-
PDF
- Split View
-
Views
-
Cite
Cite
Elisa C P Catão, Cécile Thion, R.H. Krüger, James I Prosser, Ammonia oxidisers in a non-nitrifying Brazilian savanna soil, FEMS Microbiology Ecology, Volume 93, Issue 11, November 2017, fix122, https://doi.org/10.1093/femsec/fix122
- Share Icon Share
Abstract
Low nitrification rates in Brazilian savanna (Cerrado) soils have puzzled researchers for decades. Potential mechanisms include biological inhibitors, low pH, low microbial abundance and low soil moisture content, which hinders microbial activity, including ammonia oxidation. Two approaches were used to evaluate these potential mechanisms: (i) manipulation of soil moisture and pH in microcosms containing Cerrado soil and (ii) assessment of nitrification inhibition in slurries containing mixtures of Cerrado soil and an actively nitrifying agricultural soil. Despite high ammonium concentration in Cerrado soil microcosms, little NO3− accumulation was observed with increasing moisture or pH, but in some Cerrado soil slurries, ammonia-oxidising archaea (AOA) amoA transcripts were detected after 14 days. In mixed soil slurries, the final NO3− concentration reflected the initial proportions of agricultural and Cerrado soils in the mixture, providing no evidence of nitrification inhibitors in Cerrado soil. AOA community denaturing gradient gel electrophoresis profiles were similar in the mixed and nitrifying soils. These results suggest that nitrification in Cerrado soils is not constrained by water availability, ammonium availability, low pH or biological inhibitors, and alternative potential explanations for low nitrification levels are discussed.
INTRODUCTION
Autotrophic nitrification, the sequential oxidation of ammonia to nitrite and nitrate, is a major cause of N loss in terrestrial environments. In agricultural systems, nitrification is the main pathway of N transformation, and up to 95% of total N is present as NO3− potentially leading to leaching and emission of nitric oxide (NO) and nitrous oxide (N2O) by nitrifiers and denitrifiers (Subbarao et al.2012). Inhibitors of nitrification can decrease nitrogen losses from these systems (Subbarao, Ito and Sahrawat 2006). These inhibitors target the first step in nitrification, ammonia oxidation, which is carried out by both bacterial and archaeal ammonia oxidisers. Some natural systems have lower nitrification rates and higher nitrogen fertiliser use efficiency than managed systems (Ste-Marie and Paré 1999). For example, in soils of the tropical savanna biome in Central Brazil, also called Cerrado, NO3− concentration is low or undetectable (Nardoto and Bustamante 2003), the NH4+:NO3− ratio is high and the abundance of nitrifiers is low (Catão et al.2016). These ecosystems may therefore provide a model for greater and more sustainable crop productivity and decreased demand for nitrogen fertilisers.
There are several potential explanations for low rates of nitrification in Cerrado soils, based on biological and physicochemical factors. Plants may decrease nitrification by competing for NH4+-N and by increasing the C:N ratio through increased carbon supply, thereby promoting immobilisation, while some plants produce nitrification inhibitors in plant litter and root exudates (Subbarao, Ito and Sahrawat 2006). These inhibitors target ammonia oxidation and can benefit plants by reducing competition for ammonium (Subbarao, Ito and Sahrawat 2006; Subbarao et al.2015). Both ammonia-oxidising archaea (AOA) and ammonia-oxidising bacteria (AOB) are present in these soils (Catão et al.2016) but the relatively high ammonium concentration in Cerrado soil [3–22 μg N g−1 soil, (Nardoto and Bustamante 2003); 5–49 μg N g−1 soil (Catão et al.2016)] suggests that ammonia oxidisers are not limited by ammonia concentration and low rates of nitrification in Cerrado soils may be better explained by production of biological nitrification inhibitors.
Low nitrification rates in acidic soils have been described for many years (De Boer and Kowalchuk 2001). Inhibition of ammonia oxidation in low pH soil was traditionally considered to be due to the low availability of ammonia, through ionisation to NH4+, but may be alleviated by growth in soil aggregates or on surfaces (De Boer et al.1991; Allison and Prosser 1993), urease activity (De Boer, Duyts and Laanbroek 1989; Burton and Prosser 2001) or growth of acidophilic archaeal ammonia oxidisers (Gubry-Rangin et al.2011; Lehtovirta-Morley et al.2011). Meta-analysis of net nitrification rates in a wide range of soils (Booth, Stark and Rastetter 2005) suggests that pH limitation may not be widespread, but increased nitrification following amendment of Cerrado soil with calcium carbonate (Rosolem, Foloni and Oliveira 2003) provides evidence for pH limitation in soil.
