-
PDF
- Split View
-
Views
-
Cite
Cite
Sergio Pérez-Ortega, Rüdiger Ortiz-Álvarez, T.G. Allan Green, Asunción de los Ríos, Lichen myco- and photobiont diversity and their relationships at the edge of life (McMurdo Dry Valleys, Antarctica), FEMS Microbiology Ecology, Volume 82, Issue 2, November 2012, Pages 429–448, https://doi.org/10.1111/j.1574-6941.2012.01422.x
- Share Icon Share
Abstract
Lichen-forming fungi are among the most diverse group of organisms in Antarctica. Being poikilohydric, lichens are able to cope with harsh environmental conditions that exclude other organisms like vascular plants. The McMurdo Dry Valleys (Victoria Land, Continental Antarctica) are a hyperarid cold desert where macroscopic life is reduced to a few lichens and bryophyte species. We investigated the diversity of lichen-forming fungi and their associated photobionts in three valleys (Garwood, Marshall, and Miers). Correct identification of lichen-forming fungi from extreme ecosystems is complicated by the presence of numerous sterile and extremely modified thalli. To overcome this problem, we used a combined approach for the identification of the species present in the area, the first involving identification by means of standard characters and the second, two DNA-based (ITS region) species delimitation methods (General Mixed Yule-Coalescent model and genetic distances). In addition, we also used ITS sequences for the identification of the photobionts associated with the mycobionts. We studied the relationships between both bionts and assessed the degree of selectivity and specificity found in those associations. We also looked for landscape level spatial patterns in these associations. The two DNA-based methods performed quite differently, but 27 species of lichen-forming fungi and five putative species of photobionts were found in the studied area. Although there was a general trend for low selectivity in the relationships, high specificity was found in some associations and differential selectivity was observed in some lichen-forming fungi. No spatial structure was detected in the distribution of photobionts in the studied area.
Introduction
Lichenization is the strategy of symbiotic trophically specialized fungi (mycobionts) that obtain carbon from their photobionts (green algae and/or cyanobacteria) (Hawksworth & Honegger, 1994). With c. 13 500 (Hawksworth, 2001) accepted species and more than 18 000 estimated (Sipman & Aptroot, 2001), lichens dominate ecosystems that cover c. 8% of Earth surface. Being poikilohydric, lichens are able to cope with the most extreme conditions for life and can grow in places where environmental conditions are not suitable for other macroorganisms like plants. Such an extreme environment is the Antarctic continent, which is mostly covered by ice, and terrestrial ice-free areas represent < 0.5% of the territory (Peat et al., 2007). The largest of these free-ice areas are the McMurdo Dry Valleys in Southern Victoria Land. Classified as a hyperarid cold desert, the Dry Valleys are characterized by the extreme cold, windy, and dry conditions and a lack of liquid-water available for life as snow usually sublimes rather than melting. In this severe environment, only a few very highly specialized communities of microorganisms are able to survive (Pointing et al., 2009). Lichens, although able to survive in these extreme conditions, have to deal with a specially severe environment such as the extreme low temperatures (mean annual temperature is −19.8 °C) and dark winters. Their poikilohydric lifestyle means that their water content tends to equilibrate with that of the surrounding environment (Kappen & Valladares, 2007). They are only active when hydrated, and in the Dry Valleys, liquid-water is rarely available. When they are active, they have to suffer the high light and associated UV levels during clear days (Kappen et al., 1998; Green, 2009).
Lichens are among the most diverse organisms present in this challenging environment; however, research on them is scarce (Friedmann et al., 1980; Kappen et al., 1981; De los Ríos et al., ,b; Sancho et al., 2007; Ruprecht et al., 2010) and no local checklist is so far available. In addition, the taxonomy of Antarctic lichens is still not very well known, and the identification of lichen samples from Antarctica can be a very difficult task (Hertel, 1987, 1988), despite the recent release of a regional flora (Øvstedal & Lewis-Smith, 2001). Lichen thalli usually show considerable modifications in response to strong winds, freeze-thaw changes, and the extreme temperatures of Antarctica. In some cases, this leads to the adoption of endolithic growth forms (Friedmann, 1982; De los Ríos et al.,,b). Furthermore, in many cases, thalli can be reduced to few sterile thallus squamules. These changes have not been always taken into consideration, and this has led to the description of numerous superfluous names (Castello & Nimis, 1995a). For these reasons, we performed a combined approach in which we used standard morphological, anatomical, and chemical characters for the identification of the species together with a classification of specimens based on DNA sequences. DNA-based species delimitation has been already used successfully to delimit several groups of lichens (Kroken & Taylor, 2001; Wirtz et al., 2008; Leavitt et al., 2011). Although several DNA regions, such as a portion of the mitochondrial gene cytochrome oxidase I, have been explored as potential barcodes for fungi, ITS is accepted as the best candidate for DNA barcoding for most fungal groups (Seifert, 2009). In addition, it is the most sequenced region for this group of organisms (Begerow et al., 2010). Further, this region has been recently selected and tested for its use in species delimitation and identification in lichens with a high degree of success (Del Prado et al., 2010; Kelly et al., 2011).
Knowledge about the photobionts of Antarctic lichens is also scarce and is limited to a few species from scattered localities (Aoki et al., 1998; Romeike et al., 2002; Wirtz et al., 2003; De los Ríos et al., 2005b; Engelen et al., 2010). Lichens with asexual strategies (soredia, isidia, and thallus fragmentation) can disperse both bionts together (Wornik & Grube, 2010), but in those species that reproduce sexually, the germinating fungal spores must find suitable photobionts for lichenization. Studies on photobiont diversity and on fungal selectivity toward the photobiont in lichens have proliferated during the last decade at the species level, for a few organisms (Kroken & Taylor, 2000; Romeike et al., 2002; Yahr et al., 2004; Blaha et al., 2006; Guzow-Krzemińska, 2006; Ohmura et al., 2006; Hauck et al., 2007; Muggia et al., 2008; Nelsen & Gargas, 2008; Pérez-Ortega et al., 2010; Werth & Sork, 2010; Fernández-Mendoza et al., 2011), and also, but less so, at the family level (Helms et al., 2001; Otálora et al., 2010). The large increase in studies on the identification of photobionts diversity, both within species and within communities, is attributable to the availability of specific primers for photobionts, especially those for the internal transcriber spacer (ITS), one of the most variable regions used in these studies (Kroken & Taylor, 2000; Yahr et al., 2004; Ohmura et al., 2006; Doering & Piercey-Normore, 2009; Fernández-Mendoza et al., 2011). We aimed to demonstrate the photobiont diversity present in lichen symbioses in one of the harshest environments on Earth, the McMurdo Dry Valleys, using ITS sequences from the photobionts. Further, we aimed to determine whether spatial patterns exist in the distribution of photobionts in the McMurdo Dry Valleys as very few studies have focused on the study of photobiont diversity and selectivity at a community level (Beck, 1999; Beck et al., 2002; Doering & Piercey-Normore, 2009; Bačkor et al., 2010).
The terms ‘specificity and selectivity’ have been used to define the degree of interaction between lichen photo- and mycobionts (Galun & Bubrick, 1984; Beck et al., 2002). Beck et al. (2002) revised both terms as they had been variously applied in the literature. Thus, selectivity (applied to one of the bionts in the symbiosis) refers to the taxonomic range of partners that are selected. Specificity should be used for the symbiotic association as a whole and, therefore, depends on the range and taxonomic relatedness of acceptable partners, that is, the degree of selectivity of the partaking bionts. Based on our lichen identifications, we aimed to investigate the degree of selectivity by the mycobiont toward the photobiont in the lichens present in the study area. Wirtz et al. (2003) demonstrated low selectivity toward their photobionts in some cyanobacteria-containing lichens in maritime Antarctica and attributed this to high selectivity toward the photobionts being detrimental in Antarctic lichens. Mycobionts with low selectivity are able to colonize a wider range of habitats where different photobiont pools are available (Romeike et al., 2002; Wirtz et al., 2003). Hence, we expected a very low selectivity toward the photobiont in the harsh environment of the Dry Valleys.
The aims of this study can be summarized in the following questions:
What is the actual diversity of lichen myco- and photobionts in the McMurdo Dry Valleys?
Is there any type of spatial structure at the landscape level in the photobionts present in the area?
Do the lichens occurring in this harsh environment actually show a low selectivity toward their photobionts?
Materials and methods
Sampling location
Samples were collected in the southern part of the Dry Valleys, Southern Victoria Land, continental Antarctica. The area, approximately bounded by 78°00′–78°10′S latitude and 163°45′–164°15′E longitude, contains three ice-free valleys, Garwood, Marshall and Miers (from north to south), and hills reaching 800–1000 m between them (Fig. 1). The region is ultraoligotrophic and is classed as a hyperarid cold desert. Annual precipitation is < 100 mm, mean air temperature is −19.8 °C although in midsummer (December and January) it can be around 0 °C and in midwinter (July and August) as low as −45 °C. Relative humidity is generally low, down to 10%, while soil moisture is around 1–2% or lower. Winds can be very strong and have been measured up to over 140 km h−1. The Dry Valley area is one of the coldest and driest areas in the world. Three sampling areas were used: A, the western part of the hills between Garwood and Marshall Valleys. Sampling sites ranged from the near the valley floor (about 150 m altitude and mainly moraine drift) to 800–100 m on the hills (granite and marble rocks). B, an area of higher ground between the top of the Miers and Garwood Valleys; sample sites were as low as 250 m in the Miers but typically around 400 m. The main rock types were granite, schist, and mafic. C, the western end of the hills forming the south side of the Miers Valley. Sample sites were between 400 and 800 m, and predominant rock types were granites and gneiss. All sampling was approved by the appropriate New Zealand authority. Samples were collected in all accessible areas from the two base camps located within the Miers and Garwood valleys. Intensive collections were not carried out because of current restrictions that require the number of samples to be kept to a minimum.
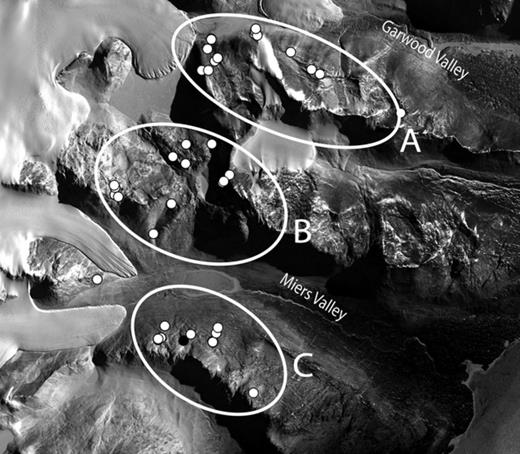
General view of the studied area showing the sampling points (white dots). A, B, and C denote the three geographical areas in which samples have been divided. The Marshall Valley lies between the Miers and Garwood Valleys. The image is approximately 15 km across and 12 km high and the white on the left shows glaciers.
Morphological identification of lichen samples
Lichens collected for this study were identified using standard morphological, anatomical, and chemical characters. Øvstedal & Lewis-Smith (2001) was used as the starting point for most of the identifications although additional available literature was also used for most of the groups. Thin-layer chromatography was used to detect secondary lichen metabolites in some groups according to Orange et al. (2001).
Molecular analyses
Specimens were examined under the dissecting microscope, and small pieces of thallus were cut with the help of sterilized razor blade and needle. Although lichenicolous fungi were not common, special care was taken to avoid possible cross-contaminations. When epilithic thallus was not present, a small piece of the endolithic thallus beneath the apothecia, including algae, was used for DNA extraction. Samples were placed in 1.5 mL microcentrifugue tubes, and DNA was extracted using either DNEasy Plant Mini Kit® (Qiagen) or a modified version of the CTAB method (Cubero et al., 1999). Primers ITS1T and ITS4T, specific for Trebouxia species (Kroken & Taylor, 2000), were used to amplify a nuclear ribosomal DNA segment which includes the ITS1, 5.8S, and ITS2 regions. The homologous region from the mycobiont genome was amplified using the fungal-specific primer pair ITS1F (Gardes & Bruns, 1993) and ITS4 (White et al., 1990). PCRs were prepared to a final volume of 30 μL; containing 1.5 μL of each primer (10 μM), 6 μL of dNTPs (1 mM), 1.2 μL of MgCl2 (50 mM), 3 μL of reaction buffer (Biotools®), 0.6 U of DNA polymerase (Biotools®); final volume was reached by adding distilled water. Amplification of the fungal ITS conditions was by the following procedure: one initial denaturing step at 94 °C for 4 min followed by five cycles of 1 min at 94 °C, 1 min at 55 °C, and 1 min at 72 °C; after 30 cycles of 30 s at 94 °C, 1 min at 50 °C, and 1 min at 72 °C; ending up by an extension step of 10 min at 72 °C, after which the samples were kept at 4 °C. PCR amplifications for algal ITS were set up following the conditions given in Kroken & Taylor (2000). Two samples, s223 and s225, failed to amplify the photobiont region ITS, so we used the primer pair SR1 and SR12 for the 18S ribosomal DNA (Nakayama et al., 1996) following their PCR conditions. PCR products were purified and cleaned using QIAGEN quick spin columns (Qiagen®). Both complementary DNA strands were sequenced at MACROGEN (South Korea).
Sequence alignment and phylogenetic analysis
Sequence fragments obtained were checked and assembled in seqmanii v.5.07© (DNASTAR Inc.). Sequence alignments were performed using muscle 3.8 (Edgar, 2004) and manually checked and corrected in bioedit 7.0.5.2 (Hall, 1999). Sequences ends and ambiguously aligned regions were trimmed and removed using gblocks 0.91b (Castresana, 2000), except for the genetic distances analysis, in which only sequence ends were trimmed. gblocks parameters were adjusted to favor minimizing the number of regions excluded, with the option ‘With Half’ set for the allowed gap positions. For the analysis of mycobiont sequences, only the regions ITS1, 5.8S, and ITS2 were used in the subsequent analyses. In the case of photobiont sequences, we also included a small portion (83 nt) of the nuLSU, shared by all the obtained sequences. For the analysis of photobiont sequences, alignment was collapsed into haplotypes with collapse1.2 (D. Posada, available at http://darwin.uvigo.es) with gaps treated as a fifth state. These haplotypes were subsequently aligned with sequences from representative species of Trebouxia available at GenBank and also with the most similar hits for each haplotype found in a BLAST search. Nucleotide substitution models were statistically selected with the help of jmodeltest (Posada, 2008, program available at http://darwin.uvigo.es). Model selection was made according to the Akaike's information criterion (Akaike, 1974); the General Time Reversible substitution model (Tavaré, 1986) with estimation assuming a gamma distribution (GTR + G) had the lowest −ln L value. Phylogenetic analysis for photobionts was carried out using the Metropolis coupled Bayesian Markov Chain Monte Carlo algorithm (MC)3 implemented in the software MrBayes v.3.1.2 (Ronquist & Huelsenbeck, 2003). Maximum likelihood (ML) analyses were carried out using the online version of phyml 3.0 (Guindon et al., 2010, available at http://www.atgc-montpellier.fr/phyml/), conducting a nonparametric bootstrap analysis (1000 replicates) to assess branch support (Felsenstein, 1985). The (MC)3 analysis was run for 5000K generations starting from a random tree, employing 12 simultaneous chains and using the default temperature of 0.2. Every 200th tree was sampled while the first 5000 trees were discarded as burn-in after examination of runs in tracer v1.5 (Rambaut & Drummond, 2007). Phylogenetic trees were drawn using the program FigTree v1.3.1 (available at http://tree.bio.ed.ac.uk/software/figtree/). Statistical parsimony networks (Templeton et al., 1992) for the photobiont ITS were calculated using the software tcs 1.21 (Clement et al., 2000, available at http://darwin.uvigo.es), using gaps as a fifth character.
