate reduction (Johnson et al. 2009). This metabolism was observed in all enrichment cultures from Copahue sediments (Table 2).
Phylogenetic affiliation of sequences from low pH enrichment clones, based on EZBioCloud database.
Clone . | Accession no. . | Top-hit strain; accession no.; % similarity (EzBioCloud) . | Phylogenetic assignment Class, family, genus . |
---|---|---|---|
AL1ac_a1 | MK450144 | Peptoccocaceae bacterium CL4 (‘D. acidavidus’); EF061086; 98% | Bacteria, Firmicutes, Clostridia, Clostridiales, Peptoccocaceae, Desulfitobacterium |
B9–3ac_a8 | MK450147 | ||
LMa2ac_a17 | MK450146 | ||
B9–2ac_a2 | MK450143 | ||
LMiac_a12 | MK450145 | ||
B9–2ac_b3 | MK450148 | Alicyclobacillus tolerans strain K1T; AF137502; 99.4% | Bacteria, Firmicutes, Bacilli, Bacillales, Alicyclobacillaceae, Alicyclobacillus |
B9–3ac_b10 | MK450149 | ||
LMiac_b5 | MK450150 | ||
LMiac_c14 | MK450151 | Sulfobacillus thermotolerans strain Kr1T; DQ124681; 98.1% | Bacteria, Firmicutes, Clostridia, Clostridiales, Family XVII, Sulfobacillus |
B9–3ac_c11 | MK450152 | ||
AL1ac_d6 | MK450153 | Acidithiobacillus (At.) ferriphilus strain M20T; KR905751; 99% At. ferridurans strain ATCC 33020T; AJ278719; 98.9% | Bacteria, Proteobacteria, Gammaproteobacteria, Acidithiobacillales, Acidithiobacillaceae, Acidithiobacillus |
LMa2ac_e4 | MK450154 | At. ferridurans strain ATCC 33 020T; KR905751; 99.9% At. ferriphilus strain M20T; AJ278719; 99.1% | |
AL1ac_f9 | MK450155 | Athalassotoga saccharophila NAS-01T; AB898788; 94.9% | Bacteria; Thermotogae; Thermotogae_c; Mesoaciditogales; Mesoaciditogaceae; Athalassotoga |
LMa2ac_f16 | MK450156 |
Clone . | Accession no. . | Top-hit strain; accession no.; % similarity (EzBioCloud) . | Phylogenetic assignment Class, family, genus . |
---|---|---|---|
AL1ac_a1 | MK450144 | Peptoccocaceae bacterium CL4 (‘D. acidavidus’); EF061086; 98% | Bacteria, Firmicutes, Clostridia, Clostridiales, Peptoccocaceae, Desulfitobacterium |
B9–3ac_a8 | MK450147 | ||
LMa2ac_a17 | MK450146 | ||
B9–2ac_a2 | MK450143 | ||
LMiac_a12 | MK450145 | ||
B9–2ac_b3 | MK450148 | Alicyclobacillus tolerans strain K1T; AF137502; 99.4% | Bacteria, Firmicutes, Bacilli, Bacillales, Alicyclobacillaceae, Alicyclobacillus |
B9–3ac_b10 | MK450149 | ||
LMiac_b5 | MK450150 | ||
LMiac_c14 | MK450151 | Sulfobacillus thermotolerans strain Kr1T; DQ124681; 98.1% | Bacteria, Firmicutes, Clostridia, Clostridiales, Family XVII, Sulfobacillus |
B9–3ac_c11 | MK450152 | ||
AL1ac_d6 | MK450153 | Acidithiobacillus (At.) ferriphilus strain M20T; KR905751; 99% At. ferridurans strain ATCC 33020T; AJ278719; 98.9% | Bacteria, Proteobacteria, Gammaproteobacteria, Acidithiobacillales, Acidithiobacillaceae, Acidithiobacillus |
LMa2ac_e4 | MK450154 | At. ferridurans strain ATCC 33 020T; KR905751; 99.9% At. ferriphilus strain M20T; AJ278719; 99.1% | |
AL1ac_f9 | MK450155 | Athalassotoga saccharophila NAS-01T; AB898788; 94.9% | Bacteria; Thermotogae; Thermotogae_c; Mesoaciditogales; Mesoaciditogaceae; Athalassotoga |
LMa2ac_f16 | MK450156 |
Type strain
Phylogenetic affiliation of sequences from low pH enrichment clones, based on EZBioCloud database.
