-
PDF
- Split View
-
Views
-
Cite
Cite
Dániel G. Knapp, Gábor M. Kovács, Interspecific metabolic diversity of root-colonizing endophytic fungi revealed by enzyme activity tests, FEMS Microbiology Ecology, Volume 92, Issue 12, December 2016, fiw190, https://doi.org/10.1093/femsec/fiw190
- Share Icon Share
Although dark septate endophytes (DSE) represent a worldwide dispersed form group of root-colonizing endophytic fungi, our knowledge on their role in ecosystem functioning is far limited. In this study, we aimed to test if functional diversity exists among DSE fungi representing different lineages of root endophytic fungal community of semiarid sandy grasslands. To address this question and to gain general information on function of DSE fungi, we adopted api-ZYM and BioLog FF assays to study those non-sporulating filamentous fungi and characterized the metabolic activity of 15 different DSE species. Although there were striking differences among the species, all of the substrates tested were utilized by the DSE fungi. When endophytes characteristic to grasses and non-grass host plants were separately considered, we found that the whole substrate repertoire was used by both groups. This might illustrate the complementary functional diversity of the communities root endophytic plant-associated fungi. The broad spectra of substrates utilized by these root endophytes illustrate the functional importance of their diversity, which can play role not only in nutrient mobilization and uptake of plants from with nutrient poor soils, but also in general plant performance and ecosystem functioning.
INTRODUCTION
Fungal endophytes colonize plant tissues without causing visible symptoms on the host (Saikkonen et al. 1998) and can be found in the vast majority of plant species (Porras-Alfaro and Bayman 2011; Card et al. 2016). These diverse endophytes can be grouped based on several features among which the plant organ colonized generally has major significance (e.g. see in Rodriguez et al. 2009). Similarly to other plant organs, roots are also colonized by broad spectra of fungal endophytes with potentially diverse function (Vandenkoornhuyse et al. 2002). Dark septate endophytes (DSE) compose a form group of root endophytes with melanized septated intraradical hyphae (Jumpponen and Trappe 1998). Although their name referring to pigmented hyphae persists in the literature, some species can have hyaline structures and the degree of melanization of the hyphae can be highly variable (Peterson, Wagg and Pautler 2008). DSE fungi are frequent in environments with strong abiotic stress, such as arid and semiarid ecosystems (Jumpponen and Trappe 1998; Mandyam and Jumpponen 2005; Knapp, Pintye and Kovács 2012). These fungi are asexual (but see Knapp et al. 2015) and even their asexual spore production is rare. Although DSE fungi share in planta morphological features, they belong to several distant lineages of Ascomycotina (Addy, Piercey and Currah 2005; Andrade-Linares and Franken 2013; Sieber and Grünig 2013).
DSE fungi are worldwide dispersed, but their roles in ecosystem functioning are still elusive (Mandyam and Jumpponen 2005) especially when compared to our knowledge on the mutualistic root-colonizing mycorrhizal fungi (Smith and Read 2008). Different functions can be assigned to DSE fungi based on inoculation experiments with several species. For example, Harpophora oryzae was reported to form biotrophic interaction with the host and increases plant resistance to a pathogen (Su et al. 2013). DSE species were found to be able for enzymatic degradation of detrital organic nutrients (e.g. Caldwell, Jumpponen and Trappe 2000). Although the possibility of direct nutrient exchange across symbiotic structures (e.g. across hyaline hyphae) was raised in previous studies (e.g. Mandyam and Jumpponen 2008) and bidirectional nutrient transfer was measured (Usuki and Narisawa 2007), reciprocal transfer between fungi and plants has not unambiguously been confirmed to date. Several studies hypothesized that DSE fungi might be important as (latent) saprobes and play role in host nutrition by degrading substrates (Mandyam and Jumpponen 2005; Porras-Alfaro et al. 2008; Mandyam, Loughin and Jumpponen 2010). These root endophytes might help the degradation of soil organic matter similarly to e.g. ericoid mycorrhizal fungi, which possess wide range of saprotrophic capabilities and are involved in the mobilization of complex polymers of nutrient poor soils providing nutritional benefits for plant partners (Read and Perez-Moreno 2003; Smith and Read 2008). However to date, substrate-degrading capability and enzymatic activity of only few DSE fungi were tested. For example, Caldwell, Jumpponen and Trappe (2000) studied Cadophora finlandica (syn. Phialophora finlandia), Phialocephala fortinii and six related DSE isolates, and proved the production of variety of extracellular enzymes and the utilization of different carbon, nitrogen and phosphorus sources. In their review, Mandyam and Jumpponen (2005) summarized the results of previous studies of carbohydrate-degrading and proteolytic enzymes of DSE fungi. Mandyam, Loughin and Jumpponen (2010) investigated the hydrolyzation of complex carbon molecules by five enzymes of eight and two different isolates of two DSE species.
