-
PDF
- Split View
-
Views
-
Cite
Cite
Christin Zachow, Jamshid Fatehi, Massimiliano Cardinale, Ralf Tilcher, Gabriele Berg, Strain-specific colonization pattern of Rhizoctonia antagonists in the root system of sugar beet, FEMS Microbiology Ecology, Volume 74, Issue 1, October 2010, Pages 124–135, https://doi.org/10.1111/j.1574-6941.2010.00930.x
- Share Icon Share
To develop effective biocontrol strategies, basic knowledge of plant growth promotion (PGP) and root colonization by antagonists is essential. The survival and colonization patterns of five different biocontrol agents against Rhizoctonia solani AG2-2IIIB in the rhizosphere of greenhouse-grown sugar beet plants were analysed in single and combined treatments. The study included bacteria (Pseudomonas fluorescens L13-6-12, Pseudomonas trivialis RE*1-1-14, Serratia plymuthica 3Re4-18) as well as fungi (Trichoderma gamsii AT1-2-4, Trichoderma velutinum G1/8). Microscopic analysis by confocal laser scanning microscopy revealed different colonization patterns for each DsRed2/green fluorescent protein-labelled strain. Bacteria and T. velutinum G1/8 colonized the root surface and the endorhiza in single and co-culture, while for T. gamsii AT1-2-4, only the transfer of spores was observed. Whereas Pseudomonas strains formed large microcolonies consisting of hundreds of cells, S. plymuthica was arranged in small endophytic clusters or clouds around the entire root system. In co-culture, each strain showed its typical pattern and occupied specific niches on the root, without clear evidence of morphological interactions. PGP was only observed for four strains with rhizosphere competence and not for T. gamsii AT1-2-4. The results provide useful information on which combination of strains to test in larger biocontrol experiments directed to applications.
Introduction
The widespread soil-borne fungus Rhizoctonia solani Kühn (teleomorph: Thanatephorus cucumeris [Frank] Donk) is responsible for serious damage to many economically important agricultural and horticultural crops as well as to trees (Sneh et al., 1996). In the last few years, the importance of R. solani has increased considerably in Europe (Grosch et al., 2005b). Although some broad-spectrum fungicides against this pathogen are available, the control of R. solani is difficult, because of the ability to survive as sclerotia under adverse environmental conditions for many years, its saprophytic activity, and its extremely wide host range. For example, no possibility to control late root rot disease caused by R. solani AG2-2IIIB, which cause high yield losses in sugar beet (Beta vulgaris L.), is currently available (Tilcher, 2002).
Therefore, in the last decades, R. solani was a preferred target pathogen in biological control research (Elad et al., 1980; Thrane et al., 2000; Kiewnick et al., 2001; Walker et al., 2002; reviewed in Grosch et al., 2005b). However, the problem of developing effective and consistent biological control strategies for Rhizoctonia is linked to the special ecological behaviour of the pathogen. Knowledge of the biocontrol agents (BCAs) and their ability to colonize the root system and interact with the pathogen and host plant can help to solve these problems. A superior rhizosphere competence is a key feature for consistent biological control activity in the rhizosphere, a fact shown for bacterial (Lugtenberg & Kamilova, 2009) as well as fungal strains (Lu et al., 2004). The steps of colonization include recognition, adherence, invasion (only endophytes and pathogens), growth, and several strategies to establish interaction (Sørensen, 1997; Berg, 2009); plant–microorganism signalling is often involved in colonization processes (Bais et al., 2006). Study of the colonization of plants has been considerably facilitated by the application of fluorescent proteins, which are used as vital markers and reporter genes (Jansson, 2003; Bloemberg, 2007), but the majority of studies were carried out under gnotobiotic conditions and used only single strains (Chin-A-Woeng et al., 1997; Tombolini et al., 1999; Lu et al., 2004; Götz et al., 2006; Olivain et al., 2006; Chacón et al., 2007).
During the last few years, we have developed strategies to select BCAs against Rhizoctonia using a hierarchical screening scheme (Faltin et al., 2004) and to assess their biocontrol capacity (Grosch et al., 2005a, 2006). In these studies, interesting candidates belonging to bacteria, for example, Serratia plymuthica 3Re4-18 (Berg et al., 2005), Pseudomonas fluorescens L13-6-12 (Lottmann et al., 1999), and Pseudomonas trivialis RE*1-1-14 (Zachow et al., 2008), and to the fungal genus Trichoderma, for example Trichoderma gamsii AT1-2-4 (Zachow et al., 2008), and Trichoderma velutinum G1/8 (Grosch et al., 2006) were selected. Interestingly, application of single strains, but especially combinations of these strains, showed antagonistic activities in vitro, but also plant growth promotion (PGP) and biocontrol activity against R. solani on sugar beet in different field trials (G. Berg, unpublished data). In contrast, no pathogenic potential to Caenorhabditis elegans, which poses a risk to human health (Zachow et al., 2009) and no or only a negligible short-term impact on the microbial community in the rhizosphere were found (Scherwinski et al., 2008). Despite their promising interaction with sugar beet plants, the in situ effect should be optimized and stabilized by studying the colonization pattern and their interaction within the root system.
The objective of this study was to monitor the colonization behaviour in the root system of sugar beet as well as the PGP effect by the selected BCAs, and to translate the information into efficient biocontrol strategies. The selection contained strains from sugar beet (RE*1-1-14, AT1-2-4) as well as from other host plants (3Re4-18, L13-6-12, G1/8). We used the red fluorescent protein gene (DsRed2) for the bacterial BCAs and the green fluorescent protein (GFP) for the transformation of two different Trichoderma strains, and studied single and combined treatments in different bioassays (germination pouches, sterile and nonsterile soil) with confocal laser scanning microscopy (CLSM) and plate counting.
Materials and methods
Fungal and bacterial strains
Three strains of bacteria and two fungal biocontrol strains derived from endo- and ectophytic microenvironments of different crops were used in this study (Table 1). The antagonistic in vitro activity against different plant pathogens was assessed according to Zachow et al. (2008).
Strains | EMBL number | Origin | Antagonistic activity against | References | ||||||||
A. c. | B. c. | G. b. | P. b. | P. u. | R. s.1 | R. s.2 | S. r. | V. d. | ||||
Pseudomonas fluorescens L13-6-12 | FN675867 | Rhizosphere of potato | ND | + | ND | + | ND | +++ | +++ | + | +++ | Lottmann et al. (1999), Faltin et al. (2004), Scherwinski et al. (2008) |
Serratia plymuthica 3Re4-18 | FN675868 | Endorhiza of potato | ND | ++ | ND | +++ | ND | +++ | +++ | +++ | +++ | Berg et al. (2005), Faltin et al. (2004), Scherwinski et al. (2008) |
Pseudomonas trivialis RE*1-1-14 | FN675869 | Endorhiza of sugar beet | ND | − | ND | ++ | − | +++ | +++ | + | − | Present study |
Trichoderma gamsii AT1-2-4 | FN675870 | Rhizosphere of sugar beet | +++ | +++ | +++ | +++ | +++ | +++ | +++ | + | +++ | Present study |
Trichoderma velutinum G1/8 | FN675871 | Sclerotia of R. solani on potato tuber | ND | +++ | +++ | + | ND | +++ | +++ | + | +++ | Grosch et al. (2006) |
Strains | EMBL number | Origin | Antagonistic activity against | References | ||||||||
A. c. | B. c. | G. b. | P. b. | P. u. | R. s.1 | R. s.2 | S. r. | V. d. | ||||
Pseudomonas fluorescens L13-6-12 | FN675867 | Rhizosphere of potato | ND | + | ND | + | ND | +++ | +++ | + | +++ | Lottmann et al. (1999), Faltin et al. (2004), Scherwinski et al. (2008) |
Serratia plymuthica 3Re4-18 | FN675868 | Endorhiza of potato | ND | ++ | ND | +++ | ND | +++ | +++ | +++ | +++ | Berg et al. (2005), Faltin et al. (2004), Scherwinski et al. (2008) |
Pseudomonas trivialis RE*1-1-14 | FN675869 | Endorhiza of sugar beet | ND | − | ND | ++ | − | +++ | +++ | + | − | Present study |
Trichoderma gamsii AT1-2-4 | FN675870 | Rhizosphere of sugar beet | +++ | +++ | +++ | +++ | +++ | +++ | +++ | + | +++ | Present study |
Trichoderma velutinum G1/8 | FN675871 | Sclerotia of R. solani on potato tuber | ND | +++ | +++ | + | ND | +++ | +++ | + | +++ | Grosch et al. (2006) |
Inhibition of microsclerotia; A. c., Aphanomyces cochlioides; B. c., Botrytis cinerea; G. b., Guignardia bidwellii; P. b., Phoma betae; P. u., Pythium ultimum; R. s.1, Rhizoctonia solani AG2-2III B; R. s.2,R. solani AG4; S. r., Sclerotium rolfsii; V. d., Verticillium dahliae V25; +, 0–5 mm; ++, 5–10 mm; +++, >10 mm radius of zone of inhibition in a dual-culture assay; −, no suppression; ND, not determined.