DNA-based species delimitation in lichen-forming fungi
Two different approaches were followed for lichen species delimitation. The first method uses an algorithm introduced by Pons et al. (2006) that delimits groups of specimens based on the rates of lineage branching between different groups. Using an ultrametric tree, the algorithm looks for the species boundaries differentiating between intraspecific and interspecific branching patterns (Pons et al., 2006). The General Mixed Yule-Coalescent model (GMYC) implemented in this algorithm is fitted to an ultrametric tree and optimizes the threshold position of the switch between intraspecific (modelled by a neutral coalescent model) and the interspecific (ruled by a stochastic birth only or Yule model). Thus, events older than the threshold are considered as speciation events, and younger ones are considered as coalescent events. A standard log-likelihood ratio test comparing the likelihood for the mixed model to that obtained assuming a single branching process for the entire tree is used to assess whether there is significant evidence for species recognition. Monaghan et al. (2009) presented an improvement in the original algorithm in which the threshold between intraspecific and interspecific processes is assigned independently to each lineage. Both versions of the GMYC algorithm were implemented in R (R Development Core Team) using the command GMYC of the APE library (Paradis et al., 2004).
Phylogenetic relationship among fungal specimens and the ultrametric tree needed for the implementation of the GMYC algorithm was obtained using beast v1.6.1 (Drummond & Rambaut, 2007). A GTR + I + G model of substitution (Tavaré, 1986) was used in the analysis. The uncorrelated lognormal relaxed clock (Drummond et al., 2006) was used with a fixed clock rate of 1.0. Tree prior was adjusted to a Yule speciation process. Four independent searches of 20 000 000 generations each were run sampling every 200 steps and starting from a random tree. Outputs of all searches were combined using log-combiner v.1.5.3. TreeAnnotator v.1.5.3 was used to generate a single tree by the method of maximum clade credibility (MCC), removing the first 10 000 trees as burnin after examination of runs in tracer v1.5 (Rambaut & Drummond, 2007).
The second approach used for species delimitation was based on genetic distances. Genetic distances have been recently shown as a reliable method for species delimitation in lichens (Del Prado et al., 2010). We followed the scheme proposed in Del Prado et al. (2010) with slight modification to set groups of specimens. Genetic distances among fungal sequences were calculated after the alignment of sequences and the removal of ambiguous regions using gblocks 0.91b (Castresana, 2000). Pairwise ML distances among sequenceswere calculated with tree-puzzle 5.2 (Schmidt et al., 2002) using the GTR + I + G model of substitution (Tavaré, 1986) as it was inferred in jmodeltest (Posada, 2008). Genetic distances were imported in mothur 1.15 (Schloss et al., 2009), and groups of specimens were calculated according to two genetic thresholds: ≥ 0.05% and ≥ 0.02% of dissimilarity.
Specificity and fungal selectivity toward the photobiont
Specificity was estimated from the frequency of certain photobiont haplotypes that were found in the different lichen samples. We used the fungal groups as defined by a threshold of ≥ 0.05 genetic distance dissimilarity (those groups were almost coincident with those obtained by standard identification) and photobiont haplotypes. Data were analyzed in two different ways, first using a raw dataset where the frequency of photobiont haplotypes were not taken into account (absence vs. presence), and a second dataset in which we used standardized photobiont data to avoid the effect of photobiont abundance in the search of fungal–photobiont relationships. For that purpose, we calculated the relative frequency of a fungal–photobiont association by dividing by the total number of that photobiont haplotype. First, we tested in both datasets the independence of fungal ‘species’ and photobiont haplotypes by mean of a chi-squared test, using Monte Carlo estimation of exact P-values. Correspondence analysis was used to detect selectivity associations between certain fungal groups and photobiont haplotypes. In addition, we tested, by means of Spearman correlations, the independence of the lichen samples in terms of their photobiont composition. These analyses were carried out in spss 12.
To compare the differential selectivity showed by the lichen species found in the study area, we calculated the nucleotide diversity (π) and haplotype diversity (Hd) of the photobionts associated with each lichen species. π is a measure of polymorphism in populations (Nei, 1987), and Hd is a measure of the uniqueness of the haplotypes present at the populations (Nei, 1987). Genetic diversity calculations were carried out in dnasp v5 (Librado & Rozas, 2009).
Spatial analysis of the photobiont genetic structure
To characterize the spatial genetic structure of the photobiont, we only used those samples with ITS sequences (excluding the nu18S sequences). We built a ML genetic distance matrix, as is described in the previous section, for the mycobionts using the GTR + G model of nucleotide substitution as inferred by jmodeltest (Posada, 2008). A geographic distance matrix was constructed based on Euclidean distances with the geographical coordinates taken for each specimen and using the GEOGRAPHIC distance matrix generator v.1.2.3 (available at http://biodiversityinformatics.amnh.org/open_source/gdmg). Distances between samples collected on the same rock were artificially set at 0.1 m.
We tested our dataset for relationship between matrices by means of a Mantel test with 9999 permutations. Mantel coefficients obtained with this test can be interpreted as a parametric Pearson correlation (Matesanz et al., 2011). Mantel test were performed in the R statistical environment (R Development Core Team, 2010), using the package vegan (Oksanen et al., 2011). Lichen samples were collected primarily in three main locations (see Fig. 1), so we tested for photobiont spatial structure among these three groups of samples by means of an amova analysis (Excoffier et al., 1992), using the package pegas (Paradis, 2010), implemented in R (R Development Core Team, 2010). Spatial structure within the three groups was tested using Mantel tests. Finally, we tested the explanatory value of the altitude in the matrix of genetic distances of the photobionts using a Mantel test. A partial Mantel test was also performed using the geographic distance matrix, the altitude matrix, and the genetic distance matrix.
Results
Lichen-forming fungi diversity and species delimitation
We analyzed a total of 124 lichen-forming fungi samples that were first named by the standard approach using morphological, anatomical, and chemical characters. A total of 122 ITS sequences were recovered and used for DNA-based species delimitation (we failed repeatedly to amplify the ITS region from specimens s196, s204 and s182). The specimen s107 did not recover any close relative in a BLAST search with a score higher than 95%; the external appearance resembled to that of a Polysporina species (no mature asci found), so we suspect that the sequence is a contamination by an endolithic lichen-forming fungus. The final alignment used for the distance analysis was 575 bp length (333 variable characters). The alignment for the phylogenetic and subsequent GMYC analyses was 509 bp (290 variable characters) after removing ambiguous aligned regions using gblocks 0.91b (Castresana, 2000). For computing the ML distance matrix in tree-puzzle 5.2 (Schmidt et al., 2002), we used the following values estimated in jmodeltest (Posada, 2008): rate matrix r(AC) = 0.8771, r(AG) = 4.1170, r(AT) = 1.1080, r(CG) = 0.8881, r(CT) = 2.1328, r(GT) = 1; base frequencies π(A) = 0.2299, π(C) = 0.2636, π(G) = 0.2969, π(T) = 0.2096; gamma shape parameter α = 1.442; and the proportion of invariable sites P(invar) = 0.3280.
The different methods used for DNA delimitation of species produced slightly different results, which are summarized in Fig. 2 and Table 1. The likelihood of the GMYC model based in a single threshold (Pons et al., 2006) was significantly higher than the null model of uniform branching rates (Table 2). The model estimated 23 different species, with a confidence interval ranging from 21 to 26. The likelihood of the multiple GMYC model (Monaghan et al., 2009) was also significantly higher than the null model (Table 2), and it estimated 31 putative species, with an interval of 22–39. The delimitation based on genetic distances recovered 21 groups based on a 5% cutoff and 26 groups following a 2% cutoff.
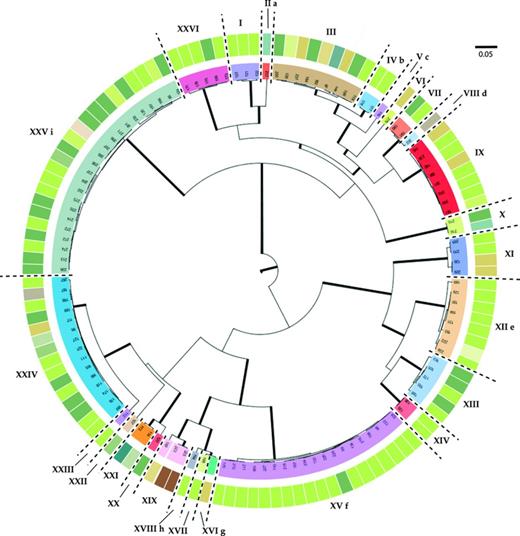
MCC phylogenetic tree showing the relationships between the mycobiont samples studied. Branches in bold are those with a posterior probability (PP) ≥ 0.95. Groups inferred by a genetic distance of 0.02% of dissimilarity and supported by morphological data are separated by dashed lines and denote by roman numerals (correspondences can be found in Table 1). Each sample has associated with the photobiont haplotype found associated (depicted with a color code, corresponding to colors and haplotypes showed in Fig. 3). Conflicts among DNA-based species delimitation methods are depicted by means of letters: a, Carbonea sp. (s217) is included in Carbonea vorticosa (I) using the 0.05 cutoff; b, Austrolecia sp. 2 (s177 and s178) was included in Austrolecia sp. 1 using the 0.05 cutoff; c, Austrolecia sp. 3 (s116) was included in Austrolecia sp. 1 using the 0.05 cutoff; d, Austrolecia sp. 3 (s116) was included in Austrolecia sp. 1 using the 0.05 cutoff and the single GMYC; e, multiple GMYC recognized s169 as a different taxon; f, multiple GMYC split Umbilicaria aprina (XV) as two different taxa; g, neither of the GMYC algorithms nor the 0.05 cutoff were able to recognize Polysporina sp. as a different entity different from Polysporina frigida; h, single GMYC failed to recognize Acarospora cf. nitrophila as an independent entity; i, multiple GMYC split Rhizoplaca macleanii in two taxa.
