-
PDF
- Split View
-
Views
-
Cite
Cite
Xiaoli Shi, Shengnan Li, Fan Fan, Min Zhang, Zhen Yang, Yunfeng Yang, Mychonastes dominates the photosynthetic picoeukaryotes in Lake Poyang, a river-connected lake, FEMS Microbiology Ecology, Volume 95, Issue 1, January 2019, fiy211, https://doi.org/10.1093/femsec/fiy211
- Share Icon Share
Abstract
Lake Poyang, which is connected to the Yangtze River, is the largest freshwater lake in China and experiences large and frequent changes in water levels. The seasonal diversity and composition of photosynthetic picoeukaryotes (PPEs) in Lake Poyang were investigated from flow-cytometry-sorted samples using MiSeq high-throughput sequencing. Flow cytometric counting indicated that PPEs accounted for 97% of the total picophytoplankton abundance in spring, reaching a maximum value of 6.30 × 104 cells mL−1. PPEs in Lake Poyang showed lower diversity than those in other investigated lakes in the lower reaches of the Yangtze River and were dominated by one OTU (66.29%) affiliated with Mychonastes (Chlorophyceae). Other minor classes of PPEs were found to be sporadically abundant in specific seasons, i.e. Chrysophyceae prevailed in spring and summer, while Eustigmatophyceae was mainly present in winter. This study reports coccal green algae of Mychonastes in Lake Poyang; additionally, these algae are reportedly representative of the prominent plankton in this river-connected lake ecosystem but are often overlooked due to their lack of morphological features. Finally, the sequencing results from the sorted samples of Lake Poyang revealed that the proportion of PPEs was quite low, with an average of 36% of total reads. Many OTUs belonging to heterotrophic picoeukaryotes were also identified in the sorted samples, most of which were affiliated with terrestrial fungi, including Basidiomycota and Ascomycota. The spores of these fungi can disperse in the aquatic environment during the flood seasons, yet their effect on PPEs is still unclear.
INTRODUCTION
The essential role of autotrophic picophytoplankton (<3 µm) (APP) as a contributor to plankton biomass and to carbon and nutrient cycling has long been established (Bell and Kalff 2001). The relative importance of APP biomass and primary production declines systematically with increasing trophic status in both marine and freshwater systems (Agawin, Duarte and Agusti 2000; Bell and Kalff 2001). However, this viewpoint has been challenged by a recent study revealing that APP could represent more than 50% of the total primary production in highly eutrophic lakes with algal blooms (Li et al. 2016). This finding highlights the importance of investigating and studying APP in eutrophic lakes. APP include photosynthetic picoeukaryotes (PPEs) and prokaryotic photoautotrophs (Callieri and Stockner 2002). Picocyanobacteria (PCY) comprise the majority of picophytoplankton in large, deep, oligotrophic and mesotrophic lakes; in contrast, the maximum occurrence of PPEs is mainly associated with the conditions of spring mixing and nutrient-replete water (Weisse 1993; Callieri 2007). PPEs are much more diverse than PCY, and molecular phylogenetic methods (Vaulot et al. 2008) have identified PPEs in most algal classes.
Lake Poyang, which is connected to the Yangtze River, is the largest freshwater lake in China. Changes in water levels are frequent, and hydrology plays an important role in shaping algal communities. The frequent mixing of the lake and the eutrophic conditions favor the growth of r-strategist species, such as diatoms (Izaguirre and Vinocur 1994). Thus, the phytoplankton community structure in a river lake may share similar characteristics with that in a river (Reynolds, Descy and Padisák 1994). Although the nutrient concentrations were determined to be relatively high, the phytoplankton biomass was low (Liu et al. 2016) due to the low light availability (Wu et al. 2013). Surveys on the community structure of phytoplankton through microscopy have confirmed the dominance of Bacillariophyta in Lake Poyang. In addition to Bacillariophyta, minute coccal green algae (probably PPEs) have also been reported to be prominent in river-connected lakes (Reynolds, Descy and Padisák 1994); however, due to their small cells lacking apparent morphological features, they are usually missing from current datasets for Lake Poyang.
Diversity surveys of PPEs in freshwater aquatic systems remain scarce, and high-throughput sequencing techniques have been applied in very few of the studies. Cryptophytes, stramenopiles and alveolates were by far the most abundant supergroups that were detected, based on 18S rRNA gene sequencing of filtered samples (Simon et al. 2014); however, the results based on fluorescence in situ hybridization (FISH) experiments have confirmed the high abundance of small-sized Chlorophyta (Lepère et al. 2006; Lepère, Domaizon and Debroas 2008; Lepère et al. 2010). Recently, Chlorophyta have been found at a high abundance in freshwater ecosystems by using flow cytometric sorting and high-throughput sequencing (Li et al. 2017).
In this study, we launched a seasonal investigation on the abundance and community structure of PPEs in Lake Poyang. The objectives of this study were (1) to describe the diversity of PPEs using flow cytometric sorting and high-throughput sequencing, (2) to unveil the taxonomic affiliation of the coccal green algae, (3) to investigate the potential patterns of seasonal variation in the community of PPEs in Lake Poyang and (4) to explore how the community dynamics of PPEs are correlated with the local environmental parameters.
MATERIALS AND METHODS
Sample collection and analysis
Sampling was conducted seasonally throughout 1 year, i.e. in January, April, July and October of 2015, representing winter, spring, summer and autumn, respectively. The surface area of Lake Poyang changes greatly between the wet season (i.e. summer) and other seasons, and six sampling sites representing different lake regions were selected for investigation (Fig. 1). Thus, a total of 24 samples were collected for the investigation.
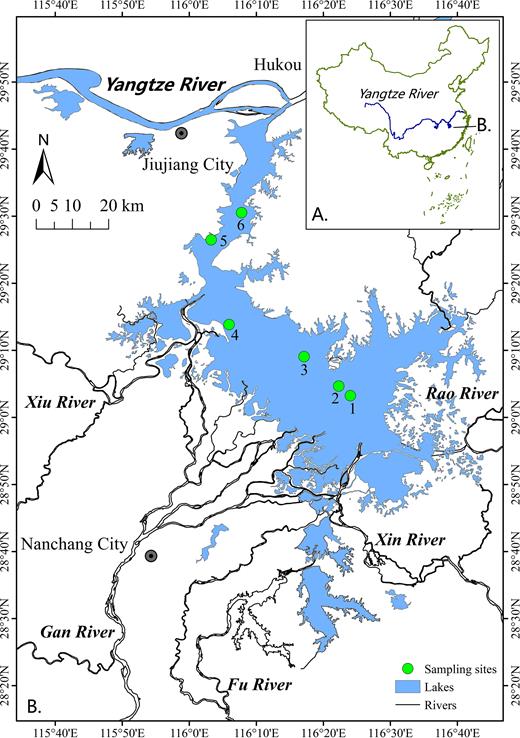
Water samples from the surface water, intermediate water and bottom water were collected and mixed to obtain water samples representing the whole water column. Environmental parameters, including pH, water temperature, dissolved oxygen and turbidity, were measured using a Hydrolab Data Sonde 5 sensor (OTT Hydromet GmbH, Germany) in situ. The water transparency was determined using a Secchi disk (SD). The suspended solids (SS), chemical oxygen demand (CODMn), chlorophyll a (Chl a) and nutrient concentrations including total nitrogen (TN), total phosphorus (TP), dissolved total nitrogen (DTN), dissolved total phosphorus (DTP), nitrate (NO3−), ammonium (NH4+) and phosphate (PO43−) were analyzed according to the APHA standard methods (Walter 1998). The concentrations of bacteria and heterotrophic flagellates (HFs) were analyzed using flow cytometry after samples were stained with SYBR Green I, according to Christaki et al. (2011) and Gong et al. (2017).