Strains | EMBL number | Origin | Antagonistic activity against | References | ||||||||
A. c. | B. c. | G. b. | P. b. | P. u. | R. s.1 | R. s.2 | S. r. | V. d. | ||||
Pseudomonas fluorescens L13-6-12 | FN675867 | Rhizosphere of potato | ND | + | ND | + | ND | +++ | +++ | + | +++ | Lottmann et al. (1999), Faltin et al. (2004), Scherwinski et al. (2008) |
Serratia plymuthica 3Re4-18 | FN675868 | Endorhiza of potato | ND | ++ | ND | +++ | ND | +++ | +++ | +++ | +++ | Berg et al. (2005), Faltin et al. (2004), Scherwinski et al. (2008) |
Pseudomonas trivialis RE*1-1-14 | FN675869 | Endorhiza of sugar beet | ND | − | ND | ++ | − | +++ | +++ | + | − | Present study |
Trichoderma gamsii AT1-2-4 | FN675870 | Rhizosphere of sugar beet | +++ | +++ | +++ | +++ | +++ | +++ | +++ | + | +++ | Present study |
Trichoderma velutinum G1/8 | FN675871 | Sclerotia of R. solani on potato tuber | ND | +++ | +++ | + | ND | +++ | +++ | + | +++ | Grosch et al. (2006) |
Strains | EMBL number | Origin | Antagonistic activity against | References | ||||||||
A. c. | B. c. | G. b. | P. b. | P. u. | R. s.1 | R. s.2 | S. r. | V. d. | ||||
Pseudomonas fluorescens L13-6-12 | FN675867 | Rhizosphere of potato | ND | + | ND | + | ND | +++ | +++ | + | +++ | Lottmann et al. (1999), Faltin et al. (2004), Scherwinski et al. (2008) |
Serratia plymuthica 3Re4-18 | FN675868 | Endorhiza of potato | ND | ++ | ND | +++ | ND | +++ | +++ | +++ | +++ | Berg et al. (2005), Faltin et al. (2004), Scherwinski et al. (2008) |
Pseudomonas trivialis RE*1-1-14 | FN675869 | Endorhiza of sugar beet | ND | − | ND | ++ | − | +++ | +++ | + | − | Present study |
Trichoderma gamsii AT1-2-4 | FN675870 | Rhizosphere of sugar beet | +++ | +++ | +++ | +++ | +++ | +++ | +++ | + | +++ | Present study |
Trichoderma velutinum G1/8 | FN675871 | Sclerotia of R. solani on potato tuber | ND | +++ | +++ | + | ND | +++ | +++ | + | +++ | Grosch et al. (2006) |
Inhibition of microsclerotia; A. c., Aphanomyces cochlioides; B. c., Botrytis cinerea; G. b., Guignardia bidwellii; P. b., Phoma betae; P. u., Pythium ultimum; R. s.1, Rhizoctonia solani AG2-2III B; R. s.2,R. solani AG4; S. r., Sclerotium rolfsii; V. d., Verticillium dahliae V25; +, 0–5 mm; ++, 5–10 mm; +++, >10 mm radius of zone of inhibition in a dual-culture assay; −, no suppression; ND, not determined.
Screening for PGP on lettuce seedlings
PGP assays were performed according to Faltin et al. (2004), with some modifications. Briefly, surface-sterilized (1% NaOCl, 5 min) lettuce seeds (Lactuca sativa cv. ‘Attraktion’) (Lidl, Neckarsulm, Germany) were pregerminated for 2 days in Petri dishes containing Gamborgs B5 medium (basal salt mixture including vitamins; Duchefa Biochemie, Haarlem, the Netherlands) solidified with 7 g L−1 plant agar (Duchefa Biochemie) in moist chambers at 22 °C for 2 days. The sterility of the seeds was proved by plating on nutrient agar. Standard 24-well microplates (Roth, Karlsruhe, Germany) were filled with 2 mL of plant agar. One pregerminated seed was placed in each well, followed by the addition of 10 μL of conidia (≈103 conidia) or bacterial suspension (105 cells mL−1). Bacterial cells were obtained by harvesting cells of an overnight culture grown in nutrient broth (NB, 30 °C, 120 r.p.m.) by centrifugation and resuspension in a physiological sodium chloride (NaCl) solution (0.85%). As a control, lettuce seedlings were treated with 10 μL of a sterile 0.85% NaCl solution. Each strain was tested in 24 replicates. To determine the effect of the BCAs on the plant, 2 weeks after incubation (22 °C, 16/8 h day/night, and artificial lights), the fresh weight was determined in a phytochamber (Binder KBWF 720, Tuttlingen, Germany) by gently removing and weighing the plants. Foliar areas were measured by photographing the collected leaves using a WILD M3B stereo microscope (Heerbrugg, Switzerland) with an adapted Nikon AF-S Nikkor IF-ED camera. Surface areas (mm2) and the length of the leaves and roots were measured using imagej software (Abramoff et al., 2004, http://rsb.info.nih.gov/ij/index.html). Samples from independent experiments were processed and imaged under identical conditions. The overall significant differences between the treatments were determined using anova and Tukey's post hoc test.
Labelling of bacterial and fungal biocontrol strains
Three bacterial strains were genetically transformed with a rhizosphere-stable plasmid pME6031-DsRed2 (constructed by J. Fatehi, unpublished data). The general strategy for the construction of this vector was adopted from Bloemberg et al. (2000), with further modifications. Briefly, the DsRed2 gene inserted into plasmid pBBR1MCS-5 (kindly provided by Dr Kovach, Louisiana State University) was introduced into the rhizosphere-stable vector pME6031 (generously provided by Dr Heeb, University of Nottingham, UK) and the resulting plasmid was used for the transformation of the bacterial strains. The overnight bacterial culture in Luria–Bertani (LB; Sifin, Berlin, Germany) was used for inoculation of 50 mL LB incubated at 28 °C for around 5 h (A600 nm∼0.5). The cells were incubated on ice for 30 min, harvested by centrifugation at 5000 g (4 °C, 15 min), washed three times with 1 mL of ice-cold double-distilled water, and then resuspended in a small volume (0.5–1.5 mL) of ice-cold 10% glycerol. Approximately 200–300 ng of plasmid (5 μL) was mixed with 50 μL of the cells into an ice-cold electroporation cuvette (2 mm gap width). The electroporation of bacterial cells was carried out in a BioRad Gene Pulser II (BioRad, Hercules, CA) adjusted to 2.5 kV, 200 Ω, and 25 μF. Immediately after electroporation, the cells were diluted with 1 mL LB medium supplemented with 20 mM MgCl2 and incubated on a rotary shaker at 50 r.p.m. for 2 h at 30 °C, before they were plated on LB agar supplemented with the 40 μg mL−1 of tetracycline. Single colonies of transformants were selected and transferred onto new LB plates containing 40 μg mL−1 of tetracycline.