Low water availability decreases nitrification (Placella and Firestone 2013; Thion and Prosser 2014) by increasing osmotic stress and reducing mobility of ammonia within the soil. In the seasonally dry Cerrado biome, N2O and NO emissions increase after rainfall or addition of artificial rainwater (Pinto et al.2002, 2006) providing evidence for limitation of nitrification during dry seasons.
Limited nitrification is alleviated by agriculture due to fertilisation, liming, tillage or plant community change. Considering the extensive conversion of Cerrado soils to agricultural production (Marris 2005; Catão et al.2016), it is important to understand adaptation of natural ecosystems to limit N loss. The aim of this study was to test three hypotheses regarding potential mechanisms for the low nitrification rates: presence of biological nitrification inhibitors, low water availability and low pH. The presence of plant-derived nitrification inhibitors was tested by analysing (i) the growth of AOB and AOA in the presence of aqueous extract from Cerrado soil and (ii) the effect of Cerrado soil on ammonia oxidation by a nitrifying soil (Craibstone) in soil slurries. The effects of low water availability and low pH on nitrification were tested by manipulating Cerrado soil water content and pH in microcosms.
MATERIALS AND METHODS
Soil sampling
Cerrado soil was sampled from an undisturbed shrubland (Campo sujo), with some sparse shrubs over a continuous grass layer (Eiten 1972), where graminoids can account for around 45% of total aboveground biomass, leading to a contribution of 46% of relative abundance of fine roots (Castro and Kauffman 1998). Campo sujo is dominated by plants from the families Asteraceae, Leguminosae and Poaceae (Tannus and Assis 2004). The average monthly precipitation and temperature, measured at the nearest meteorological centre in 2014 (∼30 km from the farm; Pirenopolis—GO, Station 83376, 15°50΄60΄S 48°57΄36΄W), were 143 mm (range 0–317 mm) and 23.4°C (range 21°C–25.6°C), respectively. Triplicate soil samples were obtained from the upper 10 cm of soil, pooled before sieving (2-mm mesh size) and then stored at 4°C. The climate in the Cerrado biome is tropical (Köppen Aw), and samples were collected at the beginning of the dry season (May 2014). Campo sujo and Cerrado sensu stricto are usually found on oxisols, with low nutrient content, low pH, and high content of aluminium (Reatto, Correia and Spera 1998). The soil, which was well aerated and well drained, is classified as sandy loam with 20.8% clay and had an initial pH of 5.6 (±0.04). Physicochemical parameters from the Campo sujo sample were previously described (Catão et al.2016): organic matter content was 42.6 (±2.4) g kg−1, cation exchange capacity 6 (±0.6) cmolc dm−3, available phosphorus 1.8 (±0.13) mg dm−3, aluminium 1.2 (±0.12) cmolc dm−3 and Fe 165.4 (±41.0) mg dm−3. Craibstone soil, used in this study as a reference nitrifying soil, was sampled from an experimental agricultural field (Scottish Agricultural College, Craibstone, Scotland, Grid reference NJ872104) and maintained at pH 5.5 since 1961.
Cultivation of ammonia oxidisers with soil extracts
Aqueous extracts of Craibstone and Campo sujo soils were prepared by blending 20 g soil in 2 volumes of sterile distilled water for 40 s, rotating in 50-mL sterile tubes for 1 h, centrifuging (3000 × g for 15 min) and sterilising by progressive filtration through filters with 10-mm, 5-mm, 0.45-μm and 0.22-μm pore size. NH4+ and NO3− concentrations in the filtrates were below the level of detection (data not shown).
Pure cultures of AOA (Candidatus Nitrosocosmicus franklandus; Lehtovirta-Morley et al.2016) and AOB (Nitrosospira briensis #128, N. tenuis #NV-12, N. multiformis— NCIMB11849, ATCC25196 and N. europaea—NCIMB11850, ATCC25978) were cultivated in inorganic growth medium in the dark without shaking. Candidatus Nitrosocosmicus franklandus (Lehtovirta-Morley et al.2016) was cultivated at 40°C in medium described previously (Lehtovirta-Morley et al.2011) but modified by the addition of 1 mL L−1 vitamin solution (Widdel and Bak 1992), 1 mL L−1 selenite-tungstate solution (Widdel and Bak 1992) and 2 mM NH4Cl. The pH was maintained at ∼7.5 by the addition of 10 mL L−1 1 M HEPES buffer. The AOB were grown in Skinner and Walker (1961) and incubated at 30°C. Triplicate cultures were prepared in 30-mL Universal tubes by adding 5 mL of the appropriate medium, previously inoculated with exponentially growing cells (1 mL inoculum per 100 mL 2 × concentrated medium), to a 5-mL volume of sterile distilled water, Craibstone or Campo sujo soil aqueous extract, or allylthiourea (100 μM final concentration), an ammonia oxidiser inhibitor. The cultures were grown without agitation, and growth was monitored for 26 days (AOA) and 13 days (AOB) by measuring nitrite accumulation (Shinn 1941). The
maximum specific growth rate was estimated as the slope of semi-logarithmic plots of nitrite concentration versus time.