Clone . | Accession no. . | Top-hit strain; accession no.; % similarity (EzBioCloud) . | Phylogenetic assignment Class, family, genus . |
---|---|---|---|
AL1ac_a1 | MK450144 | Peptoccocaceae bacterium CL4 (‘D. acidavidus’); EF061086; 98% | Bacteria, Firmicutes, Clostridia, Clostridiales, Peptoccocaceae, Desulfitobacterium |
B9–3ac_a8 | MK450147 | ||
LMa2ac_a17 | MK450146 | ||
B9–2ac_a2 | MK450143 | ||
LMiac_a12 | MK450145 | ||
B9–2ac_b3 | MK450148 | Alicyclobacillus tolerans strain K1T; AF137502; 99.4% | Bacteria, Firmicutes, Bacilli, Bacillales, Alicyclobacillaceae, Alicyclobacillus |
B9–3ac_b10 | MK450149 | ||
LMiac_b5 | MK450150 | ||
LMiac_c14 | MK450151 | Sulfobacillus thermotolerans strain Kr1T; DQ124681; 98.1% | Bacteria, Firmicutes, Clostridia, Clostridiales, Family XVII, Sulfobacillus |
B9–3ac_c11 | MK450152 | ||
AL1ac_d6 | MK450153 | Acidithiobacillus (At.) ferriphilus strain M20T; KR905751; 99% At. ferridurans strain ATCC 33020T; AJ278719; 98.9% | Bacteria, Proteobacteria, Gammaproteobacteria, Acidithiobacillales, Acidithiobacillaceae, Acidithiobacillus |
LMa2ac_e4 | MK450154 | At. ferridurans strain ATCC 33 020T; KR905751; 99.9% At. ferriphilus strain M20T; AJ278719; 99.1% | |
AL1ac_f9 | MK450155 | Athalassotoga saccharophila NAS-01T; AB898788; 94.9% | Bacteria; Thermotogae; Thermotogae_c; Mesoaciditogales; Mesoaciditogaceae; Athalassotoga |
LMa2ac_f16 | MK450156 |
Clone . | Accession no. . | Top-hit strain; accession no.; % similarity (EzBioCloud) . | Phylogenetic assignment Class, family, genus . |
---|---|---|---|
AL1ac_a1 | MK450144 | Peptoccocaceae bacterium CL4 (‘D. acidavidus’); EF061086; 98% | Bacteria, Firmicutes, Clostridia, Clostridiales, Peptoccocaceae, Desulfitobacterium |
B9–3ac_a8 | MK450147 | ||
LMa2ac_a17 | MK450146 | ||
B9–2ac_a2 | MK450143 | ||
LMiac_a12 | MK450145 | ||
B9–2ac_b3 | MK450148 | Alicyclobacillus tolerans strain K1T; AF137502; 99.4% | Bacteria, Firmicutes, Bacilli, Bacillales, Alicyclobacillaceae, Alicyclobacillus |
B9–3ac_b10 | MK450149 | ||
LMiac_b5 | MK450150 | ||
LMiac_c14 | MK450151 | Sulfobacillus thermotolerans strain Kr1T; DQ124681; 98.1% | Bacteria, Firmicutes, Clostridia, Clostridiales, Family XVII, Sulfobacillus |
B9–3ac_c11 | MK450152 | ||
AL1ac_d6 | MK450153 | Acidithiobacillus (At.) ferriphilus strain M20T; KR905751; 99% At. ferridurans strain ATCC 33020T; AJ278719; 98.9% | Bacteria, Proteobacteria, Gammaproteobacteria, Acidithiobacillales, Acidithiobacillaceae, Acidithiobacillus |
LMa2ac_e4 | MK450154 | At. ferridurans strain ATCC 33 020T; KR905751; 99.9% At. ferriphilus strain M20T; AJ278719; 99.1% | |
AL1ac_f9 | MK450155 | Athalassotoga saccharophila NAS-01T; AB898788; 94.9% | Bacteria; Thermotogae; Thermotogae_c; Mesoaciditogales; Mesoaciditogaceae; Athalassotoga |
LMa2ac_f16 | MK450156 |
Type strain
The other clones with lower abundance (6–20%) in enrichment cultures at low pH were affiliated to Alicyclobacillus, Sulfobacillus, Acidithiobacillus (At.) and Athalassotoga/Mesoaciditoga, the latter with low sequence identity. Members of these genera have been identified in natural acidic environments and those associated with mining activities and are involved in the biogeochemical cycling of iron and sulfur. Some differences in their distribution among the enrichment cultures were observed. Sequences related to Acidithiobacillus and Athalassotoga/Mesoaciditoga (7–13%) were only present in cultures originating from samples AL1 and LMa2, the ponds with moderate temperature similar to the optimal growth temperatures of these genera. In contrast, in enrichment cultures of higher temperature ponds, B9–2, B9–3 and LMi, acidophilic, thermophilic bacteria related to Sulfobacillus and Alicyclobacillus were detected between 13% and 23%. The