The transformation of T. gamsii AT-1-2-4 and T. velutinum G1/8 was performed using two different methods of polyethylene glycol (PEG)-mediated and Agrobacterium-mediated DNA transformations using different vectors. In PEG-mediated transformation, protoplasts were first prepared and transformation was performed according to Kistler & Benny (1992), modified by Mes et al. (1999), with some additional modifications according to Nahalkova & Fatehi (2003). Briefly, 0.1-strength potato dextrose broth (PDB; Difco, Detroit, MI) was inoculated with approximately 107 conidia and incubated at 25 °C for 18 h at 70 r.p.m. Germinated spores were harvested by centrifugation at 2000 g (4 °C, 10 min), washed three times with an MgSO4 solution (1 M MgSO4, 20 mM MES, pH 5.8), and digested for 4–5 h at 30 °C on a rotary shaker (50 r.p.m.). The digesting solution included 10 mg mL−1 of lysing enzymes (Sigma, St. Louis, MO), 10 mg mL−1 of driselase (Sigma) and 1 mg mL−1 of chitinase (Sigma) in a final volume of 25 mL of the MgSO4 solution. The protoplasts were separated by filtration through one layer of Miracloth (Calbiochem, San Diego, CA) and kept on ice during all of the following steps. After addition of four volumes of sorbitol solution (1 M sorbitol, 10 mM CaCl2, 10 mM Tris, pH 8.0), the protoplasts were centrifuged, washed, and resuspended to a final concentration of 107 protoplasts mL−1 in the same buffer. Fifteen micrograms of the vector pIGPAPA (kindly provided by B. Gillian Turgeon), harbouring both hygromycin resistance and gfp genes under the control of the isocitrate lyase gene (IL) promoter of Neurospora crassa, was prepared in 20 μL of deionized water and used for the transformation of protoplasts. The protoplast suspension (200 μL) and the plasmid solution (20 μL) were mixed and incubated for 30 min on ice. Then, 1.2 mL of PEG solution (60% w/v PEG 6000, 50 mM CaCl2, 10 mM Tris, pH 7.5) was gradually added and the mixture was incubated on ice for 30 min. Finally, the protoplasts were washed with 0.5 M MgSO4 in 0.1% w/v PDB. After centrifugation at 2000 g (4 °C, 10 min), the protoplasts were resuspended with the 0.5 M MgSO4 solution and spread on cornmeal dextrose (CMD) medium (amended with 1 M sorbitol, 10 mM Tris, pH 8.0). The protoplasts were allowed to regenerate, before overlaying the agar with selective medium (CMD containing 100 μg mL−1 hygromycin B; Gibco BRL, Grand Island, NY).
After 3–5 days, the putative transformants were transferred to a selective synthetic nutrient agar (SNA) medium (1 g KH2PO4, 1 g KNO3, 0.5 g MgSO4× 7 H2O, 0.5 g KCl, 0.2 g glucose, 0.2 g sucrose, 1 L demineralized water, 0.6 mL 1 M NaOH, and 22 g agar, pH 6.5, complemented with 100 μg mL−1 hygromycin B) amended with a sterile filter paper to improve the sporulation. The growing colonies, resembling the wild-type strains, were examined for the expression of GFP under an Aristoplan epifluorescence microscope (Leitz, Wetzlar, Germany) equipped with an Endow GFP Long Pass filter set (470/40-nm excitation filter and 500-nm emission filter, Chroma Technology Corp.). Several single spore isolates were prepared from each of the selected transformant colonies expressing GFP in order to avoid heterokaryons. The genetic stability of the transformants was evaluated by inoculation on nonselective medium (without antibiotic) for several subcultures.
Agrobacterium-mediated transformation of T. velutinum G1/8 was performed according to the method described by Rho et al. (2001), with slight modifications. The binary vector pCAMBgfp introduced into A. tumefaciens strain AGL-1, kindly provided by Dr Ane Sesma (The Sainsbury Laboratory, John Innes Centre, UK), was used for the transformation of conidia of the fungal strain. Briefly, the transformed Agrobacterium strain was grown at 28 °C for 2 days in a minimal medium supplemented with kanamycin (50 μg mL−1) (Hooykaas et al., 1979). Two millilitres of the culture was centrifuged and washed with induction medium (IM) (Bundock et al., 1995). The cells were resuspended with 5 mL of IM in the presence of 200 μM acetosyringone and were grown for 6 h. One hundred microlitres of this culture was mixed with an equal volume of conidial suspension containing approximately 107 conidia mL−1 and spread on nitrocellulose filters (0.45-μm pore; Whatman), which were placed on a coinoculation medium (IM+200 μM acetosyringone) and incubated at 25 °C for 24 h. Then, the plates were overlaid with SNA containing cefotaxime (200 μg mL−1) and hygromycin B (100 μg mL−1). The growing colonies on the surface of the agar were subcultured on the selective SNA medium. The genetic stability and expression of GFP in the transformants were evaluated as described above.
Inoculation of sugar beet seeds with DsRed2/GFP-labelled antagonists and bioassays
Sugar beet seeds (B. vulgaris L., provided by the KWS SAAT AG, Einbeck, Germany) were washed three times with sterile water. The DsRed2-labelled bacteria were grown in NB II (Sifin) supplemented with tetracycline (40 μg mL−1) for 24 h at 30 °C and 120 r.p.m. The bacterial cells were collected by centrifugation at 5000 g for 5 min and resuspended in a fresh NB medium without the addition of any antibiotics. The cell suspension was adjusted to an OD corresponding to a cell count of 109 cells mL−1. In 9-cm-diameter Petri dishes, 100 seeds were mixed with 15 mL of the cell suspensions of each strain and incubated at room temperature for at least 3 h, followed by washing steps with sterile water. Seeds were air dried at 20 °C for 24 h, before they were sown into a sterile soil/sand mixture (5 : 1). In addition, six seeds were aseptically placed in each germination pouch (Mega International, Minneapolis, MN), which had been filled with 10 mL of sterile water. For each treatment, 10 pouches were prepared. The pouches were placed upright in metal racks, with one treatment per rack and two empty pouches at both ends of the racks to prevent cross-contamination. The pots and germination pouches were placed in a phytochamber (Binder KBWF 720) at 22 °C, 16/8 h day/night, and artificial lights. Seeds incubated with sterile water for at least 3 h served as a control. After 14 days, bacteria were reisolated on a tetracycline-containing medium (40 μg mL−1). Different parts according to the length of the seedling roots were analysed to determine the cell densities: (1) the first third of the root (base), (2) the second third (middle), and (3) the last third (root tip).
Trichoderma gamsii AT1-2-4 and T. velutinum G1/8 were cultivated on SNA medium with 100 mg mL−1 hygromycin B and covered with 2–3 small pieces of a sterile filter paper. Conidia were harvested after 7 days of cultivation at room temperature with a sterile 0.85% NaCl solution and adjusted to approximately 106 conidia mL−1. In 6-cm-diameter Petri dishes, 50 seeds were incubated in 5 mL of the conidial suspension and incubated on a rotary shaker at 120 r.p.m. for 18 h at room temperature. Further steps were carried out as described for bacteria.