Data on enzyme production and substrate utilization of soil microbes can be used for assessing metabolic diversity and enables metabolic fingerprinting, characterization and delimitation of taxa of different microorganisms (Nannipieri, Kandeler and Ruggiero 2002). With the help of different enzyme assays, high amount of metabolic information could relatively easily be gained. One of those tests is the api-ZYM assay, originally designed for fingerprinting of bacteria, is a simple, rapid, semiquantitative test of 19 constitutively expressed lipid, protein and carbohydrate-degrading enzymes of non-pretreated organisms (e.g. Humble, King and Phillips 1977). It has been used to characterize both communities (e.g. Bending, Turner and Jones 2002) and species or serotypes (e.g. Ahmed et al. 2013) of bacteria. Up to now only few studies examined fungal enzyme activities with this test (e.g. Bidochka, Burke and Ng 1999; Tekere et al. 2001), perhaps because its application for filamentous fungi, especially for non-sporulating taxa, is challenging. Another assay for physiological characterization, BioLog FF MicroPlate was designed to identify and/or characterize sporulating fungi and yeasts. MicroPlates supplied with 95 different carbon sources enable fingerprinting of metabolic activity of fungi based on the degree of substrate degradation. BioLog FF test has been used for e.g. characterization of closely related fungal species (e.g. Singh 2009) and was successfully applied to distinguish different genera and species, e.g. in the case of the well-known root-colonizing Oiodendron species (Rice and Currah 2005).
Besides our poor knowledge on saprobic capabilities of DSE fungi, the role of ascomycetes in decomposition generally is not well known compared to roles of basidiomycetes in decomposition of plant material (Baldrian et al. 2011). As generally ascomycetes dominate in root-associated endophytic fungal communities of semiarid grasslands (e.g. Porras-Alfaro et al. 2008; Herrera et al. 2010; Knapp, Pintye and Kovács 2012), investigation of saprobic capabilities of the frequent ascomycetous DSEs could provide information on their general role in ecosystem functioning.
During previous mycorrhizal status study of plant communities of sandy areas on the Great Hungarian Plain, the majority of the plant species was found to be colonized by DSE fungi (Kovács and Szigetvári 2002). Thereafter, in the compositional diversity study of root endophytic fungi of those areas, we identified 15 lineages of DSE fungi (Knapp, Pintye and Kovács 2012; Knapp et al. 2015) most of which were found to be common in other arid and semiarid areas, too (Knapp, Pintye and Kovács 2012; Knapp et al. 2015). Functional interspecific heterogeneity of fungi representing same life style is a well-known phenomenon (e.g. Munkvold et al. 2004; Eastwood et al. 2011), and this diversity may have important role in niche segregation and functioning as showed for e.g. mycorrhizal fungi (e.g. Sanders et al. 1998; van der Heijden et al. 1998; Bever et al. 2001; Munkvold et al. 2004). Based on the common presence of DSE fungal taxa in the same environments, microhabitats and even in the same roots, we hypothesized that there might be a functional heterogeneity coupled with taxonomical diversity of root-colonizing DSE fungi. To address this question and to gain general information on function of DSEs, we aimed to adopt api-ZYM and BioLog FF assays and use them for the characterization of metabolic activity of 15 different species of filamentous non-sporulating root endophytic fungi.
MATERIALS AND METHODS
One isolate of each of the 15 DSE lineages (Knapp, Pintye and Kovács 2012; Knapp et al. 2015) originating from Hungarian semiarid sandy grasslands was involved into this study (Table 1). These ascomycetous species represent different genera and orders; some of them are associated with grasses others with dicots (woody and herbaceous plants) and some colonize both (Table 1). Here we grouped these fungal species into four categories according to the host plants: DSE fungi associated with (i) woody or herbaceous plants, (ii) gramineous hosts, (iii) both grasses and woody or herbaceous plants and DSEs with (iv) ambiguous host preferences due to the few number of isolates (Table 1). All of these 15 fungi have been considered as DSE based on our in vitro resynthesis experiments (Knapp, Pintye and Kovács 2012): strains were considered as DSE if they colonized the roots, formed microsclerotia and caused no visible negative effect on the plant. Details on the area, the isolation and storage of the strains were published previously (Knapp, Pintye and Kovács 2012). Thirteen DSE isolates could be classified at species or genus level—we use those names in this study—whereas two isolates only on higher taxonomic level (Table 1). One of them, Helotiales sp. REF052, probably represents a novel taxa in the order Helotiales. Although Eurotiales sp. REF165 has identical ITS sequence with the type of Penicillium nodositatum (strain: CBS 333.90, GenBank accession: NR_103703.1), as the taxonomy of this species is ambiguous and has been excluded from Penicillium sensu stricto (Visagie et al. 2013), to avoid misunderstandings, we do not use the genus/species name.