Soil incubation in slurries
Soil slurries were established in 250-mL sterile Erlenmeyer flasks containing 20 g soil and 100 mL sterile distilled water, stirred at 100 rpm and maintained at 30°C in the dark. Individual flasks contained Campo sujo soil, Craibstone soil or mixtures of Campo sujo and Craibstone soils in 1:1 or 4:1 ratios. Before incubation and 1, 7, 14, and 21 days after incubation, soil slurry aliquots (8 mL) were centrifuged at 3000 × g for 15 min. After immediate measurement of pH in 2 mL of supernatant, the remaining supernatant (6 mL) was stored at –20°C for quantification of inorganic N (see below). The soil pellet was frozen in liquid nitrogen and stored at –80°C for molecular analysis.
Soil incubation in microcosms
Campo sujo soil was incubated in sealed microcosms consisting of 140-mL sterile serum glass bottles containing 10 g soil. The soil had an initial water content of 24.9 ± 0.03 g H20 100 g−1 dry soil, corresponding to a matric potential of –0.15 ± 0.01 MPa. Microcosms were incubated for 4 days in the dark at 30°C (acclimation period) and then divided into two groups. The ‘dried soil’ group was left to air dry, reaching a moisture content of 8.66 g H20 100 g−1 dry soil (–6.34 ± 2.98 MPa matric potential). In the ‘moist soil’ group, the moisture content was adjusted to 37.9 ± 0.3 g H20 100 g−1 dry soil by adding sterile distilled water. Soil in half of the dried soil microcosms was rewetted to 39.6 ± 1.92 g H20 100 g−1 dry soil (–0.11 ± 0.02 MPa) (‘water pulse’ treatment), and the soil in the remaining dried soil microcosms was kept dry (‘dry’ treatment). Finally, the pH of soil in half of the moist soil microcosms was increased to 6.34 ± 0.09 with CaCO3 (‘pH treatment’). The pH of soil in the remaining microcosms (‘dry’, ‘water pulse’ and ‘moist’ treatments) was 5.21 ± 0.02, which was slightly lower than the initial value of the sampled soil and was not adjusted. The four treatments were performed in triplicate, with or without the addition of the ammonia oxidation inhibitor acetylene (0.01% of headspace volume). The soil microcosms were incubated in the dark at 30°C, and aerobic conditions were maintained by removing the seals for 5–10 min twice weekly. The ‘moist’ and ‘water pulse’ microcosms were watered weekly to maintain moisture content. The microcosms were sampled destructively after 6 h and 1, 3, 7, 14 and 21 days, with additional sampling after 28 days for the pH treatment. For each microcosm, half of the soil was used for chemical analysis and the remainder was stored at –80°C for molecular analysis.
Soil physicochemical analyses
Water matric potential was measured using a WP4C Dewpoint PotentiaMeter (Decagon, Pullman, UK), and pH was determined in water. Soil NH4+ and NOx (NO2− + NO3−) concentrations were determined colorimetrically by flow injection analysis (FIA star 5010 Analyser, Foss Tecator AB, Höganäs, Sweden) (Allen 1989) after extraction from 2 g wet soil in 10 mL KCl (1 M), for the microcosm soil, or directly from slurry supernatant. Because NO2− concentration was below the level of detection, NOx is expressed as μg NO3−-N g−1 dry soil (ppm). Nitrification inhibition was assessed as the decrease in nitrate concentration as a percentage of that of Craibstone soil at each time point.
Molecular analysis
Nucleic acids were extracted from 0.5 g soil as previously described (Nicol et al.2005), suspended in diethylpyrocarbonate-treated water and immediately stored at –80°C. An aliquot was treated with DNase and the RNA was reverse transcribed, as described previously (Tourna et al.2008). The nucleic acid not used for cDNA generation was considered DNA only and its concentration was estimated using a NanoDrop 1000 Spectrophotometer (Thermo Scientific, Loughborough, UK).