CLSM
After 2–30 days, the colonization patterns of bacteria (DsRed2) and fungi (GFP) on the roots of sugar beet seedlings were monitored for fluorescence light under an Aristoplan epifluorescence microscope (Leitz). For a more in-depth investigation, transformed bacteria and fungi were observed using a Leica TCS SP CLSM (Leica Microsystems, Wetzlar, Germany) equipped with argon and helium/neon lasers. Three confocal light channels were observed simultaneously plus a further bright field channel. Filter settings were adjusted to achieve the maximum signal from each fluorescent protein and low background autofluorescence of the plant tissue. Maximum projections of an appropriate number of 0.8–1-μm-depth optical slices were applied to visualize the root sections (confocal stacks). Up to 15 scans per optical slice were averaged to improve the image resolution and to reduce noise. The software imaris® 6.0 (Bitplane, Zurich, Switzerland) was used for 3D rendering of confocal stacks and the generation of surface-spot models. Furthermore, the confocal stacks were imported in imagej and the brightness/contrast was equally adjusted in all optical slices. image surfer (http://imagesurfer.cs.unc.edu/) was then used to visualize the stacks using the ‘volume rendering’ method (Feng et al., 2007). Single TIFF snapshots were imported in coral draw X4 to be assembled and labelled.
Determination of bacterial and fungal abundances
For reisolation, tetracycline-resistant, DsRed2-labelled bacteria and hygromycin B-resistant GFP-labelled Trichoderma were used. Before transplanting, bacterial and fungal colonization of the sugar beet seeds was assessed. In each treatment, five seeds were pestled in 2 mL sterile 0.85% NaCl, and CFUs were determined by serial dilution plating on an appropriate selective medium. For reisolation of bacteria, NB medium (Sifin) complemented with 40 μg mL−1 tetracycline and, for reisolation of Trichoderma strains, SNA containing 100 μg mL−1 hygromycin B was used. To determine the abundances of bacterial and Trichoderma strains in pot or germination pouch experiments, sugar beet seedlings were uprooted and the roots were cut into three pieces as described previously. Each part was weighed, shaken, and pestled in 2 mL sterile 0.85% NaCl, and CFUs were determined by serial dilution plating on an appropriate selective medium as described previously. Each experiment was repeated at least two times.
Results
PGP on lettuce seedlings
In an in vitro bioassay, the PGP effect of the bacterial and fungal strains was evaluated on a lettuce seedlings assay, which is a fast assay to monitor PGP in microtitre plates according to Faltin et al. (2004). The PGP effect of the BCAs to the seedlings was monitored by measuring the fresh weight, the area of leaves, and the length of the leaves and roots. In this assay, the best effect was found on the length of the lettuce leaves, which was statistically significantly increased as a result of bacterial and fungal treatments. The length of the leaves (mm) of the plants treated with T. velutinum G1/8 was the highest (14.38±2.35***), followed by treatments with P. fluorescens L13-6-12 (10.61±0.75*), P. trivialis RE*1-1-14 (10.58±0.66*), and S. plymuthica 3Re4-18 (9.38±0.62) in comparison with the untreated control plants (8.14±0.59) and T. gamsii AT1-2-4 (9.75±1.04). The same PGP effect was measured for the size of the leaf surface (mm2). The highest promotion was found for the treatments with G1/8 (62.07±7.33***), L13-6-12 (47.80±4.88), RE*1-1-14 (48.41±4.65), and 3Re4-18 (37.70±4.03) in comparison with the untreated control (28.47±3.46) and AT1-2-4 (29.07±4.39). In T. velutinum G1/8 treatments, all the growth parameters measured, including the weight, length, and surface area of the leaves and root length, were statistically significantly enhanced (P≤0.001) in comparison with both the untreated control and the T. gamsii AT1-2-4 treatment. The latter showed no impact on plant growth.
Labelling of bacterial and fungal biocontrol strains
The bacterial strains P. fluorescens L13-6-12, P. trivialis RE*1-1-14, and S. plymuthica 3Re4-18 were transformed using the rhizosphere-stable vector pME6031-DsRed2. Single colony isolates from the transformed bacteria were obtained. The transformants cultured on both selective and nonselective growth media showed a bright red fluorescent colour, and they were stable after several subculturings. The colony shape, size, and growth as well as the antagonistic in vitro properties of these transformants did not differ from those of the wild-type strains.
The transformation of T. gamsii AT1-2-4 and T. velutinum G1/8 was first carried out with a PEG-mediated protocol using two different sets of vectors containing a selective resistance gene to hygromycin B antibiotic and the GFP gene. From each strain, several colonies were obtained, which were capable of growing on the selective medium, and some of these transformants in T. gamsii AT1-2-4 showed a relatively good expression of GFP. However, none of the transformants of T. velutinum G1/8 showed detectable GFP expression. In this strain, there were two to three transformants with a relatively good level of GFP expression, but they had lost their sporulation abilities. Nucleus staining showed multinucleated fate of hyphae of T. gamsii AT1-2-4 and T. velutinum G1/8 with mononucleolus conidia (data not shown). Therefore, single conidium isolation was carried out in order to obtain homokaryotic transformants. Agrobacterium-mediated transformation was performed with T. velutinum G1/8, which yielded around six transformants, which grew rapidly on the selective medium, and they appeared moderate to very bright green. The transformants were also compared with the wild-type strain and on the basis of colony morphology, sporulation, and antagonistic in vitro activity from each strain, one transformant with bright green fluorescence was selected.
Microscopic monitoring of bacterial and fungal biocontrol strains
The colonization patterns of the labelled bacterial (DsRed) and Trichoderma (GFP) strains were monitored with CLSM in different bioassays. For sugar beet seedlings cultivated in sterile soil, microcolonies of P. fluorescens L13-6-12 were observed mainly on the upper parts of the roots. Each of the microcolonies consisted of tens to hundreds bacterial cells, forming an interconnected network between epidermal cells, in the rhizoplane (Fig. 1a). A preferential root colonization was also found for the endophytic P. trivialis RE*1-1-14. The cells were arranged in microcolonies between the epidermal cells. Each of them contained several hundred cells and was especially dense in cracks and compartments between root cells, as well as in junctions of lateral roots with the main root (Fig. 1b). The surface models generated by imaris® 6.0 software calculated signals from CLSM analysis and converted them to three-dimensional images. Interestingly, the bacterial cell of P. trivialis RE*1-1-14 appeared to be inside the plant tissue or possibly embedded in a polymer matrix surrounding the roots (Fig. 1c). Root colonization patterns were also examined for S. plymuthica 3Re4-18. However, in contrast to the two pseudomonads, which were found close to the root (rhizoplane), the cells were often found as surrounding clouds. The cells showed two different colonization patterns: single cells covering the entire root surface or endophytic in the rhizosphere (Figs 1d and 2c). Aggregate formation similar to those of the two pseudomonads was observed: the cells formed a small cluster between epidermal cells (Fig. 1e). The three-dimensional images revealed the same endophytic colonization pattern as that found for the endophyte P. trivialis RE*1-1-14 (Fig. 1f).