Samples used in this study with their corresponding laboratory code, the photobiont haplotype found associated in that sample, their affiliation to the groups obtained by the different DNA-based species delimitation methods, and the accession numbers for the ITS mycobiont and photobiont sequences
Laboratory code | Algal hap | Distance 0.05 | Distance 0.02 | GMYC single | GMYC multiple | Fungal ITS | Algal ITS | |
Carbonea vorticosa | s122 | hap3 | I | I | I | I | JX036053 | JX036174 |
Carbonea vorticosa | s123 | hap3 | I | I | I | I | JX036054 | JX036175 |
Carbonea vorticosa | s121 | hap3 | I | I | I | I | JX036052 | JX036173 |
Carbonea sp. | s217 | hap15 | I | II | II | II | JX036120 | JX036241 |
Austrolecia sp. 1 | s119 | hap3 | III | III | III | III | JX036050 | JX036171 |
Austrolecia sp. 1 | s128 | hap8 | III | III | III | III | JX036059 | JX036180 |
Austrolecia sp. 1 | s168 | hap9 | III | III | III | III | JX036073 | JX036194 |
Austrolecia sp. 1 | s184 | hap1 | III | III | III | III | JX036089 | JX036210 |
Austrolecia sp. 1 | s199 | hap1 | III | III | III | III | JX036103 | JX036224 |
Austrolecia sp. 1 | s200 | hap1 | III | III | III | III | JX036104 | JX036225 |
Austrolecia sp. 1 | s231 | hap6 | III | III | III | III | JX036134 | JX036253 |
Austrolecia sp. 1 | s182 | hap10 | III | III | III | III | JX036087 | JX036208 |
Austrolecia sp. 1 | s97 | hap17 | III | III | III | III | JX036154 | JX036273 |
Austrolecia sp. 2 | s177 | hap3 | III | IV | IV | IV | JX036082 | JX036203 |
Austrolecia sp. 2 | s178 | hap3 | III | IV | IV | IV | JX036083 | JX036204 |
Austrolecia sp. 3 | s116 | – | III | V | V | V | JX036047 | – |
Lecanora sp. 1 | s275 | hap6 | VI | VI | VI | VI | JX036149 | JX036268 |
Lecanora cf. flotowiana | s190 | hap1 | VII | VII | VII | VII | JX036095 | JX036216 |
Lecanora cf. flotowiana | s191 | hap3 | VII | VII | VII | VII | JX036096 | JX036217 |
Lecanora cf. mons-nivis | s202 | hap13 | VII | VIII | VII | VIII | JX036106 | JX036227 |
Lecidella greenii | s300 | hap3 | IX | IX | IX | IX | JX036150 | JX036269 |
Lecidella greenii | s301 | hap3 | IX | IX | IX | IX | JX036151 | JX036270 |
Lecidella greenii | s266 | hap9 | IX | IX | IX | IX | JX036141 | JX036260 |
Lecidella greenii | s230 | hap3 | IX | IX | IX | IX | JX036133 | JX036252 |
Lecidella greenii | s203 | hap3 | IX | IX | IX | IX | JX036107 | JX036228 |
Lecidella greenii | s192 | hap3 | IX | IX | IX | IX | JX036097 | JX036218 |
Lecidella greenii | s181 | hap9 | IX | IX | IX | IX | JX036086 | JX036207 |
Lecidella greenii | s115 | hap6 | IX | IX | IX | IX | JX036046 | JX036168 |
Rhizoplaca sp. | s215 | hap1 | X | X | X | X | JX036118 | JX036239 |
Rhizoplaca sp. | s216 | hap15 | X | X | X | X | JX036119 | JX036240 |
Huea sp. | s269 | hap3 | XI | XI | XI | XI | JX036143 | JX036262 |
Huea sp. | s270 | hap3 | XI | XI | XI | XI | JX036144 | JX036263 |
Huea sp. | s229 | hap6 | XI | XI | XI | XI | JX036132 | JX036251 |
Huea sp. | s126 | hap6 | XI | XI | XI | XI | JX036057 | JX036178 |
Caloplaca sp. 1 | s169 | hap3 | XII | XII | XII | XIIa | JX036074 | JX036195 |
Caloplaca sp. 1 | s129 | hap3 | XII | XII | XII | XIIb | JX036060 | JX036181 |
Caloplaca sp. 1 | s130 | hap3 | XII | XII | XII | XIIb | JX036061 | JX036182 |
Caloplaca sp. 1 | s131 | hap3 | XII | XII | XII | XIIb | JX036062 | JX036183 |
Caloplaca sp. 1 | s193 | hap3 | XII | XII | XII | XIIb | JX036098 | JX036219 |
Caloplaca sp. 1 | s194 | hap3 | XII | XII | XII | XIIb | JX036099 | JX036220 |
Caloplaca sp. 1 | s222 | hap16 | XII | XII | XII | XIIb | JX036125 | JX036246 |
Caloplaca sp. 1 | s224 | hap3 | XII | XII | XII | XIIb | JX036127 | JX036247 |
Caloplaca cf. sublobulata | s172 | hap3 | XIII | XIII | XIII | XIII | JX036077 | JX036198 |
Caloplaca cf. sublobulata | s186 | hap3 | XIII | XIII | XIII | XIII | JX036091 | JX036212 |
Caloplaca cf. sublobulata | s163 | hap1 | XIII | XIII | XIII | XIII | JX036068 | JX036189 |
Caloplaca cf. sublobulata | s105 | hap1 | XIII | XIII | XIII | XIII | JX036036 | JX036158 |
Caloplaca cf. sublobulata | s100 | hap1 | XIII | XIII | XIII | XIII | JX036035 | JX036157 |
Caloplaca cf. citrina | s135 | hap3 | XIV | XIV | XIV | XIV | JX036066 | JX036187 |
Caloplaca cf. citrina | s136 | hap3 | XIV | XIV | XIV | XIV | JX036067 | JX036188 |
Umbilicaria aprina | s98 | hap3 | XV | XV | XV | XVa | JX036155 | JX036274 |
Umbilicaria aprina | s99 | hap3 | XV | XV | XV | XVa | JX036156 | JX036275 |
Umbilicaria aprina | s234 | hap3 | XV | XV | XV | XVa | JX036137 | JX036256 |
Umbilicaria aprina | s228 | hap3 | XV | XV | XV | XVa | JX036131 | JX036250 |
Umbilicaria aprina | s227 | hap3 | XV | XV | XV | XVa | JX036130 | JX036249 |
Umbilicaria aprina | s226 | hap1 | XV | XV | XV | XVa | JX036129 | JX036248 |
Umbilicaria aprina | s220 | hap3 | XV | XV | XV | XVa | JX036123 | JX036244 |
Umbilicaria aprina | s219 | hap3 | XV | XV | XV | XVa | JX036122 | JX036243 |
Umbilicaria aprina | s218 | hap3 | XV | XV | XV | XVa | JX036121 | JX036242 |
Umbilicaria aprina | s167 | hap3 | XV | XV | XV | XVa | JX036072 | JX036193 |
Umbilicaria aprina | s134 | hap3 | XV | XV | XV | XVa | JX036065 | JX036186 |
Umbilicaria aprina | s133 | hap3 | XV | XV | XV | XVa | JX036064 | JX036185 |
Umbilicaria aprina | s132 | hap3 | XV | XV | XV | XVa | JX036063 | JX036184 |
Umbilicaria aprina | s110 | hap3 | XV | XV | XV | XVa | JX036041 | JX036163 |
Umbilicaria aprina | s109 | hap3 | XV | XV | XV | XVa | JX036040 | JX036162 |
Umbilicaria aprina | s108 | hap3 | XV | XV | XV | XVa | JX036039 | JX036161 |
Umbilicaria aprina | s170 | hap3 | XV | XV | XV | XVb | JX036075 | JX036196 |
Umbilicaria aprina | s210 | hap3 | XV | XV | XV | XVb | JX036113 | JX036234 |
Umbilicaria aprina | s211 | hap3 | XV | XV | XV | XVb | JX036114 | JX036235 |
Polysporina sp. | s164 | hap3 | XVII | XVI | XVII | XVII | JX036069 | JX036190 |
Polysporina frigida | s188 | hap3 | XVII | XVII | XVII | XVII | JX036093 | JX036214 |
Acarospora cf. nitrophila | s183 | hap10 | XVIII | XVIII | XIX | XVIII | JX036088 | JX036209 |
Sarcogyne privigna | s223 | hap18 | XIX | XIX | XIX | XIX | JX036126 | JX036276 (nuSSU) |
Sarcogyne privigna | s225 | hap18 | XIX | XIX | XIX | XIX | JX036128 | JX036277 (nuSSU) |
Sarcogyne privigna | s185 | hap11 | XIX | XIX | XIX | XIX | JX036090 | JX036211 |
Lecidea polypycnidophora | s120 | hap1 | XX | XX | XX | XX | JX036051 | JX036172 |
Lecidea cancriformis | s113 | hap4 | XXI | XXI | XXI | XXI | JX036044 | JX036166 |
Lecidea cancriformis | s114 | hap5 | XXI | XXI | XXI | XXI | JX036045 | JX036167 |
Unidentified lichen species | s107 | hap2 | XXII | XXII | XXII | XXII | JX036038 | JX036160 |
Buellia sp. | s112 | hap3 | XXIII | XXIII | XXIII | XXIII | JX036043 | JX036165 |
Buellia frigida | s111 | hap3 | XXIV | XXIV | XXIV | XXIV | JX036042 | JX036164 |
Buellia frigida | s117 | hap6 | XXIV | XXIV | XXIV | XXIV | JX036048 | JX036169 |
Buellia frigida | s118 | hap1 | XXIV | XXIV | XXIV | XXIV | JX036049 | JX036170 |
Buellia frigida | s127 | hap7 | XXIV | XXIV | XXIV | XXIV | JX036058 | JX036179 |
Buellia frigida | s165 | hap1 | XXIV | XXIV | XXIV | XXIV | JX036070 | JX036191 |
Buellia frigida | s166 | hap3 | XXIV | XXIV | XXIV | XXIV | JX036071 | JX036192 |
Buellia frigida | s174 | hap1 | XXIV | XXIV | XXIV | XXIV | JX036079 | JX036200 |
Buellia frigida | s176 | hap3 | XXIV | XXIV | XXIV | XXIV | JX036081 | JX036202 |
Buellia frigida | s180 | hap3 | XXIV | XXIV | XXIV | XXIV | JX036085 | JX036206 |
Buellia frigida | s189 | hap1 | XXIV | XXIV | XXIV | XXIV | JX036094 | JX036215 |
Buellia frigida | s195 | hap3 | XXIV | XXIV | XXIV | XXIV | JX036100 | JX036221 |
Buellia frigida | s221 | hap3 | XXIV | XXIV | XXIV | XXIV | JX036124 | JX036245 |
Buellia frigida | s267 | hap3 | XXIV | XXIV | XXIV | XXIV | JX036142 | JX036261 |
Buellia frigida | s187 | hap12 | XXIV | XXIV | XXIV | XXIV | JX036092 | JX036213 |
Buellia frigida | s96 | hap5 | XXIV | XXIV | XXIV | XXIV | JX036153 | JX036272 |
Rhizoplaca macleanii | s213 | hap1 | XXV | XXV | XXV | XXVa | JX036116 | JX036237 |
Rhizoplaca macleanii | s214 | hap1 | XXV | XXV | XXV | XXVa | JX036117 | JX036238 |
Rhizoplaca macleanii | s212 | hap1 | XXV | XXV | XXV | XXVa | JX036115 | JX036236 |
Rhizoplaca macleanii | s209 | hap3 | XXV | XXV | XXV | XXVa | JX036112 | JX036233 |
Rhizoplaca macleanii | s208 | hap1 | XXV | XXV | XXV | XXVa | JX036111 | JX036232 |
Rhizoplaca macleanii | s207 | hap14 | XXV | XXV | XXV | XXVb | JX036110 | JX036231 |
Rhizoplaca macleanii | s206 | hap1 | XXV | XXV | XXV | XXVb | JX036109 | JX036230 |
Rhizoplaca macleanii | s205 | hap9 | XXV | XXV | XXV | XXVb | JX036108 | JX036229 |
Rhizoplaca macleanii | s201 | hap1 | XXV | XXV | XXV | XXVb | JX036105 | JX036226 |
Rhizoplaca macleanii | s232 | hap3 | XXV | XXV | XXV | XXVa | JX036135 | JX036254 |
Rhizoplaca macleanii | s235 | hap1 | XXV | XXV | XXV | XXVa | JX036138 | JX036257 |
Rhizoplaca macleanii | s236 | hap1 | XXV | XXV | XXV | XXVa | JX036139 | JX036258 |
Rhizoplaca macleanii | s237 | hap7 | XXV | XXV | XXV | XXVa | JX036140 | JX036259 |
Rhizoplaca macleanii | s271 | hap1 | XXV | XXV | XXV | XXVb | JX036145 | JX036264 |
Rhizoplaca macleanii | s272 | hap3 | XXV | XXV | XXV | XXVa | JX036146 | JX036265 |
Rhizoplaca macleanii | s273 | hap3 | XXV | XXV | XXV | XXVa | JX036147 | JX036266 |
Rhizoplaca macleanii | s274 | hap3 | XXV | XXV | XXV | XXVa | JX036148 | JX036267 |
Rhizoplaca macleanii | s124 | hap1 | XXV | XXV | XXV | XXVc | JX036055 | JX036176 |
Rhizoplaca macleanii | s125 | hap1 | XXV | XXV | XXV | XXVc | JX036056 | JX036177 |
Rhizoplaca macleanii | s197 | hap3 | XXV | XXV | XXV | XXVc | JX036101 | JX036222 |
Rhizoplaca macleanii | s198 | hap3 | XXV | XXV | XXV | XXVc | JX036102 | JX036223 |
Rhizoplaca macleanii | s233 | hap9 | XXV | XXV | XXV | XXVc | JX036136 | JX036255 |
Rhizoplaca macleanii | s95 | hap9 | XXV | XXV | XXV | XXVc | JX036152 | JX036271 |
Lecanora sp. 2 | s175 | hap3 | XXVI | XXVI | XXVI | XXVI | JX036080 | JX036201 |
Lecanora sp. 2 | s179 | hap3 | XXVI | XXVI | XXVI | XXVI | JX036084 | JX036205 |
Lecanora sp. 2 | s173 | hap3 | XXVI | XXVI | XXVI | XXVI | JX036078 | JX036199 |
Lecanora sp. 2 | s171 | hap3 | XXVI | XXVI | XXVI | XXVI | JX036076 | JX036197 |
Lecanora sp. 2 | s106 | hap1 | XXVI | XXVI | XXVI | XXVI | JX036037 | JX036159 |
Laboratory code | Algal hap | Distance 0.05 | Distance 0.02 | GMYC single | GMYC multiple | Fungal ITS | Algal ITS | |
Carbonea vorticosa | s122 | hap3 | I | I | I | I | JX036053 | JX036174 |
Carbonea vorticosa | s123 | hap3 | I | I | I | I | JX036054 | JX036175 |
Carbonea vorticosa | s121 | hap3 | I | I | I | I | JX036052 | JX036173 |
Carbonea sp. | s217 | hap15 | I | II | II | II | JX036120 | JX036241 |
Austrolecia sp. 1 | s119 | hap3 | III | III | III | III | JX036050 | JX036171 |
Austrolecia sp. 1 | s128 | hap8 | III | III | III | III | JX036059 | JX036180 |
Austrolecia sp. 1 | s168 | hap9 | III | III | III | III | JX036073 | JX036194 |
Austrolecia sp. 1 | s184 | hap1 | III | III | III | III | JX036089 | JX036210 |
Austrolecia sp. 1 | s199 | hap1 | III | III | III | III | JX036103 | JX036224 |
Austrolecia sp. 1 | s200 | hap1 | III | III | III | III | JX036104 | JX036225 |
Austrolecia sp. 1 | s231 | hap6 | III | III | III | III | JX036134 | JX036253 |
Austrolecia sp. 1 | s182 | hap10 | III | III | III | III | JX036087 | JX036208 |
Austrolecia sp. 1 | s97 | hap17 | III | III | III | III | JX036154 | JX036273 |
