-
PDF
- Split View
-
Views
-
Cite
Cite
Susanne Schreiter, Guo-Chun Ding, Rita Grosch, Siegfried Kropf, Kai Antweiler, Kornelia Smalla, Soil type-dependent effects of a potential biocontrol inoculant on indigenous bacterial communities in the rhizosphere of field-grown lettuce, FEMS Microbiology Ecology, Volume 90, Issue 3, December 2014, Pages 718–730, https://doi.org/10.1111/1574-6941.12430
- Share Icon Share
Abstract
Bacterial biocontrol strains used as an alternative to chemical fungicides may influence bacterial communities in the rhizosphere and effects might differ depending on the soil type. Here we present baseline data on the effects of Pseudomonas jessenii RU47 on the bacterial community composition in the rhizosphere of lettuce grown in diluvial sand, alluvial loam and loess loam at the same field site. 16S rRNA gene fragments amplified from total community DNA were analyzed by denaturing gradient gel electrophoresis (DGGE) and pyrosequencing. DGGE fingerprints revealed that in three consecutive years (2010–2012) RU47 had a slight but statistically significant effect on the bacterial community composition in one (2010), two (2011) or all the three soils (2012). However, these effects were much less pronounced compared with the influence of soil types. Additional pyrosequence analysis of samples from 2011 showed that significant changes in bacterial community compositions in response to RU47 inoculation occurred only in alluvial loam. Different taxonomic groups responded to the RU47 application depending on the soil type. Most remarkable was the increased relative abundance of OTUs belonging to the genera Bacillus and Paenibacillus in alluvial loam. Pyrosequencing allowed side-effects of the application of bacterial inoculants into the rhizosphere to be identified.
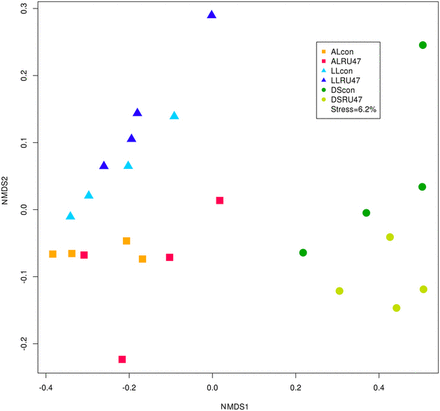
The introduction of the potential biocontrol inoculant Pseudomonas jesseniiRU47 into three soil types at the same field site had a negligible effect on the bacterial community in the rhizosphere of field-grown lettuce.
Introduction
The soilborne pathogen Rhizoctonia solani AG1-IB can be responsible for losses of up to 70% in field production of lettuce (Davis et al., 1997). The pathogen is difficult to control because of the long persistence of its sclerotia in soil (Ogoshi, 1996). Efficient control strategies against R. solani such as the soil fumigant methyl bromide were phased out because of their stratospheric ozone depletion potential and their ability to contaminate groundwater (Guns, 1989). Additionally, the pathogen carries a high acute toxicity risk for humans due to its carcinogenicity and neurotoxicity (Barry et al., 2012; Bulathsinghala & Shaw, 2014). The use of bacterial strains represents an environmentally friendly control strategy for chemical substances (Martin, 2003). In combination with other management activities such as crop rotation, biofumigation and removal of infected plant tissue, use of biocontrol inoculants could be an important component of integrated pest management (Barriere et al., 2014).
In the last decade a number of potential strains for biocontrol of R. solani AG1-IB have been isolated and characterized under greenhouse and field conditions (Adesina et al., 2009; Chowdhury et al., 2013). Furthermore, the ability of inoculants to colonize the rhizosphere, termed rhizocompetence, has been the focus of several studies (Götz et al., 2006; Adesina et al., 2009; Lugtenberg & Kamilova, 2009; Chowdhury et al., 2013; Xue et al., 2013). In view of legislation on biocontrol strains, information on potential effects on the soil microbiota should be considered, as soil microbes are increasingly recognized as important drivers for soil functions related to plant health and growth (Berendsen et al., 2012; Pieterse et al., 2014). With the advent of 16S rRNA gene-based molecular fingerprinting, detailed monitoring of rhizosphere microbial community changes in response to inoculation became feasible (Adesina et al., 2009; Grosch et al., 2012). To assess the extent of shifts caused in response to inoculants in comparison with other factors shaping soil and rhizosphere bacterial diversity such as the soil type, plant species, cultivar or plant growth stage, an appropriate experimental design is needed (Berg & Smalla, 2009). Furthermore, effects of inoculants on the microbial community in the rhizosphere might differ depending on the soil type. However, these effects are difficult to assess under field conditions, as many parameters such as cropping history, agricultural management practice and weather conditions influence the soil bacterial community composition (Costa et al., 2006; Berg et al., 2014). For the first time, a unique experimental plot system with three soil types under identical agricultural management practice and weather conditions for more than 10 years (Rühlmann & Ruppel, 2005) has created the opportunity to study the effect of soil type on the bacterial community composition in the bulk soil and in the rhizosphere under field conditions.