DSE group1 . | DSE taxon . | Isolate no. . | Order . | Host plant2 . |
---|---|---|---|---|
DSE-1 | Cadophora sp. | DSE1049a | Helotiales | Non-grass |
DSE-2 | Helotiales sp. | REF052b | Helotiales | Non-grass |
DSE-3 | Rhizopycnis vagum | REF081b | Pleosporales | Non-grass |
DSE-4 | Aquilomyces patris | CBS135661b,c | Pleosporales | Non-grass |
DSE-5 | Alternaria sp. | REF104b | Pleosporales | Uncertain |
DSE-6 | Setophoma terrestris | REF109b | Pleosporales | Non-grass |
DSE-7 | Darksidea alpha | CBS135650b,c | Pleosporales | Grass |
DSE-8A* | Flavomyces fulophazii | CBS135731b,c | Pleosporales | Grass |
DSE-8B* | Periconia macrospinosa | DSE2036 | Pleosporales | Grass |
DSE-9 | Embellisia sp. | REF147b | Pleosporales | Mixed |
DSE-10 | Curvularia sp. | REF156b | Pleosporales | Mixed |
DSE-11 | Microdochium bolley | REF158b | Xylariales | Grass |
DSE-12 | Eurotiales sp. | REF165b | Eurotiales | Uncertain |
DSE-13 | Plectosphaerella sp. | REF166b | Hypocreales | Uncertain |
DSE-14 | Fusarium sp. | REF210b | Hypocreales | Uncertain |
DSE group1 . | DSE taxon . | Isolate no. . | Order . | Host plant2 . |
---|---|---|---|---|
DSE-1 | Cadophora sp. | DSE1049a | Helotiales | Non-grass |
DSE-2 | Helotiales sp. | REF052b | Helotiales | Non-grass |
DSE-3 | Rhizopycnis vagum | REF081b | Pleosporales | Non-grass |
DSE-4 | Aquilomyces patris | CBS135661b,c | Pleosporales | Non-grass |
DSE-5 | Alternaria sp. | REF104b | Pleosporales | Uncertain |
DSE-6 | Setophoma terrestris | REF109b | Pleosporales | Non-grass |
DSE-7 | Darksidea alpha | CBS135650b,c | Pleosporales | Grass |
DSE-8A* | Flavomyces fulophazii | CBS135731b,c | Pleosporales | Grass |
DSE-8B* | Periconia macrospinosa | DSE2036 | Pleosporales | Grass |
DSE-9 | Embellisia sp. | REF147b | Pleosporales | Mixed |
DSE-10 | Curvularia sp. | REF156b | Pleosporales | Mixed |
DSE-11 | Microdochium bolley | REF158b | Xylariales | Grass |
DSE-12 | Eurotiales sp. | REF165b | Eurotiales | Uncertain |
DSE-13 | Plectosphaerella sp. | REF166b | Hypocreales | Uncertain |
DSE-14 | Fusarium sp. | REF210b | Hypocreales | Uncertain |
Host plant types to which isolates of the DSE species are generally associated based on our published and unpublished results; non-grass: woody or herbatious plants, grass: gramineous hosts, mixed: grasses and woody or herbatious hosts, uncertain: the species represented by few isolates and cannot clearly assigned to host types; aStrain introduced by Vági et al. (2014); bStrains introduced by Knapp, Pintye and Kovács (2012); cStrains introduced by Knapp et al. (2015).
DSE group1 . | DSE taxon . | Isolate no. . | Order . | Host plant2 . |
---|---|---|---|---|
DSE-1 | Cadophora sp. | DSE1049a | Helotiales | Non-grass |
DSE-2 | Helotiales sp. | REF052b | Helotiales | Non-grass |
DSE-3 | Rhizopycnis vagum | REF081b | Pleosporales | Non-grass |
DSE-4 | Aquilomyces patris | CBS135661b,c | Pleosporales | Non-grass |
DSE-5 | Alternaria sp. | REF104b | Pleosporales | Uncertain |
DSE-6 | Setophoma terrestris | REF109b | Pleosporales | Non-grass |
DSE-7 | Darksidea alpha | CBS135650b,c | Pleosporales | Grass |
DSE-8A* | Flavomyces fulophazii | CBS135731b,c | Pleosporales | Grass |
DSE-8B* | Periconia macrospinosa | DSE2036 | Pleosporales | Grass |
DSE-9 | Embellisia sp. | REF147b | Pleosporales | Mixed |
DSE-10 | Curvularia sp. | REF156b | Pleosporales | Mixed |
DSE-11 | Microdochium bolley | REF158b | Xylariales | Grass |
DSE-12 | Eurotiales sp. | REF165b | Eurotiales | Uncertain |
DSE-13 | Plectosphaerella sp. | REF166b | Hypocreales | Uncertain |
DSE-14 | Fusarium sp. | REF210b | Hypocreales | Uncertain |
DSE group1 . | DSE taxon . | Isolate no. . | Order . | Host plant2 . |
---|---|---|---|---|
DSE-1 | Cadophora sp. | DSE1049a | Helotiales | Non-grass |
DSE-2 | Helotiales sp. | REF052b | Helotiales | Non-grass |
DSE-3 | Rhizopycnis vagum | REF081b | Pleosporales | Non-grass |
DSE-4 | Aquilomyces patris | CBS135661b,c | Pleosporales | Non-grass |
DSE-5 | Alternaria sp. | REF104b | Pleosporales | Uncertain |
DSE-6 | Setophoma terrestris | REF109b | Pleosporales | Non-grass |
DSE-7 | Darksidea alpha | CBS135650b,c | Pleosporales | Grass |
DSE-8A* | Flavomyces fulophazii | CBS135731b,c | Pleosporales | Grass |
DSE-8B* | Periconia macrospinosa | DSE2036 | Pleosporales | Grass |
DSE-9 | Embellisia sp. | REF147b | Pleosporales | Mixed |
DSE-10 | Curvularia sp. | REF156b | Pleosporales | Mixed |
DSE-11 | Microdochium bolley | REF158b | Xylariales | Grass |
DSE-12 | Eurotiales sp. | REF165b | Eurotiales | Uncertain |
DSE-13 | Plectosphaerella sp. | REF166b | Hypocreales | Uncertain |
DSE-14 | Fusarium sp. | REF210b | Hypocreales | Uncertain |
Host plant types to which isolates of the DSE species are generally associated based on our published and unpublished results; non-grass: woody or herbatious plants, grass: gramineous hosts, mixed: grasses and woody or herbatious hosts, uncertain: the species represented by few isolates and cannot clearly assigned to host types; aStrain introduced by Vági et al. (2014); bStrains introduced by Knapp, Pintye and Kovács (2012); cStrains introduced by Knapp et al. (2015).