Flow cytometric sorting
The samples used for flow cytometric analysis were fixed with paraformaldehyde with a final concentration of 1% and were quickly frozen with liquid nitrogen and kept at −70°C prior to analysis. Fixation using paraformaldehyde is efficient at preserving cellular structure and pigment and has little effect on picophytoplankton fluorescence (Troussellier, Courties and Zettelmaier 1995). However, the influence of paraformaldehyde on preserving DNA for molecular analysis was species dependent and could not be predicted for natural samples (Eckford-Soper and Daugbjerg 2015). Samples were thawed on ice and passed through a 300-mesh sieve (50 μm in pore size) to avoid blocking the nozzle. All analyses were performed with a FACSJazzSE flow cytometer (Becton Dickinson, San José, CA, USA). Full details have been previously described (Li et al. 2016). Next, 3-μm standard beads (SPHERO, URFP-30–2, 3.0 μm, Spherotech, CA, USA) were used as a reference for the control size of cells. The PPE cells were then discriminated based on their higher forward scattering (FSC, a proxy for cell size) and rich far-red fluorescence from Chl a. Between 100 000 and 150 000 PPE cells were sorted in enrichment mode into Eppendorf tubes containing 180 μL of lysis buffer (Tris-HCl, pH of 8; EDTA-Na2 2 mM; Triton X-100, 1.2%) (Marie et al. 2010). All sorted samples were stored at −20°C until extraction.
DNA extraction, PCR and sequencing
DNA was extracted from the sorted PPEs using a DNeasy Blood and Tissue extraction kit (Qiagen), as previously described (Li et al. 2017). The universal eukaryote primers Ek-NSF573 (5′-CGCGGTAATTCCAGCTCCA-3′) and Ek-NSR951 (5′-TTGGYRAATGCTTTCGC-3′) were used to amplify the V4 region of the 18S rRNA (Mangot et al. 2013). PCRs were performed with a final volume of 50 µL that contained 10 µL of 5x reaction buffer, 6 µL of dNTP (2.5 mM), 0.6 µL of each primer (10 µM), 1.25 µL of Herculase II fusion DNA polymerase (Stratagene, Agilent Technologies), 15 µL of extracted DNA template and 16.55 µL of ddH2O. The amplification conditions included the following steps: 2 min at 95°C, 30 cycles of 20 s at 95°C, 20 s at 55°C and 30 s at 72°C, and a final extension at 72°C for 3 min. The PCR products were purified using a PCR purification kit (Agencourt AMPure XP, Beckman) according to the manufacturer's instructions. Amplicons were then sent for sequencing on an Illumina MiSeq platform at the Center for Genetic and Genomic Analysis (Genesky Biotechnologies Inc., Shanghai, China).
Sequence processing and taxonomic affiliation
Cutadapt (Martin 2011) was used to truncate the reads at the tail primer if one was detected. The terminal bases of the paired reads with low quality scores (< Q15) were also eliminated using the FastX toolkit. The paired reads were then merged using FLASH (Magoč and Salzberg 2011). All of the 1807 106 merged reads were cleaned and filtered against the following quality criteria: (i) a minimum sequence length of 300 bp, (ii) an expected number of errors < 2, (iii) no sequencing errors in either the forward or reverse primers, (iv) no mismatches in the barcode labels, (v) no Ns and (vi) no chimeras (checked with UCHIME) (Edgar et al. 2011). For all 24 samples, a total of 1351 661 (79.2%) cleaned sequences were retained and used for the subsequent analysis. Samples were pooled together, dereplicated and clustered to define operational taxonomic units (OTUs) using the cluster_otus algorithm and a 97% similarity threshold (Zwirglmaier et al. 2015). A representative sequence of each OTU was aligned against the SILVA 128 database (BLAST threshold e-value = e−6) for taxonomic annotation. The OTUs affiliated with Streptophyta or Metazoa were excluded from further analyses.
Statistical analyses
Statistical analyses and visualizations were implemented in the R environment (version 3.2.1, http://www.r-project.org). The relationships among the environmental variables and the abundances of the picophytoplankton groups and the dominant OTUs were assessed by Spearman correlation. Two-way (i.e. seasons and sites) analysis of variance (ANOVA) was conducted to identify the statistical temporal and spatial differences between variables. The quality of the sampling effort was assessed by the calculation of rarefaction curves (Hughes et al. 2001). The alpha diversity indexes, including OTU richness, ACE and Shannon diversity, were estimated after conducting random resampling to the minimum sample size of 33 344; this prevented possible effects from different sampling depths per sample. Redundancy analysis (RDA) was performed to test the correlations between the environmental factors and the composition of the dominant OTUs among the different samples in Lake Poyang (expressed in relative read abundance). The dominant OTUs were defined as OTUs that occurred in more than 6 samples and with mean read proportions >0.1% in each sample.
RESULTS
Environmental conditions
The main physical and chemical parameters of Lake Poyang were monitored seasonally throughout an entire year, i.e. in January, April, July and October of 2015. Most variables exhibited seasonal differences. For example, the pH was the most alkaline (pH of 9.0) and the DO concentration was the highest (13.10 mg L−1) in autumn. The SD reading was approximately 0.83 m in summer, which was much greater than the depths observed in the other three seasons. In general, the concentrations of nutrients related to nitrogen, such as total nitrogen, nitrate and ammonium, were highest in winter. The bacteria and HF concentrations reached their maximum values in spring, with 9.23 × 106 cells mL−1 and 196 cells mL−1, respectively, followed by summer (Table 1).
