-
PDF
- Split View
-
Views
-
Cite
Cite
Liberata Gualtieri, Francesco Nugnes, Anna G. Nappo, Marco Gebiola, Umberto Bernardo, Life inside a gall: closeness does not favour horizontal transmission of Rickettsia between a gall wasp and its parasitoid, FEMS Microbiology Ecology, Volume 93, Issue 7, July 2017, fix087, https://doi.org/10.1093/femsec/fix087
- Share Icon Share
Abstract
The incidence of horizontal transmission as a route for spreading symbiont infections is still being debated, but a common view is that horizontal transfers require intimate between-species relationships. Here we study a system that meets ideal requirements for horizontal transmission: the gall wasp Leptocybe invasa and its parasitoid Quadrastichus mendeli (Hymenoptera: Eulophidae). These wasps belong to the same subfamily, spend most of their lives inside the same minute gall and are both infected by Rickettsia, a maternally inherited endosymbiotic bacteria that infects several arthropods, sometimes manipulating their reproduction, like inducing thelytokous parthenogenesis in L. invasa. Despite intimate contact, close phylogenetic relationship and the parasitoid's host specificity, we show that host and parasitoid do not share the same Rickettsia. We provide indirect evidence that Rickettsia infecting Q. mendeli may be inducing thelytokous parthenogenesis, as the symbiont is densely present in the reproductive apparatus and is vertically transmitted. Phylogenetic analyses based on 16S and gltA placed this symbiont in the leech group. The confirmed and presumed parthenogenesis-inducing Rickettsia discovered so far only infect eulophid wasps, and belong to three different groups, suggesting multiple independent evolution of the parthenogenesis inducing phenotype. We also show some degree of cospeciation between Rickettsia and their eulophid hosts.
INTRODUCTION
Rickettsia are obligate intracellular bacteria that reside in the cytoplasm of eukaryotic cells (Weinert 2015). Although they are best known as human pathogens vectored mainly by hematophagous arthropods such as ticks, fleas and lice (Merhej and Raoult 2011), some Rickettsia are facultative endosymbionts of extremely diverse hosts, including vertebrates, arthropods, annelids, amoebae and plants (Perlman, Hunter and Zchori-Fein 2006; Weinert et al.2009b). Together with other endosymbiotic bacteria (e.g. Wolbachia, Cardinium, Arsenophonus and Spiroplasma) as well as microsporidian protists, Rickettsia are able to manipulate the reproduction of their hosts (Kageyama, Narita and Watanabe 2012). Usually, obligate intracellular bacteria are maternally transmitted (Merhej and Raoult 2011). However, phylogenetic studies, mostly focused on Wolbachia, indicate that horizontal transmission of symbionts has occurred frequently over evolutionary time scales (Vavre et al.1999; Russell and Moran 2005; Raychoudhury et al.2009). Only a few studies though have adequately characterised the Wolbachia symbionts, making necessary a re-evaluation of some cases, because distinct species may have been incorrectly treated as one (Morrow et al.2014). Similarly, although the discrepancy between host and Rickettsia phylogenies suggests that horizontal transmission among host species has occurred multiple times also in this group (Weinert, Welch and Jiggins 2009a), the lack of a standard multilocus genotyping system may have led to an overestimation of the actual number of cases. Indeed, sequencing of a single locus is usually not sufficient to characterise bacterial strains due to high recombination rates (Baldo et al.2006). Two routes favouring horizontal transmission have been hypothesised: (i) interspecific hybridisation followed by introgression of mtDNA (Raychoudhury et al.2009; Jäckel, Mora and Dobler 2013) and (ii) trophic interactions, which occur when different species share the same food resource (Morrow et al.2014). Trophic interactions are especially relevant in the case of host-parasitoid systems, also considering the ability of parasitoids to feed onto hosts, which may or may not result in the hosts’ death. Here horizontal transmission can happen in either direction. Transfer from the host to the parasitoid has been documented for Rickettsia and Wolbachia. Rickettsia infecting Bemisia tabaci (Gennadius) are transferred to Eretmocerus parasitoids, although it is not then vertically transmitted (Chiel et al.2009), and the parasitoid Leptopilina boulardi (Barbotin, Carton and Kelner-Pillault) acquires Wolbachia from its infected host Drosophila simulans (Sturtevant) (Heath et al.1999). Recently, Johannesen (2017) inferred the horizontal transfer of Wolbachia from the host Urophora cardui (L.) to its specific parasitoid Eurytoma serratulae Fabricius, based on the sharing of the same symbiont (Wolbachia sp.). On the other hand, a direct transfer from the parasitoid to the host should be more difficult because the host must survive after having been parasitised (Vavre et al.1999). However, parasitoids can act like vectors. They can transmit a symbiont from one parasitoid to another when they attack the same host, like Trichogramma wasps that acquire Wolbachia by sharing the same host eggs as infected individuals of the same or different species (Huigens et al. 2000, 2004). Or they can transmit a symbiont without getting infected themselves, like Eretmocerus sp. nr. furuhashii that transmitted Wolbachia from infected to uninfected specimens of B. tabaci by host feeding (Ahmed et al.2015) or Aphidius colemani that transmitted Hamiltonella and Regiella between its Aphis fabae hosts by sequential non-lethal probing of infected and uninfected specimens (Gehrer and Vorburger 2012). There is also evidence that plants can mediate transfer of Rickettsia (Caspi-Fluger et al.2012) and Wolbachia (Li et al.2017) from infected to uninfected individuals of B. tabaci.