Different analyses of 16S rRNA have shown that soil types not only strongly shape the bacterial community composition in the rhizosphere, but also influence the extent of the so-called rhizosphere effect. In response to the growing lettuce plant, several genera of bacteria significantly increased in the rhizosphere in comparison with the corresponding bulk soil (Schreiter et al., 2014a). Remarkably, the effects of the soil types on the rhizocompetence and biocontrol activity of two potential biocontrol inoculants of R. solani AG1-IB, namely Pseudomonas jessenii RU47 and Serratia plymuthica 3Re4-18, were negligible (Schreiter et al., 2014b). The root exudate composition of lettuce, which was recently shown by Neumann et al. (2014) to differ only quantitatively between the soil types, seemed to support the successful establishment of the inoculant strains. DGGE analysis of 16S rRNA gene fragments amplified from total community DNA (TC-DNA) of lettuce rhizosphere samples taken 2 weeks after planting (2WAP) in 2011 indicated a weak effect of the inoculant strains on the bacterial community composition. The effect of inoculants on the bacterial community composition was clearly stronger for 3Re4-18 than for RU47 (Schreiter et al., 2014b). Based on the less pronounced effect of RU47 and the reliable soil type-independent rhizocompetence and biocontrol activity, it was decided to focus the present study specifically on the effects of RU47.
In the present study, the effect of the inoculant RU47 on the rhizosphere bacterial community of field-grown lettuce was monitored for three consecutive years. We hypothesized that the effects of the inoculant on the bacterial community composition in the rhizosphere of lettuce would be influenced by the soil type. Lettuce rhizosphere pellets were obtained after destructive sampling and recovery from a subset of the complete root system of three plants. DGGE analysis of 16S rRNA gene fragments was performed for all samples and subjected to statistical analysis. Pyrosequence analysis was done for the rhizosphere samples collected in 2011, the year when the best biocontrol effects by RU47 were observed.
Materials and methods
Design of field experiments
Lettuce (Lactuca sativa L.) was selected as a model plant to evaluate the effect of repeated applications of the inoculant P. jessenii RU47 on the bacterial community compositions in the rhizosphere in three soil types: diluvial sand (DS), alluvial loam (AL) and loess loam (LL). The three soils were stored at the same field site in a unique experimental plot system, each arranged in separate blocks at the Leibniz Institute of Vegetable and Ornamental Crops (IGZ, Großbeeren, Germany, 52°33′N, 13°22′E) in three consecutive years. Each block consists of 24 plots sized 2 × 2 m with a depth of 75 cm (Rühlmann & Ruppel, 2005). All three soil types were characterized by the same crop history. Following crops were cultivated on each block in the experimental plot system in the seasons before the start of the experiment: pumpkin, nasturtium, pumpkin, amaranth, wheat, wheat, pumpkin, nasturtium, wheat, wheat, and lettuce. Four plots with lettuce with and without RU47 inoculation were established in each soil type from 2010 to 2012.
Lettuce seeds (cv. Tizian; Syngenta, Bad Salzuflen, Germany) were sown in seedling trays filled with the respective soil type and incubated at 12 °C for 48 h and transferred to the greenhouse to grow at about 20/15 °C (day/night). All seedling trays were watered daily and fertilized weekly (0.2% Wuxal TOP N; Wilhelm Haug GmbH & Co. KG, Düsseldorf, Germany) to maintain the substrate moisture. Lettuce seedlings were planted at the 3–4 leaf stage (4 weeks after sowing) in six rows per plot (36 plants per plot) with a within-row and intra-row distance of 30 cm each. Fertilizer (Kalkamon, 27% N; TDGmbH Lommatzsch, Germany) was added to each plot, based on a chemical analysis of the soil type before planting. The same nitrogen amount of 157 kg ha−1 was adjusted to each soil type. Lettuce was overhead-irrigated based on the computer program berest (Gutezeit et al., 1993) as described in detail by Schreiter et al. (2014a). Each treatment included four replicates or plots.
Bacterization of lettuce
The rifampicin-resistant inoculant P. jessenii RU47 was retrieved from the Julius Kühn-Institut strain collection by restreaking the stock culture stored in Luria–Bertani broth (ROTH, Karlsruhe, Germany) with 20% glycerol at −80 °C on King agar B plates (Merck KGaA, Darmstadt, Germany) supplemented with rifampicin (75 μg mL−1). For lettuce seed treatment a single colony of RU47 was resuspended and spread on King agar B plates (Merck KGaA) supplemented with rifampicin (75 μg mL−1). Cells were scraped off from the bacterial lawn on the King B agar and were suspended in 15 mL sterile 0.3% NaCl solution with a density corresponding to 108 CFU mL−1 adjusted in a spectrometer. A total of c.100 lettuce seeds were coated with 250 μL of the bacterial suspension or 0.3% NaCl (control seeds).