Archaeal and bacterial amoA genes, which encode subunit A of ammonia monooxygenase, were quantified in a MasterCycler thermal cycler (Eppendorf, Hamburg, Germany) using standard curves, as described previously (Catão et al.2016). PCR amplification was carried out using primers crenamo23f and crenamo616r for archaeal amoA genes (Tourna et al.2008) and amoA1F and amoA2R for bacterial amoA genes (Rotthauwe, Witzel and Liesack 1997). Each 20-μL reaction contained 1 × QuantiFast PCR Master Mix (for AOA) or QuantiTect Master Mix (for AOB) (Qiagen, Crawley, UK), 0.4 μM of each primer for AOA amoA or 0.6 μM of each primer for AOB amoA, 2 μg μL−1 BSA (Promega) and 2 μL DNA (or cDNA). Archaeal amoA genes and transcripts were amplified using the following cycling conditions: 15 min at 95°C, followed by 40 cycles of 15 s at 94°C and 90 s at 60°C. Bacterial amoA genes and transcripts were amplified using the following cycling conditions: 15 min at 95°C, followed by 45 cycles of 1 min at 94°C, 1 min at 55°C and 1 min at 72°C. To exclude fluorescence contamination of potential primer dimers, SYBR Green fluorescence was measured after 5 s at 80°C or after 8 s at 83°C, for AOA and AOB, respectively. Melting curves between 65°C and 95°C were analysed for each run. AOB amoA transcripts were below the detection limit (5 copies μL−1). Efficiency of amplification and r2 for DNA were, respectively, 0.92 and 0.998 for archaeal amoA and 104.6 and 0.993 for bacterial amoA.
AOA community composition in soil slurries was assessed before and after incubation for 21 days by denaturing gradient gel electrophoresis (DGGE) of archaeal amoA gene using the primers described above in a linear gradient of 15%–55% denaturant, as described previously (Nicol et al.2005).
Statistical analysis
All analyses were conducted using R version (3.2.2). The effect of aqueous soil extracts on pure AOA and AOB cultures was analysed by testing differences in specific growth rate between treatments by one-way analysis of variance. Differences between nitrification rate in soil slurries were evaluated using a repeated-measures linear mixed model (package nlme) (Pinheiro et al.2015). Each slurry was considered a subject with random effect to analyse the effect of treatment (Campo sujo soil, Craibstone soil or soil mixture), time and their interaction on inorganic N concentration and amoA gene (and transcript) abundance. The NO3− concentration in the Campo sujo slurries was below the limit of detection; therefore, these samples were excluded from the analysis. Gene abundance data were log-transformed to achieve a normal distribution. When the interaction between independent variables was not significant, it was removed to analyse the effect of time or treatment independently over concentration of soil NH4+ and NOx. Two-way analysis of variance, with treatment and time as independent factors, was performed to evaluate differences in mineralisation and NO3− in the soil microcosms.
RESULTS
Effects of soil extracts on ammonia oxidiser cultures
To assess the presence of nitrification inhibitors in the soil, pure cultures of four AOB and one AOA were grown in liquid batch culture in medium containing aqueous soil extracts, water (negative control) or allylthiourea (positive control). Extracts from Campo sujo and Craibstone soils had no significant effect on the growth of any of the ammonia-oxidising strains tested (Fig. 1 and Fig. S1, Supporting Information). Allylthiourea completely inhibited all AOB cultures tested, but did not inhibit the growth of the AOA Candidatus Nitrosocosmicus franklandus (Fig. 1 and Fig S1).
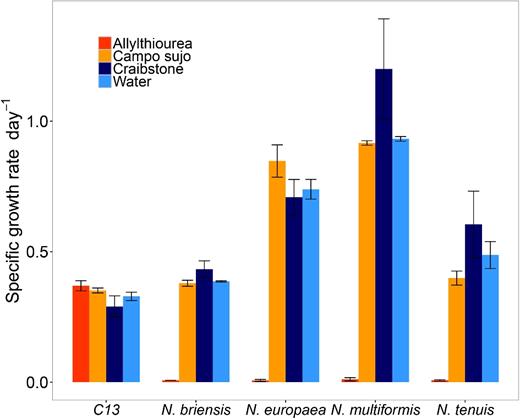
Effect of aqueous extracts of Campo sujo and Craibstone soils on the growth of ammonia oxidiser. Maximum specific growth rate was estimated during exponential growth of the ammonia-oxidising archaea (AOA) Candidatus Nitrosocosmicus franklandia (C13) and four soil ammonia-oxidising bacteria (N. briensis, N. europaea, N. multiformis, N. tenuis) in liquid batch cultures containing aqueous extracts of Campo sujo soil or Craibstone soil, water (negative control), or 100 μM allylthiourea (positive control). Error bars represent standard deviation of the mean from triplicate cultures.