In nature, intra- and interspecific horizontal obligate symbionts transfers should occur between organisms that interact in close confinement because these bacteria are incapable of surviving outside the host's tissues (Huigens et al.2004). Several examples have been reported in very different groups such as the plant–plant system where insects seem to act as vectors of infection (Pinto-Carbó et al.2016), the sponge-sponge system where the symbiont is transmitted by marine water (Sipkema et al.2015) and the mite–mite system in which the Cardinium symbionts are supposed to move among different species (Ospina, Massey and Verle Rodrigues 2016). Besides an intimate ecological association that provides horizontal transmission opportunities, phylogenetic similarity of donor and recipient species should facilitate horizontal transmission (Boivin et al.2014; Weinert 2015). In this study, we have chosen a system meeting ideal requirements to investigate the natural occurrence of horizontal transmission of Rickettsia. The system consists of the blue gum chalcid Leptocybe invasa Fisher and La Salle, a gall wasp that feeds on many Eucalyptus species and that reproduces parthenogenetically due to a Rickettsia infection, and its parasitoid Quadrastichus mendeli Kim and La Salle (Mendel et al.2004; Kim et al.2008; Nugnes et al.2015). Both species are believed to be uncommon in their native area (Australia), and all the specimens released for classical biological control programmes in Israel, Kenya and India descend from few individuals (Kim et al.2008; Nugnes et al.2015, 2016). Quadrastichus mendeli, recently recorded in Italy (Nugnes et al.2016), is also uniparental (males have never been observed in this species), develops exclusively on L. invasa (Kim et al.2008) and is infected by Rickettsia as well (our preliminary observations). The following characteristics make this host–parasitoid system an excellent model for this study: (i) both species are thelytokous; (ii) both belong to the subfamily Tetrastichinae; (iii) both are infected by Rickettsia; (iv) Q. mendeli is monophagous; and (v) host and parasitoid live in a very confined space, the microhabitat of the minute L. invasa gall. The main goal of this work was to assess whether horizontal transmission was the route of infection of Rickettsia in this host–parasitoid association by thoroughly characterising the Rickettsia strain infecting Q. mendeli. As all confirmed and presumed parthenogenesis-inducing (PI) Rickettsia hitherto identified infect eulophid wasps, we also looked for evidence of congruent phylogenies between Rickettsia and their eulophid hosts.
MATERIALS AND METHODS
Insect sampling and DNA extraction
Leaves and sprouts harbouring galls of Eucalyptus camaldulensis Dehnhardt, infested with L. invasa, were sampled from 2013 to 2015 in Italy at localities in the provinces of Naples (Portici, San Giorgio a Cremano and Ercolano) and Rome (Bracciano), placed in plastic boxes and stored at room temperature. The only parasitoid reared was Q. mendeli, and all specimens matched the original description of the species (Kim et al.2008). No males were collected. Emerged parasitoid specimens were killed in 95% ethanol. Data on examined specimens are summarised in Table 1. For DNA extraction, we selected at least three Q. mendeli specimens from each locality. Each specimen emerged from a different gall among those collected from 15 trees on different dates. DNA was extracted from whole single individuals (15 females) using a Chelex and proteinase K-based method (Gebiola et al.2009). Although specimens of L. invasa collected in distant countries such as Italy, Argentina and Tunisia harbour a single Rickettsia strain (Nugnes et al.2015), we also analysed 12 specimens of L. invasa from sites not previously sampled and reared from the same galls from which Q. mendeli emerged (Rome, San Giorgio a Cremano and Ercolano).