Environmental parameters . | Spring . | Summer . | Autumn . | Winter . |
---|---|---|---|---|
T (°C) | 14.9 ± 1.4 | 28.7 ± 2.5 | 21.8 ± 0.8 | 10.1 ± 0.7 |
pH | 7.32 ± 0.15 | 7.72 ± 0.65 | 9.70 ± 1.23 | 9.14 ± 0.95 |
DO (mg L−1) | 8.83 ± 0.57 | 9.03 ± 3.65 | 13.10 ± 3.34 | 10.87 ± 1.54 |
SD (m) | 0.34 ± 0.12 | 0.83 ± 0.48 | 0.28 ± 0.16 | 0.33 ± 0.16 |
TN (mg L−1) | 1.65 ± 0.34 | 1.44 ± 0.42 | 1.67 ± 0.80 | 3.09 ± 1.06 |
TP (mg L−1) | 0.17 ± 0.13 | 0.08 ± 0.06 | 0.10 ± 0.04 | 0.13 ± 0.06 |
NO3− (mg L−1) | 1.22 ± 0.29 | 0.82 ± 0.27 | 0.80 ± 0.20 | 1.44 ± 0.45 |
NH4+ (mg L−1) | 0.11 ± 0.10 | 0.17 ± 0.15 | 0.34 ± 0.16 | 0.89 ± 0.55 |
PO43- (mg L−1) | 0.04 ± 0.01 | 0.03 ± 0.02 | 0.04 ± 0.01 | 0.05 ± 0.01 |
Bacteria (× 106 cells mL−1) | 9.23 ± 2.45 | 6.96 ± 1.41 | 1.62 ± 0.65 | 2.80 ± 1.67 |
HF (cells mL−1) | 196 ± 97 | 78 ± 71 | 30 ± 26 | 21 ± 19 |
Environmental parameters . | Spring . | Summer . | Autumn . | Winter . |
---|---|---|---|---|
T (°C) | 14.9 ± 1.4 | 28.7 ± 2.5 | 21.8 ± 0.8 | 10.1 ± 0.7 |
pH | 7.32 ± 0.15 | 7.72 ± 0.65 | 9.70 ± 1.23 | 9.14 ± 0.95 |
DO (mg L−1) | 8.83 ± 0.57 | 9.03 ± 3.65 | 13.10 ± 3.34 | 10.87 ± 1.54 |
SD (m) | 0.34 ± 0.12 | 0.83 ± 0.48 | 0.28 ± 0.16 | 0.33 ± 0.16 |
TN (mg L−1) | 1.65 ± 0.34 | 1.44 ± 0.42 | 1.67 ± 0.80 | 3.09 ± 1.06 |
TP (mg L−1) | 0.17 ± 0.13 | 0.08 ± 0.06 | 0.10 ± 0.04 | 0.13 ± 0.06 |
NO3− (mg L−1) | 1.22 ± 0.29 | 0.82 ± 0.27 | 0.80 ± 0.20 | 1.44 ± 0.45 |
NH4+ (mg L−1) | 0.11 ± 0.10 | 0.17 ± 0.15 | 0.34 ± 0.16 | 0.89 ± 0.55 |
PO43- (mg L−1) | 0.04 ± 0.01 | 0.03 ± 0.02 | 0.04 ± 0.01 | 0.05 ± 0.01 |
Bacteria (× 106 cells mL−1) | 9.23 ± 2.45 | 6.96 ± 1.41 | 1.62 ± 0.65 | 2.80 ± 1.67 |
HF (cells mL−1) | 196 ± 97 | 78 ± 71 | 30 ± 26 | 21 ± 19 |
HF: Heterotrophic flagellates
The values are the means ± standard deviation (s.d.) of the samples in each season.
Environmental parameters . | Spring . | Summer . | Autumn . | Winter . |
---|---|---|---|---|
T (°C) | 14.9 ± 1.4 | 28.7 ± 2.5 | 21.8 ± 0.8 | 10.1 ± 0.7 |
pH | 7.32 ± 0.15 | 7.72 ± 0.65 | 9.70 ± 1.23 | 9.14 ± 0.95 |
DO (mg L−1) | 8.83 ± 0.57 | 9.03 ± 3.65 | 13.10 ± 3.34 | 10.87 ± 1.54 |
SD (m) | 0.34 ± 0.12 | 0.83 ± 0.48 | 0.28 ± 0.16 | 0.33 ± 0.16 |
TN (mg L−1) | 1.65 ± 0.34 | 1.44 ± 0.42 | 1.67 ± 0.80 | 3.09 ± 1.06 |
TP (mg L−1) | 0.17 ± 0.13 | 0.08 ± 0.06 | 0.10 ± 0.04 | 0.13 ± 0.06 |
NO3− (mg L−1) | 1.22 ± 0.29 | 0.82 ± 0.27 | 0.80 ± 0.20 | 1.44 ± 0.45 |
NH4+ (mg L−1) | 0.11 ± 0.10 | 0.17 ± 0.15 | 0.34 ± 0.16 | 0.89 ± 0.55 |
PO43- (mg L−1) | 0.04 ± 0.01 | 0.03 ± 0.02 | 0.04 ± 0.01 | 0.05 ± 0.01 |
Bacteria (× 106 cells mL−1) | 9.23 ± 2.45 | 6.96 ± 1.41 | 1.62 ± 0.65 | 2.80 ± 1.67 |
HF (cells mL−1) | 196 ± 97 | 78 ± 71 | 30 ± 26 | 21 ± 19 |
Environmental parameters . | Spring . | Summer . | Autumn . | Winter . |
---|---|---|---|---|
T (°C) | 14.9 ± 1.4 | 28.7 ± 2.5 | 21.8 ± 0.8 | 10.1 ± 0.7 |
pH | 7.32 ± 0.15 | 7.72 ± 0.65 | 9.70 ± 1.23 | 9.14 ± 0.95 |
DO (mg L−1) | 8.83 ± 0.57 | 9.03 ± 3.65 | 13.10 ± 3.34 | 10.87 ± 1.54 |
SD (m) | 0.34 ± 0.12 | 0.83 ± 0.48 | 0.28 ± 0.16 | 0.33 ± 0.16 |
TN (mg L−1) | 1.65 ± 0.34 | 1.44 ± 0.42 | 1.67 ± 0.80 | 3.09 ± 1.06 |
TP (mg L−1) | 0.17 ± 0.13 | 0.08 ± 0.06 | 0.10 ± 0.04 | 0.13 ± 0.06 |
NO3− (mg L−1) | 1.22 ± 0.29 | 0.82 ± 0.27 | 0.80 ± 0.20 | 1.44 ± 0.45 |
NH4+ (mg L−1) | 0.11 ± 0.10 | 0.17 ± 0.15 | 0.34 ± 0.16 | 0.89 ± 0.55 |
PO43- (mg L−1) | 0.04 ± 0.01 | 0.03 ± 0.02 | 0.04 ± 0.01 | 0.05 ± 0.01 |
Bacteria (× 106 cells mL−1) | 9.23 ± 2.45 | 6.96 ± 1.41 | 1.62 ± 0.65 | 2.80 ± 1.67 |
HF (cells mL−1) | 196 ± 97 | 78 ± 71 | 30 ± 26 | 21 ± 19 |
HF: Heterotrophic flagellates
The values are the means ± standard deviation (s.d.) of the samples in each season.
Dynamics of APP abundance and correlations with environmental parameters
During our investigation, APP abundance was highest in summer, with 1.02 × 105 cells mL−1, and was followed by spring and autumn, each with approximately 6.00 × 104 cells mL−1; the APP abundance dropped to its lowest value in winter (i.e. 1.11 × 104 cells mL−1). The abundance of PPEs reached its maximum value in spring, with 6.30 × 104 cells mL−1, followed by summer, autumn and winter. PPEs were the major contributors to the total picophytoplankton abundance in spring and winter, accounting for 97% and 77%, respectively. In contrast, PCY were dominant in summer and autumn (Fig. 2). The abundance of PPEs was positively related to the SD reading and the abundances of bacteria and HFs but negatively correlated with pH, ammonium and phosphate. In contrast, temperature seemed to be the key factor affecting the concentration of PCY, and it was positively related to PCY throughout the year (Table 2).
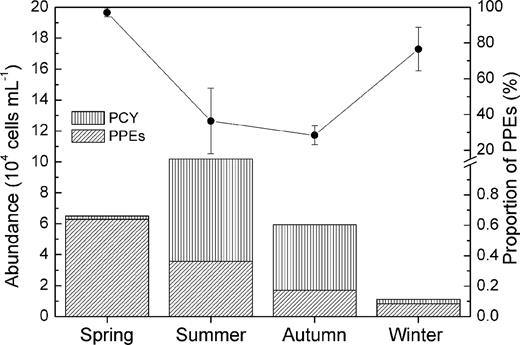
The abundance composition of photosynthetic picoeukaryotes and picocyanobacterial (the columns) measured using flow cytometric counting, and the percentage of PPEs (the line) relative to total picophytoplankton abundance.