Acidithiobacillus genus was represented by three distinct species At. ferriduransT, At. ferriphilusT and At. ferrooxidansT. These bacteria are facultatively anaerobic, mesophilic, chemolitho-autotrophic sulfur/iron-oxidisers (some species and strains also oxidise hydrogen) that have been isolated from acidic environments with moderate temperature values, such as mine-impacted waters and geothermal sites (Kelly and Wood 2000; Hedrich and Johnson 2013; Falagán and Johnson 2016). Other sequences in AL1 and LMa2 enrichment cultures were found to be distantly related to anaerobic, moderately thermophilic fermentative bacteria Mesoaciditoga lauensisT and Athalassotoga saccharophilaT (Reysenbach et al. 2013; Itoh et al. 2016). The closest cultivated relatives of the sequences retrieved from cultures LMi, B9–2 and B9–3 samples were found in a gold-recovery plant (DQ124681), lead-zinc ore (AF137502) and geothermal sites (AY007662, AY371274, AF450135). The sequences had a high similarity to Sulfobacillus thermotolerans Kr1, Alicyclobacillus tolerans K1 and unidentified strains that have been tentatively assigned to the genus Alicyclobacillus (Johnson et al. 2001, 2003, Yahyaet al. 2008). Sulfobacillus and Alicyclobacillus are also facultatively anaerobic, acidophilic iron/sulfur oxidizing bacteria, but with the ability to grow at higher temperatures than species of Acidithiobacillus genus (20–60°C and 20–55°C, respectively) (Karavaiko et al. 2005; Bogdanova et al. 2006).
In view of their metabolic features and the fact that they are present in low relative abundance, the latter groups of microorganisms would seemingly not be contributing to the sulfidogenic activity of the enrichment cultures (Fig. 2). However, their presence might be attributable to their ability to grow and resist facultative/anaerobic conditions and the fact that they might be present in the original sample. Bacteria related to Acidithiobacillus, Sulfobacillus and Alicyclobacillus genera have been previously detected at geothermal Copahue area (Lavalle et al. 2005; Chicacchiarini et al. 2010; Urbieta et al. 2014). Also, members of Sulfobacillus and Alicyclobacillus form endospores, which can survive in environments with low oxygen conditions (Karavaiko et al. 2005; Bogdanova et al. 2006). To confirm the viability of these bacteria, a 10 mL aliquot of each enrichment culture was incubated under aerobic conditions (at 30°C and 45°C) using sulfur and iron as energy source (Johnson 1995). A decrease in pH was observed, confirming sulfur oxidation. The 16S rRNA gene sequences retrieved from these cultures, using the same methodology described for anaerobic enrichment, showed similar sequences to the ones previously described (see Supplementary Material Table 1, Supporting Information). On the other hand, in cultures from samples AL1 and LMa2 some sequences (6–13.3%, Fig. 2) were only remotely related to the recently described Athalassotoga/Mesoaciditoga genera. The only two species described for these genera were found to be moderately thermophilic (30–60°C), acid-tolerant, heterotrophic and anaerobic bacteria, able to use sulfur compounds as electron acceptor and metabolise a variety of complex organic compounds as carbon and energy sources (Itoh et al. 2016; Reysenbach et al. 2013). In addition, species related to the Thermotogae phylum were not detected in previous studies from Copahue volcano but are widespread in many anaerobic environments such as hydrothermal vents, marine sediments and terrestrial hot springs, among others (Tobler and Benning 2011; Souza Brito et al. 2014), and therefore they might also be present in the original sediments samples. The low sequence similarity to this taxon suggests the presence of a novel genus in our samples.