Isolates were cultured onto modified MMN (Marx 1969) media covered by cellophane foil enabling us to easily remove mycelium without remnants of the medium. Growing characteristics varied among the isolates, and the slowest growing species were Helotiales sp. REF052, Rhizopycnis vagum, Darksidea alpha and Plectosphaerella sp. REF166 compared to the others. The cultures were grown at room temperature in the dark for 10–12 days, and then ∼0.15–0.20 g mycelia were picked from the marginal region of the colonies. The mycelium was shredded in sterile conditions using scalpel and blades, and then soaked in Eppendorf tubes in 1 ml sterile distilled water (SDW) and in FF-IF broth (Biolog Inc., Hayward, CA, USA) for api-ZYM (API ZYM System, bioMérieux, Marcy-I'Etoile, France) and BioLog FF microplates (Biolog Inc., Hayward, CA, USA), respectively. The tubes were vortexed for 30 min for the thorough disruption of the hyphal aggregates.
To prepare inoculum for api-ZYM assays, the 1 ml suspension was further diluted to 4 ml with SDW in a 15 ml Falcon tube and vortexed for 5 min. A 2 ml portion of suspension was taken, 65 μl of it was examined with light microscope to check that the inoculum content is roughly consistent and 65–65 μl was used for inoculation of the api-ZYM assays. The assays were incubated on 37°C for 4.5 h in dark. The manufacturer's instructions were followed in all subsequent steps (bioMérieux, Marcy-I'Etoile). Color reactions were estimated and scored from 0 to 5 according to the instructions. Values 1 to 5 were positive reactions with increasing strength depending on intensity, while 0 meant no reaction.
For the BioLog FF tests, mycelia were washed twice with IF-FF broth to reduce the effect of exudates and enzymes likely present on the hyphae, and then diluted in 14 ml IF-FF broth, and incubated on 26°C for 7 days (vortexed twice a day). Before inoculation of the MicroPlates, optical density (OD) measurement, microscopic examination and a quick viability test (growth test on agar) of the suspension were performed using 100 μl aliquots taken from each sample. The suspension was considered ready for inoculation, if OD of the samples was around 0.20 at 750 nm, equal mass of hyphal fragments were present and hyphal growth was observed on MMN media. Then, 100–100 μl of suspension was added into each of the 96 wells and BioLog FF MicroPlates were incubated at 26°C for 10 days. The first (A1) well of the plate served as a reference ‘control well’ providing information on activity of the suspension itself in absence of any carbon sources. Absorbance was read on 1, 2, 4, 7 and 10 days post-inoculation using a microplate reader (TECAN, Sunrise) at 492 and 750 nm, which correlates with oxidation of test substrate and turbidity of hyphal mass, respectively. Carbon source utilization is related to growing characteristics, which is highly variable among DSE species; we considered a carbon source as utilized, if the OD was higher than the control at both 492 and 750 nm on either the 4th or 10th days. Measurements on the 4th day were chosen according to the manual of BioLog-FF since the majority of the OD values reached their stationary phase by this time, while the results gained on the 10th day were used for some slow-growing isolates reached stationary phase later. OD of the 95 wells of the BioLog FF MicroPlate measured at 492 nm was standardized with OD of the control well, and the higher value of either the 4th or 10th day measurements was used for analyses. These values were classified into six categories: 0 (null reaction), 1 (0 < OD ≤ 0.25), 2 (0.25 < OD ≤ 0.5), 3 (0.5 < OD ≤ 1), 4 (1 < OD ≤ 1.5) and 5 (1.5 ≤ OD), which were used for principal component analysis (PCA), cluster analyses and heat map presentations. Previous studies using these tests, if described at all, used one measurement to characterize one taxa (e.g. Bidochka, Burke and Ng 1999; Singh et al. 2010; Ahmed et al. 2013) or used biological replicates (e.g. Kubicek et al. 2003; Druzhinina et al. 2010) or used both assumptions (Rice and Currah 2005). Using rough estimations (api-ZYM) and categorized values (BioLog FF) for characterization of enzyme activity, one measure could serve reliable information on general enzymatic activities of a fungus. The method applied here for non-sporulating filamentous fungi has much higher probability for false negative (i.e. non-inoculated wells) than for false positive (i.e. contaminations) results, so the metabolic activities found here are more probably underestimations than overestimations of functional diversity of those fungi.