Specimens investigated and analyses performed in the study. +: specimen Rickettsia sp. positive in specific PCR.
![]() |
![]() |
Specimens investigated and analyses performed in the study. +: specimen Rickettsia sp. positive in specific PCR.
![]() |
![]() |
Detection of bacterial symbionts
Denaturing gradient gel electrophoresis (DGGE) was used to check for the presence of bacterial symbionts in all specimens of Q. mendeli listed in Table 1. The V3 hypervariable region of bacterial 16S rDNA was amplified by a nested PCR as per Nugnes et al. (2015). The three known PI Rickettsia strains (isolated from the eulophid wasps Neochrysocharis formosa, Pnigalio soemius and L. invasa) were used as positive controls. PCR products were subjected to DGGE analysis slightly modifying the technique described by Muyzer, De Waal and Uitterlinden (1993) to increase the resolution of the analysis (denaturing gradient 40%–52%; 90V for 20 h). All DGGE bands were excised, dissolved in ddH2O, used as templates for PCR with the primers 341f (to which a 40-bp GC clamp was added) and 518r, and direct sequenced. Sequences were then compared with those obtained by direct amplifications and checked against the GenBank database by Blast searches. In addition, diagnostic PCRs were performed with specific primers targeting the 16S rRNA gene of all known sex manipulator symbionts as per Nugnes et al. (2015), to which here we added Microsporidia amplified by seminested PCR using primer pairs V1F and 1492R (Weiss et al.1994) and V1F and 530R (Baker et al.1995).
Molecular characterisation and phylogenetic analyses
Rickettsia isolated from Q. mendeli and L. invasa were genetically characterised at the strain level through a multigenic approach. We tried to amplify the same genes used for the previous characterisation of PI Rickettsia of L. invasa, that is, 16S rDNA, atpA, gltA, the intergenic spacer rpmE-tRNAfMet (Nugnes et al.2015) and also coxA, ompA and Sca1 genes using primers listed in Table S1 (Supporting Information). The amplification of 16S rDNA was carried out using a nested PCR with the Rickettsia-specific primers Rb-F and Rb-R (Gottlieb et al.2006), using as template a longer fragment amplified with the universal primers 27F and 1513R, with the following programme: 3 min of initial denaturation at 94°C, 40 cycles at 94°C for 1 min, 55°C for 1 min and 72°C for 1 min and a final extension of 5 min at 72°C.
For the citrate synthase (gltA) gene, some authors have evaluated only the 5΄ portion (about 600 bp) (Weinert et al.2009b) while others, mostly working on Rickettsia harboured by Deronectes water beetles, sequenced only the 3΄ portion (about 400 bp) (Küchler, Kehl and Dettner 2009). We amplified both portions of this gene overlapping by about 300 bp, the first with primers rcit 133f-Qm 500rev (this study) and the second with primers gltAQm-F (this study) -rcit 1197r. This allowed us to use taxa for which either fragment was sequenced. PCRs and cycling conditions for gltA were set as described by Goodacre et al. (2006) with annealing at 57°C and 1 min extension steps, for the rpmE-tRNAfMet as described in Fournier et al. (2004) and for atpA, coxA, ompA and Sca1 genes as described in references listed in Table S1. Selected genes missing for taxa used in phylogenetic analyses were amplified as above, except for rpmE-tRNAfMet, for which a new reverse primer had to be designed on the Rickettsia belli genome (CP000087) to amplify the R. bellii strain infecting P. soemius (Table S1). PCR products were visualised using 1.2% agarose gel electrophoresis, and the fragments amplified were directly sequenced at the Genomics Genetics Biology facility in Perugia, Italy. Chromatograms were assembled using Bioedit 7.2.5 (Hall 1999) and edited manually, and sequences were aligned using the G-INS-I algorithm in MAFFT 7 (http://mafft.cbrc.jp/alignment/software/) (Katoh and Standley 2013) and deposited in GenBank with accession numbers reported in Table 1.