Austrolecia sp. 2 | s177 | hap3 | III | IV | IV | IV | JX036082 | JX036203 |
Austrolecia sp. 2 | s178 | hap3 | III | IV | IV | IV | JX036083 | JX036204 |
Austrolecia sp. 3 | s116 | – | III | V | V | V | JX036047 | – |
Lecanora sp. 1 | s275 | hap6 | VI | VI | VI | VI | JX036149 | JX036268 |
Lecanora cf. flotowiana | s190 | hap1 | VII | VII | VII | VII | JX036095 | JX036216 |
Lecanora cf. flotowiana | s191 | hap3 | VII | VII | VII | VII | JX036096 | JX036217 |
Lecanora cf. mons-nivis | s202 | hap13 | VII | VIII | VII | VIII | JX036106 | JX036227 |
Lecidella greenii | s300 | hap3 | IX | IX | IX | IX | JX036150 | JX036269 |
Lecidella greenii | s301 | hap3 | IX | IX | IX | IX | JX036151 | JX036270 |
Lecidella greenii | s266 | hap9 | IX | IX | IX | IX | JX036141 | JX036260 |
Lecidella greenii | s230 | hap3 | IX | IX | IX | IX | JX036133 | JX036252 |
Lecidella greenii | s203 | hap3 | IX | IX | IX | IX | JX036107 | JX036228 |
Lecidella greenii | s192 | hap3 | IX | IX | IX | IX | JX036097 | JX036218 |
Lecidella greenii | s181 | hap9 | IX | IX | IX | IX | JX036086 | JX036207 |
Lecidella greenii | s115 | hap6 | IX | IX | IX | IX | JX036046 | JX036168 |
Rhizoplaca sp. | s215 | hap1 | X | X | X | X | JX036118 | JX036239 |
Rhizoplaca sp. | s216 | hap15 | X | X | X | X | JX036119 | JX036240 |
Huea sp. | s269 | hap3 | XI | XI | XI | XI | JX036143 | JX036262 |
Huea sp. | s270 | hap3 | XI | XI | XI | XI | JX036144 | JX036263 |
Huea sp. | s229 | hap6 | XI | XI | XI | XI | JX036132 | JX036251 |
Huea sp. | s126 | hap6 | XI | XI | XI | XI | JX036057 | JX036178 |
Caloplaca sp. 1 | s169 | hap3 | XII | XII | XII | XIIa | JX036074 | JX036195 |
Caloplaca sp. 1 | s129 | hap3 | XII | XII | XII | XIIb | JX036060 | JX036181 |
Caloplaca sp. 1 | s130 | hap3 | XII | XII | XII | XIIb | JX036061 | JX036182 |
Caloplaca sp. 1 | s131 | hap3 | XII | XII | XII | XIIb | JX036062 | JX036183 |
Caloplaca sp. 1 | s193 | hap3 | XII | XII | XII | XIIb | JX036098 | JX036219 |
Caloplaca sp. 1 | s194 | hap3 | XII | XII | XII | XIIb | JX036099 | JX036220 |
Caloplaca sp. 1 | s222 | hap16 | XII | XII | XII | XIIb | JX036125 | JX036246 |
Caloplaca sp. 1 | s224 | hap3 | XII | XII | XII | XIIb | JX036127 | JX036247 |
Caloplaca cf. sublobulata | s172 | hap3 | XIII | XIII | XIII | XIII | JX036077 | JX036198 |
Caloplaca cf. sublobulata | s186 | hap3 | XIII | XIII | XIII | XIII | JX036091 | JX036212 |
Caloplaca cf. sublobulata | s163 | hap1 | XIII | XIII | XIII | XIII | JX036068 | JX036189 |
Caloplaca cf. sublobulata | s105 | hap1 | XIII | XIII | XIII | XIII | JX036036 | JX036158 |
Caloplaca cf. sublobulata | s100 | hap1 | XIII | XIII | XIII | XIII | JX036035 | JX036157 |
Caloplaca cf. citrina | s135 | hap3 | XIV | XIV | XIV | XIV | JX036066 | JX036187 |
Caloplaca cf. citrina | s136 | hap3 | XIV | XIV | XIV | XIV | JX036067 | JX036188 |
Umbilicaria aprina | s98 | hap3 | XV | XV | XV | XVa | JX036155 | JX036274 |
Umbilicaria aprina | s99 | hap3 | XV | XV | XV | XVa | JX036156 | JX036275 |
Umbilicaria aprina | s234 | hap3 | XV | XV | XV | XVa | JX036137 | JX036256 |
Umbilicaria aprina | s228 | hap3 | XV | XV | XV | XVa | JX036131 | JX036250 |
Umbilicaria aprina | s227 | hap3 | XV | XV | XV | XVa | JX036130 | JX036249 |
Umbilicaria aprina | s226 | hap1 | XV | XV | XV | XVa | JX036129 | JX036248 |
Umbilicaria aprina | s220 | hap3 | XV | XV | XV | XVa | JX036123 | JX036244 |
Umbilicaria aprina | s219 | hap3 | XV | XV | XV | XVa | JX036122 | JX036243 |
Umbilicaria aprina | s218 | hap3 | XV | XV | XV | XVa | JX036121 | JX036242 |
Umbilicaria aprina | s167 | hap3 | XV | XV | XV | XVa | JX036072 | JX036193 |
Umbilicaria aprina | s134 | hap3 | XV | XV | XV | XVa | JX036065 | JX036186 |
Umbilicaria aprina | s133 | hap3 | XV | XV | XV | XVa | JX036064 | JX036185 |
Umbilicaria aprina | s132 | hap3 | XV | XV | XV | XVa | JX036063 | JX036184 |
Umbilicaria aprina | s110 | hap3 | XV | XV | XV | XVa | JX036041 | JX036163 |
Umbilicaria aprina | s109 | hap3 | XV | XV | XV | XVa | JX036040 | JX036162 |
Umbilicaria aprina | s108 | hap3 | XV | XV | XV | XVa | JX036039 | JX036161 |
Umbilicaria aprina | s170 | hap3 | XV | XV | XV | XVb | JX036075 | JX036196 |
Umbilicaria aprina | s210 | hap3 | XV | XV | XV | XVb | JX036113 | JX036234 |
Umbilicaria aprina | s211 | hap3 | XV | XV | XV | XVb | JX036114 | JX036235 |
Polysporina sp. | s164 | hap3 | XVII | XVI | XVII | XVII | JX036069 | JX036190 |
Polysporina frigida | s188 | hap3 | XVII | XVII | XVII | XVII | JX036093 | JX036214 |
Acarospora cf. nitrophila | s183 | hap10 | XVIII | XVIII | XIX | XVIII | JX036088 | JX036209 |
Sarcogyne privigna | s223 | hap18 | XIX | XIX | XIX | XIX | JX036126 | JX036276 (nuSSU) |
Sarcogyne privigna | s225 | hap18 | XIX | XIX | XIX | XIX | JX036128 | JX036277 (nuSSU) |
Sarcogyne privigna | s185 | hap11 | XIX | XIX | XIX | XIX | JX036090 | JX036211 |
Lecidea polypycnidophora | s120 | hap1 | XX | XX | XX | XX | JX036051 | JX036172 |
Lecidea cancriformis | s113 | hap4 | XXI | XXI | XXI | XXI | JX036044 | JX036166 |
Lecidea cancriformis | s114 | hap5 | XXI | XXI | XXI | XXI | JX036045 | JX036167 |
Unidentified lichen species | s107 | hap2 | XXII | XXII | XXII | XXII | JX036038 | JX036160 |
Buellia sp. | s112 | hap3 | XXIII | XXIII | XXIII | XXIII | JX036043 | JX036165 |
Buellia frigida | s111 | hap3 | XXIV | XXIV | XXIV | XXIV | JX036042 | JX036164 |
Buellia frigida | s117 | hap6 | XXIV | XXIV | XXIV | XXIV | JX036048 | JX036169 |
Buellia frigida | s118 | hap1 | XXIV | XXIV | XXIV | XXIV | JX036049 | JX036170 |
Buellia frigida | s127 | hap7 | XXIV | XXIV | XXIV | XXIV | JX036058 | JX036179 |
Buellia frigida | s165 | hap1 | XXIV | XXIV | XXIV | XXIV | JX036070 | JX036191 |
Buellia frigida | s166 | hap3 | XXIV | XXIV | XXIV | XXIV | JX036071 | JX036192 |
Buellia frigida | s174 | hap1 | XXIV | XXIV | XXIV | XXIV | JX036079 | JX036200 |
Buellia frigida | s176 | hap3 | XXIV | XXIV | XXIV | XXIV | JX036081 | JX036202 |
Buellia frigida | s180 | hap3 | XXIV | XXIV | XXIV | XXIV | JX036085 | JX036206 |
Buellia frigida | s189 | hap1 | XXIV | XXIV | XXIV | XXIV | JX036094 | JX036215 |
Buellia frigida | s195 | hap3 | XXIV | XXIV | XXIV | XXIV | JX036100 | JX036221 |
Buellia frigida | s221 | hap3 | XXIV | XXIV | XXIV | XXIV | JX036124 | JX036245 |
Buellia frigida | s267 | hap3 | XXIV | XXIV | XXIV | XXIV | JX036142 | JX036261 |
Buellia frigida | s187 | hap12 | XXIV | XXIV | XXIV | XXIV | JX036092 | JX036213 |
Buellia frigida | s96 | hap5 | XXIV | XXIV | XXIV | XXIV | JX036153 | JX036272 |
Rhizoplaca macleanii | s213 | hap1 | XXV | XXV | XXV | XXVa | JX036116 | JX036237 |
Rhizoplaca macleanii | s214 | hap1 | XXV | XXV | XXV | XXVa | JX036117 | JX036238 |
Rhizoplaca macleanii | s212 | hap1 | XXV | XXV | XXV | XXVa | JX036115 | JX036236 |
Rhizoplaca macleanii | s209 | hap3 | XXV | XXV | XXV | XXVa | JX036112 | JX036233 |
Rhizoplaca macleanii | s208 | hap1 | XXV | XXV | XXV | XXVa | JX036111 | JX036232 |
Rhizoplaca macleanii | s207 | hap14 | XXV | XXV | XXV | XXVb | JX036110 | JX036231 |
Rhizoplaca macleanii | s206 | hap1 | XXV | XXV | XXV | XXVb | JX036109 | JX036230 |
Rhizoplaca macleanii | s205 | hap9 | XXV | XXV | XXV | XXVb | JX036108 | JX036229 |
Rhizoplaca macleanii | s201 | hap1 | XXV | XXV | XXV | XXVb | JX036105 | JX036226 |
Rhizoplaca macleanii | s232 | hap3 | XXV | XXV | XXV | XXVa | JX036135 | JX036254 |
Rhizoplaca macleanii | s235 | hap1 | XXV | XXV | XXV | XXVa | JX036138 | JX036257 |
Rhizoplaca macleanii | s236 | hap1 | XXV | XXV | XXV | XXVa | JX036139 | JX036258 |
Rhizoplaca macleanii | s237 | hap7 | XXV | XXV | XXV | XXVa | JX036140 | JX036259 |
Rhizoplaca macleanii | s271 | hap1 | XXV | XXV | XXV | XXVb | JX036145 | JX036264 |
Rhizoplaca macleanii | s272 | hap3 | XXV | XXV | XXV | XXVa | JX036146 | JX036265 |
Rhizoplaca macleanii | s273 | hap3 | XXV | XXV | XXV | XXVa | JX036147 | JX036266 |
Rhizoplaca macleanii | s274 | hap3 | XXV | XXV | XXV | XXVa | JX036148 | JX036267 |
Rhizoplaca macleanii | s124 | hap1 | XXV | XXV | XXV | XXVc | JX036055 | JX036176 |
Rhizoplaca macleanii | s125 | hap1 | XXV | XXV | XXV | XXVc | JX036056 | JX036177 |
Rhizoplaca macleanii | s197 | hap3 | XXV | XXV | XXV | XXVc | JX036101 | JX036222 |
Rhizoplaca macleanii | s198 | hap3 | XXV | XXV | XXV | XXVc | JX036102 | JX036223 |
Rhizoplaca macleanii | s233 | hap9 | XXV | XXV | XXV | XXVc | JX036136 | JX036255 |
Rhizoplaca macleanii | s95 | hap9 | XXV | XXV | XXV | XXVc | JX036152 | JX036271 |
Lecanora sp. 2 | s175 | hap3 | XXVI | XXVI | XXVI | XXVI | JX036080 | JX036201 |
Lecanora sp. 2 | s179 | hap3 | XXVI | XXVI | XXVI | XXVI | JX036084 | JX036205 |
Lecanora sp. 2 | s173 | hap3 | XXVI | XXVI | XXVI | XXVI | JX036078 | JX036199 |
Lecanora sp. 2 | s171 | hap3 | XXVI | XXVI | XXVI | XXVI | JX036076 | JX036197 |
Lecanora sp. 2 | s106 | hap1 | XXVI | XXVI | XXVI | XXVI | JX036037 | JX036159 |
Samples used in this study with their corresponding laboratory code, the photobiont haplotype found associated in that sample, their affiliation to the groups obtained by the different DNA-based species delimitation methods, and the accession numbers for the ITS mycobiont and photobiont sequences
Laboratory code | Algal hap | Distance 0.05 | Distance 0.02 | GMYC single | GMYC multiple | Fungal ITS | Algal ITS | |
Carbonea vorticosa | s122 | hap3 | I | I | I | I | JX036053 | JX036174 |
Carbonea vorticosa | s123 | hap3 | I | I | I | I | JX036054 | JX036175 |
Carbonea vorticosa | s121 | hap3 | I | I | I | I | JX036052 | JX036173 |
Carbonea sp. | s217 | hap15 | I | II | II | II | JX036120 | JX036241 |
Austrolecia sp. 1 | s119 | hap3 | III | III | III | III | JX036050 | JX036171 |
Austrolecia sp. 1 | s128 | hap8 | III | III | III | III | JX036059 | JX036180 |
Austrolecia sp. 1 | s168 | hap9 | III | III | III | III | JX036073 | JX036194 |
Austrolecia sp. 1 | s184 | hap1 | III | III | III | III | JX036089 | JX036210 |
Austrolecia sp. 1 | s199 | hap1 | III | III | III | III | JX036103 | JX036224 |
Austrolecia sp. 1 | s200 | hap1 | III | III | III | III | JX036104 | JX036225 |
Austrolecia sp. 1 | s231 | hap6 | III | III | III | III | JX036134 | JX036253 |
Austrolecia sp. 1 | s182 | hap10 | III | III | III | III | JX036087 | JX036208 |
Austrolecia sp. 1 | s97 | hap17 | III | III | III | III | JX036154 | JX036273 |
Austrolecia sp. 2 | s177 | hap3 | III | IV | IV | IV | JX036082 | JX036203 |
Austrolecia sp. 2 | s178 | hap3 | III | IV | IV | IV | JX036083 | JX036204 |
Austrolecia sp. 3 | s116 | – | III | V | V | V | JX036047 | – |
Lecanora sp. 1 | s275 | hap6 | VI | VI | VI | VI | JX036149 | JX036268 |
Lecanora cf. flotowiana | s190 | hap1 | VII | VII | VII | VII | JX036095 | JX036216 |
Lecanora cf. flotowiana | s191 | hap3 | VII | VII | VII | VII | JX036096 | JX036217 |
Lecanora cf. mons-nivis | s202 | hap13 | VII | VIII | VII | VIII | JX036106 | JX036227 |
Lecidella greenii | s300 | hap3 | IX | IX | IX | IX | JX036150 | JX036269 |
Lecidella greenii | s301 | hap3 | IX | IX | IX | IX | JX036151 | JX036270 |
Lecidella greenii | s266 | hap9 | IX | IX | IX | IX | JX036141 | JX036260 |
Lecidella greenii | s230 | hap3 | IX | IX | IX | IX | JX036133 | JX036252 |
Lecidella greenii | s203 | hap3 | IX | IX | IX | IX | JX036107 | JX036228 |
Lecidella greenii | s192 | hap3 | IX | IX | IX | IX | JX036097 | JX036218 |
Lecidella greenii | s181 | hap9 | IX | IX | IX | IX | JX036086 | JX036207 |
Lecidella greenii | s115 | hap6 | IX | IX | IX | IX | JX036046 | JX036168 |
Rhizoplaca sp. | s215 | hap1 | X | X | X | X | JX036118 | JX036239 |