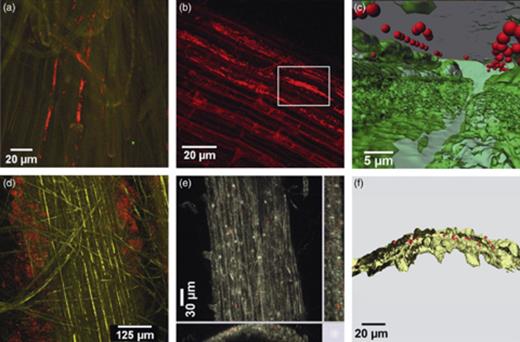
Root epidermis of a sugar beet seedling 14 days after inoculation. Visualization of DsRed-marked bacteria with CLSM. (a) Maximum projection of a confocal stack the region located approximately 3 cm under the stem and lateral roots colonized by Pseudomonas fluorescens L13-6-12 at a high cell density. The cells were observed in the intercellular space of the epidermis cell layer, forming clusters containing several hundred cells in the rhizoplane. (b) Maximum projection of a confocal stack showing Pseudomonas trivialis RE*1-1-14 aligned along the epidermis cells and at the junctions of the epidermis to the lateral root. (c) Surface model of the framed area of (b) obtained with imaris® 6.0 (Bitplane) clearly showing the bacterial cells also colonizing the internal parts of the surface of the sugar beet seedling. Green, root; red, bacterial cells; grey, surface matrix. (d) Maximum projection of confocal stack showing Serratia plymuthica 3Re4-18 cells (red) colonizing the surrounding area of the root (yellow), the rhizosphere. (e) xy, xz, and yz maximum projections showing a clear endophytic localization of small colonies and single cells of S. plymuthica 3Re4-18. (f) Surface model of (e) showing the endophytic localization of S. plymuthica 3Re4-18 in the three-dimensional space.
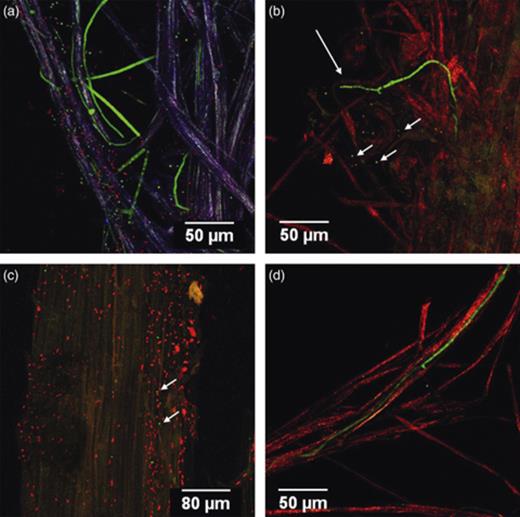
Colonization pattern of DsRed-transformed bacteria and GFP-transformed Trichoderma velutinum G1/8 in co-culture (a) Pseudomonas fluorescens L13-6-12 cells (red) and T. velutinum G1/8 conidia (green) colonized in a loose arrangement in the lateral roots (purple). Hyphae (green) grew in parallel to lateral roots as well as coiled around the lateral roots. (b) Bacterial cells of Serratia plymuthica 3Re4-18 (red) forming smaller microcolonies on the root surface as well as larger colonies in submontane areas and T. velutinum G1/8 hyphae colonized a root hair. Short arrow, conidia; long arrow, hyphae. (c) Serratia plymuthica 3Re4-18 (red) cells showing a loose arrangement around the main root. Ungerminated conidia (green) indicated with arrows were in between the cells. (d) Fungal hyphae and Pseudomonas trivialis RE*1-1-14 cells grew together with the lateral roots and root hairs colonizing the entire root surface.
Sugar beet seeds separately inoculated with the conidia of the GFP-tagged Trichoderma strains were cultivated in sterile soil. Two weeks after planting, the seedlings were harvested and examined using CLSM. Trichoderma gamsii AT1-2-4 conidia had been transferred from seeds by the growing roots of sugar beet seedlings. The conidia were attached to the root surface and root hairs, but none had been germinated after 14 days (Fig. 3a). Because of the missing effect in terms of PGP and germination, we excluded this strain from further experiments.
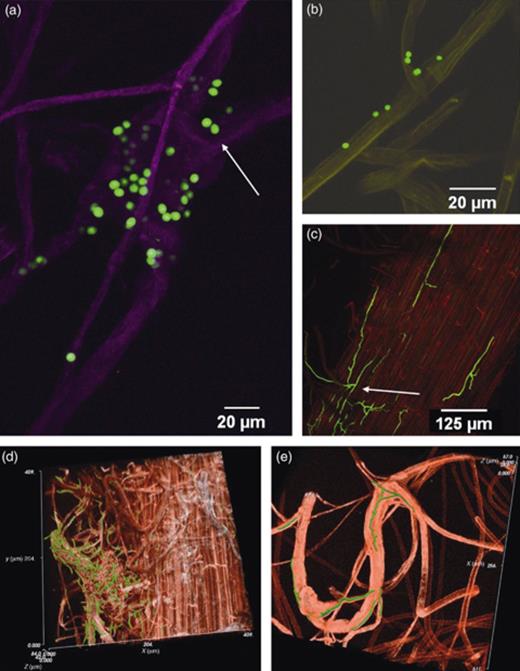
Characteristic growth of GFP-transformed Trichoderma gamsii AT1-2-4 and Trichoderma velutinum G1/8. Observations were performed using CLSM on 14-day-old sugar beet seedlings cultivated in sterile soil. (a) Spores of T. gamsii AT1-2-4 (green) were attached to root hairs/lateral roots (purple) and located in between aggregations of several root hairs. (b) Spores of T. velutinum G1/8 on sugar beet seedlings' root. (c) Characteristic growth of GFP-transformed T. velutinum G1/8. Mycelia growing on the surface of sugar beet roots and several hyphae were between the epidermis cells and following the cell borders. Single hypha leaves the network on the surface and growth together with the lateral root. (d) Fungal hyphae branched and were arranged in a net covering a thick lateral root at a high density. The confocal stacks of the CLSM pictures were imported in imagej and the brightness/contrast was equally adjusted in all optical slices. image surfer was then used to visualize the stacks using the ‘volume rendering’ and the ‘isosurface’ method. Red, root; green, hyphae. (e) A fungal hypha grew in parallel to the lateral root and branched out following the branches of the lateral roots.
On seedlings inoculated with conidia of T. velutinum G1/8, the nongerminated conidia were also found attached to roots and root hairs (Fig. 3b). The green fluorescent hyphae of the fungus were abundantly adhered to the roots' surface and the epidermis, where they had settled in the grooves, guiding the hyphal growth along the root cell direction (Fig. 3c). The analysis with image surfer showed three-dimensional images of T. velutinum G1/8 colonizing the root system of the sugar beet seedling. Extensive colonization by a dense network of hyphae was observed on the root surface. The hyphae also grew aligned to the main and lateral root surfaces, as well as to root hairs (Fig. 3d and e). Here, they established a strong contact with the epidermis.
In the coupled bacterium–fungus inoculation of sugar beet seeds using two strains in different arrangements, the same colonization behaviours were observed in each experiment as those found for single inoculations. In co-cultures of P. fluorescens L13-6-12 and T. velutinum G1/8, the bacterial cells and the fungal hyphae and conidia were found to be very close together on different parts of the root system. Nevertheless, there were also locations in which only one of the microorganisms was present. Similar to S. plymuthica 3Re4-18, the cells of P. fluorescens L13-6-12 were able to colonize the rhizosphere, where they formed loose aggregates (Fig. 2a). The root colonization pattern of S. plymuthica 3Re4-18 coinoculated with T. velutinum G1/8 was the same as that observed in the single-inoculation experiment. The bacterial cells, as well as the conidia, were loosely arranged around the root surface. The fungal hyphae were also found among single bacterial cells (Fig. 2b and c). With P. trivialis RE*1-1-14, T. velutinum G1/8 grew alongside the root hairs and branched toward adjacent hairs. The bacterial cells and the T. velutinum G1/8 hyphae were found to be close together physically and endophytic in the root system. The bacterial cells covered the entire rhizoplane and colonized the root hairs; especially, T. velutinum G1/8 hyphae grew in parallel to and on the root hairs (Fig. 2d).