For young lettuce plant inoculation, RU47 was grown in nutrient broth (NB II; SIFIN GmbH, Berlin, Germany) supplemented with rifampicin (75 μg mL−1) on a rotary shaker (90 r.p.m.) for 16 h at 29 °C. The cells were harvested by centrifugation at 13 000 g for 5 min and resuspended in sterile 0.3% NaCl solution and adjusted to a density corresponding to 107 or 108 CFU mL−1. Each lettuce seedling was treated with 20-mL bacterial solutions of RU47 (107 CFU mL−1) 1 week before transplanting into the field and with 30 mL (108 CFU mL−1) at the 4-leaf stage 2 days after planting. The seedlings of the control were drenched with 0.3% NaCl, respectively.
Sampling and DNA extraction
Lettuce rhizosphere samples were collected 3 weeks after planting (3WAP) in 2010 and 2 weeks after planting (2WAP) in 2011 and 2012. The complete root system of three lettuce plants with adhering soil was combined per replicate (four replicates per treatment and soil type). Loosely adhering soil was removed from the root by vigorous shaking but in the following years an additional root wash was applied by dipping the lettuce roots of each replicate briefly into 300 mL sterile tap water, followed by a Stomacher blending step repeated three times. To obtain the microbial rhizosphere/rhizoplane pellets, roots were treated as described by Schreiter et al. (2014b). TC-DNA was extracted from the pellets obtained with the FastDNA® SPIN Kit for Soil (MP Biomedicals, Heidelberg, Germany) according to the manufacturer's protocol after a harsh lysis step with the FastPrep®-24 Instrument (MP Biomedicals). The TC-DNA was purified with GENECLEAN SPIN® Kit (MP Biomedicals) and afterwards diluted 1 : 10 with 10 mM Tris HCl.
Analysis of 16S rRNA gene fragments by DGGE
PCR reactions with TC-DNA of rhizosphere samples were performed for amplification of 16S rRNA gene fragments using the bacterial primers F984-GC and R1378 as described by Heuer et al. (1997) but using GoTaq® Flexi (Promega, Mannheim, Germany) in 2012, instead of the Taq DNA polymerase (Stoffel fragment; ABI, Darmstadt, Germany) used in 2010 and 2011. To analyze the Actinobacteria, Alpha- and Betaproteobacteria in the 2010 and 2012 samples, a nested primer approach was used. The PCR product of the first PCR was used as a template for the bacterial primers F984 and R1378. All primers from this study are summarized in Supporting Information, Table S1. The PCR products were analyzed by DGGE as described by Weinert et al. (2009), and silver staining was performed according to Heuer et al. (2001).
Bacterial DGGE fingerprints were evaluated with gelcompar II version 6.5 (Applied Maths, Sint-Martens-Latem, Belgium). The normalization and background subtraction was performed on the basis of each DGGE gel image (Schreiter et al., 2014a). The Pearson correlation coefficient as a curve-based method was chosen to obtain the similarity matrices. These were used for construction of a dendrogram by an unweighted pair group method with arithmetic mean (UPGMA) as well as for statistical analysis by the permutation test, where the d-value was calculated as average overall correlation coefficients within the groups minus the average overall correlation coefficients between samples from different groups (Kropf et al., 2004) and displayed in percentages.
Analysis of 16S rRNA gene fragments by pyrosequencing
TC-DNA from rhizosphere samples (RU47 treatments and controls) obtained 2WAP from the field experiment 2011 were sent to the Biotechnology Innovation Center (BIOCANT, Cantanhede, Portugal) for barcoded pyrosequencing. PCR reactions were performed in 40-μL volumes with Advantage Taq (Clontech) using 0.2 M of both primers 338F and 802R (Table S1), 0.2 mM dNTPs, 1× polymerase mix and 6% DMSO. The PCR conditions were 94 °C for 4 min, followed by 25 cycles of 94 °C for 30 s, 44 °C for 45 s and 68 °C for 60 s and a final elongation step at 68 °C for 10 min. The amplicons were quantified by fluorimetry with PicoGreen (Invitrogen, Carlsbad, CA), pooled at equimolar concentrations and sequenced in the A direction with GS 454 FLX Titanium chemistry, according to the manufacturer's instructions (Roche, 454 Life Sciences, Branford, CT). The raw pyrosequence reads (fasta files) were processed using an automatic pipeline implemented at BIOCANT. In a first step, sequencing reads were assigned to the appropriate samples based on the respective barcode. Then, reads were quality filtered to minimize the effects of random sequencing errors by elimination of sequence reads with < 100 bp and sequences that contained more than two undetermined nucleotides. Sequences were additionally cut for the reverse primer if present.