Rhizoplaca sp. | s216 | hap15 | X | X | X | X | JX036119 | JX036240 |
Huea sp. | s269 | hap3 | XI | XI | XI | XI | JX036143 | JX036262 |
Huea sp. | s270 | hap3 | XI | XI | XI | XI | JX036144 | JX036263 |
Huea sp. | s229 | hap6 | XI | XI | XI | XI | JX036132 | JX036251 |
Huea sp. | s126 | hap6 | XI | XI | XI | XI | JX036057 | JX036178 |
Caloplaca sp. 1 | s169 | hap3 | XII | XII | XII | XIIa | JX036074 | JX036195 |
Caloplaca sp. 1 | s129 | hap3 | XII | XII | XII | XIIb | JX036060 | JX036181 |
Caloplaca sp. 1 | s130 | hap3 | XII | XII | XII | XIIb | JX036061 | JX036182 |
Caloplaca sp. 1 | s131 | hap3 | XII | XII | XII | XIIb | JX036062 | JX036183 |
Caloplaca sp. 1 | s193 | hap3 | XII | XII | XII | XIIb | JX036098 | JX036219 |
Caloplaca sp. 1 | s194 | hap3 | XII | XII | XII | XIIb | JX036099 | JX036220 |
Caloplaca sp. 1 | s222 | hap16 | XII | XII | XII | XIIb | JX036125 | JX036246 |
Caloplaca sp. 1 | s224 | hap3 | XII | XII | XII | XIIb | JX036127 | JX036247 |
Caloplaca cf. sublobulata | s172 | hap3 | XIII | XIII | XIII | XIII | JX036077 | JX036198 |
Caloplaca cf. sublobulata | s186 | hap3 | XIII | XIII | XIII | XIII | JX036091 | JX036212 |
Caloplaca cf. sublobulata | s163 | hap1 | XIII | XIII | XIII | XIII | JX036068 | JX036189 |
Caloplaca cf. sublobulata | s105 | hap1 | XIII | XIII | XIII | XIII | JX036036 | JX036158 |
Caloplaca cf. sublobulata | s100 | hap1 | XIII | XIII | XIII | XIII | JX036035 | JX036157 |
Caloplaca cf. citrina | s135 | hap3 | XIV | XIV | XIV | XIV | JX036066 | JX036187 |
Caloplaca cf. citrina | s136 | hap3 | XIV | XIV | XIV | XIV | JX036067 | JX036188 |
Umbilicaria aprina | s98 | hap3 | XV | XV | XV | XVa | JX036155 | JX036274 |
Umbilicaria aprina | s99 | hap3 | XV | XV | XV | XVa | JX036156 | JX036275 |
Umbilicaria aprina | s234 | hap3 | XV | XV | XV | XVa | JX036137 | JX036256 |
Umbilicaria aprina | s228 | hap3 | XV | XV | XV | XVa | JX036131 | JX036250 |
Umbilicaria aprina | s227 | hap3 | XV | XV | XV | XVa | JX036130 | JX036249 |
Umbilicaria aprina | s226 | hap1 | XV | XV | XV | XVa | JX036129 | JX036248 |
Umbilicaria aprina | s220 | hap3 | XV | XV | XV | XVa | JX036123 | JX036244 |
Umbilicaria aprina | s219 | hap3 | XV | XV | XV | XVa | JX036122 | JX036243 |
Umbilicaria aprina | s218 | hap3 | XV | XV | XV | XVa | JX036121 | JX036242 |
Umbilicaria aprina | s167 | hap3 | XV | XV | XV | XVa | JX036072 | JX036193 |
Umbilicaria aprina | s134 | hap3 | XV | XV | XV | XVa | JX036065 | JX036186 |
Umbilicaria aprina | s133 | hap3 | XV | XV | XV | XVa | JX036064 | JX036185 |
Umbilicaria aprina | s132 | hap3 | XV | XV | XV | XVa | JX036063 | JX036184 |
Umbilicaria aprina | s110 | hap3 | XV | XV | XV | XVa | JX036041 | JX036163 |
Umbilicaria aprina | s109 | hap3 | XV | XV | XV | XVa | JX036040 | JX036162 |
Umbilicaria aprina | s108 | hap3 | XV | XV | XV | XVa | JX036039 | JX036161 |
Umbilicaria aprina | s170 | hap3 | XV | XV | XV | XVb | JX036075 | JX036196 |
Umbilicaria aprina | s210 | hap3 | XV | XV | XV | XVb | JX036113 | JX036234 |
Umbilicaria aprina | s211 | hap3 | XV | XV | XV | XVb | JX036114 | JX036235 |
Polysporina sp. | s164 | hap3 | XVII | XVI | XVII | XVII | JX036069 | JX036190 |
Polysporina frigida | s188 | hap3 | XVII | XVII | XVII | XVII | JX036093 | JX036214 |
Acarospora cf. nitrophila | s183 | hap10 | XVIII | XVIII | XIX | XVIII | JX036088 | JX036209 |
Sarcogyne privigna | s223 | hap18 | XIX | XIX | XIX | XIX | JX036126 | JX036276 (nuSSU) |
Sarcogyne privigna | s225 | hap18 | XIX | XIX | XIX | XIX | JX036128 | JX036277 (nuSSU) |
Sarcogyne privigna | s185 | hap11 | XIX | XIX | XIX | XIX | JX036090 | JX036211 |
Lecidea polypycnidophora | s120 | hap1 | XX | XX | XX | XX | JX036051 | JX036172 |
Lecidea cancriformis | s113 | hap4 | XXI | XXI | XXI | XXI | JX036044 | JX036166 |
Lecidea cancriformis | s114 | hap5 | XXI | XXI | XXI | XXI | JX036045 | JX036167 |
Unidentified lichen species | s107 | hap2 | XXII | XXII | XXII | XXII | JX036038 | JX036160 |
Buellia sp. | s112 | hap3 | XXIII | XXIII | XXIII | XXIII | JX036043 | JX036165 |
Buellia frigida | s111 | hap3 | XXIV | XXIV | XXIV | XXIV | JX036042 | JX036164 |
Buellia frigida | s117 | hap6 | XXIV | XXIV | XXIV | XXIV | JX036048 | JX036169 |
Buellia frigida | s118 | hap1 | XXIV | XXIV | XXIV | XXIV | JX036049 | JX036170 |
Buellia frigida | s127 | hap7 | XXIV | XXIV | XXIV | XXIV | JX036058 | JX036179 |
Buellia frigida | s165 | hap1 | XXIV | XXIV | XXIV | XXIV | JX036070 | JX036191 |
Buellia frigida | s166 | hap3 | XXIV | XXIV | XXIV | XXIV | JX036071 | JX036192 |
Buellia frigida | s174 | hap1 | XXIV | XXIV | XXIV | XXIV | JX036079 | JX036200 |
Buellia frigida | s176 | hap3 | XXIV | XXIV | XXIV | XXIV | JX036081 | JX036202 |
Buellia frigida | s180 | hap3 | XXIV | XXIV | XXIV | XXIV | JX036085 | JX036206 |
Buellia frigida | s189 | hap1 | XXIV | XXIV | XXIV | XXIV | JX036094 | JX036215 |
Buellia frigida | s195 | hap3 | XXIV | XXIV | XXIV | XXIV | JX036100 | JX036221 |
Buellia frigida | s221 | hap3 | XXIV | XXIV | XXIV | XXIV | JX036124 | JX036245 |
Buellia frigida | s267 | hap3 | XXIV | XXIV | XXIV | XXIV | JX036142 | JX036261 |
Buellia frigida | s187 | hap12 | XXIV | XXIV | XXIV | XXIV | JX036092 | JX036213 |
Buellia frigida | s96 | hap5 | XXIV | XXIV | XXIV | XXIV | JX036153 | JX036272 |
Rhizoplaca macleanii | s213 | hap1 | XXV | XXV | XXV | XXVa | JX036116 | JX036237 |
Rhizoplaca macleanii | s214 | hap1 | XXV | XXV | XXV | XXVa | JX036117 | JX036238 |
Rhizoplaca macleanii | s212 | hap1 | XXV | XXV | XXV | XXVa | JX036115 | JX036236 |
Rhizoplaca macleanii | s209 | hap3 | XXV | XXV | XXV | XXVa | JX036112 | JX036233 |
Rhizoplaca macleanii | s208 | hap1 | XXV | XXV | XXV | XXVa | JX036111 | JX036232 |
Rhizoplaca macleanii | s207 | hap14 | XXV | XXV | XXV | XXVb | JX036110 | JX036231 |
Rhizoplaca macleanii | s206 | hap1 | XXV | XXV | XXV | XXVb | JX036109 | JX036230 |
Rhizoplaca macleanii | s205 | hap9 | XXV | XXV | XXV | XXVb | JX036108 | JX036229 |
Rhizoplaca macleanii | s201 | hap1 | XXV | XXV | XXV | XXVb | JX036105 | JX036226 |
Rhizoplaca macleanii | s232 | hap3 | XXV | XXV | XXV | XXVa | JX036135 | JX036254 |
Rhizoplaca macleanii | s235 | hap1 | XXV | XXV | XXV | XXVa | JX036138 | JX036257 |
Rhizoplaca macleanii | s236 | hap1 | XXV | XXV | XXV | XXVa | JX036139 | JX036258 |
Rhizoplaca macleanii | s237 | hap7 | XXV | XXV | XXV | XXVa | JX036140 | JX036259 |
Rhizoplaca macleanii | s271 | hap1 | XXV | XXV | XXV | XXVb | JX036145 | JX036264 |
Rhizoplaca macleanii | s272 | hap3 | XXV | XXV | XXV | XXVa | JX036146 | JX036265 |
Rhizoplaca macleanii | s273 | hap3 | XXV | XXV | XXV | XXVa | JX036147 | JX036266 |
Rhizoplaca macleanii | s274 | hap3 | XXV | XXV | XXV | XXVa | JX036148 | JX036267 |
Rhizoplaca macleanii | s124 | hap1 | XXV | XXV | XXV | XXVc | JX036055 | JX036176 |
Rhizoplaca macleanii | s125 | hap1 | XXV | XXV | XXV | XXVc | JX036056 | JX036177 |
Rhizoplaca macleanii | s197 | hap3 | XXV | XXV | XXV | XXVc | JX036101 | JX036222 |
Rhizoplaca macleanii | s198 | hap3 | XXV | XXV | XXV | XXVc | JX036102 | JX036223 |
Rhizoplaca macleanii | s233 | hap9 | XXV | XXV | XXV | XXVc | JX036136 | JX036255 |
Rhizoplaca macleanii | s95 | hap9 | XXV | XXV | XXV | XXVc | JX036152 | JX036271 |
Lecanora sp. 2 | s175 | hap3 | XXVI | XXVI | XXVI | XXVI | JX036080 | JX036201 |
Lecanora sp. 2 | s179 | hap3 | XXVI | XXVI | XXVI | XXVI | JX036084 | JX036205 |
Lecanora sp. 2 | s173 | hap3 | XXVI | XXVI | XXVI | XXVI | JX036078 | JX036199 |
Lecanora sp. 2 | s171 | hap3 | XXVI | XXVI | XXVI | XXVI | JX036076 | JX036197 |
Lecanora sp. 2 | s106 | hap1 | XXVI | XXVI | XXVI | XXVI | JX036037 | JX036159 |
Laboratory code | Algal hap | Distance 0.05 | Distance 0.02 | GMYC single | GMYC multiple | Fungal ITS | Algal ITS | |
Carbonea vorticosa | s122 | hap3 | I | I | I | I | JX036053 | JX036174 |
Carbonea vorticosa | s123 | hap3 | I | I | I | I | JX036054 | JX036175 |
Carbonea vorticosa | s121 | hap3 | I | I | I | I | JX036052 | JX036173 |
Carbonea sp. | s217 | hap15 | I | II | II | II | JX036120 | JX036241 |
Austrolecia sp. 1 | s119 | hap3 | III | III | III | III | JX036050 | JX036171 |
Austrolecia sp. 1 | s128 | hap8 | III | III | III | III | JX036059 | JX036180 |
Austrolecia sp. 1 | s168 | hap9 | III | III | III | III | JX036073 | JX036194 |
Austrolecia sp. 1 | s184 | hap1 | III | III | III | III | JX036089 | JX036210 |
Austrolecia sp. 1 | s199 | hap1 | III | III | III | III | JX036103 | JX036224 |
Austrolecia sp. 1 | s200 | hap1 | III | III | III | III | JX036104 | JX036225 |
Austrolecia sp. 1 | s231 | hap6 | III | III | III | III | JX036134 | JX036253 |
Austrolecia sp. 1 | s182 | hap10 | III | III | III | III | JX036087 | JX036208 |
Austrolecia sp. 1 | s97 | hap17 | III | III | III | III | JX036154 | JX036273 |
Austrolecia sp. 2 | s177 | hap3 | III | IV | IV | IV | JX036082 | JX036203 |
Austrolecia sp. 2 | s178 | hap3 | III | IV | IV | IV | JX036083 | JX036204 |
Austrolecia sp. 3 | s116 | – | III | V | V | V | JX036047 | – |
Lecanora sp. 1 | s275 | hap6 | VI | VI | VI | VI | JX036149 | JX036268 |
Lecanora cf. flotowiana | s190 | hap1 | VII | VII | VII | VII | JX036095 | JX036216 |
Lecanora cf. flotowiana | s191 | hap3 | VII | VII | VII | VII | JX036096 | JX036217 |
Lecanora cf. mons-nivis | s202 | hap13 | VII | VIII | VII | VIII | JX036106 | JX036227 |
Lecidella greenii | s300 | hap3 | IX | IX | IX | IX | JX036150 | JX036269 |
Lecidella greenii | s301 | hap3 | IX | IX | IX | IX | JX036151 | JX036270 |
Lecidella greenii | s266 | hap9 | IX | IX | IX | IX | JX036141 | JX036260 |
Lecidella greenii | s230 | hap3 | IX | IX | IX | IX | JX036133 | JX036252 |
Lecidella greenii | s203 | hap3 | IX | IX | IX | IX | JX036107 | JX036228 |
Lecidella greenii | s192 | hap3 | IX | IX | IX | IX | JX036097 | JX036218 |
Lecidella greenii | s181 | hap9 | IX | IX | IX | IX | JX036086 | JX036207 |
Lecidella greenii | s115 | hap6 | IX | IX | IX | IX | JX036046 | JX036168 |
Rhizoplaca sp. | s215 | hap1 | X | X | X | X | JX036118 | JX036239 |
Rhizoplaca sp. | s216 | hap15 | X | X | X | X | JX036119 | JX036240 |
Huea sp. | s269 | hap3 | XI | XI | XI | XI | JX036143 | JX036262 |
Huea sp. | s270 | hap3 | XI | XI | XI | XI | JX036144 | JX036263 |
Huea sp. | s229 | hap6 | XI | XI | XI | XI | JX036132 | JX036251 |
Huea sp. | s126 | hap6 | XI | XI | XI | XI | JX036057 | JX036178 |
Caloplaca sp. 1 | s169 | hap3 | XII | XII | XII | XIIa | JX036074 | JX036195 |
Caloplaca sp. 1 | s129 | hap3 | XII | XII | XII | XIIb | JX036060 | JX036181 |
Caloplaca sp. 1 | s130 | hap3 | XII | XII | XII | XIIb | JX036061 | JX036182 |
Caloplaca sp. 1 | s131 | hap3 | XII | XII | XII | XIIb | JX036062 | JX036183 |
Caloplaca sp. 1 | s193 | hap3 | XII | XII | XII | XIIb | JX036098 | JX036219 |
Caloplaca sp. 1 | s194 | hap3 | XII | XII | XII | XIIb | JX036099 | JX036220 |
Caloplaca sp. 1 | s222 | hap16 | XII | XII | XII | XIIb | JX036125 | JX036246 |
Caloplaca sp. 1 | s224 | hap3 | XII | XII | XII | XIIb | JX036127 | JX036247 |
Caloplaca cf. sublobulata | s172 | hap3 | XIII | XIII | XIII | XIII | JX036077 | JX036198 |
Caloplaca cf. sublobulata | s186 | hap3 | XIII | XIII | XIII | XIII | JX036091 | JX036212 |
Caloplaca cf. sublobulata | s163 | hap1 | XIII | XIII | XIII | XIII | JX036068 | JX036189 |
Caloplaca cf. sublobulata | s105 | hap1 | XIII | XIII | XIII | XIII | JX036036 | JX036158 |
Caloplaca cf. sublobulata | s100 | hap1 | XIII | XIII | XIII | XIII | JX036035 | JX036157 |
