-
PDF
- Split View
-
Views
-
Cite
Cite
Omneya A. Osman, Cristian Gudasz, Stefan Bertilsson, Diversity and abundance of aromatic catabolic genes in lake sediments in response to temperature change, FEMS Microbiology Ecology, Volume 88, Issue 3, June 2014, Pages 468–481, https://doi.org/10.1111/1574-6941.12312
- Share Icon Share
Abstract
The abundance and composition of genes involved in the catabolism of aromatic compounds provide important information on the biodegradation potential of organic pollutants and naturally occurring compounds in the environment. We studied catechol 2, 3 dioxygenase (C23O) and benzylsuccinate synthase (bssA) genes coding for key enzymes of aerobic and anaerobic degradation of aromatic compounds in experimental incubations with sediments from two contrasting lakes; humic lake Svarttjärn and eutrophic Vallentunasjön, respectively. Sediment cores from both lakes were incubated continuously for 5 months at constant temperatures ranging from 1.0 to 21.0 °C. The difference in C23O gene composition of the sediment analyzed at the end of the experiment was larger between lakes, than among temperature treatments within each lake. The abundance of C23O gene copies and measured respiration was positively correlated with temperature in Vallentunasjön, whereas putative C23O genes were present in lower concentrations in Svarttjärn sediments. Putative bssA genes were only detected in Svarttjärn. For both lakes, the two catabolic genes were most abundant in the surface sediment. The results emphasize the important role of temperature and nutrient availability in controlling the functional potential of sediment microorganisms and reveal differences between systems with contrasting trophic status. A better understanding of catabolic pathways and enzymes will enable more accurate forecasting of the functional properties of ecosystems under various scenarios of environmental change.
Introduction
The presence and abundance of functional genes involved in aerobic and anaerobic catabolic processes have recently been used as a method to evaluate aromatic biodegradation potential in petroleum-contaminated environments (Boll et al., 2002; Hendrickx et al., 2006). Catechol 2,3 dioxygenase (C23O) is an extradiol dioxygenase involved in metacleavage of aromatic rings under aerobic biodegradation processes (Harayama et al., 1992) and consists of five subfamilies (I.2.A–I.2.E) (Eltis & Bolin, 1996). The molecular mechanisms responsible for anaerobic degradation of toluene and other aromatic compounds have received a lot of attention over the last two decades. One example is benzylsuccinate synthase (bssA), which is a newly discovered enzyme participating in the initial step of toluene degradation. This enzyme has been isolated from different toluene degrading bacteria, including the denitrifying strain Thauera aromatica (Biegert et al., 1996; Beller & Spormann, 1997a), the sulfate-reducing strain PRTOL1 (Beller & Spormann, 1997b), the anoxygenic phototroph Blastochloris sulfoviridis (Zengler et al., 1999), the ferric iron-reducer Geobacter metallireducens (Kane et al., 2002) and methanogens (Beller & Edwards, 2000). Anaerobic toluene degradation starts by the addition of the methyl group of toluene to the double bond of fumarate to form benzylsuccinate. This is catalyzed by the action of benzylsuccinate synthase and then β-oxidation of benzylsuccinate to benzoyl-CoA will follow (Heider et al., 1999; Boll et al., 2002).
Several molecular methods directly targeting C23O and bssA genes have been reported to overcome the inherent limitations of using 16S rRNA sequencing methods to probe these phylogenetically diverse functional groups in severely polluted environments (Joshi & Walia, 1996; Mesarch et al., 2000; Widdel & Rabus, 2001; Junca & Pieper, 2003; Luz et al., 2004; Morasch et al., 2004). In natural environments, bacteria carrying C23O can use either natural aromatic compounds (lignin and humic substances) by means of the metacleavage pathway or alternative nonaromatic organic substrates without this gene being expressed (Kasuga et al., 2007). However, not much is known about the quantitative distribution and diversity of C23O genes in natural lake environments. Furthermore, there are no reports on the occurrence of bssA genes in nonpolluted aquatic environments, and it is not known how environmental drivers, such as temperature, would influence its distribution. This trait may in turn control the ability of such systems to quench sudden inputs of organic pollutants from, for example anthropogenic activities. The presence of aromatic catabolic genes is therefore likely to confer an ability of indigenous microbiota to cope with and exploit sudden pulses of aromatic pollutants and, as a consequence, enhance the potential clean-up of the environment from such contaminants (Beller et al., 2002; Kasuga et al., 2007; Winderl et al., 2007). Temperature is known to have a strong influence on biodegradation of aromatic compounds with degradation rates typically increasing with temperature (Atlas, 1981; Bossert & Bartha, 1984). However, this is believed to mainly be a result of temperature-controlled changes in enzyme activity according to the ‘Ql0’ effect (Atlas & Bartha, 1972; Gibbs et al., 1975), whereas alternative explanations, such as changes in microbial communities, are typically not considered.