Isolation and phylogenetic identification of sulfidogenic bacteria
Several isolates were recovered from enrichment cultures of all samples on overlay plates at 30°C: LMa2_E2; LMi_H1; B93_E2; B92_C3; AL1_C1. These grew as ZnS-encrusted colonies on overlay plates and were Gram-positive, spore-forming, rod-shaped bacteria. All isolates oxidised glycerol completely to CO2 at the expense of sulfate reduction, as no acetate was detected after cultivation on liquid medium at pH 3.0. All isolates were closely related to Peptoccoaceae bacterium strain CL4 (EF061086, ‘D. acidavidus’; 99% 16S rRNA gene sequence similarity) (Fig. 3). These results support those found in enrichment cultures with culture-independent techniques, where Peptoccoaceae bacterium strain CL4 was implicated to be the main bacterium to be responsible for sulfate reduction.
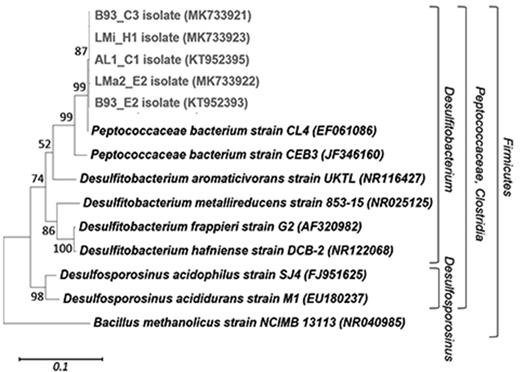
Phylogenetic affiliations of the complete 16S rRNA gene sequences (1421 bp) of five sulfidogenic isolates. The consensus phylogenetic tree was generated using MEGA with different sets of filters, which showed stable branching. Maximum Likelihood was used as the statistical method and five hundred bootstrap replicates were performed. The bar indicates a 10% estimated sequence divergence, and Bacillus methanolicus strain NCIMB 13113 was used to root the tree. The sequences from the isolates are indicated in boldface type and light grey colour. Designations after the organism names or identifiers are GenBank accession numbers.
DISCUSSION
It is generally assumed, that exposure to low pH leads to the establishment of a tolerant and resistant microbial population. However, very few acidophilic SRP have been described despite of the facts that sulfate is usually present in elevated concentrations in extremely acidic environments. A previous study performed in sediments at the naturally acidified (pH 1.8) glacial lake Caviahue at the geothermal Copahue system (Fig. 1) found high sulfate consumption and sulfide production at low pH values, predicting that microbial sulfate reduction occurs in this ecosystem (Koschorreck et al. 2003). However, until now no studies focused on the isolation of acidophilic/acid-tolerant SRPs. Indeed, the sulfidogenic enrichment cultures and, most important, pure cultures of SRBs obtained in this study revealed the existence of viable SRB in several anaerobic sediments from Copahue volcano and based on activity data are likely to be adapted to acidic conditions. This indicates that the sulfidogenic metabolic process was not adversely affected nor inhibited by the initial low pH.