The prefiltered pyrosequence data provided by BIOCANT were analyzed according to Ding et al. (2012a). Briefly, only those sequences matching the barcode and forward primer were selected for blastn analysis against a SILVA 16S rRNA gene database to truncate the unpaired regions for each sequence, only sequences with a length of more than 200 bp were included. Operational taxonomic unit (OTU) was generated with the following steps: sequences were assigned to OTUs (defined at > 97% sequences identity) with the program mothur 1.21 software (Schloss et al., 2009). Naïve Bayesian Classifier (Wang et al., 2007) was used to classify the sequences. The OTU assignment and the classification of each sequence were loaded into a MySQL-database for producing the taxonomic OTU report.
Based on the OTU report, a modified principal component test, termed PCUniRot, was performed (Ding et al., 2012b). This test supports multifactorial comparisons with high-dimensional data including the investigation of interactions of the factors after an upstream log transformation of the relative abundance in ‰ of the OTUs after adding ‘1’. It is exact under the assumption of multivariate normal data. In a further development of the basic principal component test (Läuter et al., 1996, 1998), the modification was directed towards a more effective use of the principal components in the case of small samples and a very large number of variables were included.
For the detection of OTUs determining the overall shift corresponding to the application of RU47, a multiple permutation test as suggested by Westfall & Young (1989) with the described pre-transformation of the data was used. With this test an overall family-wise error rate based on all detected OTUs of 5% was not possible. For this reason, OTUs occurring in a minimum of 10 samples were selected for further analysis. With this pre-filtering, the most abundant 901 OTUs were used for significance test.
Furthermore, the ‘r’ (version 2.14) add-on package ‘vegan’ was used to analyze the community composition and calculate the rarefaction curves as well as Pielous's evenness indices. A Tukey's honestly significant difference test, abbreviated Tukey test, under a generalized linear model via a logistic function for binomial data with the package multcomp (Hothorn et al., 2008) was performed to identify the discriminative taxa between inoculation with RU47 and control in the different soil types without a logarithmic pre-transformation of the data. Bonferroni adjustment was used for the P-value < 0.05. To visualize the microbial community composition between treatments, a non-metric multidimensional scaling (NMDS) was used based on Bray–Curtis similarity. Pyrosequence data were deposited at the NCBI Sequence Read Archive under the study accession number SRP029944.
Results
DGGE revealed minor effects of RU47 on the bacterial community in the rhizosphere of lettuce
To assess the effects of the inoculant RU47 on the bacterial community composition in the rhizosphere of lettuce grown in three soil types in three consecutive years, comparative DGGE analysis was done. Fingerprints generated from 16S rRNA gene fragments amplified from TC-DNA of the four replicate samples per treatment showed little within- or between-treatment variability in each of the three soil types. No separate clusters between inoculated and control treatments were found (Figs S1–S17), but a separate clustering depending on the soil types was observed for all 3 years. The calculated d-values (Table 1) indicated low differences between the bacterial community compositions in the rhizosphere of RU47-inoculated lettuce plants and the corresponding control plants. However, for DS soils these differences were significant in all 3 years. In 2011 the differences were also significant for lettuce grown in LL soil, whereas in 2012 significant differences between inoculated and control treatments were observed for all three soil types. The differences between the bacterial communities in the rhizosphere of inoculated and control treatments increased in all three soils, with the highest d-values (except for LL) observed in 2012.
Differences (d-values in %) between taxonomic groups obtained by denaturing gradient gel electrophoresis analysis of rhizosphere samples of lettuce grown in three soil types at the experimental plot system in Großbeeren (Germany) with and without application of the inoculant RU47
AL, alluvial loam; DS, diluvial sand; LL, loess loam.
Significant differences acquired by the permutation test as suggested by Kropf et al. (2004).
Differences (d-values in %) between taxonomic groups obtained by denaturing gradient gel electrophoresis analysis of rhizosphere samples of lettuce grown in three soil types at the experimental plot system in Großbeeren (Germany) with and without application of the inoculant RU47
AL, alluvial loam; DS, diluvial sand; LL, loess loam.
Significant differences acquired by the permutation test as suggested by Kropf et al. (2004).
The differences in bacterial community compositions caused by soil types were much higher than the differences caused by the application of RU47 (Table 1). In 2010, the differences between the soil types were rather similar to d-values, ranging from 18.8% to 19.9%; the differences of DS vs. LL and AL vs. LL soil increased strongly over time. The highest differences were recorded between DS and LL soil, with 46.6% in 2012 (Table 1). In comparison, the bacterial community compositions in the rhizosphere of lettuce grown in DS and AL soil became more similar with time, as the lowest d-value was observed in 2012.