Effects of Campo sujo soil on nitrification in Craibstone soil
Soil slurries containing Campo sujo soil, Craibstone soil or a mixture of the two soils (at ratios of 1:1 and 4:1) were incubated for up to 21 days. In all slurries, pH increased (0.4 in the Campo sujo soil, 0.8 in Craibstone soil, but only 0.1 and 0.2 for the 1:1 and 4:1 mixed samples, respectively) after the first day of incubation but did not change significantly thereafter. Net NH4+ concentration after 21 days ranged from 0.62 (±0.02) to 1.76 (±0.39) ppm (mg L−1 soil solution) for Craibstone soil and 0.87 (±0.02) to 2.20 (±0.02) ppm (mg L−1 soil solution) for Campo sujo soil (Fig. 2). Initial NH4+ concentration was higher in the mixed soil slurries than in controls, but the mixed slurries accumulated less NH4+ over the incubation period. The greatest increase in NH4+ concentration after 21 days was observed in Craibstone soil (2.9-fold).
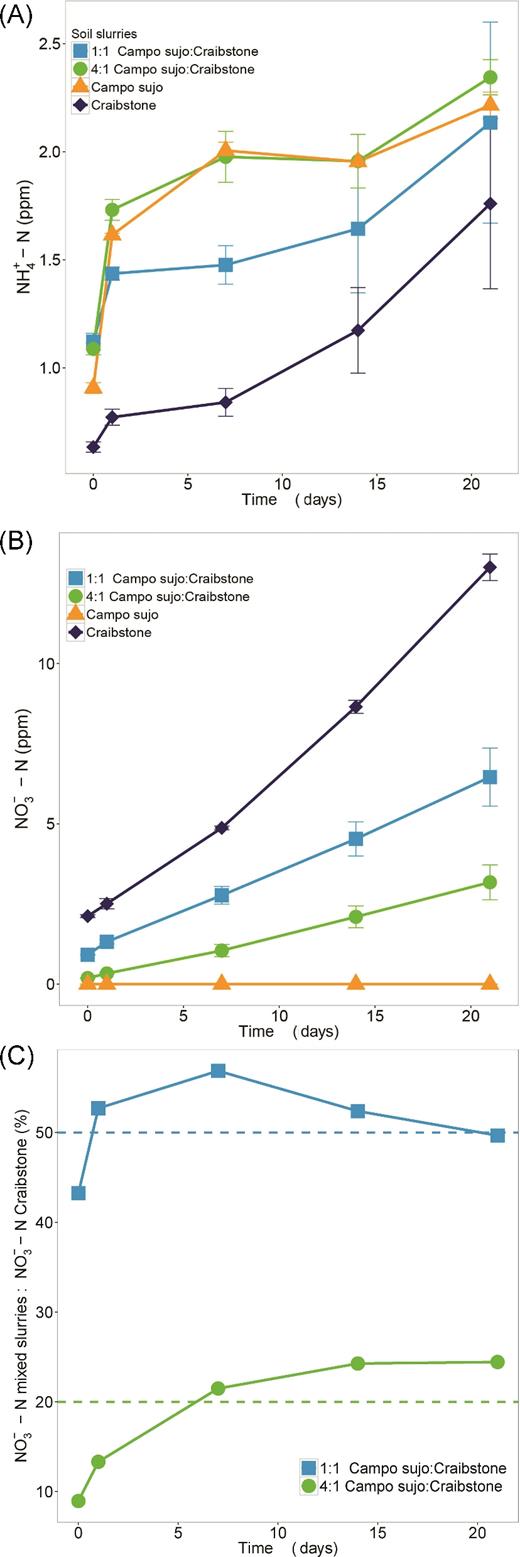
Changes in inorganic N concentration during incubation of slurries of Craibstone soil, Campo sujo soil and mixtures of these soils. (A) NH4+-N concentration and (B) NO3− -N were determined in all soil slurries. (C) NO3− concentration in the mixed slurries was expressed as the percentage of NO3− in the Craibstone soil slurry. P values for treatment, time and their interaction were calculated with a linear mixed model with repeated measures (lme4 package, R version 3.2.3) for each independent variable and their interaction, and the marginal r2 associated with the fixed effects. Error bars represent standard deviation of the means from triplicate cultures. Values below the limit of detection were plotted as zero.