Previous studies reported the enrichment and isolation of acidophilic/acid-tolerant SRB from acidic environments. Rowe et al. 2007 found Peptococcaceae bacterium strain CL4 (EF061086) in acidic sediments from an abandoned copper sulfide mine, which was physiologically characterised by Johnson et al. 2009. This isolate was distantly related to Desulfitobacterium aromaticivoransT and Desulfosporosinus acidophilusT, therefore was proposed as a new genus and specie: ‘D. acidavidus’. Later, Sanchez- Andrea et al. (2013) reported the isolation of a sulfate-reducing bacterium (isolate I-i) in Tinto River natural acid rock drainage. This isolate clustered into a group without close representatives and the closest sequence belongs to the clone HQ730640 retrieved in a former study of Tinto River sediments (Sanchez-Andrea et al. 2011). Moreover, strain CEB3 isolated by Nancucheo and Johnson (2012) from an acidic sulfidogenic bioreactor treating mine water (pH 2.4) was also classified into Peptococcaceae family. From the latter, it can be suggested that all these isolates, including the ones obtained in this study, could form a distinct taxon of acidophilic/acid tolerant SRB positioned between Desulfosporosinus and Desulfitobacterium genera. The sequences and isolates retrieved from our study have the same 16S rRNA sequence similarity with cultivated species of Desulfitobacterium than those found in the studies mentioned above, therefore falling into the same taxon (Table 2 and Fig. 3). Moreover, BLAST analysis performed with 16SrRNA sequences from SRB isolates from this study shows that they have 97% sequence similarity with clone HQ730640. To our knowledge, this study is the first report of this acidophilic/acid-tolerant group of SRB in an acidic volcanic environment. Other studies performed in acidic pools of the volcanic area of Kamchatka Peninsula regarding microbial ecology or isolation of SRB have found sequences or acidophilic SRB isolates related with Thermodesulfobium, Thermodesulfovibrio, Desulfobaba and Desulfothermobacter genera (Burgess et al. 2012; Frolov et al. 2016, 2017, 2018), which are phylogenetically very distant from our SRB.
Some of the ponds from Copahue volcano, where acidophilic/acid-tolerant SRB were isolated in this study, present low pH values (Agua del Limón pH 2.1 and Las Máquinas pH 3.0) and low organic matter content. All the acidophilic sulfate reducing strains described above were also isolated from natural environments or engineered systems at low pH and low organic matter content: 2.65–2.75 for strain CL4, 3.2 for isolate I-I and 2.4 for strain CEB3. Among the closest cultivated relatives from our isolates, Peptococcaceae bacterium CL4 is more acidophilic (capable of growing in pure culture in pH 3.0 liquid media; Johnson et al. 2009) than Desulfosporosinus acididuransT (optimum pH 5.5; Sanchez- Andrea et al. 2015). Although not fully characterised Peptococcaceae bacterium CEB3 appears to be more thermotolerant rather than mesophilic SRB and was found to be dominant in a bioreactor operating at 40°C and pH 4.0 (Santos and Johnson 2018). Therefore, one of the reasons that Copahue Peptococcaceae bacterium-solates could thrive in Copahue ponds rather than for example Desulfosporosinus acididuransT, is because of their capability to grow at higher temperatures and lower pH values. Bioremediation of acidic, metal-contaminated waters using acidophilic SRPs is attractive due to the possibility to avoid a neutralization step to contact the acidic mine water with neutrophilic SRPs. Since D. acididuransT is an incomplete oxidiser of glycerol, condition not desirable in a bioremediation context as less electrons are available for sulfate reduction and acetic acid generated increases the chemical demand of water discharged from the process. Therefore, the fact of obtaining acidophilic SRPs (related to Peptoccoaceae bacterium strain CL4) from different global locations and with the ability to oxidise completely glycerol is crucial and expand the sources to find acidophilic SRPs. On the other hand, the use of SRPs in MESs to treat mine-impacted waters at low pH and generate energy has not been widely investigated. Recently, Pozo et al. (2016, 2017) described the removal of sulfate from acid mine drainage using neutrophilic SRPs in a bioelectrochemical cell with a previous neutralization step. The tolerance to acidic conditions of the SRPs from Copahue volcano obtained in this work, make them candidates for implementing a MES where operational costs of neutralization steps will be avoided.
The presence of Peptococcaceae bacterium CL4- related species in high temperature ponds could be associated with their ability to form endospores. However, the existence of other acidophilic SRP able to grow at extreme temperatures is not excluded, since the enrichment cultures and isolation in this study were set up at 30°C while most samples had higher in situ temperatures. Also, the use of glycerol as the only electron donor could have been limited the range of SRP able to grow autotrophically. For example, Frishbain et al. (2003) enriched SRB from Yellowstone hot springs using acetate and/or H2 as electron donors. Bacteria detected includes Desulfobacula, Desulfotomaculum and Desulfomicrobium genera, who are known to be neutrophilic rather than acidophilic/acid—tolerant bacteria (Rabus et al. 2015). Moderately thermophilic, chemolitho-autotrophic and acidophilic/acid-tolerant SRMs of the genera Thermodesulfobium and Desulfothermobacter have recently been isolated and detected in acidic thermal springs and mud pools (Souza Brito et al. 2014; Frolov et al. 2017, 2018).