Abundances of bacterial and fungal biocontrol strains on sugar beet seedlings
The ability of the BCAs to colonize the root and their interactions were assessed using the DsRed2- and GFP-tagged isolates on sugar beet root seedlings. The strains were reisolated on the selective media from roots grown in different bioassays: germination pouches (Table 2a) and sterile and nonsterile soil in pot experiments (Table 2b). Before sawing, each of the treated seeds harboured approximately log10 3.0 bacterial cells and/or conidia. After 14 days, different parts of the roots were analysed to determine the cell densities. In general, the bacterial abundances ranged from log10 3.3 to log10 8.4 depending on the root section and the substrate of cultivation. In germination pouches and sterile soil, the highest abundances were found for the upper parts of the root, but the number decreased along the root towards the root tips. On average, the abundances of the bacterial cells were one order of magnitude higher for the plants cultivated in pouches compared with cultivation in soil. In pair-inoculation T. velutinum G1/8 (conidia/mycelial fragments) reisolated from germination, pouches colonized the root in similar proportions along the entire root and ranged between log10 5.3 and log10 7.1 (Table 2a). The abundances of the two Pseudomonas strains were approximately one order of magnitude higher in co-culture with S. plymuthica 3Re4-18.
Population densities of bacterial BCAs and Trichoderma velutinum G1/8 in different parts of the sugar beet roots grown in germination pouches
BCA strains | Inoculum (log10) | Root section | Population densities (CFU g−1 root fresh weight) (log10) | ||
Bacteria (CFU per seed) | T. velutinum G1/8 (spores per seed) | Bacteria | T. velutinum G1/8 | ||
P. fluorescens L13-6-12 | 3.8 ± 1.1 | 3.8 ± 0.2 | Base | 8.4 ± 0.3 | 6.6 ± 0.1 |
Middle | 8.2 ± 0.5 | 6.6 ± 0.6 | |||
Root tip | 7.1 ± 0.7 | 5.7 ± 1.6 | |||
S. plymuthica 3Re4-18 | 4.7 ± 0.5 | 3.8 ± 0.2 | Base | 8.2 ± 0.2 | 5.8 ± 1.2 |
Middle | 8.0 ± 0.5 | 5.3 ± 0.7 | |||
Root tip | 7.9 ± 0.6 | 5.9 ± 0.8 | |||
P. trivialis RE*1-1-14 | 3.8 ± 0.2 | 3.8 ± 0.2 | Base | 7.1 ± 0.5 | 6.8 ± 0.4 |
Middle | 7.1 ± 0.4 | 7.1 ± 0.2 | |||
Root tip | 6.1 ± 0.6 | 5.6 ± 0.6 |
BCA strains | Inoculum (log10) | Root section | Population densities (CFU g−1 root fresh weight) (log10) | ||
Bacteria (CFU per seed) | T. velutinum G1/8 (spores per seed) | Bacteria | T. velutinum G1/8 | ||
P. fluorescens L13-6-12 | 3.8 ± 1.1 | 3.8 ± 0.2 | Base | 8.4 ± 0.3 | 6.6 ± 0.1 |
Middle | 8.2 ± 0.5 | 6.6 ± 0.6 | |||
Root tip | 7.1 ± 0.7 | 5.7 ± 1.6 | |||
S. plymuthica 3Re4-18 | 4.7 ± 0.5 | 3.8 ± 0.2 | Base | 8.2 ± 0.2 | 5.8 ± 1.2 |
Middle | 8.0 ± 0.5 | 5.3 ± 0.7 | |||
Root tip | 7.9 ± 0.6 | 5.9 ± 0.8 | |||
P. trivialis RE*1-1-14 | 3.8 ± 0.2 | 3.8 ± 0.2 | Base | 7.1 ± 0.5 | 6.8 ± 0.4 |
Middle | 7.1 ± 0.4 | 7.1 ± 0.2 | |||
Root tip | 6.1 ± 0.6 | 5.6 ± 0.6 |
DsRed2 transformants of Pseudomonas fluorescens L13-6-12, Pseudomonas trivialis RE*1-1-14, Serratia plymuthica 3Re4-18, and Trichoderma velutinum G1/8 were inoculated on sugar beet seeds and reisolated from different parts of the root. The total amount of recovered bacteria/conidia per plant was calculated as log10 CFU g−1 root fresh weight from each section. Confidence P≤0.05.
Population densities of bacterial BCAs and Trichoderma velutinum G1/8 in different parts of the sugar beet roots grown in germination pouches
BCA strains | Inoculum (log10) | Root section | Population densities (CFU g−1 root fresh weight) (log10) | ||
Bacteria (CFU per seed) | T. velutinum G1/8 (spores per seed) | Bacteria | T. velutinum G1/8 | ||
P. fluorescens L13-6-12 | 3.8 ± 1.1 | 3.8 ± 0.2 | Base | 8.4 ± 0.3 | 6.6 ± 0.1 |
Middle | 8.2 ± 0.5 | 6.6 ± 0.6 | |||
Root tip | 7.1 ± 0.7 | 5.7 ± 1.6 | |||
S. plymuthica 3Re4-18 | 4.7 ± 0.5 | 3.8 ± 0.2 | Base | 8.2 ± 0.2 | 5.8 ± 1.2 |
Middle | 8.0 ± 0.5 | 5.3 ± 0.7 | |||
Root tip | 7.9 ± 0.6 | 5.9 ± 0.8 | |||
P. trivialis RE*1-1-14 | 3.8 ± 0.2 | 3.8 ± 0.2 | Base | 7.1 ± 0.5 | 6.8 ± 0.4 |
Middle | 7.1 ± 0.4 | 7.1 ± 0.2 | |||
Root tip | 6.1 ± 0.6 | 5.6 ± 0.6 |
BCA strains | Inoculum (log10) | Root section | Population densities (CFU g−1 root fresh weight) (log10) | ||
Bacteria (CFU per seed) | T. velutinum G1/8 (spores per seed) | Bacteria | T. velutinum G1/8 | ||
P. fluorescens L13-6-12 | 3.8 ± 1.1 | 3.8 ± 0.2 | Base | 8.4 ± 0.3 | 6.6 ± 0.1 |
Middle | 8.2 ± 0.5 | 6.6 ± 0.6 | |||
Root tip | 7.1 ± 0.7 | 5.7 ± 1.6 | |||
S. plymuthica 3Re4-18 | 4.7 ± 0.5 | 3.8 ± 0.2 | Base | 8.2 ± 0.2 | 5.8 ± 1.2 |
Middle | 8.0 ± 0.5 | 5.3 ± 0.7 | |||
Root tip | 7.9 ± 0.6 | 5.9 ± 0.8 | |||
P. trivialis RE*1-1-14 | 3.8 ± 0.2 | 3.8 ± 0.2 | Base | 7.1 ± 0.5 | 6.8 ± 0.4 |
Middle | 7.1 ± 0.4 | 7.1 ± 0.2 | |||
Root tip | 6.1 ± 0.6 | 5.6 ± 0.6 |
DsRed2 transformants of Pseudomonas fluorescens L13-6-12, Pseudomonas trivialis RE*1-1-14, Serratia plymuthica 3Re4-18, and Trichoderma velutinum G1/8 were inoculated on sugar beet seeds and reisolated from different parts of the root. The total amount of recovered bacteria/conidia per plant was calculated as log10 CFU g−1 root fresh weight from each section. Confidence P≤0.05.