Aquatic sediments host diverse microbial communities and can function as either sources or sinks for both nutrients and pollutants. Investigations of the subset of the sediment microbiota possessing the genetic machinery to degrade naturally occurring or anthropogenic aromatic compounds can help us better understand the resilience and resistance of aquatic ecosystems to disturbances and their ability to degrade such recalcitrant compounds (Tamaki et al., 2003). The organic matter accumulating in sediments of brown-colored lakes consists largely of humic substances. Even if such heteropolymeric organic acids are considered to be highly resistant to microbial degradation, they are known to participate as electron acceptors in the anaerobic oxidation of several organic substrates, such as acetate, lactate, and toluene (Lovley et al., 1996; Coates et al., 1998; Cervantes et al., 2001). Additionally, temperature dependence of the enzymatic activity lies at the core of the organic matter decomposition kinetics and little is known about the susceptibility of aquatic vs. terrestrial matter in this regard (Erhagen et al., 2013). The effects of temperature on sediment organic carbon mineralization in two Swedish lakes of contrasting trophic status (humic lake Svarttjärn and eutrophic Vallentunasjön) were previously studied by Gudasz et al. (2010). In both lakes, sediment mineralization rates responded strongly and positively to increasing temperature. The main purpose of this study was to test for temperature dependence of the sediment microbiota community possessing catabolic aromatic genes in two contrasting lake sediments. We analyzed the combined effects of sediment depth and experimentally controlled temperature regime on the abundance and composition of catechol 2, 3 dioxygenase and benzylsuccinate synthase genes, which code for key enzymes of aerobic and anaerobic degradation of aromatic compounds, respectively. We show that the occurrence of populations of the studied genes respond to temperature and vary over depth following a 5-month experimental incubation, but also observed major differences between the two studied lakes. We conclude by discussing implications for natural biodegradation potential of aromatic compounds in a future scenario of environmental change.
Material and method
Study sites
Sediment mineralization was measured in two lakes with contrasting loading of allochthonous and autochthonous organic carbon: Svarttjärn (59°53′N 15°15′E, organic matter predominantly humic substances of terrestrial origin) and Vallentunasjön (59°53′N 18°02′E, highly eutrophic with organic matter of predominantly algal origin). Dissolved organic carbon (DOC) was similar in the water from the two lakes (Svarttjärn, 27.9 mg L−1; Vallentunasjön, 26.2 mg L−1), but the DOC-specific absorbance at 250 nm (A250/DOC) differed substantially (Svarttjärn, 0.037 cm−1 mg L−1; Vallentunasjön, 0.007 cm−1 mg L−1), indicating dramatic differences in the composition of the dissolved organic matter (DOM) (Weishaar et al., 2003). High C : N ratio (22.04 ± 4.72) was found in Svarttjärn sediments, whereas Vallentunasjön had a low C : N ratio (9.07 ± 0.11). More information about lake characteristics can be found in Gudasz et al. (2010).
Experimental set up
Sediment cores sampled from the deepest point of each lake were incubated for measurement of sediment mineralization as previously described (Gudasz et al., 2010). Lake sediments were sampled during February 2010 under ice cover. Lake water temperature at the bottom was 4.8 °C. Sampled sediment was transferred undisturbed in transparent plexiglass incubation tubes, containing the upper 4- to 5-cm sediment layer. In the laboratory, the water column above the sediment was replaced with aerated bottom water. Sediment incubation tubes were kept open and a special device mixed the water column continuously throughout the duration of the experiment. Tubes were closed only for a short period of time, for sediment mineralization rate measurements. Sediment mineralization was measured as dissolved inorganic carbon (DIC) production in the dark as described by Gudasz et al. (2010). Sediment was incubated continuously for 5 months (150 days) at constant temperatures similar to seasonal strength in boreal lakes (e.g. ice cover and summer stratification), temperature has been measured in situ and in epilimnetic sediments with 21 °C in summer time. The incubation temperatures were set to 1, 3, 5, 8, 13, and 21 °C in six temperature-controlled water baths. At each temperature and for each lake, three replicate cores were incubated. The tubes were incubated in insulated water tanks with controlled temperature by Julabo coolers (F25-ED), and tubes were kept in the dark throughout the duration of the experiment. At the end of the experiment, the sediment was sampled at 1-cm intervals, where each layer was homogenized and subsampled. Samples were immediately stored at −80 °C until analysis. The dissolved oxygen concentration was measured with the PreSens optode spots placed at about 4 cm above the sediment surface. The water column was mixed continuously, without disturbing the sediment surface, for the duration of the experiment (5 months). Tubes were kept open to equilibrate with the atmosphere and were closed for shorter periods of time only to measure the DIC flux at the sediment surface. In a number of tubes and across temperatures, we have measured oxygen penetration depth with an Optode needle-type oxygen microsensor and a micromanipulator. The oxygen penetration depth varied between 2 cm at low temperature to about 3 mm at 21 °C. pH of the water column above the sediment core was 6.4 ± 0.2 in Svarttjärn and 8.3 ± 0.2 in Vallentunasjön at the beginning of the experimental incubation.
Nucleic acid extraction
Aliquots of sediment samples were kept frozen at −80 °C. For each sample, nucleic acids were extracted from 0.45 to 0.5 g of wet sediment using the Power soil DNA isolation Kit as recommended by the manufacturer (Mo Bio Laboratories Inc., CA). DNA concentration was measured using PicoGreen ds DNA Quantification kit as recommended by the manufacturer (Life Technologies Europe BV, Stockholm, Sweden).
PCR amplification of C23O and bssA genes
Previously published and validated primer sets for C23O and bssA genes were used to detect and quantify these genes in our environmental DNA samples (Supporting Information, Table S1). Several primer sets were initially tested to recover most catechol 2, 3 dioxygenase subfamilies; XYLE1-F/XYLE1-R and XYLE2-F/XYLE2-R (Hendrickx et al., 2006) and COM-F/COM-R (Táncsics et al., 2009). No putative C23O gene fragment was amplified when XYLE2 or COM primer sets were used. The XYLE1-F/XYLE1-R primer set was the only primer pair resulting in positive amplification with amplicons of the expected C23O gene fragment size in sediment samples and was thus used further in this study. Previously published primers bssA-F and bssA-R were used for amplifying the alpha subunit of benzylsuccinate synthase genes (Beller et al., 2002). These primers preferentially amplify bssA genes from denitrifiers, but have also been used successfully used to amplify the same gene from methanogenic consortia (Da Silva & Alvarez, 2004) with highly efficient gene recovery.