Spearman correlations of picophytoplankton abundances with environmental variables (n = 24).
. | PCY . | PPEs . | PPE% . |
---|---|---|---|
T | 0.57** | 0.12 | -0.64** |
pH | -0.16 | -0.38** | -0.10 |
SD | 0.13 | 0.24** | -0.07 |
TN | -0.20* | -0.16 | 0.31** |
NO3− | -0.28** | -0.05 | 0.45** |
NH4+ | -0.28** | -0.26** | 0.26** |
TP | -0.13 | 0.07 | 0.22* |
PO43− | -0.26** | -0.18 | 0.25** |
Bacteria | 0.14 | 0.31** | 0.05 |
HNF | -0.07 | 0.40** | 0.32** |
. | PCY . | PPEs . | PPE% . |
---|---|---|---|
T | 0.57** | 0.12 | -0.64** |
pH | -0.16 | -0.38** | -0.10 |
SD | 0.13 | 0.24** | -0.07 |
TN | -0.20* | -0.16 | 0.31** |
NO3− | -0.28** | -0.05 | 0.45** |
NH4+ | -0.28** | -0.26** | 0.26** |
TP | -0.13 | 0.07 | 0.22* |
PO43− | -0.26** | -0.18 | 0.25** |
Bacteria | 0.14 | 0.31** | 0.05 |
HNF | -0.07 | 0.40** | 0.32** |
*indicated significance at 0.05 level; ** indicated significance at 0.01.
Spearman correlations of picophytoplankton abundances with environmental variables (n = 24).
. | PCY . | PPEs . | PPE% . |
---|---|---|---|
T | 0.57** | 0.12 | -0.64** |
pH | -0.16 | -0.38** | -0.10 |
SD | 0.13 | 0.24** | -0.07 |
TN | -0.20* | -0.16 | 0.31** |
NO3− | -0.28** | -0.05 | 0.45** |
NH4+ | -0.28** | -0.26** | 0.26** |
TP | -0.13 | 0.07 | 0.22* |
PO43− | -0.26** | -0.18 | 0.25** |
Bacteria | 0.14 | 0.31** | 0.05 |
HNF | -0.07 | 0.40** | 0.32** |
. | PCY . | PPEs . | PPE% . |
---|---|---|---|
T | 0.57** | 0.12 | -0.64** |
pH | -0.16 | -0.38** | -0.10 |
SD | 0.13 | 0.24** | -0.07 |
TN | -0.20* | -0.16 | 0.31** |
NO3− | -0.28** | -0.05 | 0.45** |
NH4+ | -0.28** | -0.26** | 0.26** |
TP | -0.13 | 0.07 | 0.22* |
PO43− | -0.26** | -0.18 | 0.25** |
Bacteria | 0.14 | 0.31** | 0.05 |
HNF | -0.07 | 0.40** | 0.32** |
*indicated significance at 0.05 level; ** indicated significance at 0.01.
Diversity and composition of PPE assemblages
The diversity of PPEs was described based on a total of 1351 661 quality-filtered reads grouped into 359 OTUs. Almost all rarefaction curves reached saturation, suggesting that microbial diversity had been sufficiently detected (Fig. S1, Supporting Information). The alpha diversity indexes were highly variable in Lake Poyang. After resampling, the observed number of OTUs ranged from 50 to 110, with a mean of 76. The Shannon diversity index was 2.6 on average but varied between 0.7 and 3.7. The OTU richness was highest in summer and lowest in spring. The Shannon diversity index value averaged 3.2 in summer and winter, but the value was lowest in autumn (Fig. 3).
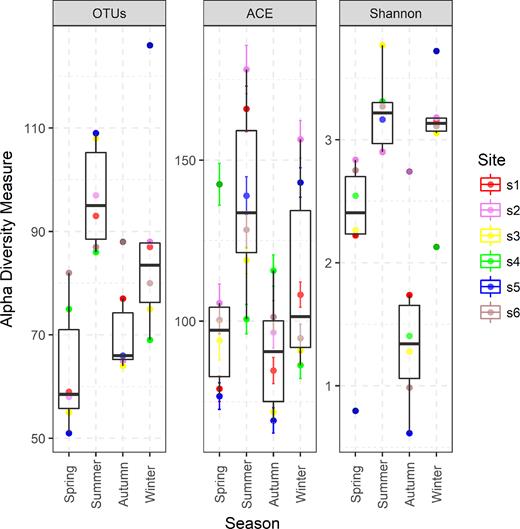
Observed OTU numbers and Shannon diversity indexes in Lake Poyang. The values were calculated after a random re-sampling to the minimum sample size of 33 344. ‘s1’ represents site 1, ‘s2’ represents site 2, and so on.
Interestingly, the number of sequences affiliated with PPEs was quite low. PPEs contributed to 37.78% of reads and represented only 122 OTUs, while nonpigmented picoeukaryotes accounted for 62.57% of reads and represented 227 OTUs. The proportion of PPEs to total sequences was very uneven. In spring, PPEs accounted for fewer than 7% of all sequences. In contrast, in autumn, PPEs accounted for more than 60% of sequences from all sample sites except one (Fig. 2, Supporting Information). The taxonomic composition of the PPEs retrieved from Lake Poyang is shown in Table 1 (Supporting Information). When compared at a high taxonomic level (i.e. phylum or class), Chlorophyta represented most of the PPE diversity. More than 95% of the Chlorophyta OTUs were related to Chlorophyceae in autumn, when the relative abundance of PPE OTUs was maximized, but the diversity value was at its minimum. During the other seasons, Chlorophyceae was also dominant; however, other classes were also observed. During spring, Mediophyceae, Trebouxiophyceae and Dinophyceae greatly contributed to PPEs at certain sampling sites. Cryptophyceae was mainly observed during summer and winter, while Eustigmatophyceae was mainly observed during winter (Fig. 4).
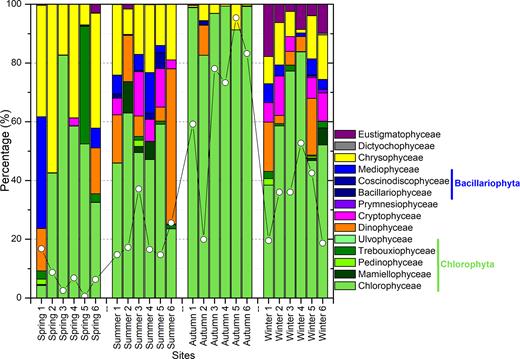
Taxonomic composition of PPEs from the sorted samples of Lake Poyang based on relative abundance of reads assigned to each group. The lines with white circles indicate the relative abundance of reads belonged to PPEs taxa in each sample.