Phylogenetic tree (Fig. 1) was inferred from a combined alignment of sequences of the internal transcribed spacer (ITS) region and 28S large subunit (LSU) of the nrDNA previously published in Knapp et al. (Knapp, Pintye and Kovács 2012; Knapp et al. 2015). Alignments were made using the online version of MAFFT v. 6 (Katoh and Toh 2008), maximum-likelihood analyses were carried out with RAxML (Stamatakis 2014) implemented in raxmlGUI v. 1.3 (Silvestro and Michalak 2012) and the tree was visualized using MEGA 5 (Tamura et al. 2011). The dendrograms of api-ZYM and BioLog data sets were constructed using the unweighted pair-group method with arithmetic average (UPGMA) method based on the coefficient of Nei and Li with TREECON (Van de Peer and De Wachter 1994). PCAs were conducted using PAST software (Hammer, Harper and Ryan 2001).
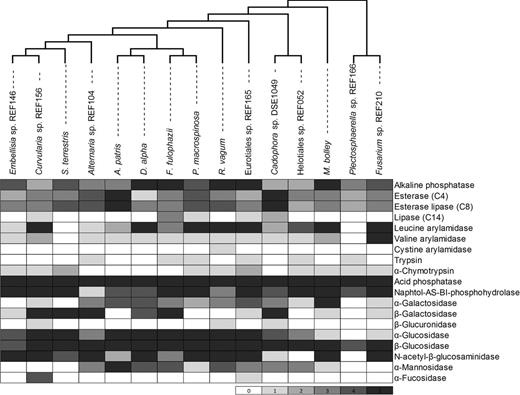
Constitutive enzyme production of the 15 DSE species determined with the api-ZYM tests. Cells are shaded according to the intensity of hydrolyzation of the 19 substrates of the test from zero (null reaction) to five (strongest reaction). The maximum likelihood (ML) tree was gained from the analysis of a combined dataset of ITS and LSU sequences.
RESULTS
With the successful adaptation of api-ZYM and BioLog FF MicroPlates systems non-sporulating filamentous fungi, we could test constitutive enzyme production and carbon source utilization of all 15 DSE species involved in the study. The inoculum preparation method presented here was successfully applied for both assays.
All of the 15 DSE species differed in the results of the enzyme activities measured with the api-ZYM assay. Each substrate of the test was degraded at least by one DSE fungus studied (Fig. 1). The majority of the species showed zero cystine arylamidase, α-fucosidase, β-glucuronidase activity, and only one, two and three species showed positive reaction for those enzymes, respectively (Fig. 1). Lipase (C14) and trypsin activities were detected in five and six DSE fungi, respectively. Besides the sparse activity of these five enzymes, DSE species could degrade majority of the 19 substrates of api-ZYM tests. All DSE species showed activity of alkaline phosphatase, esterase (C4), esterase lipase (C8), acid phosphatase, naphtol-AS-BI-phosphohydrolase and β-Glucosidase, among them acid phosphatase and β-glucosidase showed the most intensive reactions (Fig. 1). Eurotiales sp. REF165 showed the highest number of active enzymes (17 out of 19) followed by Cadophora sp. DSE1049, Rhizopycnis vagum, Flavomyces fulophazii (15) and Alternaria sp. REF104 and Microdochium bolley (14). On the other hand, constitutive enzymes of Plectosphaerella sp. REF166 were capable of degrading only eight substrates (Fig. 1).
According to the results gained with BioLog FF tests, all of the 15 DSE species had unique carbon source utilization profile. Each substrate could be degraded at least by four DSE species (Fig. 2). Periconia macrospinosa showed activity on the highest number (94) of substrates (Fig. 3): only uridine was not utilized by this species (Fig. 2). Periconia macrospinosa was followed by Eurotiales sp. REF165, Helotiales sp. REF052, M. bolley and Fusarium sp. REF210 with 93, 87, 86 and 85 carbon sources utilized, respectively (Fig. 3). Cadophora sp. DSE1049 could use the lowest number of sources: only 54 carbon substrates were used by this species (Fig. 3). A total of 27 carbon substrates were utilized by all of the 15 species. On the other hand, only less than half of the species could utilize glucuronamide, N-acetyl-D-galactosamine, N-acetyl-β-D-mannosamine, α-cyclodextrin, L-threonine, succinic acid mono-methyl-ester and 2-aminoethanol (Fig. 2). Based on our measurements, the level of substrate utilization of the 15 species was the highest on xylose and trehalose followed by sucrose, D-fructose, turanose, D-melibiose, α-keto-D-gluconic acid, stachyose and L-proline (Fig. 2).