For C23O, PCR amplification was carried out in a total volume of 20 μL per reaction with a final concentration of 1X Taq reaction buffer, 2 mM MgCl2, 1 U Taq polymerase, 0.3 μM of each primer, and 200 μM of dNTP mixture. PCR cycling conditions were as follows: initial heating at 95 °C for 5 min; followed by 30 cycles with a 1 min denaturation at 95 °C, 1 min annealing according to Table S1, and a 2 min extension at 72 °C. This was followed by a final extension at 72 °C for 10 min. Pseudomonas fluorescens carrying subfamily I.2.A catechol 2,3 dioxygenase gene was used as positive control (Wikström et al., 1996) and PCRs without DNA template was used as negative controls.
For bssA genes, PCR amplification was carried out in a 20-μL reaction mixture including 10x Taq reaction buffer, 1.5 mM MgCl2, 1 U Taq polymerase, 0.25 μM of each primer, and 200 μM of each dNTP. Taq polymerase, dNTPs, and PCR buffer for all PCRs were purchased from Invitrogen (Life Technologies Europe BV). PCR cycling conditions were as follows: 10 min initial heating at 95 °C; followed by 30 cycles with 1 min denaturation at 95 °C, 1 min annealing at 58 °C, and 2 min extension at 72 °C, followed by a final 7 min extension at 72 °C. PCRs without DNA template were used as negative controls.
Cloning of PCR products
Amplified fragments of C23O and bssA genes from four sediment samples were selected for cloning and sequencing. PCR products were first purified with a QIAquick PCR Purification Kit (Qiagen, Hilden, Germany) and subsequently cloned using a TOPO-TA PCR Cloning Kit (Invitrogen) according to instructions from the manufacturer. Transformed competent cells were grown at 37 °C overnight on LB agar supplemented with 50 μg mL−1 ampicillin. About 50 positive transformants were picked randomly from each sample with sterilized toothpicks, and PCR was carried out directly on the picked colonies to validate insert sizes using primers M13F-40 (5′-GTTTTCCCAGTCACGAC-3′) and M13R (5′-AACAGCTATGACCATG-3′). PCR cycling conditions were initial heating at 95 °C for 10 min followed by 30 cycles of 30 s denaturation at 94 °C, 30 s annealing at 48 °C, and 1 min extension at 72 °C. A final 10 min extension was carried out at 72 °C.
Sequencing of C23O and bssA gene fragments
Big Dye Terminator v3.1 (Applied Biosystems, Paisley, UK) was used for sequencing reactions and were subsequently analyzed by capillary electrophoresis on the ABI3730XL DNA Analyzer (Applied Biosystems). Obtained nucleotide sequences were first grouped based on length and were then translated to corresponding amino acid sequences. Homology searches were performed using blastx with default parameters as implemented in NCBI (Altschul et al., 1997). The deduced amino acid sequences and reference target sequences were aligned with clustalw with 20% cutoff and 15 gap opening penalty, followed by manual correction. Phylogenetic trees were constructed using the neighbor-joining (NJ) algorithm implemented in the mega 5 software (Tamura et al., 2011). Bootstrap analysis was performed by resampling 1000 times. In order to relate nucleotide sequence of each clone to the common protein motif responsible for aromatic ring cleavage, the database of protein domains, families, and functional sites expasy-prosite (http://prosite.expasy.org/), which is a resource for identification and annotation of conserved regions in protein sequences, was used for analyses of protein motif (Sigrist et al., 2010). Each nucleotide sequence was scanned against prosite patterns and profiles to predict intradomain features in each nucleotide sequence.
Nucleotide sequence accession numbers
The putative C23O and bssA gene sequences reported in this study are available in GenBank under Accession Numbers KC175607–KC175626 and KC175627–KC175643.
Amplicon diversity screening
Terminal restriction fragment length polymorphism was used for assessing the composition of the respective functional guild (aerobic and anaerobic aromatic degraders) as represented by the composition of the amplified C23O and bssA genes in each sediment sample. After isolation of DNA from the microbial community, putative C23O genes were amplified using fluorescently labeled XYLE1-HEX forward and XYLE1 reverse primers, whereas bssA genes were amplified using fluorescently labeled bssA-HEX forward and bssA reverse primers. Amplicons were purified using the QIAquick PCR Purification Kit according to manufacturer instructions (Qiagen). Theoretical terminal restriction fragments (TRF) values of the dominant phylotypes represented in the clone library were calculated based on the sequenced C23O and bssA clones using the neb cutter V2.0 (http://tools.neb.com/NEBcutter2/index.php), and the calculated TRF values were verified experimentally using the corresponding clones as templates. Purified PCR products of C23O gene were fragmented with 1 U of Sau3AI restriction endonuclease (targeting the GATC sequence motif) as instructed by the manufacturer (New England Biolabs, Schwalbach, Germany). It has previously been shown that restriction cutting with Sau3A1 can separate different clades of C23O genes within subfamily I.2.A (Junca & Pieper, 2003). Purified bssA amplicons were fragmented overnight with 1U of MspI (targeting the CCGG sequence motif) at 37 °C. Fluorescently labeled TRFs were separated and quantified by capillary gel electrophoresis using an ABI3730XL DNA Analyzer operated in Genescan mode (Applied Biosystems). The TRFLP electropherograms were analyzed with the genemapper Software v1.7 (Applied Biosystems). Relative peak areas were determined by dividing the individual TRF area by the total area of peaks ranging in size from 70 to 300 bp. Relative phylotype abundances were determined based on the relative peak areas of the corresponding TRF. For consensus profiles, individual runs were aligned using 0.5-bp confidence interval omitting peaks below 0.5% relative abundance. Statistical analyses and calculation of diversity indices were carried out using primer6 package (Primer-E, Plymouth Laboratory). To determine temperature effects, the average relative abundance of individual TRFs (%) from each sediment core, represented by four depths was calculated. For depth effects on gene assemblages, the average relative abundance of individual TRFs at the six different temperatures for each depth layer was estimated: 0–1, 1–2, 2–3, or 3–4 cm.