More distinct patterns emerged at the OTU level. Abundant OTUs were defined as OTUs that occurred in more than six samples and with mean read proportions >0.1% in each sample. Thirty-six abundant OTUs were sorted out, and these represented approximately 89% of reads retrieved from Lake Poyang. The nine dominant OTUs with relative abundances greater than 1% are listed in Table 2 (Supporting Information). The most dominant OTU (OTU86) was affiliated with Mychonastes sp., which accounted for more than 80% of reads at some sampling sites, especially during autumn. Mychonastes was also dominant during winter (Fig. 5). Additionally, there were some abundant OTUs affiliated with autotrophic stramenopiles and other groups of PPEs (Fig. 3, Supporting Information ), such as Eustigmatophyceae, Dinophyceae and Cryptophyceae.
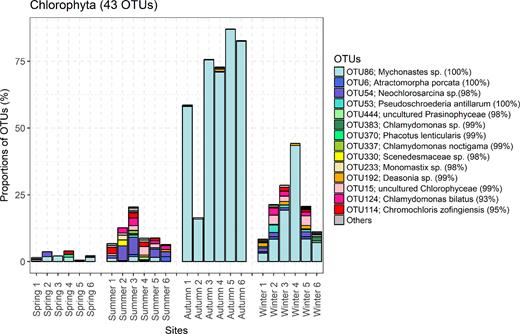
Distribution patterns of OTUs within Chlorophyta in Lake Poyang across all seasons and sites. Only part of the abundant OTUs are presented with colors. Their classifications based on blast against NCBI reference database and % sequences identity with the closest relative sequence are shown in the legend.
A large number of OTUs associated with heterotrophic picoeukaryotes were revealed. Fungi were found to be abundant in samples collected in Lake Poyang, and most observed fungi corresponded to Ascomycota and Basidiomycota; only a few sequences were affiliated with Chytridiomycota. Additionally, sequences affiliated with Ciliophora, Perkinsidae and Cercozoa were retrieved. There was no apparent seasonal distributional pattern for these heterotrophic picoeukaryotes (Fig. 6).
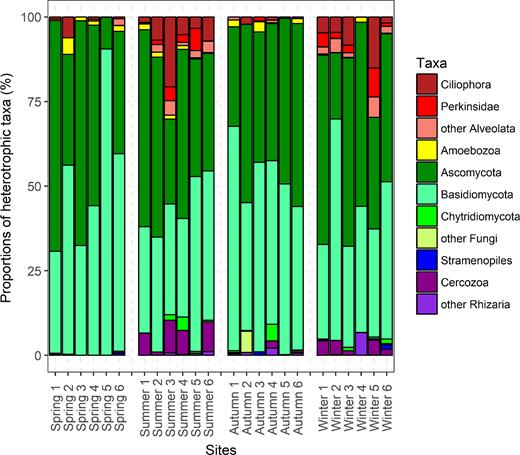
Taxonomic composition of heterotrophic picoeukaryotes from the sorted samples of Lake Poyang based on relative abundance of reads assigned to each group.
Correlation between environmental factors and dominant OTUs
RDA between the environmental factors and the 36 dominant OTUs showed that the composition of the dominant OTUs was significantly correlated with environmental factors (Fig. 7). The samples from each season were mostly clustered together, especially samples from summer and autumn, which indicated there was a similar composition of dominant OTUs within the same season. Spring samples were characterized by high concentrations of bacteria and HFs and low pH values. In summer, samples were mostly associated with high temperature, the SD reading and bacterial concentrations. However, samples collected in autumn were strongly affiliated with high DO concentrations and pH values.
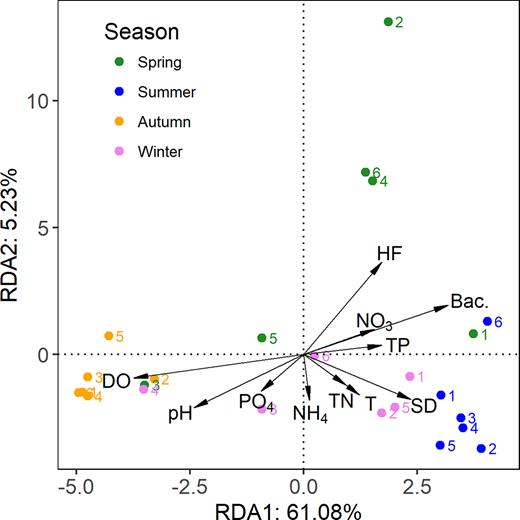
Redundancy analysis (RDA) biplots of samples and environmental parameters based on the relative abundances of the dominant OTUs in Lake Poyang.
The relationships between the environmental parameters and the top nine most dominant OTUs are listed in Table 3. Unlike the abundances of PPEs, which were significantly related to temperature, the community of the dominant PPE OTUs showed a limited relationship with temperature. For example, the overwhelmingly dominant OTU86 was significantly positively related to pH, the SD reading, concentrations of DO and bacterial values but negatively related to the concentrations of HFs. Nutrient concentrations did not seem to have any significant influence on OTU86. In addition, DO was significantly positively or negatively correlated with five of the nine most dominant OTUs (Table 3).
The spearman relationship and their corresponding significance values between environmental parameters and the top nine dominant OTUs of PPEs (n = 24).
. | . | T . | pH . | SD . | TN . | TP . | NO3− . | NH4+ . | PO43− . | DO . | Bac. . | HF . |
---|---|---|---|---|---|---|---|---|---|---|---|---|
OTU86 | r | 0.312 | 0.421 | 0.445 | 0.169 | 0.221 | 0.231 | 0.181 | 0.368 | 0.690 | 0.582 | -0.418 |
p | 0.137 | 0.041 | 0.029 | 0.429 | 0.299 | 0.278 | 0.396 | 0.077 | 0.000 | 0.003 | 0.042 | |
OTU54 | r | 0.276 | 0.425 | 0.291 | 0.143 | 0.136 | 0.291 | 0.082 | 0.280 | 0.582 | 0.345 | 0.193 |
p | 0.192 | 0.039 | 0.167 | 0.505 | 0.526 | 0.168 | 0.703 | 0.186 | 0.003 | 0.099 | 0.367 | |
OTU209 | r | 0.359 | 0.069 | 0.381 | 0.174 | 0.151 | 0.046 | 0.268 | 0.130 | 0.283 | 0.031 | 0.137 |
p | 0.085 | 0.750 | 0.066 | 0.416 | 0.482 | 0.831 | 0.205 | 0.546 | 0.181 | 0.887 | 0.524 | |
OTU15 | r | 0.098 | 0.115 | 0.328 | 0.378 | 0.209 | 0.150 | 0.084 | 0.148 | 0.229 | 0.154 | -0.155 |
p | 0.649 | 0.591 | 0.118 | 0.069 | 0.327 | 0.485 | 0.696 | 0.491 | 0.281 | 0.472 | 0.470 | |
OTU56 | r | 0.143 | 0.428 | 0.349 | 0.070 | 0.103 | 0.266 | 0.109 | 0.193 | 0.620 | 0.327 | 0.170 |
p | 0.505 | 0.037 | 0.094 | 0.747 | 0.630 | 0.209 | 0.613 | 0.366 | 0.001 | 0.118 | 0.428 | |
OTU124 | r | 0.163 | 0.016 | 0.342 | 0.292 | 0.032 | 0.369 | 0.111 | 0.069 | 0.451 | 0.000 | -0.161 |