NO3− concentration also increased in all soil slurries during incubation (P < 0.0001, Fig. 2B), except in those containing Campo sujo only, in which NO3− was below the detection limit. In the mixed soil slurries, NO3− production was equivalent to or higher than the 50% and 20% expected for the 1:1 and 4:1 ratios of Campo sujo soil and Craibstone soil, respectively (Fig. 2C), providing no evidence for inhibition of Craibstone soil nitrification by Campo sujo soil.
Ammonia oxidiser amoA gene abundance in the soil slurries did not change significantly during the incubation period, even when significant NO3− accumulation was observed (Fig. 3). AOA amoA abundance in the Campo sujo-only slurries was approximately three orders of magnitude lower than that of Craibstone-only slurries (Fig. 3A). AOA amoA abundance in mixed soil slurries was lower than that of Craibstone-only slurries until day 14, after which differences were not significant (P = 0.132). AOB amoA gene abundance in the Campo sujo-only slurries was also approximately three orders of magnitude lower than that of Craibstone-only slurries, and even significantly different (P = 0.024) at day 21, when Campo sujo-only AOB abundance was no longer significantly different from the those in the mixed samples (Fig. 3B).
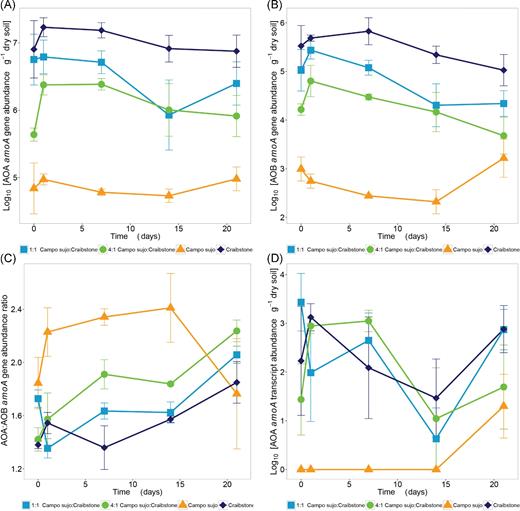
Changes in amoA gene abundance in slurries of Craibstone soil, Campo sujo soil and mixtures of these soils. (A) Ammonia-oxidising archaea (AOA) amoA, (B) ammonia-oxidising bacteria (AOB) amoA, (C) AOA:AOB amoA ratio and (D) AOA amoA transcripts were quantified during incubation of soil slurries. P values for treatment, time and their interaction were calculated with a linear mixed model with repeated measures (lme4 package, R version 3.2.3) for each independent variable and their interaction, and the marginal r2 associated with the fixed effects. Error bars represent standard deviation of the means from triplicate cultures.
The AOB amoA gene abundance was lower than AOA amoA gene abundance in all slurries at each time point. The AOA:AOB amoA gene ratio did not change significantly in the Campo sujo-only slurries but increased in the Craibstone-only and mixed soil slurries (Fig. 3C). In all slurries, AOB amoA transcripts were below the level of detection (5 μL−1). The AOA amoA transcripts were detected in all slurries containing Craibstone soil throughout incubation but were detected in the Campo sujo-only slurries only at day 21 (Fig. 3D).
Before incubation, DGGE profiles of amoA genes amplified from Craibstone soil contained more bands (potential OTUs) than profiles of Campo sujo soil (Fig. S2, Supporting Information) and did not change significantly after incubation for 21 days. DGGE profiles of the AOA amoA genes in the 1:1 mixed slurry were similar to those of Craibstone soil, possibly masking the less abundant amoA genes from the Campo sujo soil (Fig. S2).
Effects of soil pH and moisture content
The effects of pH and moisture content on nitrification in Campo sujo soil were investigated in soil microcosms. Net mineralisation was determined as the increase in concentration of inorganic N (NH4+-N + NO3− -N) during incubation, assuming that other nitrogen cycle processes were not significant (Fig. S3, Supporting Information). Mineralisation in the dry soil did not increase after wetting, in contrast to the expected ‘Birch’ effect (Birch 1964) (Fig. S3). In addition, soil pH did not change significantly with time in the microcosms and remained at 5.2 for the ‘water pulse’, ‘moist’ and ‘dry’ treatments and at 6.3 for the ‘pH’ treatment, in which pH was increased artificially with CaCO3. Nitrate concentration did not increase significantly in any of the treatments (Fig. S3), and no significant difference was observed between treatments (P = 0.14). No significant differences were observed between samples incubated with or without acetylene, except for NO3−-N concentrations in the ‘moist’ microcosms. After incubation for 21 days, the NO3− -N concentration was lower in the acetylene-treated moist microcosms than in those without added acetylene (Fig. S3).