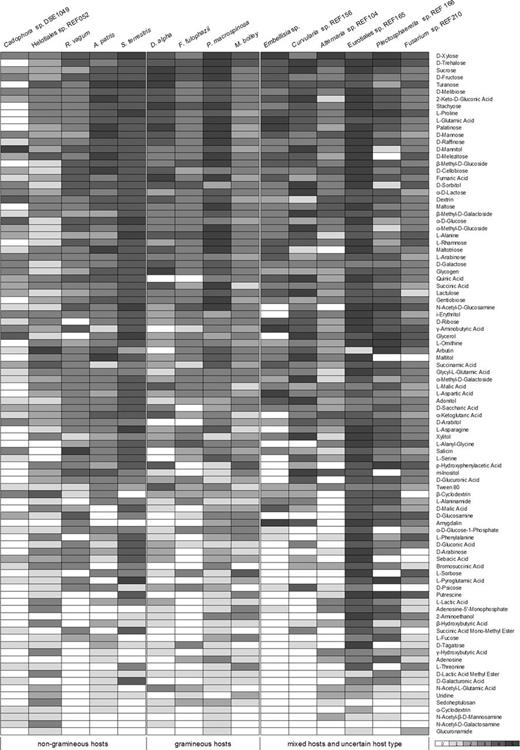
Carbon source utilization of the 15 DSE species gained from BioLog FF MicroPlates assay. The categorized values (0–5) are derived from measurements at 492 nm. Cells are shaded according to the intensity of utilization of the 95 carbon sources of the MicroPlates from zero (null reaction) to five (strongest reaction).
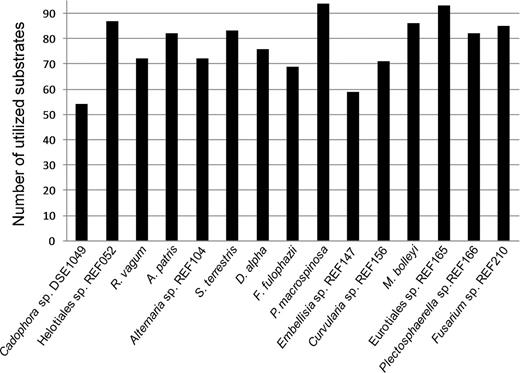
Number of utilized substrates out of the 95 carbon sources present on the BioLog FF MicroPlates by the 15 DSE fungi.
The clustering of 15 DSE species based on results of the api-ZYM tests and BioLog FF MicroPlates were different; only groups formed by M. bolley and Fusarium sp. REF210 and by Alternaria sp. REF104 and Embellisia REF147 were present on both dendrograms (Fig. 4). The topology of both dendrograms (Fig. 4) differed from the grouping on the phylogenetic tree of the 15 DSE species (Fig. 1). DSE species of the same order and DSEs of same host plant types did not clustered together on the UPGMA dendrograms (Fig. 4). The first two axes of the PCA ordination of standardized and categorized OD values of the 15 DSE species represented more than 45% (33.1 and 12.8) of the total variation (Fig. 5). The PCA using BioLog FF data showed joined ordination of DSE species of the five orders and the ordination did not correspond with the host plant types (Fig. 5). PCAs based on BioLog FF results converted to presence-absence data did not show any grouping related to considered features of the species (data not shown).

Dendrogram of the 15 DSE species based on (a) values (0–5) of api-ZYM tests and (b) categorized values (0–5) of BioLog FF MicroPlate measurements at 492 nm. The dendrograms were constructed using the UPGMA average method based on the Nei-Li coefficient. DSEs of grasses (triangle), non-grass plants (circle) and fungi of both plant types (triangle and circle) are shown. The scale bars indicate a percentage of dissimilarity among isolates.
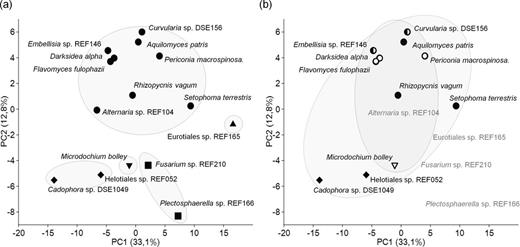
PCA of categorized OD values (0–5) derived from BioLog FF system of the 15 DSE species. PC1 accounts for 33.1% and PC2 for 12.8% of the variation. (a) DSEs of the same orders are surrounded by solid lines. Pleosporalean species are marked by dots, while species belonging to Helotiales, Hypocreales, Eurotiales and Xylariales by diamonds, squares, triangle and inverse triangle, respectively. (b) DSE species of grasses are marked by empty symbols and circled by solid line, DSEs of non-grass hosts are marked by black symbols and circled by dashed line, half black symbols indicates DSEs of mixed hosts, while symbols for species cannot clearly assigned to host types are not shown.