To reveal soil type-dependent effects of the RU47 treatment on less abundant populations in the rhizosphere of lettuce, DGGE fingerprints were generated for Actinobacteria, Alpha- and Betaproteobacteria from samples taken in 2010 and 2012 (Table 1). UPGMA analysis revealed soil type-dependent clusters for the taxonomic groups analyzed in both years (Figs S8, S10, S16 and S18). Interestingly, Betaproteobacteria rhizosphere fingerprints of lettuce grown in DS and AL soils in 2012 showed higher similarities with each other compared to the 2010 LL rhizosphere fingerprints (Fig. S14). In contrast to this, the Actinobacteria fingerprints of AL and LL rhizospheres were more similar to each other than those of DS rhizospheres in 2010 and 2012 (Figs S16 and S18). The effect of RU47 on the Betaproteobacteria community composition was only significant for lettuce grown in AL in 2010, and in all soils the d-values decreased in 2012 compared with 2010 (Table 1), whereas the RU47 inoculation significantly influenced the Actinobacteria community composition in the rhizosphere of lettuce grown in DS soil in both years. In the two loamy soils, rather strong effects of RU47 on the Actinobacteria community composition were only detected in 2012. Overall, increased d-values indicating higher differences between the Actinobacteria communities in the rhizosphere of inoculated and control treatments were recorded for all three soils in 2012.
The analysis of the Actinobacteria, Alpha- and Betaproteobacteria confirmed that the effect of RU47 was minor compared with the effects of different soil types. However, it also became clear that both the RU47 and the soil type effects differed for the three taxonomic groups analyzed.
Pyrosequence data
To determine the taxonomic composition of bacterial communities in the rhizosphere of lettuce and to identify major responders to RU47 inoculation, pyrosequencing of 16S rRNA gene fragments amplified from a total of 24 rhizosphere samples of control and RU47 treatments from the three soil types in 2011 was carried out (four replicates per soil type and treatment). Altogether, 103 178 sequences with a sequence length of more than 200 bp were used for the analysis. The sequences were classified into 18 phyla, 52 classes, 83 orders, 175 families and 394 genera, and clustered to 7076 OTUs based on 97% sequence identity. In both treatments and all three soil types, the dominant phyla (with more than 1% relative abundance) were the Proteobacteria, followed by Actinobacteria, Bacteroidetes, Firmicutes and Acidobacteria (Table 2). The classes within the Proteobacteria with the highest relative abundance were the Betaproteobacteria, followed by Alphaproteobacteria; the Gamma- and Deltaproteobacteria were less abundant (Table 2). Based on the Tukey test, statistically significant differences between RU47-treated and control samples at the phylum level were observed only for the Firmicutes in the AL rhizospere. A 1.5-fold increase in the relative abundance of the phylum Firmicutes was observed in response to the RU47 inoculation. None of the other phyla and classes analyzed changed significantly in their relative abundance in response to the RU47 inoculation.
Relative abundance (in %) of dominant phyla and classes from the rhizosphere of lettuce grown in three soil types in 2011 in the control plot and the plot inoculated with Pseudomonas jessenii RU47 obtained by pyrosequencing
AL, alluvial loam; DS, diluvial sand; LL, loess loam.
Significantly enriched taxa in the rhizosphere between control and RU47-treated samples in each soil type, identified by Tukey test under a generalized linear model via logistic function for binomial data.
Relative abundance (in %) of dominant phyla and classes from the rhizosphere of lettuce grown in three soil types in 2011 in the control plot and the plot inoculated with Pseudomonas jessenii RU47 obtained by pyrosequencing
AL, alluvial loam; DS, diluvial sand; LL, loess loam.
Significantly enriched taxa in the rhizosphere between control and RU47-treated samples in each soil type, identified by Tukey test under a generalized linear model via logistic function for binomial data.
Rarefaction analysis was performed to compare bacterial richness between soil types and treatments. The result revealed that bacterial richness varied between soil type, with the lowest richness for DS soil and the highest for LL soil. However, the influence of RU47 on richness was not pronounced for the three soil types (Fig. 1a). Interestingly, the evenness indicated by Pielous's indices was lower in the control than in the RU47-treated samples for the two loamy soils (Fig. 1b). But in the DS soil the evenness was comparable between control samples and RU47-treated samples.
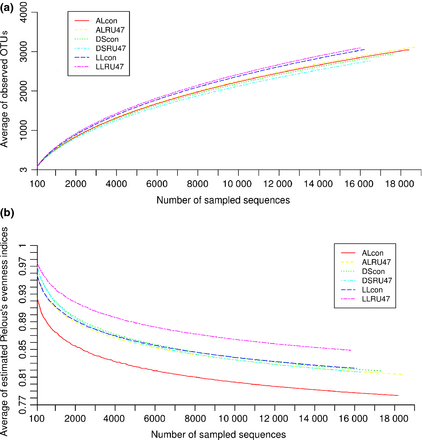
Rarefaction analysis (a) based on OTUs (> 97% sequence identity) and Pielous's evenness indices (b) for lettuce rhizosphere samples treated with RU47 or untreated control (con) from three soils (DS, AL, LL) in 2011.