Q-PCR of C23O and bssA genes
Quantitative PCR tests were performed with the amplification conditions optimized for conventional PCR. It was not necessary to modify PCR conditions to obtain log-linear relationships between gene copy number and cycle threshold (Ct). Quantitative polymerase chain reaction (qPCR) was carried out using a Chromo4 real-time PCR thermocycler (BIO-Rad, CA). The polymerase chain reaction was performed in 20 μL volumes using 0.2 mL qPCR tubes (Bio-Rad). The reaction mixture contained 1× PerfeCTa SYBR Green Fast Mix (Quanta Biosciences, Gaithersburg), 300 nM of each primer, 8% (w/v) BSA (New England Biolabs), 10 ng DNA sample and RNase and DNase free water to a final volume of 20 μL. Reactions for each sample were carried out in duplicate. For C23O genes, the amplification was performed with an initial denaturation step at 95 °C for 5 min, followed by 40 cycles of 30 s denaturation at 94 °C, 30 s annealing at 61.5 °C, and 45 s extension at 72 °C. For bssA, amplification conditions were identical except that annealing was carried out at 58.0 °C for 30 s. Melting curve analysis was performed from 55 to 95 °C to rule out unspecific amplification products. Real-time PCR conditions for quantification of bssA genes has previously been established and tested by Beller et al. (2002).
DNA extracts were tested for inhibitory effects before quantification by determining the number of C23O genes or bssA target molecules in a dilution series of DNA extracts from environmental sample (Stubner, 2002). Serial dilution of DNA extracts (100 ng μL−1, 10 ng μL−1, 1 ng μL−1 and 0.1 ng μL−1) was used to calculate the corresponding gene copy number and monitor qPCR amplification efficiency. DNA extracts with either 10 or 1 ng μL−1 template concentration resulted in equally high amplification efficiency, and 10 ng μL−1 was chosen for all qPCR assays. In addition, selected sediment DNA extracts were spiked with internal standards to validate the quantitative performance of the assay. For the calibration standard, clones of the respective genes targeted in our study were used to obtain standard curves for the group-specific C23O and bssA genes. Plasmids were extracted using Qiagen plasmid isolation kit (Qiagen), and DNA concentrations were quantified using a PicoGreen assay (Invitrogen, Stockholm, Sweden). Copy numbers of plasmids were calculated according to Stubner (2002). Plasmids were serially diluted between 10 and 1010 target molecules per microliter and used to construct standard curves of C23O and bssA gene copies.
All data analyses were performed with opticon monitor 3.1 software (Bio-Rad). The PCR efficiency for each individual sample was derived from the slope of the regression line fitted to a subset of baseline-corrected data points in the log-linear phase using LinReg PCR (Ramakers et al., 2003). The amplification efficiencies were between 93.8% and 98.9%, and the correlation factors (r2) were never below 0.98 for either assay. To estimate the actual gene copy number which corresponds to the representation of each functional guild in each temperature treatment, the average gene copy concentration from the four depths in each core were divided by wet weight of sediment samples used for the DNA extraction. This quantification assumes 100% extraction efficiency and hence represents a conservative abundance estimate.
Results
Aerobic aromatic carbon degraders: C23O genes across lakes
The composition of putative C23O genes based on T-RFLP analysis of Lake sediments from Svarttjärn and Vallentunasjön was significantly different between the lakes (ANOSIM, global R = 0.8, P< 0.01). Samples from Svarttjärn sediment clustered closely together and were clearly different from Vallentunasjön, demonstrating that the lake sediments feature different communities of bacteria with aerobic ring-breaking capacity (Fig. S1).