Population densities of bacterial BCAs in different parts of the sugar beet roots grown in pot experiments
BCA strains | Inoculum (CFU per seed) (log10) | Root section | Population densities (CFU g−1 root fresh weight) (log10) | |
Nonsterile soil | Sterile soil | |||
P. fluorescens L13-6-12 | 3.8 ± 1.1 | Base | 3.8 ± 0.9 | 6.3 ± 2.2 |
Middle | 4.5 ± 0.4 | 3.8 ± 0.8 | ||
Root tip | 4.6 ± 0.8 | 4.0 ± 0.7 | ||
S. plymuthica 3Re4-18 | 4.7 ± 0.5 | Base | 3.6 ± 1.2 | 6.4 ± 1.5 |
Middle | 3.3 ± 1.2 | 4.6 ± 0.5 | ||
Root tip | 4.2 ± 1.9 | 3.8 ± 0.2 | ||
P. trivialis RE*1-1-14 | 3.8 ± 0.2 | Base | 3.7 ± 1.3 | 6.6 ± 1.7 |
Middle | 3.8 ± 1.1 | 4.9 ± 0.5 | ||
Root tip | 3.9 ± 2.1 | 4.2 ± 0.7 |
BCA strains | Inoculum (CFU per seed) (log10) | Root section | Population densities (CFU g−1 root fresh weight) (log10) | |
Nonsterile soil | Sterile soil | |||
P. fluorescens L13-6-12 | 3.8 ± 1.1 | Base | 3.8 ± 0.9 | 6.3 ± 2.2 |
Middle | 4.5 ± 0.4 | 3.8 ± 0.8 | ||
Root tip | 4.6 ± 0.8 | 4.0 ± 0.7 | ||
S. plymuthica 3Re4-18 | 4.7 ± 0.5 | Base | 3.6 ± 1.2 | 6.4 ± 1.5 |
Middle | 3.3 ± 1.2 | 4.6 ± 0.5 | ||
Root tip | 4.2 ± 1.9 | 3.8 ± 0.2 | ||
P. trivialis RE*1-1-14 | 3.8 ± 0.2 | Base | 3.7 ± 1.3 | 6.6 ± 1.7 |
Middle | 3.8 ± 1.1 | 4.9 ± 0.5 | ||
Root tip | 3.9 ± 2.1 | 4.2 ± 0.7 |
DsRed2 transformants of Pseudomonas fluorescens L13-6-12, Pseudomonas trivialis RE*1-1-14, and Serratia plymuthica 3Re4-18 were inoculated on sugar beet seeds and reisolated from different parts of the root. The total amount of recovered bacteria per plant was calculated as log10 CFU g−1 root fresh weight from each section. Confidence P≤0.05.
Population densities of bacterial BCAs in different parts of the sugar beet roots grown in pot experiments
BCA strains | Inoculum (CFU per seed) (log10) | Root section | Population densities (CFU g−1 root fresh weight) (log10) | |
Nonsterile soil | Sterile soil | |||
P. fluorescens L13-6-12 | 3.8 ± 1.1 | Base | 3.8 ± 0.9 | 6.3 ± 2.2 |
Middle | 4.5 ± 0.4 | 3.8 ± 0.8 | ||
Root tip | 4.6 ± 0.8 | 4.0 ± 0.7 | ||
S. plymuthica 3Re4-18 | 4.7 ± 0.5 | Base | 3.6 ± 1.2 | 6.4 ± 1.5 |
Middle | 3.3 ± 1.2 | 4.6 ± 0.5 | ||
Root tip | 4.2 ± 1.9 | 3.8 ± 0.2 | ||
P. trivialis RE*1-1-14 | 3.8 ± 0.2 | Base | 3.7 ± 1.3 | 6.6 ± 1.7 |
Middle | 3.8 ± 1.1 | 4.9 ± 0.5 | ||
Root tip | 3.9 ± 2.1 | 4.2 ± 0.7 |
BCA strains | Inoculum (CFU per seed) (log10) | Root section | Population densities (CFU g−1 root fresh weight) (log10) | |
Nonsterile soil | Sterile soil | |||
P. fluorescens L13-6-12 | 3.8 ± 1.1 | Base | 3.8 ± 0.9 | 6.3 ± 2.2 |
Middle | 4.5 ± 0.4 | 3.8 ± 0.8 | ||
Root tip | 4.6 ± 0.8 | 4.0 ± 0.7 | ||
S. plymuthica 3Re4-18 | 4.7 ± 0.5 | Base | 3.6 ± 1.2 | 6.4 ± 1.5 |
Middle | 3.3 ± 1.2 | 4.6 ± 0.5 | ||
Root tip | 4.2 ± 1.9 | 3.8 ± 0.2 | ||
P. trivialis RE*1-1-14 | 3.8 ± 0.2 | Base | 3.7 ± 1.3 | 6.6 ± 1.7 |
Middle | 3.8 ± 1.1 | 4.9 ± 0.5 | ||
Root tip | 3.9 ± 2.1 | 4.2 ± 0.7 |
DsRed2 transformants of Pseudomonas fluorescens L13-6-12, Pseudomonas trivialis RE*1-1-14, and Serratia plymuthica 3Re4-18 were inoculated on sugar beet seeds and reisolated from different parts of the root. The total amount of recovered bacteria per plant was calculated as log10 CFU g−1 root fresh weight from each section. Confidence P≤0.05.
Discussion
Rhizosphere competence is a major factor for successful biocontrol approaches (Lugtenberg & Kamilova, 2009). In our study, we found a high rhizosphere competence for four of the selected five promising biocontrol strains. Only one strain, T. gamsii AT1-2-4, was not able to colonize the roots of sugar beet and showed no positive impact on plant growth. Rhizosphere competence was analysed by (1) monitoring the microbial abundances in different parts of the roots and by (2) analysing the colonization pattern of single and combined treatments. For both approaches, we used autofluorescent protein genes for the genetic transformation of microorganisms, which offer possibilities to study BCAs in their natural environment (Bloemberg, 2007). All transformations were successful, and we obtained fluorescent transformants similar to the wild-type strains in morphology and antagonistic traits. The vector pME6031-DsRed2 has a broad host range and it is suitable for the monitoring of rhizosphere gram-negative bacteria.
The bacterial strains, which we selected in previous studies (Faltin et al., 2004; Grosch et al., 2005a; Zachow et al., 2008), were found to be competent root colonizers. They were able to spread on the root system and to colonize different areas of the main and lateral roots as well as root hairs in high abundances in the germination pouch and soil. The abundance determined for each of the biocontrol strains was about log10 3.0 CFUs per seed. After germination of the seeds, the abundance of the bacteria increased rapidly by three to five orders of magnitude. Generally, in sterile soil and germination pouches, the highest bacterial abundances were found in the upper parts of the roots, where the lateral roots had increased the root surface. A similar gradient of colonization has been observed in the sugar beet rhizosphere for different rifampicin-resistant mutants of Arthrobacter histidinolovorans, Bacillus megaterium, Cytophaga johnsonae, P. fluorescens, and P. syringae by Walker et al. (2002). Schmidt et al. (2004) found that the density of the antagonistic P. fluorescens B5 colonizing sugar beet roots reached 105–106 CFUs per plant after 12 days of growth, independent of the inoculum concentration. In the pair bacterium–fungus inoculation, the abundances of T. velutinum G1/8 varied depending on the bacterial strain applied. However, along the root, the cell numbers of T. velutinum G1/8 were consistent and showed no statistically significant differences. In co-culture with the bacteria P. fluorescens L13-6-12 and P. trivialis RE*1-1-14, the cell numbers of T. velutinum G1/8 were almost the same in the first two upper parts and decreased by one order of magnitude on the root tip. In the co-cultures with S. plymuthica 3Re4-18, the T. velutinum G1/8 cell numbers were one order of magnitude lower than found in co-cultures with the two pseudomonads and showed a slight increase in abundance on the root tip. These differences could be explained by the fact that in in vitro dual cultures, S. plymuthica 3Re4-18 demonstrated antagonistic properties towards T. velutinum G1/8, while Pseudomonas had no negative impact on Trichoderma growth (C. Zachow, unpublished data). Interestingly, our microscopic monitoring revealed a coexistence of the bacterial cells and Trichoderma hyphae in different niches.