The comparison of the bacterial community compositions performed by NMDS using Bray–Curtis similarities confirmed that the RU47- and control treatments did not cluster separately, although soil type-dependent clusters were observed (Fig. 2). Using the PCUniRot for each soil type separately, a statistically significant effect of the RU47 inoculation on the bacterial community composition was observed in the AL rhizospere only. Two OTUs responsible for this effect could be identified as OTU 402 and OTU 5304. These OTUs shared 98% sequence identity with Pseudomonas brassicacearum (EU391388) and Pseudomonas reinekei (AM293556), respectively. Both of these OTUs also had a high sequence identity of 98–99% to the applied biocontrol strain RU47.
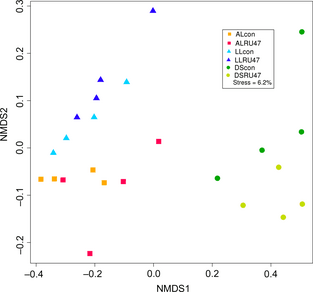
NMDS based on the Bray–Curtis similarity for lettuce rhizosphere samples treated with RU47 or untreated control (con) from three soils (DS, AL, LL) in 2011.
Several genera with significant changes in relative abundance in response to the RU47 inoculation were identified by the Tukey test. Most importantly, the genera with significantly changed relative abundance in the lettuce rhizosphere differed among the soil types. The most abundant responders to RU47 inoculation in AL rhizosphere were the genera Paenibacillus and Bacillus (Table 3). In addition, the less abundant genus Bdellovibrio showed a twofold increase in relative abundance in response to RU47 (Table 3). In DS rhizosphere, the genera Methylophilus, Fluviicola and Cytophaga significantly increased in relative abundance (two- and threefold; Table 3). In contrast, the genera Nocardioides and Marmoricola, belonging to the Actinobacteria, decreased in relative abundance in DS rhizosphere of RU47 treatments compared with the control (Table 3). In the LL soil only the genus Bdellovibrio displayed a significantly changed relative abundance in response to the RU47 treatment. Similar to the AL rhizospere, the relative abundance of Bdellovibrio doubled in LL rhizosphere in response to RU47 inoculation (Table 3).
Relative abundance (in %) of taxonomic groups in the rhizosphere of lettuce, grown in the three soil types responding to the treatment with the inoculants RU47
AL, alluvial loam; DS, diluvial sand; LL, loess loam.
Significantly enriched taxa in the rhizosphere between control and RU47-treated samples in each soil type, identified by Tukey test under a generalized linear model via logistic function for binomial data.
Relative abundance (in %) of taxonomic groups in the rhizosphere of lettuce, grown in the three soil types responding to the treatment with the inoculants RU47
AL, alluvial loam; DS, diluvial sand; LL, loess loam.
Significantly enriched taxa in the rhizosphere between control and RU47-treated samples in each soil type, identified by Tukey test under a generalized linear model via logistic function for binomial data.
The 50 most abundant OTUs were selected and their relative abundance was visualized in a heatmap (Fig. 3). Among the 50 dominant OTUs, 42 were affiliated to the Proteobacteria, with the majority being Betaproteobacteria (22), followed by Alpha- (10) and Gammaproteobacteria (10). The remaining dominant OTUs belonged to the Firmicutes (4), Actinobacteria (3) and Bacteroidetes (1). With a few exceptions, most dominant OTUs displayed rather high sequence identities (97–100%) to 16S rRNA gene sequences of isolates. Significant changes in the relative abundance of dominant OTUs in response to the application of RU47 were only observed for six OTUs. Among them were three OTUs with high sequence identity to P. reinekei and P. brassicacearum which were detected in the lettuce rhizosphere in all three soils. The fourth OTU identified as Pseudomonas graminis did not show this treatment-dependent response. In contrast to the few OTUs responding to the RU47 inoculation, approximately two-thirds of the dominant OTUs showed a clear soil type-dependent relative abundance (Fig. 3).