Phylogenies of the genus Rickettsia were constructed on a gltA alignment and a concatenated alignment of 16S rDNA and gltA using maximum likelihood (ML) and Bayesian inference (BI) in RAxML 7.0.4 (Stamatakis 2006) and MrBayes 3.2 (Ronquist et al.2012), respectively. Homologous sequences from other principal Rickettsia strains available from GenBank (accessions available in Supporting Information Table S2) were included in the analyses. Wolbachia infecting Nasonia vitripennis (Walker) (Hymenoptera: Pteromalidae), Wolbachia infecting Encarsia formosa (Gahan) (Hymenoptera: Aphelinidae) and the related species Orientia tsutsugamushi (Hayashi) were used as outgroups. For both the datasets, the GTR + G evolutionary model, as selected by jModeltest (Posada 2008) and PartitionFinder (Lanfear et al.2012), were implemented in ML and BI analyses. For both gltA and concatenated dataset, the best partitioning schemes found by PartitionFinder was by codon position 1, 2 and 3 separately. ML trees were obtained after 10 000 pseudoreplicates on the original alignment, starting from a random parsimonious tree and with default initial rearrangement settings and number of rate categories. ML clades were considered as supported when bootstrap support was >70%.
For BI, two independent runs of four simultaneous Monte Carlo Markov chains (MCMCs) ran for five and eight million generations for gltA and concatenated alignments, respectively. Trees were sampled every 1000 generations. Convergence of the separate runs was checked using the average deviation of split frequencies diagnostic (<0.01) and confirmed in Tracer 1.6 (Rambaut et al.2014). The burnin value was set at 25% of sampled topologies and the threshold for clade acceptance at 0.95.
Fluorescence microscopy
After detection of bacterial symbionts with DGGE and sequencing of the resulting bands, localisation of Rickettsia isolated from Q. mendeli within the host's tissues was carried out using fluorescence in situ hybridization (FISH) with the Rickettsia-specific probe RickPn-Cy3 (Giorgini et al.2010) and the universal probe EUB338-Cy5. Ovaries, mature eggs and guts (with Malpighian tubules) were extracted from adult females in PBS buffer under a stereomicroscope. To improve the localisation of Rickettsia symbiont in L. invasa performed by Nugnes et al. (2015), which showed that the symbiont is present in ovaries and eggs, we also evaluated the presence of bacteria in its gut. Samples were fixed in pre-chilled ethanol (–20°C) for 2 h. Immediately after fixation, a Turbo RNA FISH was performed by the following protocol that combines two methods (Sakurai et al.2005; Shaffer et al.2013). Fixed samples were placed on a siliconised slide in 20 μl of hybridisation buffer (20 mM Tris-HCl pH 8.0, 0.9 M NaCl, 0.01% sodium dodecyl sulfate, 30% formamide) containing 50 μM of probe. Samples were hybridised for 5 min in a humidified chamber at 37°C, and then subjected first to a quick wash followed by three washes (one minute each) with washing buffer (0.3 M NaCl, 0.03 M sodium citrate, 0.01% sodium dodecyl sulfate). Mounting medium with DAPI (0.7μg/ml) was used to counterstain nuclei of the host cells. Stained samples were observed under a ZEISS Axiophot 2 epifluorescence microscope and under the Leica confocal TCS SP5 microscope. Images obtained on multiple focal planes were stacked with the software Leica application Suite, Advances fluorescence 2.4.1. The specificity of the observed signals was verified with no-probe control and RNase-digested control.
Host–symbiont cophylogeny analyses
Cophylogenetic analyses were performed using a host tree obtained by a concatenated analysis of the mitochondrial cytochrome c oxidase subunit I (COI), the expansion segment D2 of the 28S ribosomal subunit (28S-D2) and the Internal Transcribed Spacer 2 (ITS2) of all known hymenopteran species harbouring PI Rickettsia (as in Nugnes et al.2016) and a symbiont tree obtained by a concatenated analysis of 16S rDNA, gltA and rpmE-tRNAfMet (Table 1 and Table S1). Nasonia vitripennis and its Wolbachia symbiont were used as outgroups. Trees were obtained by BI using the GTR+G+I and GTR+G model for the host and symbiont dataset, running the MCMC for two and three million generations, respectively. Tree sampling, convergence and burnin were set as described above.
To examine the congruence between phylogenies of PI Rickettsia species and their eulophid hosts, a tree-based method implemented in the program Jane 4.0 (Conow et al.2010) and a distance-based method, Parafit (Legendre, Desdevises and Bazin 2002) as implemented by CopyCat (Meier-Kolthoff et al.2007), were used. The Jane software compares the symbiont and host tree topologies by optimally mapping the first onto the second using different event costs to find the optimal solutions to reconcile the two phylogenetic trees (Mendlová et al.2012). Five types of events are contemplated: cospeciation, duplication, failure to diverge, loss and duplication with host switch. The analysis was performed with 500 generations and a population size of 100 as parameters of the genetic algorithm (Viale et al.2015). Furthermore, significant matching of host and symbiont bacterial phylogenies was assessed by computing the costs of 500 replicates with random tip mapping and comparing the resulting costs to the cost of the original associations. ParaFit was used to test the null hypothesis of random association between host and parasite data sets. Distance matrices for hosts and symbionts were calculated using the trees obtained as above. Tests of random association were performed with 999 999 permutations globally across both matrices of host–parasite association.