p | 0.447 | 0.939 | 0.102 | 0.166 | 0.883 | 0.076 | 0.607 | 0.748 | 0.027 | 1.000 | 0.453 | |
OTU346 | r | 0.397 | 0.332 | 0.341 | 0.706 | 0.120 | 0.486 | 0.619 | 0.320 | 0.034 | 0.137 | -0.424 |
p | 0.054 | 0.113 | 0.103 | 0.000 | 0.577 | 0.016 | 0.001 | 0.128 | 0.874 | 0.524 | 0.039 | |
OTU114 | r | 0.120 | 0.343 | 0.423 | 0.186 | 0.102 | 0.051 | 0.192 | 0.180 | 0.375 | 0.494 | 0.178 |
p | 0.577 | 0.101 | 0.039 | 0.385 | 0.636 | 0.812 | 0.369 | 0.401 | 0.071 | 0.014 | 0.406 | |
OTU204 | r | 0.134 | 0.078 | 0.282 | 0.582 | 0.255 | 0.303 | 0.018 | 0.129 | 0.482 | 0.384 | 0.144 |
p | 0.534 | 0.716 | 0.182 | 0.003 | 0.228 | 0.150 | 0.934 | 0.548 | 0.017 | 0.064 | 0.503 |
. | . | T . | pH . | SD . | TN . | TP . | NO3− . | NH4+ . | PO43− . | DO . | Bac. . | HF . |
---|---|---|---|---|---|---|---|---|---|---|---|---|
OTU86 | r | 0.312 | 0.421 | 0.445 | 0.169 | 0.221 | 0.231 | 0.181 | 0.368 | 0.690 | 0.582 | -0.418 |
p | 0.137 | 0.041 | 0.029 | 0.429 | 0.299 | 0.278 | 0.396 | 0.077 | 0.000 | 0.003 | 0.042 | |
OTU54 | r | 0.276 | 0.425 | 0.291 | 0.143 | 0.136 | 0.291 | 0.082 | 0.280 | 0.582 | 0.345 | 0.193 |
p | 0.192 | 0.039 | 0.167 | 0.505 | 0.526 | 0.168 | 0.703 | 0.186 | 0.003 | 0.099 | 0.367 | |
OTU209 | r | 0.359 | 0.069 | 0.381 | 0.174 | 0.151 | 0.046 | 0.268 | 0.130 | 0.283 | 0.031 | 0.137 |
p | 0.085 | 0.750 | 0.066 | 0.416 | 0.482 | 0.831 | 0.205 | 0.546 | 0.181 | 0.887 | 0.524 | |
OTU15 | r | 0.098 | 0.115 | 0.328 | 0.378 | 0.209 | 0.150 | 0.084 | 0.148 | 0.229 | 0.154 | -0.155 |
p | 0.649 | 0.591 | 0.118 | 0.069 | 0.327 | 0.485 | 0.696 | 0.491 | 0.281 | 0.472 | 0.470 | |
OTU56 | r | 0.143 | 0.428 | 0.349 | 0.070 | 0.103 | 0.266 | 0.109 | 0.193 | 0.620 | 0.327 | 0.170 |
p | 0.505 | 0.037 | 0.094 | 0.747 | 0.630 | 0.209 | 0.613 | 0.366 | 0.001 | 0.118 | 0.428 | |
OTU124 | r | 0.163 | 0.016 | 0.342 | 0.292 | 0.032 | 0.369 | 0.111 | 0.069 | 0.451 | 0.000 | -0.161 |
p | 0.447 | 0.939 | 0.102 | 0.166 | 0.883 | 0.076 | 0.607 | 0.748 | 0.027 | 1.000 | 0.453 | |
OTU346 | r | 0.397 | 0.332 | 0.341 | 0.706 | 0.120 | 0.486 | 0.619 | 0.320 | 0.034 | 0.137 | -0.424 |
p | 0.054 | 0.113 | 0.103 | 0.000 | 0.577 | 0.016 | 0.001 | 0.128 | 0.874 | 0.524 | 0.039 | |
OTU114 | r | 0.120 | 0.343 | 0.423 | 0.186 | 0.102 | 0.051 | 0.192 | 0.180 | 0.375 | 0.494 | 0.178 |
p | 0.577 | 0.101 | 0.039 | 0.385 | 0.636 | 0.812 | 0.369 | 0.401 | 0.071 | 0.014 | 0.406 | |
OTU204 | r | 0.134 | 0.078 | 0.282 | 0.582 | 0.255 | 0.303 | 0.018 | 0.129 | 0.482 | 0.384 | 0.144 |
p | 0.534 | 0.716 | 0.182 | 0.003 | 0.228 | 0.150 | 0.934 | 0.548 | 0.017 | 0.064 | 0.503 |
The spearman relationship and their corresponding significance values between environmental parameters and the top nine dominant OTUs of PPEs (n = 24).
. | . | T . | pH . | SD . | TN . | TP . | NO3− . | NH4+ . | PO43− . | DO . | Bac. . | HF . |
---|---|---|---|---|---|---|---|---|---|---|---|---|
OTU86 | r | 0.312 | 0.421 | 0.445 | 0.169 | 0.221 | 0.231 | 0.181 | 0.368 | 0.690 | 0.582 | -0.418 |
p | 0.137 | 0.041 | 0.029 | 0.429 | 0.299 | 0.278 | 0.396 | 0.077 | 0.000 | 0.003 | 0.042 | |
OTU54 | r | 0.276 | 0.425 | 0.291 | 0.143 | 0.136 | 0.291 | 0.082 | 0.280 | 0.582 | 0.345 | 0.193 |
p | 0.192 | 0.039 | 0.167 | 0.505 | 0.526 | 0.168 | 0.703 | 0.186 | 0.003 | 0.099 | 0.367 | |
OTU209 | r | 0.359 | 0.069 | 0.381 | 0.174 | 0.151 | 0.046 | 0.268 | 0.130 | 0.283 | 0.031 | 0.137 |
p | 0.085 | 0.750 | 0.066 | 0.416 | 0.482 | 0.831 | 0.205 | 0.546 | 0.181 | 0.887 | 0.524 | |
OTU15 | r | 0.098 | 0.115 | 0.328 | 0.378 | 0.209 | 0.150 | 0.084 | 0.148 | 0.229 | 0.154 | -0.155 |
p | 0.649 | 0.591 | 0.118 | 0.069 | 0.327 | 0.485 | 0.696 | 0.491 | 0.281 | 0.472 | 0.470 | |
OTU56 | r | 0.143 | 0.428 | 0.349 | 0.070 | 0.103 | 0.266 | 0.109 | 0.193 | 0.620 | 0.327 | 0.170 |
p | 0.505 | 0.037 | 0.094 | 0.747 | 0.630 | 0.209 | 0.613 | 0.366 | 0.001 | 0.118 | 0.428 | |
OTU124 | r | 0.163 | 0.016 | 0.342 | 0.292 | 0.032 | 0.369 | 0.111 | 0.069 | 0.451 | 0.000 | -0.161 |
p | 0.447 | 0.939 | 0.102 | 0.166 | 0.883 | 0.076 | 0.607 | 0.748 | 0.027 | 1.000 | 0.453 | |
OTU346 | r | 0.397 | 0.332 | 0.341 | 0.706 | 0.120 | 0.486 | 0.619 | 0.320 | 0.034 | 0.137 | -0.424 |
p | 0.054 | 0.113 | 0.103 | 0.000 | 0.577 | 0.016 | 0.001 | 0.128 | 0.874 | 0.524 | 0.039 | |
OTU114 | r | 0.120 | 0.343 | 0.423 | 0.186 | 0.102 | 0.051 | 0.192 | 0.180 | 0.375 | 0.494 | 0.178 |
p | 0.577 | 0.101 | 0.039 | 0.385 | 0.636 | 0.812 | 0.369 | 0.401 | 0.071 | 0.014 | 0.406 | |
OTU204 | r | 0.134 | 0.078 | 0.282 | 0.582 | 0.255 | 0.303 | 0.018 | 0.129 | 0.482 | 0.384 | 0.144 |
p | 0.534 | 0.716 | 0.182 | 0.003 | 0.228 | 0.150 | 0.934 | 0.548 | 0.017 | 0.064 | 0.503 |
. | . | T . | pH . | SD . | TN . | TP . | NO3− . | NH4+ . | PO43− . | DO . | Bac. . | HF . |
---|---|---|---|---|---|---|---|---|---|---|---|---|
OTU86 | r | 0.312 | 0.421 | 0.445 | 0.169 | 0.221 | 0.231 | 0.181 | 0.368 | 0.690 | 0.582 | -0.418 |