The XYLE1 primer set was used to amplify C23O genes from both lakes, and 250-bp putative catechol 2,3 dioxygenase gene fragments were recovered from sediment samples of Vallentunasjön, while the corresponding gene product from Svarttjärn was 200 bp. To validate the PCR assay and determine the phylogenetic affiliation of these functional genes, PCR products from two sediment samples from each of the lakes were cloned and sequenced. Phylogenetic trees were constructed based on deduced amino acid sequences (Fig. 0001). No closely matching sequences were available in GenBank for any of the cloned genes. Among the closest matches in GenBank based on amino acid identity were extradiol dioxygenase genes, clone V6 and V19 had 69–62.5% identity to such dioxygenases in Pseudomonas sp. 5K-VPa, Pseudomonas fluorescens pLP6a and Chloroflexus aurantiacus. Several clones, V2, V26, and S12, were instead closely related to other ring cleavage dioxygenases such as biphenyl dioxygenase (cumB) and naphthalene dehydrogenase (Fig. 0001). Clone V11 had 60% identity to Leptothrix cholodnii SP6 (related to C23O subfamily I.2.C) and clone V28 had 67% identity to Acidovorax sp. JS42. Half of the clones cluster into a monophyletic group without closely related sequences in the database, indicating the presence of a new group of putative C23O, sharing identities with gentisate 1,2 dioxygenase genes mediating extradiol cleavage of the benzene ring (Lack, 1959). Two clones from Lake Svarttjärn (S12 and S29) showed low similarity to chlorobenzene dioxygenase genes (tcbAa), which encode both an aromatic ring dioxygenase and a dihydrodiol dehydrogenase (Werlen et al., 1996). Four clones shared protein sequence motif: a 2Fe-2S ferredoxin-type iron-sulfur binding region that mediate electron transfer in aromatic biodegradation mechanisms was identified by expasy-prosite. Six of the clones could be directly linked to TRF of characteristic size (207, 222, 223, 225, 256, and 237 bp) enabling linkages of the sequence based phylogenetic analysis and the T-RFLP community analysis (Fig. 0001).
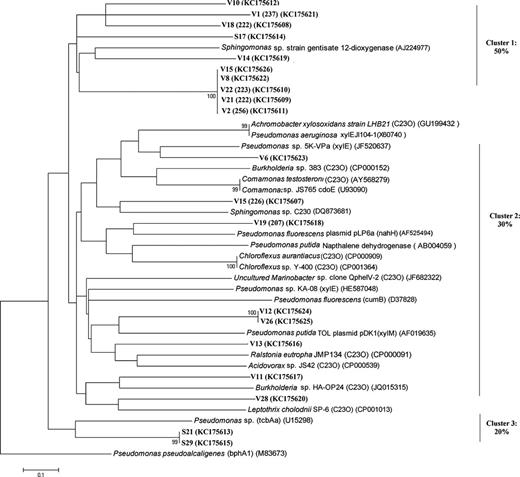
Phylogenetic tree of the deduced amino acid sequences of putative C23O gene fragments of the clone libraries from Lake Svarttjärn (S) and Lake Vallentunasjön (V). The tree is based on a neighbor-joining method. Clones are marked in boldface and some of them have TRF fragment size (between brackets) as verified by T-RFLP analysis. Bootstrap values above 50% are expressed as percentages of 1000 replications and indicated at the branch points. Bar, 0.1 nucleotide substitutions per site. GenBank accession numbers of clones and reference sequences are indicated between brackets. Percentage of clones that cluster together is shown at the right side.
BssA clone libraries and phylogenetic analysis in Lake Svarttjärn
Environmental bssA amplicons were readily amplified from sediment DNA samples originating from Lake Svarttjärn. In contrast, no such amplicons were obtained from the sediment DNA extracted from Lake Vallentunasjön. Most of the cloned bssA sequences from Lake Svarttjärn formed a distinct new group of putative bssA genes that branch from the known delta- and betaproteobacterial bssA reference sequences (Fig. 0002). Two clusters of bssA-like genes closely related to Azoarcus sp. EbN1 and Desulfobacterium cetonicum strain DSM 7267 were also recovered. This is the first report of putative bssA genes in natural lake sediments as bssA was previously only detected in polluted aquifers and marine sediments (Winderl et al., 2007 and Acosta-González1 et al., 2013). Seven of the clones could be linked to a unique fragment size in the T-RFLP analysis (248, 252, 283, 284, and 293 bp, Fig. 0002).
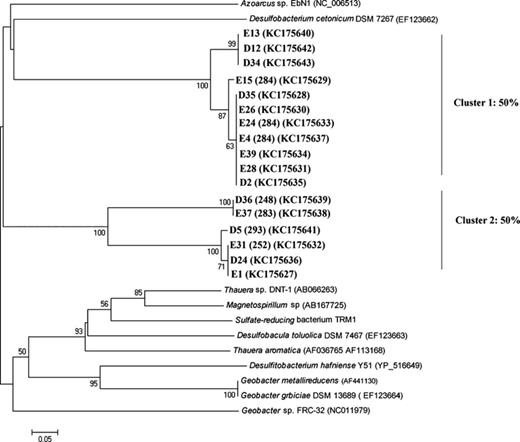
Phylogenetic tree of the deduced amino acid sequences of putative bssA gene fragments of the clone libraries from Lake Svarttjärn. D and E represent clone from two different sediment samples. The tree is based on a neighbor-joining method. Clones are marked in boldface and some of them have TRF fragment size (between brackets) as verified by T-RFLP analysis. Bootstrap values above 50% are expressed as percentages of 1000 replications and indicated at the branch points. Bar, 0.05 nucleotide substitutions per site. GenBank accession numbers of clones and reference sequences are indicated between brackets. Percentage of clones that cluster together is shown at the right side.
Effect of temperature on the relative abundance of putative C23O and bssA genes
Variable incubation temperature caused a considerable change in a number of TRF of C23O genes (C23O T-RFs). Some T-RFs respond positively to temperature while some were negatively affected suggesting ecological differentiation in the C23O-holding community (Fig. 0003). Interestingly, the relative abundance of some C23O T-RFs in Lake Vallentunasjön (190, 220, 252, and 282) respond positively to temperature, whereas they display the opposite response to temperature or disappear altogether at increased temperature in Lake Svarttjärn. These observations imply that different mechanisms of control are acting on these communities in the two lakes. In addition, several C23O T-RFs were only recovered in lake Vallentunasjön (230, 255, 278, and 285 bp) suggesting a more diverse community of the aerobic aromatic-degrading community in this lake.