Caloplaca cf. citrina | s135 | hap3 | XIV | XIV | XIV | XIV | JX036066 | JX036187 |
Caloplaca cf. citrina | s136 | hap3 | XIV | XIV | XIV | XIV | JX036067 | JX036188 |
Umbilicaria aprina | s98 | hap3 | XV | XV | XV | XVa | JX036155 | JX036274 |
Umbilicaria aprina | s99 | hap3 | XV | XV | XV | XVa | JX036156 | JX036275 |
Umbilicaria aprina | s234 | hap3 | XV | XV | XV | XVa | JX036137 | JX036256 |
Umbilicaria aprina | s228 | hap3 | XV | XV | XV | XVa | JX036131 | JX036250 |
Umbilicaria aprina | s227 | hap3 | XV | XV | XV | XVa | JX036130 | JX036249 |
Umbilicaria aprina | s226 | hap1 | XV | XV | XV | XVa | JX036129 | JX036248 |
Umbilicaria aprina | s220 | hap3 | XV | XV | XV | XVa | JX036123 | JX036244 |
Umbilicaria aprina | s219 | hap3 | XV | XV | XV | XVa | JX036122 | JX036243 |
Umbilicaria aprina | s218 | hap3 | XV | XV | XV | XVa | JX036121 | JX036242 |
Umbilicaria aprina | s167 | hap3 | XV | XV | XV | XVa | JX036072 | JX036193 |
Umbilicaria aprina | s134 | hap3 | XV | XV | XV | XVa | JX036065 | JX036186 |
Umbilicaria aprina | s133 | hap3 | XV | XV | XV | XVa | JX036064 | JX036185 |
Umbilicaria aprina | s132 | hap3 | XV | XV | XV | XVa | JX036063 | JX036184 |
Umbilicaria aprina | s110 | hap3 | XV | XV | XV | XVa | JX036041 | JX036163 |
Umbilicaria aprina | s109 | hap3 | XV | XV | XV | XVa | JX036040 | JX036162 |
Umbilicaria aprina | s108 | hap3 | XV | XV | XV | XVa | JX036039 | JX036161 |
Umbilicaria aprina | s170 | hap3 | XV | XV | XV | XVb | JX036075 | JX036196 |
Umbilicaria aprina | s210 | hap3 | XV | XV | XV | XVb | JX036113 | JX036234 |
Umbilicaria aprina | s211 | hap3 | XV | XV | XV | XVb | JX036114 | JX036235 |
Polysporina sp. | s164 | hap3 | XVII | XVI | XVII | XVII | JX036069 | JX036190 |
Polysporina frigida | s188 | hap3 | XVII | XVII | XVII | XVII | JX036093 | JX036214 |
Acarospora cf. nitrophila | s183 | hap10 | XVIII | XVIII | XIX | XVIII | JX036088 | JX036209 |
Sarcogyne privigna | s223 | hap18 | XIX | XIX | XIX | XIX | JX036126 | JX036276 (nuSSU) |
Sarcogyne privigna | s225 | hap18 | XIX | XIX | XIX | XIX | JX036128 | JX036277 (nuSSU) |
Sarcogyne privigna | s185 | hap11 | XIX | XIX | XIX | XIX | JX036090 | JX036211 |
Lecidea polypycnidophora | s120 | hap1 | XX | XX | XX | XX | JX036051 | JX036172 |
Lecidea cancriformis | s113 | hap4 | XXI | XXI | XXI | XXI | JX036044 | JX036166 |
Lecidea cancriformis | s114 | hap5 | XXI | XXI | XXI | XXI | JX036045 | JX036167 |
Unidentified lichen species | s107 | hap2 | XXII | XXII | XXII | XXII | JX036038 | JX036160 |
Buellia sp. | s112 | hap3 | XXIII | XXIII | XXIII | XXIII | JX036043 | JX036165 |
Buellia frigida | s111 | hap3 | XXIV | XXIV | XXIV | XXIV | JX036042 | JX036164 |
Buellia frigida | s117 | hap6 | XXIV | XXIV | XXIV | XXIV | JX036048 | JX036169 |
Buellia frigida | s118 | hap1 | XXIV | XXIV | XXIV | XXIV | JX036049 | JX036170 |
Buellia frigida | s127 | hap7 | XXIV | XXIV | XXIV | XXIV | JX036058 | JX036179 |
Buellia frigida | s165 | hap1 | XXIV | XXIV | XXIV | XXIV | JX036070 | JX036191 |
Buellia frigida | s166 | hap3 | XXIV | XXIV | XXIV | XXIV | JX036071 | JX036192 |
Buellia frigida | s174 | hap1 | XXIV | XXIV | XXIV | XXIV | JX036079 | JX036200 |
Buellia frigida | s176 | hap3 | XXIV | XXIV | XXIV | XXIV | JX036081 | JX036202 |
Buellia frigida | s180 | hap3 | XXIV | XXIV | XXIV | XXIV | JX036085 | JX036206 |
Buellia frigida | s189 | hap1 | XXIV | XXIV | XXIV | XXIV | JX036094 | JX036215 |
Buellia frigida | s195 | hap3 | XXIV | XXIV | XXIV | XXIV | JX036100 | JX036221 |
Buellia frigida | s221 | hap3 | XXIV | XXIV | XXIV | XXIV | JX036124 | JX036245 |
Buellia frigida | s267 | hap3 | XXIV | XXIV | XXIV | XXIV | JX036142 | JX036261 |
Buellia frigida | s187 | hap12 | XXIV | XXIV | XXIV | XXIV | JX036092 | JX036213 |
Buellia frigida | s96 | hap5 | XXIV | XXIV | XXIV | XXIV | JX036153 | JX036272 |
Rhizoplaca macleanii | s213 | hap1 | XXV | XXV | XXV | XXVa | JX036116 | JX036237 |
Rhizoplaca macleanii | s214 | hap1 | XXV | XXV | XXV | XXVa | JX036117 | JX036238 |
Rhizoplaca macleanii | s212 | hap1 | XXV | XXV | XXV | XXVa | JX036115 | JX036236 |
Rhizoplaca macleanii | s209 | hap3 | XXV | XXV | XXV | XXVa | JX036112 | JX036233 |
Rhizoplaca macleanii | s208 | hap1 | XXV | XXV | XXV | XXVa | JX036111 | JX036232 |
Rhizoplaca macleanii | s207 | hap14 | XXV | XXV | XXV | XXVb | JX036110 | JX036231 |
Rhizoplaca macleanii | s206 | hap1 | XXV | XXV | XXV | XXVb | JX036109 | JX036230 |
Rhizoplaca macleanii | s205 | hap9 | XXV | XXV | XXV | XXVb | JX036108 | JX036229 |
Rhizoplaca macleanii | s201 | hap1 | XXV | XXV | XXV | XXVb | JX036105 | JX036226 |
Rhizoplaca macleanii | s232 | hap3 | XXV | XXV | XXV | XXVa | JX036135 | JX036254 |
Rhizoplaca macleanii | s235 | hap1 | XXV | XXV | XXV | XXVa | JX036138 | JX036257 |
Rhizoplaca macleanii | s236 | hap1 | XXV | XXV | XXV | XXVa | JX036139 | JX036258 |
Rhizoplaca macleanii | s237 | hap7 | XXV | XXV | XXV | XXVa | JX036140 | JX036259 |
Rhizoplaca macleanii | s271 | hap1 | XXV | XXV | XXV | XXVb | JX036145 | JX036264 |
Rhizoplaca macleanii | s272 | hap3 | XXV | XXV | XXV | XXVa | JX036146 | JX036265 |
Rhizoplaca macleanii | s273 | hap3 | XXV | XXV | XXV | XXVa | JX036147 | JX036266 |
Rhizoplaca macleanii | s274 | hap3 | XXV | XXV | XXV | XXVa | JX036148 | JX036267 |
Rhizoplaca macleanii | s124 | hap1 | XXV | XXV | XXV | XXVc | JX036055 | JX036176 |
Rhizoplaca macleanii | s125 | hap1 | XXV | XXV | XXV | XXVc | JX036056 | JX036177 |
Rhizoplaca macleanii | s197 | hap3 | XXV | XXV | XXV | XXVc | JX036101 | JX036222 |
Rhizoplaca macleanii | s198 | hap3 | XXV | XXV | XXV | XXVc | JX036102 | JX036223 |
Rhizoplaca macleanii | s233 | hap9 | XXV | XXV | XXV | XXVc | JX036136 | JX036255 |
Rhizoplaca macleanii | s95 | hap9 | XXV | XXV | XXV | XXVc | JX036152 | JX036271 |
Lecanora sp. 2 | s175 | hap3 | XXVI | XXVI | XXVI | XXVI | JX036080 | JX036201 |
Lecanora sp. 2 | s179 | hap3 | XXVI | XXVI | XXVI | XXVI | JX036084 | JX036205 |
Lecanora sp. 2 | s173 | hap3 | XXVI | XXVI | XXVI | XXVI | JX036078 | JX036199 |
Lecanora sp. 2 | s171 | hap3 | XXVI | XXVI | XXVI | XXVI | JX036076 | JX036197 |
Lecanora sp. 2 | s106 | hap1 | XXVI | XXVI | XXVI | XXVI | JX036037 | JX036159 |
Summary of the single and multiple GMYC analyses showing the number of groups retrieved by each algorithm, the confidence intervals, the values of the likelihood of the null models, and the single and multiple GMYC and their likelihood ratio tests
Model | Ngroups | Confidence intervals | Likelihood null model | Likelihood GMYC model | Likelihood ratio |
Single | 23 | 21–26 | 948.6204 | 970.3171 | 43.39334* |
Multiple | 31 | 22–39 | 948.6204 | 971.5584 | 45.87608* |
Model | Ngroups | Confidence intervals | Likelihood null model | Likelihood GMYC model | Likelihood ratio |
Single | 23 | 21–26 | 948.6204 | 970.3171 | 43.39334* |
Multiple | 31 | 22–39 | 948.6204 | 971.5584 | 45.87608* |
*p < 0.001.
Summary of the single and multiple GMYC analyses showing the number of groups retrieved by each algorithm, the confidence intervals, the values of the likelihood of the null models, and the single and multiple GMYC and their likelihood ratio tests
Model | Ngroups | Confidence intervals | Likelihood null model | Likelihood GMYC model | Likelihood ratio |
Single | 23 | 21–26 | 948.6204 | 970.3171 | 43.39334* |
Multiple | 31 | 22–39 | 948.6204 | 971.5584 | 45.87608* |
Model | Ngroups | Confidence intervals | Likelihood null model | Likelihood GMYC model | Likelihood ratio |
Single | 23 | 21–26 | 948.6204 | 970.3171 | 43.39334* |
Multiple | 31 | 22–39 | 948.6204 | 971.5584 | 45.87608* |
*p < 0.001.
The results of implemented DNA-based methods for the delimitation of species coincided for most of the taxa found in the study area. Further, these taxa usually also corresponded to species that were well delimited from a morphological, anatomical, or chemical point of view, that is, using standard characters. However, there are some groups for which the different methods used for species delimitation disagreed. All DNA-based methods (except the genetic distance method using a 0.05% cutoff) distinguished the specimen s217 (Carbonea sp.) from Carbonea vorticosa, from which it differs in 43 positions in the ITS sequence. Re-examination of the Carbonea specimens revealed a clear difference in ascospores sizes (7−8 × 4 μm in Carbonea sp. vs. 8−12 × 4−5 μm in C. vorticosa) and a slightly stronger pigmentation of the hypothecium in Carbonea sp. The specimens identified by us as Austrolecia antarctica using standard characters turned out to be three different taxa, well differentiated by all the DNA-based methods used, again with the exception of the genetic distance-based method using a 0.05% cutoff that distinguished only one species. Specimens named as Austrolecia sp. 1 (see Table 1) shared the same ITS sequence with the exception of the specimen s182, which differed in one position. Specimens s177 and s178 (Austrolecia sp. 2) differed in two positions from each other and in 11 and 13 positions, respectively, in the most common sequence found in Austrolecia sp. 1. The only specimen corresponding to Austrolecia sp. 3 (s116) differed in 23 positions from the most common sequence in Austrolecia sp. 1, and in 15 and 13 positions from the specimens of Austrolecia sp. 2. These three taxa differed slightly from A. antarctica in their ascospores size ranges and also in minor characters of the hymenium. Further studies are being carried out to shed light on the taxonomy of the group.
Single GMYC and the genetic distance-based method with a 0.05% cutoff both failed to differentiate the specimen s202 (Lecanora cf. mons-nivis) from Lecanora cf. flotowiana (s190 and s191) although they are clearly different taxa, and had very different morphological and anatomical characters and ITS sequences that differed in 24 positions. Specimen s169 is differentiated from other specimens attributed to Caloplaca sp. 1 only by the multiple GMYC. All the Caloplaca sp. 1 shared an identical ITS sequences, whereas the specimen s169 differed in 12 positions from the rest of the group. Anatomical and morphological differences are not obvious as material for specimen s169 is scanty, which prevents more thorough further analysis. The multiple GMYC also differed from the other methods in the assignation of the specimens referred to Umbilicaria aprina. This method generated an extra group for the specimens s170, s210, and s211 (see Table 1), which only differed in two positions in the ITS sequence. No morphological or chemical deviating characters were observed in these specimens.
None of the GMYC algorithms, including the 0.05 cutoff, were able to differentiate the specimen s164 (Polysporina sp.) from Polysporina frigida (s188), in spite of their evident morphological and anatomical differences (e.g., much larger ascospores: 7−9.5 × 3−4 μm in Polysporina sp. vs. 4.5−6 × 2.5−3.5 μm in P. frigida) and differing in 21 positions in their ITS sequence. The single GMYC also failed to recognize the specimen s183 (Acarospora cf. nitrophila) as a different entity despite there being obvious morphological, anatomical, and molecular differences. The multiple GMYC produced three different groups for the specimens classified under Rhizoplaca macleanii (group XXV). Group XXVa (see Table 1) differed in one position from group XXVb and two positions from group XXVc. Group XXVb differed in a single position from group XXVc.