RESULTS
Detection of bacterial symbionts
DGGE profiles of the PCR-amplified 16S rRNA gene showed a single dominant band occurring in all tested Q. mendeli specimens at a height different than that of Rickettsia infecting L. invasa. Sequencing confirmed that this band corresponds to Rickettsia (hereafter Qm Rickettsia), and all sequences obtained from this band were identical. Some samples presented other less-pronounced bands, identified by sequencing as environmental bacteria, Stenotrophomonas, Klebsiella, Citrobacter and Pantoea eucalypti Brady. PCR screening with universal and specific primers did not result in any amplification of known sex manipulating symbionts or other bacteria besides the Rickettsia identified by DGGE.
Molecular characterisation and phylogenetic analyses
We successfully amplified 16S rDNA, the intergenic spacer region rpmE-tRNAfMet and a fragment of ∼1000 bp of citrate synthase (gltA), whereas attempts to amplify the ATP synthase F1 (atpA), cytochrome c oxidase A (coxA), outer membrane protein A (ompA) or surface cell antigen (Sca1) genes were unsuccessful (Table S1). For each gene amplified, the sequences were identical in all tested Q. mendeli specimens (Table 1). ML and BI reconstructions on a concatenated alignment (1792 bp) of 16S rDNA and gltA resulted in similar trees and revealed that Qm Rickettsia phylogenetically belongs to the torix group (Fig. 1). However, phylogenetic analyses based on a longer gltA gene fragment alone showed that this group is in fact divided into two well-supported monophyletic groups, which correspond to the limoniae and leech groups proposed by Küchler, Kehl and Dettner (2009). The leech group that includes Qm Rickettsia consists mainly of symbionts of dipterans, whereas the limoniae group includes mainly symbionts from spiders. The sister relationship of these groups is not well supported (Fig. S1, Supporting Information). Sequencing of the 16S rRNA gene, gltA and rpmE-tRNAfMet of all analysed specimens of L. invasa confirmed that they harbour only the Rickettsia symbiont characterised previously (Nugnes et al.2015), which belongs to the transitional group.
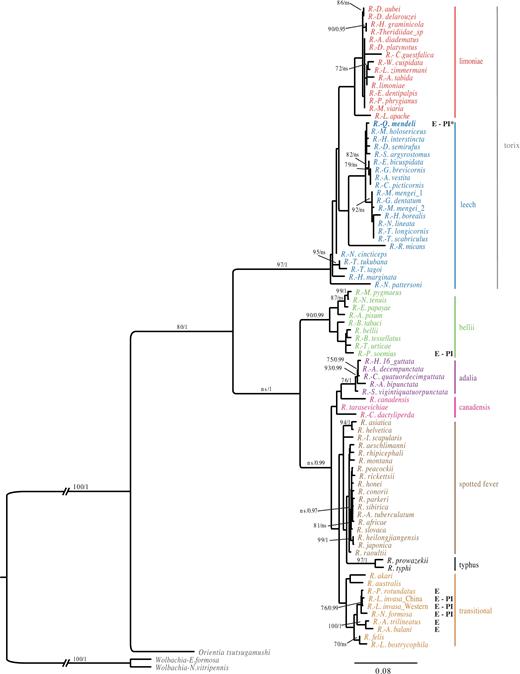
Phylogenetic placement of the Q. mendeli symbiont within the genus Rickettsia. BI majority-rule consensus tree for the concatenated 16S rRNA and citrate synthase (gltA) genes. Posterior probability values >0.95 are shown above branches, along with bootstrap values >70% for the topologically identical ML tree. ns= not significant. The host is provided whenever the symbiont is not identified at the species level. Scale bar indicates the number of substitutions per site. (E: eulophid; PI: parthenogenesis inductor; PI*: suspected parthenogenesis inductor)
Fluorescence microscopy
FISH showed a high concentration of Rickettsia in the reproductive tissues of Q. mendeli, in particular in ovaries and in mature eggs (Fig. 2a–c), as well as along the gut and Malpighian tubules of both Q. mendeli and L. invasa (Fig. 3b and Fig. S2, Supporting Information). Inside the ovaries, dense clusters of bacteria were observed in the germarium (Fig. 2a and b) and probing with the Rickettsia-specific probe and the universal bacterial probe did not reveal the occurrence of bacteria other than Rickettsia (Fig. 2b). Bacteria were spread inside the developed oocytes, but more densely distributed in the peduncle and around the nucleus (Fig. 2c). In the gut, although the two probes did not completely overlay, clusters of bacteria were spread within the gut's cells and in Malpighian tubules (Fig. 3). Negative controls (no-probe and RNase-digested controls) did not display any signal, confirming the specificity of the detected signals.