Relative abundance of selected putative C23O-TRFs in Lake Svarttjärn and Lake Vallentunasjön in response to temperature in the range from 1.0 to 21.0 °C based on T-RFLP analysis. The average relative abundance of individual TRFs (%) from each sediment core represented by four depths was calculated. Each color represents the correlation with temperature as follows: red box represents positive correlation of C23O-TRFs with temperature (P< 0.05), blue box represents negative correlation of OTUs with temperature (P< 0.05), white box represents the relative abundance of OTUs, which had no significant decrease or increase with temperature. The arrows directed to an example for C23O-TRF increase or decrease with temperature in each lake. Remaining TRFs not included in this graph were not significantly influenced by temperature increase.
For Svarttjärn sediments, a considerable diversity of putative bssA genes was detected as well (Fig. 0004). A community change in response to the experimental treatments was apparent as the relative abundance of several OTUs was significantly negatively correlated with temperature. A small number (7) of the putative bssA T-RFs increased with temperature suggesting the emergence of other populations at the highest incubation temperatures.
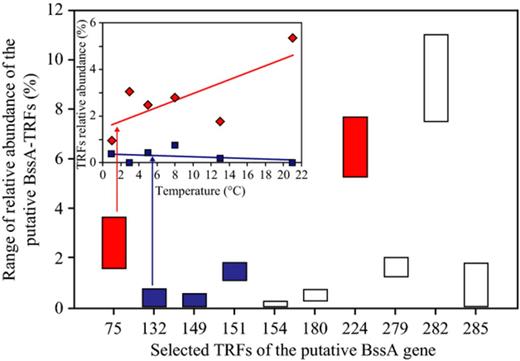
Relative abundance of bssA-TRFs in Lake Svarttjärn in response to temperature in the range from 1.0 to 21.0 °C based on t-RFLP analysis. The average relative abundance of individual TRFs (%) from each sediment core represented by four depths was calculated. Each color represents the correlation with temperature as follows: red box represents positive correlation of OTUs with temperature (P < 0.05), blue box represents negative correlation of OTUs with temperature (P< 0.05), white box represents the relative abundance of OTUs, which had no significant decrease or increase with temperature. The arrows directed to an example for bssA-TRFs increase or decrease with temperature. Remaining TRFs not included in this graph were not significantly influenced by temperature increase.
Quantitative analysis of C23O and bssA genes
The abundance of putative C23O gene copies increased significantly with increasing temperature in Vallentunasjön sediment (P< 0.01; Fig. 0005a), whereas no such response was observed in Lake Svarttjärn. The overall microbial community metabolism assessed as the DIC flux at the sediment water interface (i.e. total community respiration) was positively correlated with the putative C23O gene copy number in Lake Vallentunasjön suggesting a link with the ability to exploit aromatic organic substrates to fuel metabolism (Fig. 0005b). The highest abundance of the putative C23O gene was observed in the two upper layers in Vallentunasjön (0–1 and 1–2 cm depth; Fig. 0006).
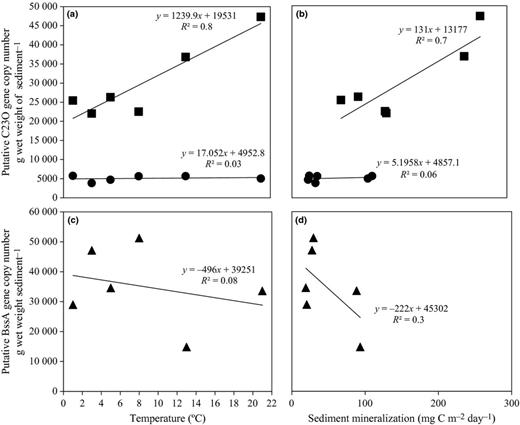
Relationship between aromatic catabolic gene and temperature or sediment mineralization. (a and b) represent the relationship between putative C23O gene and temperature or sediment mineralization in Lake Svarttjärn and Lake Vallentunasjön. (c and d) represent the relationship between putative bssA gene and temperature or sediment mineralization in Lake Svarttjärn. Gene copy number in each temperature was calculated by the average gene copy number of the four depths in each sediment core. The average gene copy number was then divided by wet weight of sediment samples. Putative C23O in Lake Svarttjärn, closed circle; putative C23O in Lake Vallentunasjön, closed square. Putative bssA in Lake Svarttjärn, closed triangle.
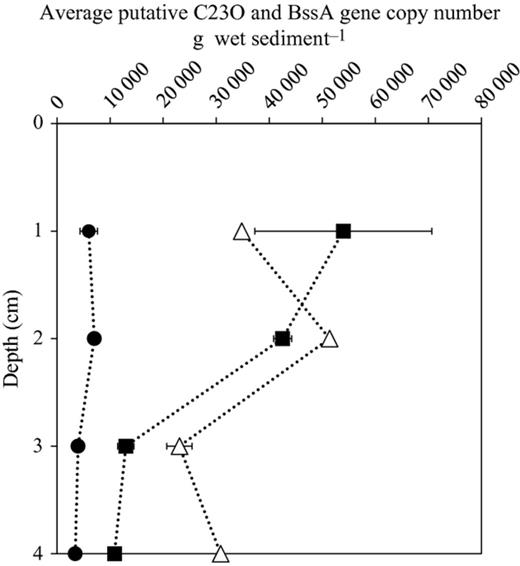
The pattern of gene copy number of putative C23O and bssA genes across depth 1–4 cm. Gene copy number in each depth was calculated by the average gene copy number of the six temperatures in each depth. The average gene copy number was divided by wet weight of sediment samples. For putative C23O, Svarttjärn lake, circle; Vallentunasjön lake; square. For putative bssA gene, triangle. Error bars represent standard deviation from two independent experiments.