Photobiont diversity and identity
One hundred and nineteen new photobiont ITS sequences were obtained. Amplification of the ITS region from the specimens s116, s223, and s225 failed. A small fragment of the 5′ of the nuLSU rDNA was also included in the analysis, which resulted in an alignment of 749 bp (745 bp after gap removing with 115 variable characters). We detected 17 different unique sequences when sequences were collapsed into haplotypes. Figure 3 depicts the statistical parsimony network (Templeton et al., 1992) of genetic relationships among the haplotypes found in this study. All the haplotypes included in the connected network could represent a single taxon (Hart & Sunday, 2007). The number of sequences sharing the same haplotype is indicated together with the code given to each haplotype. Hap3 is the most abundant one with 66 records, followed by hap1 with 26 records and hap6 and hap9, both with six records each. The rest of haplotypes have very low frequency (one or two records).
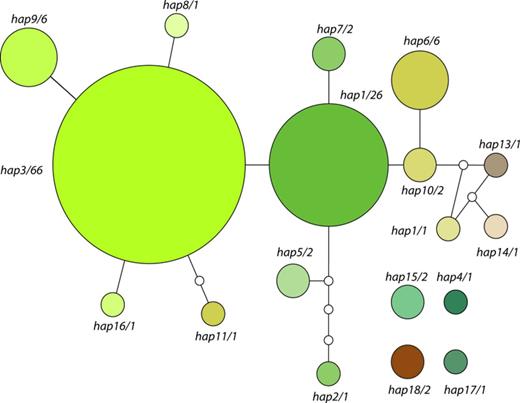
Statistical parsimony network obtained for the Trebouxia haplotypes found in this study. Number of samples found sharing that haplotype is given after the haplotype number. Size of the circles is proportional to the number of samples sharing that haplotype. Myrmecia samples have been added after the analysis to the graph to enhance visualization of Fig. 2.
We amplified a fragment of the nu18S region in the three specimens that failed with the Trebouxia-specific primer set (Kroken & Taylor, 2000). Specimen s116 failed again to amplify, but we got two identical sequences, 1686 bp length, from samples s223 and s225. A BLAST search with this sequence as template showed a 99% similarity with Myrmecia biatorellae (UTEX 907).
The alignment of the 17 ITS unique haplotypes with ITS sequences obtained from the GenBank (most similar hits, and representatives of the known species of Trebouxia) had 76 sequences, and it was 680 bp length (after removing ambiguously aligned regions and gaps by means of gblocks 0.91b (Castresana, 2000) with 597 variable positions). The topologies obtained from the Bayesian and the ML analyses were identical and are shown in Fig. 4 (50% majority rule consensus tree, midpoint rooted). Haplotypes found in lichens from the Dry Valleys fell into three different clades. First, hap4 was integrated within a well-supported clade comprising samples of Trebouxia jamesii. Close taxa obtained from the GenBank after a BLAST search include the accession numbers (GQ375366 and GQ375353 from Cetraria aculeata, EU416217 from a lichen in Parmeliaceae, and AY444765 and AY444766 from Flavocetraria nivalis from Poland. Second, hap17 is basal to clade formed by the rest of Dry Valleys haplotypes and the Trebouxia decolorans-arboricola-asymmetrica-incrustata group. Third, 15 Trebouxia haplotypes formed a group together with other five sequences retrieved from the GenBank. Three of those sequences were obtained from Xanthoria sp., but the geographic origin was uncertain as it is not detailed in the GenBank files. The accession number AJ31580 corresponds to the photobiont of an Umbilicaria species from the Antarctic Peninsula, and the accession number AY667580 to the photobiont of several lichen species collected in Granite Harbour (Ross Sea, Continental Antarctica) (De los Ríos et al., 2005b).

Fifty percent majority rule consensus phylogenetic tree obtained in the (MC)3 Bayesian analysis. Bold lines depict supported branches with a posterior probability (PP) ≥ 0.95 and a bootstrap > 70% in the ML analysis. Single asterisk denotes a branch solely supported by PP, and double asterisk denote a branch only supported by ML bootstrap.
Spatial structure of the photobiont genetic diversity
The Mantel test rejected the statically significant relationship between the matrices of the genetic distances of the photobionts and the geographic distances (rM = 0.0534, P-value: 0.1096). The relationship between the same matrices within the three designated groups (Fig. 1) was also nonsignificant (Group A: rM = −0.05602, P-value: 0.116; Group B: rM = 0.0437, P-value: 0.1073; Group C: rM = 0.0269, P-value: 0.313). Likewise, relationship between genetic distance and altitude was also not statistically significant (rM = −0.05612, P-value: 0.8591).
The analysis of the photobiont sequences sorted by geographic groups (amova) (Fig. 1) resulted in an extremely high percentage of variation attributed to within population variation (99.78%) and very low percentage of variation attributed to among population variation (0.22%). The fixation index was 0.00216, indicating a very low differentiation among populations.
Specificity and fungal selectivity toward the photobiont
Chi-squared tests using Monte Carlo estimation of exact P-values rejected the null hypothesis of independence between fungal taxa inferred by genetic distances (98% cutoff) and photobiont haplotypes in both datasets (P < 0.05). Correspondence analysis for the raw and the standardized datasets detected the same statistical correlations between photobiont hap2 and an unidentified lichen species (s107) between L.cf. mons-nivis and photobiont hap13 and between Sarcogyne privigna and photobiont hap18 (Myrmecia cf. biatorellae). The same results were obtained using the raw and standardized datasets, which indicates that abundance did not influence in our results. Further, the Spearman correlation analysis of the ordinal data showed that Acarospora sp.1, L.cf. mons-nivis, and S. privigna did not show statistically significant correlations with other taxa, so it can be treated as independent data.
Results on the genetic diversity of the photobionts found in the different lichen species are summarized in Table 3 (S. privigna excluded). Nucleotidic diversity, which is a measure of polymorphism in the populations, ranged between 0 (in C. vorticosa, Caloplaca cf. citrina and Austrolecia sp. 2) and 0.1262 in Lecidea cancriformis. Differences are particularly high among species with a high number of specimens analyzed. Thus, U. aprina (n = 19) showed a π = 14·10−5, whereas R. macleanii (n = 23) and Buellia frígida (n = 15) showed π values of 1.62 × 10−3 and 1.97 × 10−3, respectively. It is noteworthy that the maximum values correspond to Rhizoplaca sp. and L. cancriformis from which only two specimens per species were analyzed. Haplotype diversity (Hd) ranged from 0 (all the sequences shared the same haplotype) in C. vorticosa, Caloplaca cf. citrina, and Austrolecia sp. 2 (all the sequences shared the same haplotype) to 1 (all the sequences belong to different haplotypes) in Lecanora flotowiana, Rhizoplaca sp., and L. cancriformis. It is remarkable that U. aprina (n = 19) had an Hd as low as 0.105, whereas R. macleanii (n = 23), Buellia frigida (n = 15), and Austrolecia sp. 1 (n = 9) had Hd values of 0.688, 0.743 and 0.917, respectively.
Genetic diversity values of the photobionts found associated with those lichen-forming fungi taxa with more than one sample in our study (excluding Sarcogyne privigna)
Taxon code | n | h | Hd | π | |
Austrolecia sp. 1 | III | 9 | 7 | 0.917 | 0.02024 |
Austrolecia sp. 2 | IV | 2 | 1 | 0 | 0 |
Buellia frigida | XXIV | 15 | 6 | 0.743 | 0.00197 |
Caloplaca cf. citrina | XIV | 2 | 1 | 0 | 0 |
Caloplaca sp. | XII | 7 | 2 | 0.286 | 0.00039 |
Caloplaca sublobulata | XIII | 5 | 2 | 0.600 | 0.00082 |
Carbonea vorticosa | I | 3 | 1 | 0 | 0 |
Huea sp. | XI | 4 | 2 | 0.667 | 0.00272 |
Lecanora flotowiana | VII | 2 | 2 | 1 | 0.00136 |
Lecanora fuscobrunnea | XXVI | 5 | 2 | 0.400 | 0.00054 |
Lecidea cancriformis | XXI | 2 | 2 | 1 | 0.12620 |
Lecidella greenii | IX | 8 | 3 | 0.607 | 0.00161 |
Rhizoplaca macleanii | XXV | 23 | 5 | 0.688 | 0.00162 |
Rhizoplaca sp. | X | 2 | 2 | 1 | 0.04241 |
Umbilicaria aprina | XV | 19 | 2 | 0.105 | 0.00014 |
Taxon code | n | h | Hd | π | |
Austrolecia sp. 1 | III | 9 | 7 | 0.917 | 0.02024 |
Austrolecia sp. 2 | IV | 2 | 1 | 0 | 0 |
Buellia frigida | XXIV | 15 | 6 | 0.743 | 0.00197 |
Caloplaca cf. citrina | XIV | 2 | 1 | 0 | 0 |
Caloplaca sp. | XII | 7 | 2 | 0.286 | 0.00039 |
Caloplaca sublobulata | XIII | 5 | 2 | 0.600 | 0.00082 |
Carbonea vorticosa | I | 3 | 1 | 0 | 0 |
Huea sp. | XI | 4 | 2 | 0.667 | 0.00272 |
Lecanora flotowiana | VII | 2 | 2 | 1 | 0.00136 |
Lecanora fuscobrunnea | XXVI | 5 | 2 | 0.400 | 0.00054 |
Lecidea cancriformis | XXI | 2 | 2 | 1 | 0.12620 |
Lecidella greenii | IX | 8 | 3 | 0.607 | 0.00161 |
Rhizoplaca macleanii | XXV | 23 | 5 | 0.688 | 0.00162 |
Rhizoplaca sp. | X | 2 | 2 | 1 | 0.04241 |
Umbilicaria aprina | XV | 19 | 2 | 0.105 | 0.00014 |
Genetic diversity values of the photobionts found associated with those lichen-forming fungi taxa with more than one sample in our study (excluding Sarcogyne privigna)
Taxon code | n | h | Hd | π | |
Austrolecia sp. 1 | III | 9 | 7 | 0.917 | 0.02024 |
Austrolecia sp. 2 | IV | 2 | 1 | 0 | 0 |
Buellia frigida | XXIV | 15 | 6 | 0.743 | 0.00197 |
Caloplaca cf. citrina | XIV | 2 | 1 | 0 | 0 |
Caloplaca sp. | XII | 7 | 2 | 0.286 | 0.00039 |
Caloplaca sublobulata | XIII | 5 | 2 | 0.600 | 0.00082 |
Carbonea vorticosa | I | 3 | 1 | 0 | 0 |
Huea sp. | XI | 4 | 2 | 0.667 | 0.00272 |
Lecanora flotowiana | VII | 2 | 2 | 1 | 0.00136 |
Lecanora fuscobrunnea | XXVI | 5 | 2 | 0.400 | 0.00054 |
Lecidea cancriformis | XXI | 2 | 2 | 1 | 0.12620 |
Lecidella greenii | IX | 8 | 3 | 0.607 | 0.00161 |
Rhizoplaca macleanii | XXV | 23 | 5 | 0.688 | 0.00162 |
Rhizoplaca sp. | X | 2 | 2 | 1 | 0.04241 |
Umbilicaria aprina | XV | 19 | 2 | 0.105 | 0.00014 |
Taxon code | n | h | Hd | π | |
Austrolecia sp. 1 | III | 9 | 7 | 0.917 | 0.02024 |
Austrolecia sp. 2 | IV | 2 | 1 | 0 | 0 |
Buellia frigida | XXIV | 15 | 6 | 0.743 | 0.00197 |
Caloplaca cf. citrina | XIV | 2 | 1 | 0 | 0 |
Caloplaca sp. | XII | 7 | 2 | 0.286 | 0.00039 |
Caloplaca sublobulata | XIII | 5 | 2 | 0.600 | 0.00082 |
Carbonea vorticosa | I | 3 | 1 | 0 | 0 |
Huea sp. | XI | 4 | 2 | 0.667 | 0.00272 |
Lecanora flotowiana | VII | 2 | 2 | 1 | 0.00136 |
Lecanora fuscobrunnea | XXVI | 5 | 2 | 0.400 | 0.00054 |
Lecidea cancriformis | XXI | 2 | 2 | 1 | 0.12620 |
Lecidella greenii | IX | 8 | 3 | 0.607 | 0.00161 |
Rhizoplaca macleanii | XXV | 23 | 5 | 0.688 | 0.00162 |
Rhizoplaca sp. | X | 2 | 2 | 1 | 0.04241 |
Umbilicaria aprina | XV | 19 | 2 | 0.105 | 0.00014 |
Discussion
We have investigated the diversity of lichen-forming fungi, their associated photobionts, as well as the type of relationships among both bionts in one of the most adverse environments for life on Earth. The use of a combined approach (standard and molecular characters) to study the diversity of lichen-forming fungi resulted in increased lichen diversity for the McMurdo Dry Valleys. We were able to identify severely modified or endolithic sterile thalli, which would be otherwise impossible to do (i.e., using only standard characters). The accurate identification of the lichen-forming fungi gave us the opportunity to more accurately assess the degrees of specificity and selectivity toward their photobionts.
Total lichen-forming fungi diversity in the area amounted to a total of 26 different taxa (27 using the 0.02% cutoff). This is, without doubt, a high figure compared to other lichen studies carried out in continental Antarctica and taking into account the extreme environmental conditions present in the area. Thus, in Southern Victoria Land, only seven taxa were reported on the Beacon sandstone formation in the McMurdo Dry Valleys (Hale, 1987), 22 species from the Kar Plateau (76°S) in Southern Victoria Land (Seppelt et al., 1995) and 29 species from Botany Bay, Granite Harbour, 77°S (Seppelt et al., 2010), the last two places with something milder conditions than the McMurdo Dry Valleys. Green et al. (2011) found around 30 species at Mt. Kyffin, 84°S latitude near the Beardmore Glacier, while Smith (1988) reported 27 species from Wilkes Land at 66°S and Green et al. (2007) reported c. 30 species from Casey and Davies stations, also at 66°S. Perhaps the richest place studied in the Ross Sea area so far is Edmonson Point in Terra Nova Bay, with 56 species (Castello & Nimis, 1995b). Thus, the number of lichens found in our study is close to the average figure found on other lichen diversity studies in continental Antarctica.
Some of the results related to the lichen-forming diversity raise taxonomic novelties and questions, and these are being addressed in forthcoming work. Here, we prefer to focus our discussion on the performance of the DNA-based species identification approaches used in our study, as we think it can help in future floristic studies in remote areas or extreme environments.