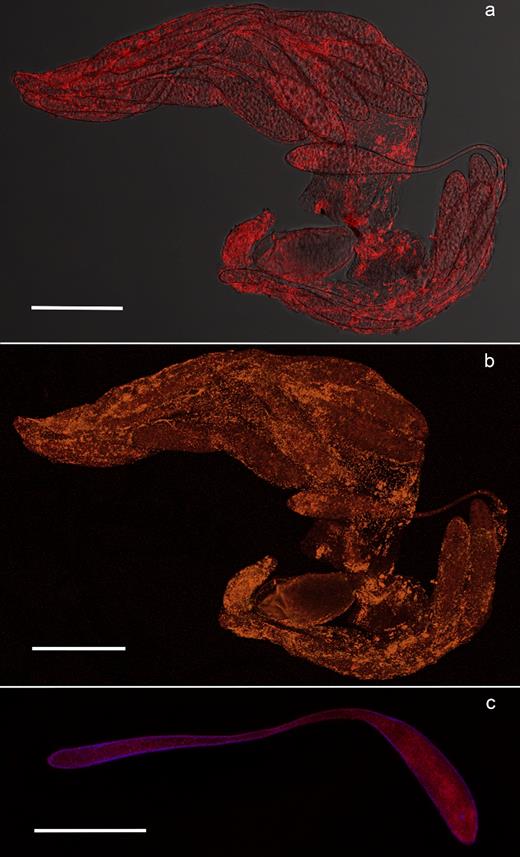
FISH on Q. mendeli ovarioles and eggs. Distribution of Rickettsia in the ovary (a and b) and the egg (c) of Q. mendeli. In the ovary, Rickettsia bacteria (bright red spots) are inside the developing oocytes (a). Merged image of ovarioles simultaneously stained with the Rickettsia-specific probe RickPn-Cy3 and the universal bacterial probe EUB338-Cy5, showing Rickettsia cells in orange (b). Within the egg, bacteria are distributed in the head and in the peduncle (c). DAPI-stained nuclei are blue. Scale bar = 100 μm.
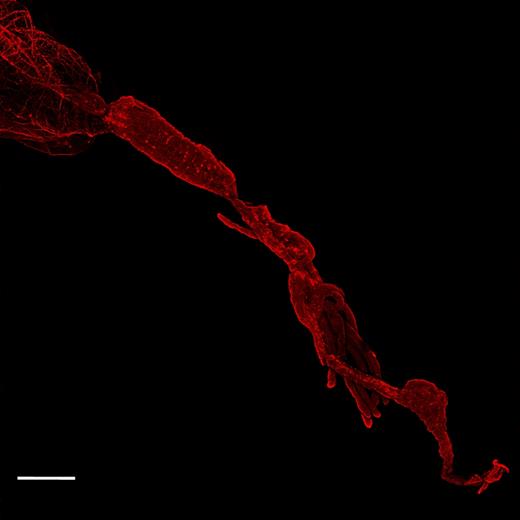
FISH on Q. mendeli gut. Rickettsia bacteria, stained with the Rickettsia-specific probe RickPn-Cy3 (bright red spots), are concentrated along the gut tube and Malpighian tubules. Scale bar = 100 μm.
Host–symbiont cophylogeny analyses
The tanglegram built from the host and symbiont phylogenetic trees (Fig. 4a), and individual associations between PI Rickettsia species and their eulophid insect hosts, suggested that the trees did not perfectly match (Fig. S3, Supporting Information), but the reconciliation analysis indicated a significant cophylogeny between wasps and their PI symbionts, with more cospeciation events than expected by chance (P < 0.05) (Fig. 4b). Four cospeciation events were suggested with one event of duplication and host switch and one of loss, for a significant global cost of three. The global test of ParaFit indicated a not significant congruence between insect hosts and their symbionts (P = 0.17641).