For putative bssA genes identified in Svarttjärn, gene copy numbers seemed to be higher at low temperatures (1.0–8.0 °C) than at high temperature (13.0 and 21.0 °C; Fig. 0005c) but there was no significant linear correlation between putative bssA gene copy numbers and temperature within the studied temperature range (1.0–21.0 °C; P> 0.05). Total respiration was also not significantly correlated with the gene copy number of bssA genes within the studied temperature range (1.0–21.0 °C; Fig. 0005d). The highest bssA gene concentration was detected at 2.0 cm depth (Fig. 0006).
Discussion
Temperature effects on C23O gene abundance and microbial community shift
Studies targeting the abundance and diversity of genes coding for central enzymes involved in the catabolism of specific organic compounds can contribute to a better understanding of the in situ potential for biodegradation and the mechanisms controlling this metabolic trait. In the present study, we observed how catechol 2, 3 dioxygenase and benzylsuccinate synthase and the organisms hosting the coding genes, responded to changing temperature and contrasting sediment depth. Putative C23O genes were highly abundant in Vallentunasjön compared with Svarttjärn and in the former lake, temperature had a positive influence on the C23O gene abundance in the studied temperature interval (1–21 °C; Fig. 0005). A high concentration of the C23O gene has previously been observed in a naturally eutrophic lake (Lake Inba, Japan). In that lake, DOM seemed to play a major role in controlling the C23O gene abundance (Kasuga et al., 2007). Hence, DOM is likely to be one critical factor shaping the diversity and functional potential of sediment microbiota and their ability to degrade aromatic pollutants.
Vallentunasjön and Svarttjärn clearly host different C23O populations (Fig. S1) and the phylogenetic analysis of these catabolic genes demonstrated the presence of putative C23O genes with low similarity to other aromatic dioxygenase genes such as biphenyl dioxygenase (cumB), naphthalene dehydrogenase, gentisate 1,2 dioxygenase, and chlorobenzene dioxygenase (tcbAa; Fig. 0001). It is known that the C23O encoded by xylE share a high degree of homology with other metacleavage genes (Eltis & Bolin, 1996) such as plasmid encoded genes for degradation of naphthalene (nahH) and phenol (dmpB) (Bartilson & Shingler, 1989). This may explain why C23O gene amplicons in our study share a high degree of similarity to alternative genes involved in catabolism of aromatic compounds (Fig. 0001).
The continuous incubation period (5 months) at constant temperatures likely resulted in the establishment of stable microbial communities with distinctive characteristics in abundance and diversity of aromatic catabolic genes. The TRFLP-based C23O gene survey indicated a substantial variation in the aromatic-degrading subset of the microbial community (Fig. 0003). The contrasting C23O communities emerging at low and high temperatures are in agreement with recent studies showing that sediment mineralization is primarily constrained by temperature and the quality of the organic matter (Bergström et al., 2010; Gudasz et al., 2010). In Vallentunasjön, the relative abundance of several TRFs increased with temperature indicating that the overall increase in this gene pool was due to proliferation and growth of specific groups. The decline in the relative abundance of other C23O populations might on the other hand reflect a stable or decreasing absolute population size. Most of the specific C23O-TRFs originating from Vallentunasjön grouped in clusters 1 and 2 in the phylogenetic tree (Fig. 0001), indicating a rather diverse microbial community carrying C23O in this lake. Some of the clones with identity to C23O and other dioxygenase genes related to Sphingomonas and Pseudomonas spp. had same size of the temperature increased 222 bp C23O-TRF fragment (Fig. 0001).
It is well known that microbial community composition is strongly influenced by changes in temperature (Robador et al., 2009), and temperature also correlates positively with bacterial biomass and organic matter mineralization (Gudasz et al., 2012). Vallentunasjön is rich in nutrients and biologically labile autochthonous organic matter and this is also where the abundance of C23O genes is highest and features a dynamic temperature response. Hence, an array of metabolic changes paralleled by changes in microbial community composition is likely to take place at increased temperature in this system. Analogously to our study, shifts in the relative abundance of active methanotrophs was recently reported in arctic lake sediments incubated in a temperature gradient from 4 to 21 °C (He et al., 2012). In contrast, Svarttjärn sediments receive mostly recalcitrant allochthonous organic matter imported from the watershed. Sediment microbiota possessing C23O genes in this system are thus likely to be constrained by low availability of organic substrates and nutrients and accordingly consequently featured a weak (nonsignificant) response to increased temperatures, even after 5 months consecutive experimental manipulation.
Anaerobic biodegradation of aromatic compounds
Lake sediments are typically characterized by a shallow oxygenated zone at the surface with large underlying zones where oxygen is absent and alternative electron acceptors are being used, such as nitrate, iron oxides and sulfate (Fenchel & Finlay, 1995; Braker et al., 2001; Sobek et al., 2009; Gudasz et al., 2012). Our results suggest that sediments in Lake Svarttjärn compared with Vallentunasjön, host an alternative anaerobic pathway for biodegradation of aromatic compounds, inferred from the presence of bssA genes rather than C23O genes. This is likely reflecting the environmental constraints on Svarttjärn sediments with high inputs of humic acids and infrequent mixing and hence also poor or irregular aeration of the surface lake sediment. In contrast to C23O in Vallentunasjön, the abundance of putative bssA genes was not influenced by the experimental manipulation of temperature.