DISCUSSION
With the help of the api-ZYM tests of constitutive enzyme production and BioLog FF MicroPlate tests of carbon source utilization, we revealed interspecific differences of metabolic capabilities of 15 root endophytic fungal species originating from semiarid sandy regions. Hence, beside the taxonomic diversity of root-colonizing endophytes, functional diversity of these fungal communities exists. Although previous results indicated the functional heterogeneity of root endophytic fungi and enzymatic activities were also screened (e.g. Rice and Currah 2005; Mandyam, Loughin and Jumpponen 2010; Day and Currah 2011; Lacercat-Didier et al. 2016), we are not aware of any previous work, which screened more than hundred substrates to study functional diversity of root endophytes originating from the same environment. The 15 species studied here are DSE taxa present in different regions worldwide (see in Knapp, Pintye and Kovács 2012; Knapp et al. 2015). Some lineages which were frequent on our sampling sites were also commonly found as dominant members of root-associated fungal communities of other semiarid areas (Knapp, Pintye and Kovács 2012; Knapp et al. 2015). The majority of these fungi are frequent in US Prairie ecosystems (e.g. Khidir et al. 2010; Mandyam, Loughin and Jumpponen 2010; Bokati, Herrera and Poudel 2016), and some of them (e.g. Darksidea spp., Microdochium bolley, Periconia macrospinosa) were found in both the western (Knapp, Pintye and Kovács 2012) and the eastern part of the Eurasian steppe belt (Su, Guo and Hyde 2010; Knapp et al. 2015).
As the api-ZYM test was originally designed for bacteria, relatively few previous studies used this assay for constitutive enzyme production of filamentous fungi. Tekere et al. (2001) studied isolates of 10 white rot fungi common in evergreen forests of Zimbabwe and found absence of trypsin and lipase production, and only poor activity of α-fucosidase, α-mannosidase, chymotrypsin and cystine arylamidase could be detected. We also found zero or weak activity of these enzymes in case of the ascomycete root-colonizing DSE fungi. On the other hand, enzymes we found highly active (e.g. acid phosphatase and naphtol-AS-BI-phosphohydrolase) showed strong activity in white rot basidiomycetes, too (Tekere et al. 2001). Using hyphal fragments for inoculation of the test, we found high acid phosphatase and β-glucosidase activities in DSE, similarly to Jain, Lacey and Stevens (1991) who, for inoculation of api-ZYM assays, used filtrated extracellular enzymes and spore suspension of 11 conidial ascomycete species of Aspergillus, Eurotium and Penicillium. BioLog FF MicroPlates have been mainly used for metabolic characterization of yeasts or conidial fungi and for investigation of substrate utilization, growth, secondary metabolite and antimicrobial production of these fungi (e.g. Stefanowicz 2006; Singh 2009; Druzhinina et al. 2010; Lakhesar, Backhouse and Kristiansen 2010; Di Lonardo et al. 2013). Some studies using BioLog FF MicroPlates for profiling aimed to apply these data for supporting information for separation of clades (e.g. Rice and Currah 2005) or examine the metabolic diversity of fungal lineages (e.g. Kubicek et al. 2003). In these studies, preparation of inoculum for the assay, timing of measurements or data analyses and its interpretation were highly variant corresponding with the different goals of the works and the different taxa investigated. Limitations of the api-ZYM and BioLog tests (Preston-Mafham, Boddy and Randerson 2002; Stefanowicz 2006) are known and the inoculum preparation of filamentous fungi might harbor sources of further problems. In our study, with a modified inoculum preparation method BioLog FF MicroPlates could successfully be used for the study of non-sporulating filamentous fungi. Using this simple method for preparation of mycelial suspension, these assays can be used for metabolic characterization of any non-sporulating species and also for sporulating filamentous fungi making the comparison of such taxa much more reliable.
To date, enzymatic capability of only few DSE fungi of different ecosystems was tested using limited number of substrates. Caldwell, Jumpponen and Trappe (2000) investigated widespread helotialean DSE fungi of alpine and boreal regions: type isolates of Cadophora finlandica (UAMH 8151) and Phialocephala fortinii (FAP7) and six isolates of the Phialocephala fortinii s.l.—Acephala applanata species complex (PAC). All isolates were found to utilize cellulose, laminarin, starch, xylan and fatty acid ester as carbon source as well as proteins and ribonucleic acids; no differences in their substrate utilization capabilities was described. Caldwell, Jumpponen and Trappe (2000) reported the ability of these DSE fungi to access the major organic forms of carbon, nitrogen and phosphorus commonly found in plant debris and soil organic matter. In their study, Mandyam, Loughin and Jumpponen (2010) tested amylase, cellulase, laccase, tyrosinase and gelatinase enzymes, hydrolyzing complex carbon molecules, possessed by isolates of two grass-associated DSE species dominant in Konza prairie (Kansas, USA). They investigated eight Periconia macrospinosa and two Microdochium sp. isolates. In addition, utilization of organic and inorganic N sources by P. macrospinosa isolates was also screened: they were able to use both forms. These two species were also involved in our study; however, there were only two overlapping sources (alanine and glycine); although the methods used were different, both Periconia and Microdochium were able to utilize these two sources in both studies. However, Mandyam, Loughin and Jumpponen (2010) found most of the P. macrospinosa and Microdochium sp. isolates to produce all of the five extracellular enzymes they tested. Using higher number of substrates of the two enzyme assays, we found unambiguous differences of metabolic activity of the two taxa. Recently, Lacercat-Didier et al. (2016) studied two DSE fungi isolated from poplar, which seem to be conspecific with Helotiales sp. REF052 studied here. Carbon source utilization of the isolates was studied using growth assays on minimal medium complemented with different compounds. The isolates were able to grow on fructose, glucose, maltose, mannitol, sucrose, xylose, and cellobiose (Lacercat-Didier et al. 2016). Using the BioLog FF test, we found similar results: Helotiales sp. REF052 was able to use all of those seven carbon sources. From some point of view, these DSE fungi inhabiting nutrient poor soils might have similar enzyme capacities as e.g. ericoid mycorrhizal fungi, which have broad range of plant cell-wall-degrading enzymes and extracellular enzyme activities that may potentially allow them to exploit nutrient sources from dead plant tissue (Cairney and Burke 1998) and improve host performance with access of organic sources of nitrogen (Smith and Read 2008).