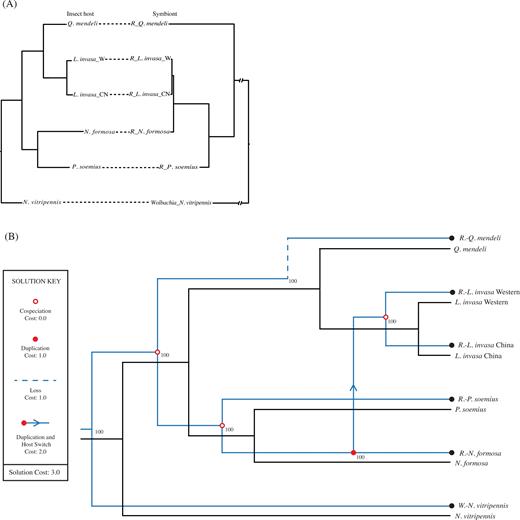
(a) Tanglegram built from the phylogenetic trees and individual associations between PI (confirmed and presumed) Rickettsia species and their eulophid insect hosts. (b) Cophylogenetic scenario between the thelytokous eulophids tree and its host ’s tree from Jane 2 (4 cospeciation events, 1 duplication, 1 duplication and host switch, 1 loss, total cost = 3). Black and blue lines represent parasitoids and their Bacteria symbionts, respectively.
DISCUSSION
We have identified a new Rickettsia strain associated to the parasitoid Q. mendeli, which is unrelated to the one infecting its host, L. invasa. Therefore, contrary to our expectation, the pest and its specific parasitoid do not share the same symbiont, even though all ideal requirements for Rickettsia symbiont transfer are met: host and parasitoid are closely related, have a long coevolutionary history and develop in the same minute gall. The lack of horizontal transmission of Rickettsia could be explained either by symbiotic specialisation or by ‘infection exclusion’. Specialisation as consequence of a long coevolutionary history can be so extreme that the symbionts become completely dependent on their host, thus unable to live in other hosts (Aanen and Bisseling 2014; Bennett et al.2014). We speculate that our failure in amplifying the atpA gene (see below) could be explained by gene loss following specialisation of Qm Rickettsia to its host. The other possible explanation is the infection exclusion. It has been shown that some microorganisms protect their insect hosts from parasitoids or pathogens by producing specific toxins or by modifying the insect immune system (Kaltenpoth and Engl 2014; Douglas 2015). This hypothesis has already been supported for ticks (Macaluso et al.2002; Oliver et al.2014; Gall et al.2016). We have shown that Rickettsia infecting Q. mendeli and L. invasa are not located only in the ovaries, but are abundantly spread also along the gut and Malpighian tubules (Fig. 3), as already shown in other insects (Gottlieb et al.2006; Behar, McCormick and Perlman 2010). This distribution suggests that Rickettsia could also have a role in nutritional provision and/or secretion processes of both insects, thus making the colonisation by other symbionts difficult. Therefore, the high density of Qm Rickettsia in the parasitoid may have prevented the horizontal transfer of L. invasa PI Rickettsia, or vice versa. Genome specialisation or infection exclusion may have prevented either insect from acquiring Rickettsia from the other, either if both insects were already infected with different Rickettsia or if only one of them was infected. However, the first scenario, which would move the beginning of symbiosis in this system far back in time, seems the most plausible.
Our phylogenetic reconstruction by concatenating 16S and gltA genes placed Qm Rickettsia in the leech group. Phylogenetic analyses based for the first time on combining two different regions of the gltA gene confirmed this finding, and also distinguished two well-supported monophyletic groups, which correspond to the limoniae and leech groups (sensu Küchler, Kehl and Dettner 2009). These groups include Rickettsia harboured by flies, spiders, leeches and a beetle, whose life cycle is often related to water (Weinert et al.2009b; Weinert 2015), and have also been treated as a single monophyletic group (torix group sensu Weinert et al.2009b). We tried including more genes, but this attempt was hampered by failures in amplifying atpA, Sca1, coxA and ompA. Similar failures had already been recorded for other Rickettsia (Ngwamidiba et al.2006; Vitorino et al.2007). We speculate that as both Sca1 and ompA are implicated in the process of adherence and invasion of host cells (Chan, Riley and Martínez 2010), they may not be present in species that are vertically transmitted and not pathogenic to mammals.