p | 0.137 | 0.041 | 0.029 | 0.429 | 0.299 | 0.278 | 0.396 | 0.077 | 0.000 | 0.003 | 0.042 | |
OTU54 | r | 0.276 | 0.425 | 0.291 | 0.143 | 0.136 | 0.291 | 0.082 | 0.280 | 0.582 | 0.345 | 0.193 |
p | 0.192 | 0.039 | 0.167 | 0.505 | 0.526 | 0.168 | 0.703 | 0.186 | 0.003 | 0.099 | 0.367 | |
OTU209 | r | 0.359 | 0.069 | 0.381 | 0.174 | 0.151 | 0.046 | 0.268 | 0.130 | 0.283 | 0.031 | 0.137 |
p | 0.085 | 0.750 | 0.066 | 0.416 | 0.482 | 0.831 | 0.205 | 0.546 | 0.181 | 0.887 | 0.524 | |
OTU15 | r | 0.098 | 0.115 | 0.328 | 0.378 | 0.209 | 0.150 | 0.084 | 0.148 | 0.229 | 0.154 | -0.155 |
p | 0.649 | 0.591 | 0.118 | 0.069 | 0.327 | 0.485 | 0.696 | 0.491 | 0.281 | 0.472 | 0.470 | |
OTU56 | r | 0.143 | 0.428 | 0.349 | 0.070 | 0.103 | 0.266 | 0.109 | 0.193 | 0.620 | 0.327 | 0.170 |
p | 0.505 | 0.037 | 0.094 | 0.747 | 0.630 | 0.209 | 0.613 | 0.366 | 0.001 | 0.118 | 0.428 | |
OTU124 | r | 0.163 | 0.016 | 0.342 | 0.292 | 0.032 | 0.369 | 0.111 | 0.069 | 0.451 | 0.000 | -0.161 |
p | 0.447 | 0.939 | 0.102 | 0.166 | 0.883 | 0.076 | 0.607 | 0.748 | 0.027 | 1.000 | 0.453 | |
OTU346 | r | 0.397 | 0.332 | 0.341 | 0.706 | 0.120 | 0.486 | 0.619 | 0.320 | 0.034 | 0.137 | -0.424 |
p | 0.054 | 0.113 | 0.103 | 0.000 | 0.577 | 0.016 | 0.001 | 0.128 | 0.874 | 0.524 | 0.039 | |
OTU114 | r | 0.120 | 0.343 | 0.423 | 0.186 | 0.102 | 0.051 | 0.192 | 0.180 | 0.375 | 0.494 | 0.178 |
p | 0.577 | 0.101 | 0.039 | 0.385 | 0.636 | 0.812 | 0.369 | 0.401 | 0.071 | 0.014 | 0.406 | |
OTU204 | r | 0.134 | 0.078 | 0.282 | 0.582 | 0.255 | 0.303 | 0.018 | 0.129 | 0.482 | 0.384 | 0.144 |
p | 0.534 | 0.716 | 0.182 | 0.003 | 0.228 | 0.150 | 0.934 | 0.548 | 0.017 | 0.064 | 0.503 |
DISCUSSION
PPE abundance and its contribution to picophytoplankton
Previous studies have revealed that the population abundance of PPEs (<3 um) is generally one order of magnitude lower than that of PCY in some meso-oligotrophic lakes (Søndergaard 1991). However, PPEs have also been found to constitute an important fraction of APP in water bodies including the near-shore station of Lake Baikal, boreal humic lakes, humic Danish lakes, acidic dystrophic lakes and eutrophic shallow lakes (Jasser and Arvola 2003; Katano et al. 2005; Izaguirre et al. 2014; Li et al. 2016; Mózes, Présing and Vörös 2006). Our results showed that in Lake Poyang, PPEs were prevalent during spring and winter, while PCY were major APP contributors during summer and autumn; these results are consistent with the results obtained from Lake Taihu and Lake Chaohu (Li et al. 2016). All three of these lakes are eutrophic. Temperature was the main influential factor on the composition of major APP groups. Additionally, PCY cell density was positively correlated with temperature, and PPEs were replaced by PCY when temperature was high (Li et al. 2016). The pH was negatively related to the concentration of PPEs, whereas PCY has been reported to rapidly decrease with a decline in pH in lakes (Stockner and Shortreed 1991). In addition, our results showed that PPE abundance was positively related to HFs, which indicated that PPEs seem to be less sensitive to grazing than are PCY. A reduction in grazing pressure is another possible explanation for APP dominance in shallow, turbid waters (Fogg 1995). Light availability is also important for regulating the structure of APP (Jasser and Arvola 2003). The abundance of PPEs was significantly and negatively related to the SD reading, implying that PPEs can adapt better to the low-light conditions than can PCY. A significant and positive correlation between the mean light attenuation coefficient and the proportion of eukaryotic biomass of picoplankton has also been reported (Pick and Agbeti 1991). Lake Poyang exhibits high clay turbidity, and light availability is the major factor limiting the growth of phytoplankton (Wu et al. 2014). Thus, PPEs might replace larger phytoplankton to play a major role in Lake Poyang with high turbidity.
Low diversity of PPEs in Lake Poyang
The river-lake interaction pattern is the driving force of the environmental variation in the Lake Poyang ecosystem. Thus, the phytoplankton community structure might also show similar characteristics to the phytoplankton community structure of the river, since an important exchange of species exists between the lake and river (Izaguirre and Vinocur 1994; Reynolds, Descy and Padisák 1994). A previous study revealed that the river had lower species richness than did the lake; this conclusion was based on the taxonomic and morphological composition of phytoplankton, which was dominated by typical fluvial species of diatoms (i.e. r-strategists) (Garcia 1997). Consistently, the values for the alpha diversity and Shannon diversity index of PPEs were lower in Lake Poyang than in Lake Taihu and other investigated lakes (Li et al. 2017; Shi et al. 2018).