Mandyam, Loughin and Jumpponen (2010) presented differences in both the number of substrates utilized and the degree of utilization by different P. macrospinosa and Microdochium sp. isolates indicating intraspecific metabolic heterogeneity. Such intraspecific functional variability was found in different DSE isolates, too (Tellenbach, Grünig and Sieber 2011; Mayerhofer, Kernaghan and Harper 2012). BioLog FF MicroPlates assay has been used to test intraspecific heterogeneity of other fungi as e.g. in the case of Trichoderma pararesei nom. prov. and Hypocrea jecorina (Druzhinina et al. 2010). Rice and Currah (2005) also noticed variances of Biolog FF results of conspecific strains (e.g. Oidiodendron chlamydosporicum, Myxotrichum arcticum) and even highly differing results of replicates of one isolate (e.g. O. griseum UAMH1403, O. tenuissimum UAMH1523). With few frequent pleosporalean species we have started a systematic survey on intraspecific diversity (Knapp, Berek-Nagy, Imrefi, Herczeg, Kovács: unpublished data), our present work aimed to focus on broad, interspecific functional diversity of DSE community of semiarid sandy grasslands.
The high amount of metabolic information derived from the two tests revealed unambiguous diversity in metabolic activity of the DSE fungal species studied. Nevertheless, the two tests showed different overall view: the clustering of the species based on the results of api-ZYM and BioLog tests differed (Fig. 4) and did not correspond with the phylogeny of DSE species either (Fig. 1). However, PCA ordination of BioLog data revealed grouping of DSE species of the same orders, including Pleosporales represented by nine taxa in our study (Fig. 5). This order seems to be the most common in root-associated fungal endophytes of grasses on semiarid areas (Porras-Alfaro et al. 2008; Herrera et al. 2010; Khidir et al. 2010; Knapp, Pintye and Kovács 2012; Knapp et al. 2015). The pleosporalean root endophytes—despite their interspecific variances—seem to share similarities in their enzymatic processes, which might correspond to their general partnership with gramineous hosts and frequency in semiarid grassland ecosystems.
DSE fungi are generally considered as generalist root colonizers with broad spectra of host species (Jumpponen and Trappe 1998); however, some DSE lineages seem to be associated with different plant groups. For example Microdochium sp., P. macrospinosa, Flavomyces fulophazii and Darksidea species are found in roots of grasses (e.g. Mandyam, Loughin and Jumpponen 2010; Knapp, Pintye and Kovács 2012; Mandyam, Fox and Jumpponen 2012; Knapp et al. 2015), while several helotialean DSE fungi such as species of the PAC group (Grünig et al. 2008), Helotitales sp. (DSE-2), Cadophora sp. (DSE-1) (Knapp, Pintye and Kovács 2012) and other species are associated with non-gramineous hosts (Sieber and Grünig 2013). The results of the analyses did not show segregation of non-grass and grass-associated DSE taxa, indicating functional similarities of DSE fungal communities of the different host groups. All of the carbon sources of BioLog FF MicroPlate (Fig. 2) were utilized when the groups of four grass-associated DSE taxa most common and frequent in Hungarian semiarid sandy grasslands (Darksidea alpha, F. fulophazii, P. macrospinosa and M. bolley) were considered. On the other hand, the group of DSE species associated with woody plants and dicots but never with grasses (Cadophora sp. DSE1049, Helotiales sp. REF052, Aquilomyces patris, Rhizopycnis vagum and Setophoma terrestris) also utilized the full spectrum of carbon source (Fig. 2). This finding on complementary enzyme spectra of root endophyte community of different plant host types fits into the ‘plant holobiont’ concept introduced recently (Vandenkoornhuyse et al. 2015). The functionally diverse DSE fungal community can play crucial role in nutrient-limited habitats and fulfill one key aspect of the required on functional complementarity (Vandenkoornhuyse et al. 2015). The broad metabolic heterogeneity of these root endophytes can be important in root turnover, carbon metabolism and nutrient mobilization for the plant hosts especially in nutrient poor environments like the semiarid sandy grasslands. The broad repertoire of substrates utilized by root endophytes illustrates the functional importance of the diversity of this fungal group in plant performance and ecosystem functioning.
We thank an anonymous reviewer for comments/suggestions helped to clarify and improve the manuscript.
FUNDING
This work was supported by the ().
Conflict of interest. None declared.
REFERENCES