The greatest divergences between groups identified by means of traditional morphological, chemical, and anatomical characters, and those from DNA-based procedures were in results from the GMYC algorithm. The single GMYC (Pons et al., 2006) recovered 23 different groups, whereas the multiple GMYC recovered 31 groups. These algorithms have been widely used since their development to identify species in all kind of organisms such as insects (Pinzón-Navarro et al., 2010), amphibians (Crawford et al., 2010), or bacteria (Powell, 2012). However, their use has also been criticized as the method may be highly affected by the sampling scheme (Lohse, 2009; but see Papadopoulou et al., 2009). In our case, we consider that the single GMYC gave an underestimation of the actual number of taxa present in the area because of the application of a single threshold cutoff. Individual species usually show different genetic diversity values as a result of their differential evolutionary histories. This variation in intraspecific genetic diversity among taxa can clearly affect the number of groups produced by this algorithm. However, this is not always so, as in the case of L. flotowiana and Lecanora mons-nivis, which are two morphologically well-separated species that differ in 24 positions in their alignment, but were considered as the same taxon by the single GMYC algorithm. The multiple GMYC showed a different behavior, and, in this case, problems were attributed to a false identification of population clusters below the species level as different taxa (Lohse, 2009). We found two clear examples of this in our data, the first was U. aprina that the multiple GMYC algorithm split in two clusters, and second the case of R. macleanii that the GMYC algorithm split in three different groups.
Distance-based methods, in our opinion, worked better. We implemented two different cutoffs, the first at 0.02 yielded 27 groups and the second at 0.05 yielded 26 groups. The only difference between them depended on the identification of the specimen s169 (Caloplaca sp.), which was included within Caloplaca cf. sublobulata using the 0.05 cutoff but was split into a different taxon using the 0.02 cutoff. Unfortunately, material of this sample was very scanty, and no clear statement is possible using standard characters.
Our study confirms previously published results. Kelly et al. (2011) carried out the first study where DNA sequences were used to identify lichen species at a field scale, in an all-group approach, not focusing in a single genus or group of species. They were able to identify up to 92.1% of specimens collected in a floristic survey using ITS sequences as a barcode. Likewise, Del Prado et al. (2010) used genetic distances for the recognition of specific entities within the family Parmeliaceae with a high degree of success and found no overlap between intra- and interspecific genetic distances. In both cases, problems arose in groups of poorly defined species (species complexes) in which standard morphological and chemical characters are not sufficient to differentiate species (cryptic species, see Crespo & Pérez-Ortega, 2009) or in those groups in which environmental modifications have been considered to be good taxonomic characters. Genetic distances seem to be a good tool for floristic studies in lichens, especially for cases like the Dry Valleys, where morphological changes because of the environment can, in many cases, make correct identification impossible. Even the problems that arose with the identification of specimen s169 (Caloplaca sp.) could probably have been solved if more similar specimens were available to be added to the dataset. Furthermore, the use of a DNA-based approach allowed the identification of cryptic taxa that could have been overlooked if only a standard morphological approach was used, as characters were often subtle. The discovery of a different Carbonea lineage (Carbonea sp., s217, group II) and the finding of three different lineages of Austrolecia (group III, IV and V) confirm the utility of a DNA-based approach for detecting cryptic or semi-cryptic lineages. The presence of cryptic or ‘semi-cryptic’ lineages is not a rare phenomenon among lichens as morphology-based species recognition methods are known to underestimate real species number (Crespo & Pérez-Ortega, 2009; Vondrák et al., 2009; Del Prado et al., 2010; Lumbsch & Leavitt, 2011).
The presence of three cryptic taxa of 26 taxa identified seems to be a high figure. However, there are no records of how common cryptic lineages are in different ecosystems, but a high number has been linked to the strong selective pressure on physiological characters in extreme environments (Nevo, 2001). The discovery of many cryptic species in polar regions or other extreme habitats is consistent with this latter point (Vrijenhoek et al., 1994; Grundt et al., 2006; Lefébure et al., 2006).
Photobiont diversity, selectivity, specificity, and spatial distribution
Four putative photobiont taxa were observed in this study, which correspond to at least three different lineages within the genus Trebouxia (Trebouxiaceae, Chlorophyta) (Fig. 4) and one taxon close to Myrmecia (Microthamniales, Chlorophyta). Hap4 was present in L. cancriformis (which also forms symbiosis with hap5). The former haplotype is included in the T. jamesii clade. Within this clade, hap4 constituted a well-supported group with four other accessions identified as T. jamesii and one as Trebouxia ‘hypogymniae’ ined. (Doering & Piercey-Normore, 2009). All these photobionts come from lichens collected in alpine, arctic, or boreal environments (AY444765 in Iceland from F. nivalis, EU416217 from Evernia divaricata from Canada, AY444765 from the Austrian Alps in F. nivalis and GQ375353 from Svalbard in Cetraria aculeata). Some of these specimens have been included within the concept of T.‘hypogymniae’ ined. (Hauck et al., 2007; Piercey-Normore, 2009). De la Torre et al. (2003) found a close relative of T. jamesii (99% similarity in BLAST search in nuSSU sequences) in a study of cryptoendolithic communities in the Mc Murdo Dry valleys, so it is likely than hap4 may represent the same taxon. The current morphological concept of T. jamesii is very broad, and it probably includes many lineages that could represent cryptic species (Kroken & Taylor, 2001; Piercey-Normore, 2009), some of which (including hap4) may be restricted to specific environments or by ecological constraints (Peksa & Škaloud, 2011).
The hap17 is isolated from the rest of accessions used in the analysis and is basal to the clade that includes most of the Trebouxia haplotypes found in this study. Hap17 was only found in one specimen (s91) that belongs to the taxon Austrolecia sp. 1, and which has very low selectivity toward its photobionts. A well as hap17, Austrolecia sp. 1 is also associated with six other different haplotypes. It is not clear why hap4 is so rare in the lichen community analyzed in the Dry Valleys. One possibility is that although this species in present in the area, its frequency is very low compared to other haplotypes. A second is, if we accept the presence of free-living Trebouxia in the area, that the lichen species present in the Dry Valleys show high selectivity toward their photobionts and they preferentially select haplotypes of the most abundant haplotype as it is better fitted to the ecological conditions of the area.
Most of the haplotypes found in this study form a group sister to the clade containing T. decolorans, T. asymmetrica, T. incrunstata, and T. arboricola. This group also includes accessions retrieved from the GenBank. Three of these taxa (AM159204, AM159205, and AM159208) have not been published, and geographic references are not available for these accession numbers. The two other accessions, AJ431580 (from Umbilicaria decussata) from Harrow Peaks, Central Victoria Land (Romeike et al., 2002) and AY667580 (from Lecidea sp., U. aprina and B. frigida) from Granite Harbour, Southern Victoria Land (De los Ríos et al., 2005b), probably belong to the same apparently undescribed algal taxon. Fell et al. (2006), in their study of the biodiversity of microeukaryotes in Antarctic Dry Valleys soils, found two Trebouxia taxa: one of them close to T. asymmetrica (based on SSU samples) and one of uncertain assignment.
Algal taxonomy largely relies on the ultrastructure on algal cells in culture (Friedl, 1989; Muggia et al., 2010). Our phylogenetic results seems to point to the presence of at least two undescribed algal taxon; however, further studies including algal cultures and a thorough study of their ultrastructure should be carried out before the formal description of new taxa.
The presence of the latter two taxa only in continental Antarctica, they are not known from other studies that have focused on or included algal samples from outside continental Antarctica (Romeike et al., 2002; Engelen et al., 2010; Fernández-Mendoza et al., 2011), suggests that they could be endemic in this extreme area. It has been shown recently that environmental conditions largely affect the distribution of photobiont taxa (Peksa & Škaloud, 2011) and that some eurioic lichen species with wide large distributions are able to lichenize with different photobiont taxa across their distribution range (Blaha et al., 2006; Fernández-Mendoza et al., 2011). Also, De Wever et al. (2009) have already shown the presence of Antarctic endemism associated with large geographical isolation in green algae, and this is also known to happen with other organisms such as cyanobacteria (Taton et al., 2006) or diatoms (Sabbe et al., 2004). Many of those endemic microchlorophytes taxa may have survived for long periods in glacial refugia in Antarctica (Convey & Stevens, 2007; De Wever et al., 2009), for instance the McMurdo Dry Valleys, which have probably had ice-free areas since the last Mid-to-Late Miocene (c. 14 Ma, Prentice et al., 1993).
Diversity in lichen communities
Studies dealing with the photobiont diversity from a community approach are scarce, so it is difficult to put the photobiont diversity results obtained in this study into a broader context. We found 17 different algal haplotypes associated with the lichen-forming fungi from the McMurdo Dry Valleys. As stated above, they probably correspond to four different taxa. Beck et al. (1998) studied the photobiont composition of the lichen association Physcietum adscendentis and found four different photobionts (three taxa in Trebouxia and one in Dictyiochloropsis) in 10 lichen taxa. Later, Beck (1999) found two different Trebouxia species associated with a lichen community growing on heavy-metal-rich rocks where seven species had T. jamesii as photobionts and two other species shared the taxon Trebouxia angustilobata. Bačkor et al. (2010) also studied the diversity of photobionts in a metal-rich dwelling lichen community and found several Asterochloris lineages and one Trebouxia lineage associated with 22 lichen species. Doering & Piercey-Normore (2009) found at least two Trebouxia species associated with seven epiphytic lichen species on Jack Pine in Manitoba. In comparison with these previous results, the photobiont diversity found in the McMurdo Dry Valleys does not seem to be much lower than those in other study areas. However, systematic studies following similar designs should be carried out in different areas and environments to obtain reliable and comparable diversity values.
Spatial structure
The selectivity toward the photobiont can suffer constraints both from phylogeny and from ecological mechanisms (Yahr et al., 2006). It was expected that differences in microclimate at a meso- or microscale might lead to specific relationships between the algal and fungal taxa found in the Dry Valleys. However, the results from our spatial structure analysis suggested that there is no geographic constraint at any scale and that the distribution of photobionts is apparently independent of altitude and local geography. Yahr et al. (2006) found a clear geographic pattern of photobiont distribution in the lichen Cladonia subtenuis across a broad geographic range suggesting an environment-dependent selectivity. Werth & Sork (2010) found a significant differentiation among the populations of algae associated to Ramalina menziesii in three different oak species but not among different sites, showing that substrate can be a more important factor than geography for some photobiont species. The spatial pattern of the genetic diversity of photobionts in Antarctica is currently under study in our group, and future results will help to shed light on the question whether the lack of genetic structure is a matter of scale or it is related with the extreme environmental conditions of the McMurdo Dry Valleys.
Specificity and selectivity
Beck et al. (2002) suggested that the term ‘specificity’ should be used for the description of the interaction between both symbionts taking into account the selectivity of both partners. Thus, a high specific relationship would be that when both partners show a high selectivity toward each other (Beck et al., 2002). Our analyses revealed clear patterns in the relationships of the algal haplotypes and the fungal taxa. The correspondence analysis detected three statistically supported relationships between algal haplotypes and fungal taxa. The relationships between the unidentified lichen-forming fungus (s107) and hap2, and between L.cf. mons-nivis and photobiont hap13 are highly specific as these fungal taxa, and the photobiont haplotypes are the only ones involved in these lichen symbioses. The result was obtained using standardized data, and this excludes the possibility of a side effect of haplotype abundance in the results. The relationship between S. privigna and its photobionts is intriguing. We have found S. privigna associated with M.cf. biatorellae (hap18) and also with a Trebouxia haplotype (hap11). Both photobionts were only found forming symbiosis with S. privigna and not with other lichen species. Myrmecia biatorellae has already been reported in the literature as the photobiont of S. privigna (Tschermak-Woess, 1978). Thus, it is particularly interesting that S. privigna, a sexual species that requires re-lichenization, maintains its high selectivity toward its photobiont, even in this extreme environment. The presence of these rare photobiont haplotypes forming lichens in the McMurdo Dry Valleys points toward highly selective lichen associations as they are retained as sole photobiont despite probably being uncommon in the pool of all available algae (Doering & Piercey-Normore, 2009). Until now, very few examples of high specificity are known among lichens, as most of the algal symbionts are able to form symbiosis with several lichen species, genera, or even families. An exception is the interaction between the fungus Mastodia tessellata (Verrucariaceae, Ascomycota) and the green alga Prasiola sp (Pérez-Ortega et al., 2010), which may be the first confirmed high specific relationship between a lichen-forming fungus and a photobiont. Studies in other microlichens, such as Lecanora species, showed that they usually had low selectivity toward their photobionts (Blaha et al., 2006; Guzow-Krzemińska, 2006; S. Pérez-Ortega & C. Printzen, unpublished results). However, information about selectivity and specificity in lichens remains scarce.
Genetic diversity measurements gave, without doubt, a more accurate perspective about the different relationships toward the photobionts shown by the different lichen species. It has been shown that a single lichen species is able to accept several photobiont strains or species (Blaha et al., 2006; Guzow-Krzemińska, 2006), but some species have a preferred species or strain (Doering & Piercey-Normore, 2009; Werth & Sork, 2010). In Antarctic lichens, Romeike et al. (2002) showed differential selectivity in Umbilicaria species from Antarctica, with species as Umbilicaria antarctica accepting up to three different algal strains and species as Umbilicaria umbilicarioides accepting just one strain. In the Dry Valleys, some species, like U. aprina, seemed to be moderately selective toward their photobionts while others, like R. macleanii, B. frigida or Austrolecia sp. 1, showed high levels of both nucleotidic and haplotypic diversity. When we take into account the fact that none of the species studied here reproduces via vegetative propagules and that all need a lichenization event to form a lichen thallus, it seems clear that not all the photobiont haplotypes found in the area are accepted equally.
Acknowledgements
We are grateful to Antarctica New Zealand (AntNZ) for logistical support. The New Zealand Foundation for Research, Science and Technology (FRST), the University of Waikato Vice Chancellor's Fund, and the Department of Biological Sciences, University of Waikato provided financial support. We thank J. Raggio (Madrid) for collecting some of the samples and U. Rupert (Salzburg) for her help in the field. The study was funded by the Spanish Education Ministry grant CTM2009-12838-C04-03. SPO was supported by a JAE-Doc postdoctoral grant (CSIC). During completion of the research, TGAG and ADR were supported by the FRST grant, Understanding, valuing, and protecting Antarctica's unique terrestrial ecosystems: predicting biocomplexity in Dry Valley ecosystems, and by the Spanish Education Ministry grant POL2006-08405 and CTM2009-12838-C04-01/03. We are also very obliged to Laura Barrios (Unidad Estadística. Área de Informática Científica. SGAI, CSIC) for her statistical advice.
References
Author notes
Editor: Nina Gunde-Cimerman