Although similar abundances were detected in the sugar beet root, we observed differences in the pattern and size of the aggregates they formed on the root. Both Pseudomonas strains, P. fluorescens L13-6-12 and P. trivialis RE*1-1-14, as well as S. plymuthica 3Re4-18 formed microcolonies on the root surface in the rhizoplane consisting of hundreds of cells. Analysis of the CLSM images revealed a large aggregation of bacterial cells and an endophytic lifestyle. Similar rhizosphere colonization was reported by Chin-A-Woeng et al. (1997): they found long-shaped microcolonies with a length of up to 200 μm for the biocontrol strain P. fluorescens WCS365 on the epidermis of tomato roots. They observed that almost all the cells of this strain and the entire root surface were enclosed in a matrix or covered by a semi-transparent film, which was absent in the seed coat. In this way, bacteria adhered to the root epidermis directly at their lateral sides colonizing the rhizoplane. Different from the two pseudomonads, S. plymuthica 3Re4-18 colonized the rhizosphere, but mainly single cells arranged in clouds were found; moreover, an endophytic life style was also observed.
Trichoderma spp. propagules or conidia applied on seeds or in soil come in contact with plant roots, germinate, and grow on root surfaces and some may infect the outer root cells (Harman et al., 2004). The green bright fluorescent conidia, germination tubes, and hyphae of the GFP-labelled strain T. velutinum G1/8 could be easily detected with CLSM in mono- and co-cultures. The hyphae were narrow between and on epidermal root cells and formed a net of hyphae covering the surface of sugar beet seedling. The colonization of the intercellular space of the epidermis was also found for Trichoderma harzianum GFP22 on tomato roots (Chacón et al., 2007). Furthermore, the hyphae of T. velutinum G1/8 aligned with lateral roots and root hairs. Three-dimensional analyses provided hints about the penetration of T. velutinum G1/8 into the root (data not shown). Assuming that Trichoderma did not behave as a ‘real’ endophyte, the penetration of the root tissue is usually restricted to the outer layer of roots (Harman et al., 2004). The green fluorescence of the GFP in conidia and hyphae was also visible in T. gamsii AT1-2-4 in culture media. However, when it was inoculated on sugar beet seeds, only the green fluorescent conidia were found on the rhizoplane, but no germinated conidia or hyphae could be detected. Similar behaviour was found for T. harzianum: in an assay for the study of niche competition at the root surface, using the GUS gene as a marker of the BCA (Green & Jensen, 1995). No hyphae of the T. harzianum strain were found on the rhizoplane, suggesting that the strain was possibly not rhizosphere competent. In the fungus–bacterium coinoculation we performed, the specific colonization pattern of each strain did not differ from those found in the single inoculation. Serratia plymuthica 3Re4-18 cells were in a loose arrangement in comparison with the tight microcolonies formed by two pseudomonads strains. No morphological interaction between the biocontrol strains was found in the microscopic analyses. Each strain occupied specific niches on the surface of the root, but they were in a close spatial arrangement to each other.
Beneficial plant-associated microorganisms have a direct positive influence on plant growth and can indirectly stimulate plant health by altering plant gene expression in roots, increasing the germination rates, root growth, yield, leaf area, tolerance to drought, shoot and root weights, and delaying leaf senescence (Berg, 2009; Lugtenberg & Kamilova, 2009). PGP has also been shown for different fungi, especially for Trichoderma spp. (Harman et al., 2004; Harman, 2006; Chacón et al., 2007). Several reports have shown that specific Trichoderma isolates occurring in the rhizosphere can result in PGP and has often been correlated with rhizosphere competence (Harman et al., 2004; Hoyos-Carvajal et al., 2009). In our study, rhizosphere competence was also correlated with the ability to promote plant growth. The highest effect of PGP was found for T. velutinum G1/8, which showed high abundances and a direct interaction with plant roots. In contrast, for T. gamsii AT1-2-4, which was not able to colonize the rhizosphere, no plant growth stimulation was found. For all three bacterial candidates, rhizosphere competence as well as PGP were found.
It is an old question as to whether autochthonous (from the same host plant) or allochthonous strains should be used for pathosystems in biocontrol strategies. While autochthonous strains are preferred from the ecological point of view, there are hints in the literature that allochthonous strains induce stronger effects in pathosystems (Berg et al., 2001). Furthermore, BCAs for commercialization are often selected according to their broad host range. Here, we used two strains from sugar beet: P. trivialis RE*1-1-14 and T. gamsii AT1-2-4 (autochthonous), and three strains from different hosts: S. plymuthica 3Re4-18 (potato endorhiza; Berg et al., 2005), P. fluorescens L13-6-12 (potato rhizosphere; Lottmann et al., 1999), and T. velutinum G1/8 (Rhizoctonia sclerotia on potato tuber; Grosch et al., 2006). With the exception of the noncolonizing sugar beet-associated strain T. gamsii AT1-2-4, all strains showed a similar competence in the sugar beet rhizosphere. Regarding PGP, the best strains belong to the allochthonous group. These results support the hypothesis of Berg et al. (2001), who evaluated potential biocontrol rhizobacteria of Verticillium dahliae from different host plants and found a better effect of allochthonous strains. The addition of R. solani to the analysis would be the next step. However, the anastomosis group AG2-2IIIB of R. solani causes late symptoms at harvest time and long-term field experiments are therefore necessary. Another important observation is that biocontrol strains with an endophytic life style can be good colonizers of the rhizosphere and endorhiza. This was postulated in different studies using endophytes in biocontrol approaches (reviewed in Berg & Hallmann, 2006) and could be confirmed in this study. All strains showed an endophytic behaviour in the root system.
Combining of strains in biocontrol approaches has a long history (Berg, 2009). Despite the many hurdles for the registration of such a product, in many cases, these combinations were extremely successful. The same was found for the BCAs selected for this study (G. Berg, unpublished data). Surprisingly, on analysing our results, the combined strains showed no morphological interaction in the rhizosphere or the endorhiza. The advantage can be that (1) they work under different environmental conditions, (2) both strains are able to induce resistance, which is a powerful mechanism in plant–microorganism interaction (Van Loon, 2007), and/or (3) they communicate with each other by N-acyl homoserine lactone-dependent or different quorum-sensing (QS) systems. Previous work has shown that especially the production of antagonistic compounds by rhizobacteria is often regulated by QS systems (Wood et al., 1997; Müller et al., 2009). The interaction between plant-associated microorganisms themselves as well as with their host plants is highly complex (Sørensen, 1997). Step-by-step identification of these complex networks will help to develop new strategies for plant protection and breeding.
Acknowledgements
We are very grateful for the technical support of Monika Schneider-Trampitsch, Barbara Fetz, and Bettina Bauer (Graz, Austria). We would like to thank Rita Grosch (Großbeeren, Germany) for the fruitful discussions about Rhizoctonia. Special thanks are due to the members of the MASE laboratorierna in Uppsala (Sweden) for valuable technical help during a research stay. We thank Christian P. Kubicek and his group (Vienna, Austria) for help with identification of the Trichoderma strains. The Austrian Science Fund FWF is acknowledged for financial support to G.B.
References
Author notes
Editor: Christoph Tebbe