We were not able to definitively prove that the Rickettsia infection is related to Q. mendeli thelytoky, as, despite numerous attempts, we were not able to rear L. invasa, and in turn Q. mendeli. This prevented us from trying to remove Rickettsia with antibiotics to restore the production of male progeny (Hagimori et al.2006; Giorgini et al.2010). However, the high density of Rickettsia in the reproductive tissues, suggesting vertical transmission, and the high transmission rate, as all specimens are infected, represent preliminary, although indirect, evidence that Qm Rickettsia may be able to induce thelytokous parthenogenesis. Moreover, thelytoky in chalcidoid wasps is almost always caused by bacterial endosymbionts (Ma and Schwander 2017). Only two exceptions are known, Trichogramma cacoeciae Marchal and Thripoctenus javae (Girault), where, as bacterial reproductive manipulators were not found, thelytoky is supposed to have a genetic origin (Vavre, De Jong and Stouthamer 2004; Monti et al.2016).
Over the last decade, Rickettsia have been shown to induce thelytokous reproduction in three wasps of the family Eulophidae, namely Neochrysocharis formosa (Westwood) (Hagimori et al.2006), Pnigalio soemius (Walker) (Giorgini et al.2010) and L. invasa (Nugnes et al.2015). These PI Rickettsia belong to two different phylogenetic groups: the strain infecting P. soemius to the bellii group (Giorgini et al.2010) and the strains infecting L. invasa and N. formosa to the transitional group (Hagimori et al.2006; Nugnes et al.2015), closely related to Rickettsia infecting other eulophid wasps (e.g. Pediobius sp. and Aulogymnus sp.) (Weinert et al.2009b), whose phenotypes are still unknown. If the involvement of Qm Rickettsia in the thelytoky of its host is confirmed, PI Rickettsia will have been found in three different phylogenetic groups (Fig. S1).
We observed no genetic variation in either Rickettsia or Q. mendeli. PCR and DGGE screening showed that Qm Rickettsia is the only bacterial symbiont, among the known reproductive manipulators, present in all examined specimens of Q. mendeli. DGGE also sporadically detected other bacteria undetectable by PCR screening, which were determined to be environmental contaminations by members of Enterobacteriaceae that are commonly found in invertebrates (Zouache et al.2009). Besides, none of the Qm Rickettsia gene fragments showed any variation, including rpmE-tRNAfMet, which is useful for distinguishing Rickettsia at the strain level (Merhej and Raoult 2011). Being an introduced biological control agent (furthermore thelytokous), the genetic variation of Q. mendeli in its new range is reduced by genetic bottleneck, as for its host L. invasa (Nugnes et al.2015). Our specimens presumably underwent a further genetic bottleneck as they spread to Italy from Israel without intentional human intervention (Nugnes et al.2016). Therefore, it may be expected that the genetic variation of its Rickettsia symbiont is similarly reduced. Although we have not sampled Q. mendeli from the native area, the likelihood that its Rickettsia is a reproductive manipulator makes it unlikely at this time to envision that other populations may acquire the PI Rickettsia from L. invasa.
Lastly, the tree-based cophylogenetic analysis showed that there was cospeciation only for L. invasa and its symbiont (but see Poisot 2015 for a critical review of cophylogenetic inference methods). Results using a distance-based method, instead, did not show any significant correlation among insect hosts and bacterial symbionts, in line with results by Viale et al. (2015). However, the symbiont phylogenetic tree is partially congruent with that of its hosts, consistent with results previously reported for other insects (Bright and Bulgheresi 2010).
In conclusion, our integrative approach has shown that (i) the parasitoid Q. mendeli and its host L. invasa do not share the same PI Rickettsia, rejecting the hypothesis of horizontal transmission; (ii) the Rickettsia strain that infects Q. mendeli belongs to the leech group; (iii) the symbiont is likely involved in its host's thelytokous reproduction; (iv) pending validation of this hypothesis, the genus Rickettsia has likely evolved the capability to determine thelytokous reproduction at least three times; (v) there has been some degree of coevolution between PI Rickettsia and their eulophid hosts.
SUPPLEMENTARY DATA
Supplementary data are available at FEMSEC online.
Acknowledgements
The authors thank Bodil Cass for kindly editing the language of an earlier draft and for insightful comments, Elia Russo for his technical help and Raffaele Sasso for providing samples. Lastly, we thank two anonymous reviewers whose comments helped improving the manuscript.
FUNDING
This research activity was supported by MIUR for the project titled: Insects and globalisation: sustainable control of exotic species in agro-forestry ecosystems Globalization Exotic Insects Sustainable Control Agro-forestry ecosystems (GEISCA) PRIN, id: 2010CXXHJE, and partly by the Campania Region-funded URCOFI project (Unità Regionale Coordinamento Fitosanitario).
Conflict of interest. None declared.