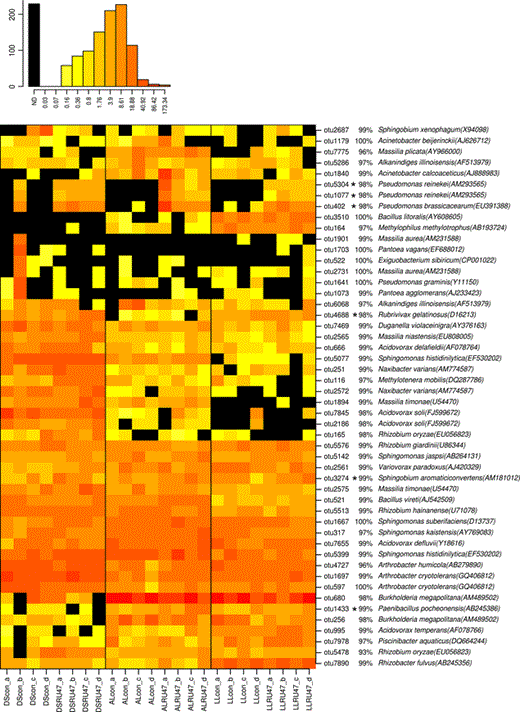
Relative abundance of the most dominant OTUs for lettuce rhizosphere samples detected 2 weeks after planting in 2011. The heatmap indicates differences by an asterisk in the relative abundance of OTUs between RU47 treatment and untreated control. The lettuce was grown in three soil types (DS, AL, LL). Each vertical column represents one sample and each horizontal row depicts one OTU. The color code grades from black (not detected), to yellow (low abundance), to orange (medium abundance) to red (high abundance). Numbers in brackets indicate the NCBI GenBank accession number of the sequence that was most similar to the consensus sequence of each OTU.
Discussion
Various factors such as soil type, plant species, cultivars, plant growth stage, cropping history and agricultural management systems were recently reported to shape the bacterial community composition in the rhizosphere of crops, as reviewed by Berg & Smalla (2009), Buee et al. (2009) and Berg et al. (2014). In the present study, we tested the hypothesis that the effects of potential biocontrol inoculants such as P. jessenii RU47 on the bacterial community composition depend on soil type, as the inoculant is not only exposed to different soil physico-chemical conditions but also interacts with different indigenous bacterial communities. Effects of the inoculant RU47 on the bacterial communities in the rhizosphere were assessed for lettuce grown under field conditions in three different soils in three consecutive years and sampled at the same plant growth stage. The experimental design and the methods used not only allowed the evaluation of shifts in the bacterial community composition in response to the inoculant application in each year, but also made it possible to reveal differences due to the repeated growth of lettuce with and without RU47 application and to identify responders to the inoculant. Bacterial DGGE fingerprints revealed that the differences in the bacterial community composition between RU47 and control treatments were minor in all three soil types compared with the differences observed between soil types (Table 1). This observation was made for all soil types in the three consecutive years. DGGE fingerprints indicated that the differences in the bacterial and actinobacterial communities between RU47 and control treatments increased in 2012 in all three soils, indicating that the repeated application of RU47 in successive lettuce cropping at the same field site likely increased the effect of the inoculants (Table 1). However, we cannot exclude that other factors such as the weather conditions might have also contributed to these increased differences. Interestingly, the d-values for Alpha- and Betaproteobacteria were lower in 2012 than in 2010, indicating a weaker effect of RU47 after repeated lettuce growth which might well be caused by the strong enrichment of Beta- and Alphaproteobacteria in the rhizosphere in consecutive lettuce cultivations (Schreiter et al., 2014a). The enrichment of Beta- and Alphaproteobacteria in the rhizosphere in previous lettuce crops may increase the relative abundance of these populations in bulk soil.
Pyrosequence analyses were done for the samples from 2011 when the best biocontrol effects by RU47 were observed and the data largely confirmed the findings obtained by DGGE. Both methods showed distinct soil type-dependent bacterial community composition in the rhizosphere of lettuce, whereas only minor differences between the bacterial community compositions of the RU47-inoculated and control treatments were detected. However, statistical analysis of pyrosequence data showed a significant effect of RU47 in the AL rhizosphere, whereas the d-value of the same sample set was not significantly different for DGGE fingerprints, despite the highest d-values being observed for the AL rhizosphere.
The soil type-dependent responders to RU47 inoculation identified through pyrosequence analysis further confirmed our hypothesis that the effects of RU47 were soil type-specific. Although the effects of RU47 on the bacterial community composition detected by DGGE fingerprints and pyrosequence data were much less pronounced compared with the effects caused by the soil types, changes in relative abundance of a few genera in response to RU47 application were observed by statistical analysis of pyrosequence data. The significant increase of the already abundant genera Bacillus and Paenibacillus in the AL rhizospere was particularly interesting, as isolates belonging to these genera often confer traits that are beneficial for plant growth and health (Beneduzi et al., 2008a, b). Strains belonging to these genera are already used as biocontrol agents, for example Bacillus subtilis CA32 and Bacillus amyloliquefaciens FZB42.