Chlorophyceae were the overwhelmingly dominant PPE taxa in Lake Poyang, which showed little diversity and were mainly attributed to the dominance of one OTU (OTU86) belonging to Mychonastes. In contrast, Chrysophyceae, which were the most diverse group of PPEs, represented only 8% of the total PPE sequences and fell within 40 OTUs. However, PPE community structure appeared to be more diverse in other lakes along the lower reaches of the Yangtze River, where Chlorophyceae, Chrysophyceae, Bacillariophyta and Cryptophyceae were equally abundant (Shi et al. 2018). The utilization of flow cytometric sorting greatly increased the proportion of Chlorophyceae sequences. However, Cryptophyta, Chrysophyceae, Dinophyceae and Haptophyta have been reported to be abundant in filtered samples (Lefranc et al. 2005; Mangot et al. 2013; Simon et al. 2014). Consistent with the results obtained from Lake Taihu and Lake Chaohu, the PPE community in Lake Poyang showed more seasonal variability than spatial variability. However, unlike Lake Taihu and Lake Chaohu, even at the class level, the PPE community in Lake Poyang could be seasonally different. Chlorophyceae was prevalent during autumn, Chrysophyceae was more abundant during spring, and Eustigmatophyceae was mainly present during winter.
Mychonastes was the dominant genus in Lake Poyang
Previous phylogenetic analyses based on 18S rRNA gene sequences have shown that a number of green algal lineages contain picoplankton species, including the genera Choricystis, Pseudodictyosphaerium, Meyerella, Marvania and Nannochloris within Trebouxiophyceae (Chlorophyta), as well as the genus Mychonastes in Chlorophyceae, in freshwater lakes (Hepperle and Krienitz 2015; Somogyi et al. 2016). Our results also revealed that Mychonastes sp. was dominant in Lake Poyang and accounted for approximately 66% of the PPE sequences. The genus Mychonastes belongs to the most common chlorophycean algae of picoplankton, which comprise spherical, ovoid or ellipsoidal algae living solitarily or in small groups (Krienitz et al. 2011). These algae are often overlooked due to their lack of distinctive morphological features. Members of the Mychonastes clade are typical freshwater algal taxa described from lakes and rivers in Europe, North America and Israel (Hanagata, Malinsky-Rushansky and Dubinsky 1999; Phillips and Fawley 2001; Hepperle and Schlegel 2015; Wu, Xu and Hu 2015).
The dominance of Mychonastes over all other species throughout the entire year in the study system was very similar to what was observed for Micromonas pusilla, which dominates certain types of ecosystems (Not et al. 2004). Like Micromonas, Mychonastes may have the broad ability to respond to light and nutrient variations; thus, because of this tolerance to a wide environmental spectrum, the genus may be only slightly affected by changes in the environment. Mychonastes homosphaera cultures grew well over a large range of photon fluxes (i.e. 30 to 700 μmol photons m−2 s−1) and could photoacclimate to low light by increasing their chlorophyll per cell through an increase in their photosynthetic unit numbers (Malinsky-Rushansky et al. 2002). Thus, Mychonastes can adapt to aquatic ecosystems with high turbidity and low light intensity. Furthermore, no significant relationship was observed between Mychonastes and nutrient concentrations, implying that Mychonastes can grow well within a large range of nutrient levels. Finally, it has been reported that M. homosphaera is highly enriched in lipid and oil contents, and the fatty acids in the microalgal oils included poly-unsaturated fatty acids (PUFAs) and mono-unsaturated fatty acids (MUFAs) (Wu, Xu and Hu 2015; Thao et al. 2017). Therefore, M. homosphaera might be a high-quality food source for zooplankton and heterotrophic flagellates (Gulati and Demott 1997). This result is in agreement with the observed negative correlation between Mychonastes and HF concentration in Lake Poyang.
High proportion of terrestrial fungi in samples analyzed by flow cytometric sorting
Our previous studies on other mesotrophic and eutrophic lakes underscored the advantage of using flow cytometric sorting for the analysis of PPEs, as over 70% of the retrieved reads belonged to PPEs (Li et al. 2017; Shi et al. 2018). In contrast, the sequencing results from sorted samples of Lake Poyang revealed that the proportion of PPEs was quite low, with an average of 36%. Specifically, PPEs accounted for fewer than 7% of all sequences during spring. Concomitant with PPEs, a large number of terrestrial fungi were also observed, and these fungi were mainly associated with Basidiomycota and Ascomycota. In contrast, aquatic fungi (i.e. Chytridiomycetes) and fungi-like organisms (Oomycetes), which are residents of aquatic environments, were only seldomly retrieved. In fact, freshwater fungi are a ubiquitous and diverse group of organisms. These fungi include representatives from different groups of fungi, namely, the zoosporic fungi, Hyphomycetes, Ascomycetes, Basidiomycetes and Coelomycetes among others. Freshwater fungi have characteristics that are advantageous for dispersal and subsequent attachment to new substrata. Spore adhesion can be achieved with sticky spore walls, such as an adhesive coating or a slightly more elaborate verrucose wall (Goh and Hyde 1996). In fact, fungal sequences were also obtained with PPE sequences from sorted marine samples (Shi et al. 2009).
As a river-connected lake, Lake Poyang experiences dramatic seasonal fluctuations that can be divided into four distinct periods, i.e. the dry season (from December to March), the rising period (from April to June), the flood season (from July to September) and the retreating period (from October to November) (Xue, Wan and Yang 2015). The biological consequences of water level fluctuations in lakes are typically reflected in the shifting dynamics of wetland communities in terms of their productivity and spatial distribution (Zhang et al. 2012). During the flood season, the input of allochthonous material from the surrounding land affects the structure and dynamics of the communities of shallow lakes (Bonetto, Castello and Wais 1987; Izaguirre and Vinocur 1994). The other corresponding ecological effects of water level fluctuations on lacustrine ecosystems have been scarcely reported. Our results revealed that PPEs can have large numbers of attached terrestrial fungal spores during the spring and summer. This implies that during the flood seasons, terrestrial fungal spores could disperse to the aquatic environment, and they might have important influences on the dynamics of PPEs. The main role of freshwater Ascomycetes, Basidiomycetes and mitosporic fungi in freshwater ecosystems is to decompose dead plant material and be involved in the degradation of animal parts. This study reports the close association between fungi and PPEs, and their function is still unclear and requires further study.
In conclusion, PPEs were the major contributors to the picophytoplankton in spring and winter in Lake Poyang. PPEs showed less diversity in Lake Poyang compared to other investigated lakes in the lower reaches of the Yangtze River. One OTU affiliated with Mychonastes (Chlorophyceae) was revealed to be the dominant PPE in Lake Poyang. This minute green coccal alga is reportedly representative of the prominent plankton in this river-connected lake ecosystem but is often overlooked due to its lack of morphological features. Finally, during the flood seasons, terrestrial fungal spores could disperse to the aquatic environment, and they might have important influences on the dynamics of PPEs.
ACKNOWLEDGEMENTS
We are thankful to Jian Zhou, Yuwei Chen and Zhaoshi Wu for their efforts in field sampling. This research was financially sponsored and supported by the National Natural Science Foundation of China (31670462 and 41431176), the Sino-French International Collaborative Research Project (41661134036) and the Investigation of Basic Science and Technology Resources (2017FY100300). An Illumina MiSeq (PE250) sequencer and sequencing services were provided by Metagene Science & Technology Co., Ltd. (Wuxi, China).
Conflicts of interest. None declared.
REFERENCES
Author notes
These authors contributed equally to this work.