We suggest that humic substances might be one direct factor responsible for the presence of the putative bssA gene in Lake Svarttjärn by acting as a potential electron acceptor for aromatic biodegradation. In an earlier experiment, a group of benzene degrading bacteria became active after the addition of humic acids acting as direct electron acceptors and resulting in a reduced humic acid that can in turn be oxidized by Fe(III) oxides (Lovley et al., 1996). Anoxic microbial oxidation of toluene to CO2 with humic substances as electron acceptors has also been demonstrated in enriched anaerobic sediments from the Amsterdam petroleum harbor (APH) and the Rhine River (Cervantes et al., 2001). Hence, we suggest that the abundance of humic acids and prevailing anaerobic conditions could be a primary factor shaping the composition of sediment bacteria in Lake Svarttjärn.
Diversity of bssA genes
Two new clusters of prevalent and abundant bssA-like genes were identified in Svarttjärn sediments. Recently, bssA-like genes were classified according to their substrate specificity (mono-aromatics and naphthalene derivatives), assuming that these genes are linked to new degrader groups in polluted sediments (Acosta-González et al., 2013). This gene is one of the main biomarkers for anaerobic aromatic biodegradation and with the paucity in existing data, it is not surprising that there are no close relatives in existing databases (Fig. 0002). At present, one may only speculate about the substrate specificity and taxonomic placement of these genes and further studies and screening of different taxa for these genes are needed because the selected primers might not target all functional guilds of bacteria carrying the gene, such as sulfate and iron reducers. The relative abundance of five bssA-TRFs was stable or changed only slightly in response to shifts in temperature while the other five bssA-TRFs have no significant response to temperature indicating the role of other factors (i.e. quality of organic matter) in controlling their growth/existence.
Microbial community shift over depth
The abundance of C23O and bssA genes changed markedly over depth with the highest abundances in the upper sediment layers (0–2 cm) followed by a decrease with increasing sediment depth (Fig. 0006). One reason for this could be that the upper sediment layer typically hosts the most active microbial communities (Fisher et al., 2002; Haglund et al., 2003). It is often observed that the community composition of sediment microorganisms change over sediment depth, at least when assessed with phospholipid fatty acid profiles or 16S rRNA gene sequences (Fierer et al., 2003; Agnelli et al., 2004; Goberna et al., 2005; Will et al., 2010). Analogously, bacterial diversity increased from the water to the surface sediment and then decreased rapidly with increasing sediment depth (Tšertova et al., 2013). It has been suggested that such changes in bacterial community composition might result from more frequent mixing of the upper sediment layers compared with deeper sediment layers. In our studied lakes, higher availability of fresh labile organic carbon and terminal electron acceptors in surface sediments likely enhanced the abundance of bacteria carrying ring-breaking enzymes (Fig. 0006), while their abundance in deeper sediments was impaired by a combination of oxygen depletion and poor substrate quality and availability.
Degradation of naturally occurring aromatic compounds
Temperature and the source of organic carbon (allochthonous or autochthonous) typically exert a strong influence on bacterial metabolic activities in lake sediments (Schallenberg & Kalff 1993), and significant decreases in organic carbon burial were found due to the such temperature effects in the sediments included in the present study (Gudasz et al., 2010). Furthermore, global warming is expected to result in increased OC mineralization (Solomon et al., 2007) and coupled to this an expected concurrent decrease in burial of recalcitrant organic carbon in boreal lake sediments. This is likely to result in shifts in microbial communities and may according to our results from Vallentunasjön also lead to higher abundances of organisms carrying genes for oxic catabolism of aromatic compounds (Fig. 0005a). One plausible reason for this response is the labile nature of the carbon feeding these sediments and associated higher overall mineralization and nutrient availability, which likely underlie a more dynamic response to the temperature manipulations in the sediment microbiota. In contrast, the abundance of C23O genes in Lake Svarttjärn was stable across the variable temperatures, suggesting that the slower utilization of terrestrially derived organic matter may lead to metabolic stability in humic lakes as previously suggested (Gudasz et al., 2012) with only minor temperature effects on bssA abundance.
In conclusion, the distribution of C23O and bssA genes in the lakes studied here was tightly linked to the quality of the sediments under scrutiny, whereas temperature effects were limited to sediments receiving mainly autochthonous organic matter inputs. Studies assessing the role and environmental distribution of C23O, bssA and other genes central to the catabolic degradation of aromatic pollutants can provide essential new knowledge about the ecology of microorganisms capable of degrading aromatic pollutants and the potential of ecosystems to cope with episodic or gradual inputs of such pollutants to the environments. So far, this has been insufficiently investigated because of the limited availability of appropriate molecular markers, but with the recent progress in this area, this is likely to change in the near future.
Acknowledgements
The study was funded by the Swedish Research Council (grant to S.B.) and postdoctoral scholarships from the Swedish Institute and Carl Tryggers foundation to O.A. Experimental sediment incubations were enabled with support from the Malméns foundation, the Swedish Research Council Formas via a grant to LEREC (Lake Ecosystem Response to Climate Change) and an instrument grant from the Knut and Alice Wallenberg Foundation to Lars Tranvik.
References
Supporting Information
Additional Supporting Information may be found in the online version of the article:
Table S1. Primer sets used in this study.
Author notes
Editor: Tillmann Lueders