DISCUSSION
Nitrification is frequently undetectable in undisturbed Cerrado ecosystems, although management and conversion to agricultural production increases nitrate production (Catão et al.2016). Previous studies suggest low abundance of AOA and AOB in Campo sujo soil (Catão et al.2016), which is also characterised by sparse shrubs over a continuous grass layer. The aim of this work was to determine whether the lack of nitrification and low abundance of ammonia oxidisers in this ecosystem were due to low pH, low soil moisture, NH4+ limitation or biological inhibition of ammonia oxidation.
Certain plants release biological nitrification inhibitors that suppress ammonia oxidation in soils (Subbarao et al.2015). For example, compounds produced by Brachiaria (Subbarao et al.2009) and Sorghum (Zakir et al.2008) inhibited a recombinant Nitrosospira europaea strain, possibly by blocking ammonia monooxygenase and hydroxylamine oxidoreductase (Subbarao et al.2008). Production of biological nitrification inhibitors can be promoted by exposure to high NH4+:NO3− ratios (Subbarao et al.2015), such as those found in Campo sujo soil (Catão et al.2016). However, aqueous extracts of Campo sujo soil did not inhibit growth of cultures of four AOB and one AOA, all of which were isolated from neutral to alkaline soils. Allylthiourea, used as a positive control, prevented growth of the AOB cultures but not the AOA culture, which is consistent with other studies reporting a greater tolerance of AOA to allylthiourea (Hatzenpichler and Lebedeva 2008; Stempfhuber et al.2015). This result demonstrates the need to test potential inhibitors against both AOA and AOB, rather than N. europaea only.
Cultivation-based studies were performed using aqueous soil extracts, and a small number of cultivated strains and potential inhibition was therefore assessed more directly by mixing Campo sujo soil with Craibstone soil, a strongly nitrifying soil with similar pH, in soil slurries (Nicol et al.2008; Zhang et al.2010). The soil slurries also provided no evidence of nitrification inhibitors in Campo sujo soil. Nitrate accumulation in mixtures of Craibstone soil and Campo sujo soil was lower than that of Craibstone soil only, but this difference was less than or equal to that predicted by the lower volume of Craibstone soil in the slurry, suggesting that nitrification was not inhibited by the Campo sujo soil. Similarly, the addition of Campo sujo soil to Craibstone soil had no apparent effect on AOA and AOB amoA gene abundances. Archaeal amoA genes were more abundant than those of bacteria in the soils, and bacterial amoA gene expression was not detected, as reported by previous studies of Craibstone soils (Zhang et al.2010). Neither AOA nor AOB amoA abundance changed significantly during incubation of any of the slurries containing Craibstone soil, despite evidence of nitrate production. However, the AOA:AOB amoA gene ratio increased, suggesting greater growth or lower death rates of AOA, but there was no evidence for growth of AOB or AOA in the Campo sujo soil. DGGE profiles of the Campo sujo soil contained fewer archaeal amoA bands than those of Craibstone soil, providing a further indication of low abundance and activity of ammonia oxidisers in Campo sujo soil. Although AOA amoA transcripts in Campo sujo-only slurries increased after incubation for 21 days, this increased gene expression did not appear to lead to a detectable level of nitrate. Nitrate reduction during denitrification was considered negligible due to previous experiments showing low NO emissions and undetectable N2O in Cerrado soils (Pinto et al.2002).
In the absence of evidence for BNI, microcosm studies were performed to determine whether the low nitrification rates in Campo sujo soil were due to low pH or low soil moisture content. Gas measurements in Cerrado soil after rainfall (natural or simulated) led to an increase in NO emissions (Pinto et al.2002), which may result from the Birch effect (Birch 1964) of increased organic matter availability after rewetting (Fierer and Schimel 2003). Soil pH is an important determinant of microbial diversity (Lauber et al.2009; Fierer et al.2012) and influences soil ammonia oxidiser abundance and activity (de Boer and Kowalchuk 2001; Nicol et al.2008), with higher transcriptional activity of Archaea than Bacteria as pH decreases (Nicol et al.2008). In our experiment, higher soil pH increased organic nitrogen mineralisation rate but did not lead to detectable nitrate production in Campo sujo soil after incubation for 28 days. Mineralisation was also lower in moist soil than in other treatments, and the increase in moisture did not lead to detectable nitrate production (see Fig. S3). Even though it can be argued that NO3− can be denitrified at higher soil moisture, low or undetectable NO and N2O emission in field measurements over the seasons (Cruvinel et al.2011) and no consistent NO3− increase after 1 day or after 3 weeks of incubation suggested limitation of nitrification by other factors.