In the study performed by Urbieta et al. (2014) a preliminary geomicrobiological model of sulfur metabolism operating in the upper zones of Copahue hot springs was outlined. Acidophilic, sulfur-oxidizing bacteria of the genus Acidithiobacillus and Thiomonas and archaea related to Sulfolobus and A. copahuensisT were postulated to have key roles in aerobic sulfur and carbon cycles. Based on results obtained by culture-dependent approaches and the related metabolisms described, sulfate-reducers and other anaerobic bacteria reported in this study also contribute to these cycles via their anaerobic sulfur and carbon metabolisms. Elemental sulfur and other reduced sulfur compounds (S2O32− and SO32−), which are present in considerable amounts in Copahue hot springs due to continuous volcanic activity and microbial sulfur oxidation (Chiacchiarini et al. 2010), could serve as electron acceptors under anaerobic conditions for many of the microbial species in the environment. Sulfate reduction might be carried out by SRB, such as ‘Desulfobacillus’, due to their presence and isolation from acidic pH cultures originating from sediments. On the other hand, other obligate anaerobes able to reduce elemental sulfur (but not sulfate) have also been detected: Athalassotoga/Mesoaciditoga-related species may reduce elemental sulfur under anoxic conditions. These bacteria can also ferment or respire complex organic matter, generating a pool of intermediates that can be act as substrates for the metabolism of SRB (Reysenbach et al. 2013; Itoh et al. 2016). Furthermore, chemolithotrophic bacteria related to Acidithiobacillus, Sulfobacillus and Alicyclobacillus may act as primary producers contributing to the carbon cycle and oxidizing elemental sulfur or sulfide under both aerobic and anaerobic conditions competing with thermophilic archaea (Urbieta et al. 2014).
CONCLUSIONS
Several studies have been carried out at the geothermal Caviahue-Copahue system in Patagonia, Argentina, but relatively few explored its anaerobic zones. As demonstrated in this study, this extreme environment has great potential for isolation of anaerobic microorganisms, particularly sulfate-reducing bacteria. The results indicate that sulfidogenic bacteria can thrive under acidic conditions found in hot spring sediments from Las Máquinas, Las Maquinitas and Agua del Limón and Baño 9. Other anaerobic or facultative anaerobic microorganisms were also detected in enrichment cultures. Considering that some of these sequences were distantly related to cultivated species, we consider Copahue volcano hot spring sediments to be a potential source of novel anaerobic species, which require further isolation and characterization. The sulfate-reducing bacteria SRB isolated in the present study have potential applications in bioremediation processes, especially those related with the treatment of mine-impacted waters and energy generation in MESs. This study is the first report of the microbial exploration of the anaerobic zones of Caviahue-Copahue geothermal system and gives more insights in the field of SRPs thriving this type of environments.
DATA AVAILABILITY
Sequencing data have been submitted to GenBank: The 16S rRNA gene sequences determined for the isolates are deposited under accession numbers: KT952395, KT952393, MK733921, MK733922, MK733923. The 16S rRNA gene sequences determined in the clone libraries are deposited under accession numbers: MK450143-MK450156 and MN453463-MN453469 (Supplementary Material, Supporting Information).
ACKNOWLEDGEMENTS
Willis G, Giaveno A and Donati E want to specially thank to EPROTEN and Dirección de Áreas Naturales Protegidas of Neuquén province for the authorization and collaboration in accessing to the sampling sites. Willis G. wants to specially thank to Inti Gadda, Federico Giagante and Camila Castro for their contribution in processing the images.
FUNDING
This work was supported by the Agencia Nacional de Promoción Científica y Tecnológica (ANPCyT), Argentinian Government [Grant Numbers PICT 2012 0623, PICT 2013 0630 and PICT 2015 0463] and Consejo Nacional de Investigación Científica y Técnica (CONICET), Argentinian Government.
Conflicts of interest. None declared.