In comparison with the composition of dominant OTUs in the rhizosphere of lettuce grown in the same soils in 2010 (Schreiter et al., 2014a), a higher proportion of the 50 dominant OTUs from the present study (2011) belonged to the Betaproteobacteria (Acidovorax, Burkholderia, Massilia, Methylophilus, Duganella, Naxibacter, Piscinibacter, Rubrivivax, Variovorax) and Gammaproteobacteria (Acinetobacter, Pantoea, Alkanindiges, Pseudomonas). This finding might be due to the modification in the protocol for the extraction of the microbial pellet from the roots. The additional root wash step was introduced to avoid large clumps of soil adhering to the root, which was observed in particular for the soils with high clay content (AL, LL). Thus the rhizosphere community composition of samples from 2011 and 2012 mainly represent the fraction of soil adhering very tightly to the root and the rhizoplane. Our data indicate that, in particular, Betaproteobacteria were more closely attached to the roots of lettuce. In comparison, Alphaproteobacteria dominated in the rhizosphere of lettuce samples from 2010, which were analyzed without root wash, suggesting that they adhered less firmly to the roots (Schreiter et al., 2014a). Although the relative abundance of six dominant OTUs changed significantly in response to RU47, only three OTUs strongly increased under the RU47 treatment. They displayed 98% sequence identity to P. brassicacearum and P. reinekei. We cannot exclude that the inoculant RU47 contributed to the OTU 5304, OTU 1077 and OTU 402, which showed a sequence identity of > 98%, respectively, to the 16S rRNA gene sequence of RU47. Thus the heatmap data should not be overinterpreted, as a partial 16S rRNA gene sequence clearly does not allow a reliable taxonomic identification at the species level. Screening the abundance of functional genes involved in antibiotic production (prnD, PCA, DAPG) in 2010 did not indicate any differences between controls and RU47 treatments (data not shown).
The present study is unique, being the first to report on the assessment of the effects of a potential biocontrol inoculant on the bacterial community composition in the rhizosphere in three soil types at the same field site in three consecutive years. Despite a good and soil type-independent rhizocompetence of RU47 in the different experiments (Schreiter et al., 2014b) the effects on the bacterial community composition in the rhizosphere were minor compared with the soil type, and likely transient. Effects of the inoculant treatment might be comparable to the effects of genetically modified potato plants, which were within the range of natural cultivar variability as reported by Weinert et al. (2009). Previous studies with other inoculants (Scherwinski et al., 2008; Grosch et al., 2012; Chowdhury et al., 2013), all of them based on fingerprinting of 16S rRNA gene fragments [single-strand conformation polymorphism (SSCP), DGGE and terminal restriction fragment length polymorphism (T-RFLP)], also reported only minor effects of inoculants. In the study by Erlacher et al. (2014), the gammaproteobacterial microbiome in the rhizosphere and phyllosphere of lettuce grown under greenhouse conditions was analyzed by pyrosequencing. Those authors found that the highest impact on the indigenous gammaproteobacterial communities due to the R. solani AG1-IB attack was reduced in the treatments with the biocontrol strain B. amyloliquefaciens. A direct metagenome sequencing approach was used recently to assess the effects of the inoculant B. amyloliquefaciens FZB42 on the bacterial community in the rhizosphere of lettuce at three different plant growth stages (Kröber et al., 2014). FZB42 was found to have almost no effect on the bacterial community composition in the rhizosphere of lettuce, whereas the growth stage had a more pronounced impact. However, the absence of replicates also prevented a more rigorous testing for significant differences. The same lettuce cultivar was used by Kröber et al. (2014) and, with a few exceptions, the metagenome sequencing revealed a similar taxonomic affiliation of the abundant classes, orders and genera as found by amplicon sequencing of 16S rRNA gene fragments in the present study. The most striking difference was the high abundance of Mycobacteria revealed by direct metagenome sequencing, which might be due to a different bacterial community composition of the AL soil used in that study. In the present study, we demonstrated the potential of next generation sequencing technologies to reveal populations changing in relative abundance in response to RU47 inoculation. With increasing sequencing depth and decreasing costs of next generation sequencing, the sensitivity of the methods and feasibility to analyze large numbers of samples will provide an important tool to assess the effects of inoculants on the soil microbiota. Despite the limitations of the 16S rRNA gene-based analysis, by providing limited taxonomic and functional information, this is a big step forward in our ability to detect even more subtle changes in the bacterial community composition in response to inoculant application. It is important to note that changes of the bacterial community composition are not per se negative but could well be beneficial, e.g. through the increased relative abundance of plant beneficial bacteria or the decreased abundance of plant pathogens. Considering functional resilience in soils, we assume that the inoculation of RU47 did not affect soil functions, or did so only transiently. Changes in the bacterial community composition could well contribute to the improved plant health in inoculant treatments previously observed by Schreiter et al. (2014b).
Acknowledgements
The authors acknowledge that the project SM59/11-1/GR568121 was funded by the Deutsche Forschungsgemeinschaft (DFG). We would also like to thank Petra Zocher, Ute Zimmerling, Sabine Breitkopf and Angelika Fandrey for their skilled technical assistance and Ilse-Marie Jungkurth for her helpful comments on the manuscript.
References
Author notes
Editor: Wietse de Boer
Deutsche Forschungsgemeinschaft (DFG)
SM59/11-1/GR568121