The low nitrification and low ammonia oxidiser abundance of Campo sujo soil, in both microcosms and slurries, were not due to NH4+ limitation. The NH4+ concentration of Campo sujo soil slurries was even higher than that of Craibstone soil slurries at the beginning of the experiment. Jack pine forest soils showed similar high concentrations of ammonium without detectable nitrate (Ste-Marie and Paré 1999). None of the treatments in this study increased nitrification in Campo sujo soil, and this soil did not inhibit nitrification in Craibstone soil or pure cultures of AOA or AOB. Similarly, in the jack pine forest soil, nitrification was not stimulated by increased pH or ammonium amendment but was stimulated by the addition of nitrifying soil from a forest floor (Ste-Marie and Paré 1999). In this study, both AOA and AOB were detected in Campo sujo soil, but at low levels that are unlikely to lead to detectable nitrate production. Consequently, these soils have much greater capacity to retain N as NH4+ through ion exchange, with minimal NO3− leaching. Furthermore, NO pulses observed after rainfall are not due to nitrifier activity. However, experiments performed either for longer than 3 weeks or with rhizosphere soil might detect differences in nitrifier inhibition/stimulation and, despite the small effect of pH and H2O on the Brazilian savanna soil nitrification rate, archaeal ammonia oxidisers started to show activity in slurries after 21 days of incubation. Taken together, our results show that low nitrification rates and ammonia oxidiser abundance in Campo sujo soil are not due to low moisture content, low pH or the presence of ammonia oxidiser inhibitors.
Although low nitrate concentrations are unlikely to be due to denitrification, nitrate assimilation and dissimilatory nitrate reduction may reduce nitrate produced by nitrifiers. However, the high ammonium concentrations, low AOA and AOB abundances, and lack of evidence for ammonia oxidiser activity and growth suggest inhibition or limitation of ammonia oxidiser growth and activity. This study was performed using soil associated with one type of vegetation sampled at the beginning of the dry season. Production of BNI may vary seasonally and with vegetation, but low nitrification rates are found in soils sampled throughout the year (Nardoto and Bustamante 2003) and ammonium and nitrate concentrations are high and low (2.5 (±0.5) μg N-NH4+ g−1 dry soil and 0.072 (±0.01) μg N-NO3− g−1 dry soil), respectively, regardless of vegetation type (Catão et al. in preparation). Inhibition of ammonia oxidiser cultures was performed with water extracts from soil, and it is possible that ammonia oxidisers were inhibited by water insoluble BNI. Other features of Cerrado soils may also be important. There is evidence for co-limitation of microbial decomposition in these soils by N and P (Kozovits et al.2007; Jacobson, da Bustamante and Kozovits 2011) and ammonia oxidisers may have been limited by P and other nutrients, although mixing with Craibstone soil would be expected to relieve this limitation. Cerrado soils are deficient in P (Goedert 1983), and the values observed for the Campo sujo are similar to the upper layer of other Cerrado soils (Parron, Bustamante and Markewitz 2011), where soil P sorption capacity is often related to Fe and Al contents (Goedert 1983). The soils investigated in this study also contain relative high Fe concentrations (165.4 ± 41.01 mg dm−3), which have been associated with reduction in net nitrification and AOA and AOB amoA gene abundance in subtropical acid soils (Jiang et al.2015). Although these possibilities suggest future experimental studies, the reasons for low nitrification rates and low ammonia oxidiser abundances in Cerrado soil remain unclear and this study provided no evidence for ammonia limitation, pH or inhibition by water extractable inhibitors.
SUPPLEMENTARY DATA
Supplementary data are available at FEMSEC online.
Acknowledgements
The authors would like to thank Professor Paul Hallett (University of Aberdeen) for advice and helpful discussions on soil physics and soil matric potential; Fabiano Bielefeld Nardoto, owner of the Tabapuã dos Pireneus farm, for allowing soil sampling; and the Scottish Agricultural College Craibstone Estate (Aberdeen, UK) for access to the Woodlands Field pH plots.
FUNDING
This work was supported by grants from the National Council of Technological and Scientific Development (CNPq); Brazilian Federal Agency for Support and Evaluation of Graduate Education (CAPES) to E. Catão; Foundation for Research Support of Distrito Federal (FAP-DF); and funding of the European Comission's FP7 programme, EU-project Ecological Function and Biodiversity Indicators in European Soils ‘EcoFINDERS’, no. 264465.
Conflict of interest. None declared.