-
PDF
- Split View
-
Views
-
Cite
Cite
Natarajan Rameshkumar, Sudha Nair, Isolation and molecular characterization of genetically diverse antagonistic, diazotrophic red-pigmented vibrios from different mangrove rhizospheres, FEMS Microbiology Ecology, Volume 67, Issue 3, May 2009, Pages 455–467, https://doi.org/10.1111/j.1574-6941.2008.00638.x
- Share Icon Share
Abstract
Genetic diversity of red-pigmented vibrios from different mangrove rhizospheres (Porteresia coarctata, Avicennia marina and Rhizophora mucronata) collected from Pichavaram mangrove, India was investigated. Twenty red–pink pigmented strains were isolated, 16S rRNA gene analyses indicted that these isolates belong to the genus Vibrio and were phylogenetically closely related to the type strains of Vibrio rhizosphaerae and Vibrio ruber. The rep-PCR analysis using GTG5 and BOX primers had similar groupings, and segregated these pigmented Vibrio isolates including two type strains into seven unique genotypic groups (REP groups A1–A7). The rhizosphere of P. coarctata harbors highly genetically diverse groups of red-pigmented vibrios compared to other plants. Multilocus sequence analysis using four genetic loci (pyrH, recA, rpoA, 16S rRNA) clearly supported the hypothesis that strains MSSRF38 (REP group A5) and MSSRF39 (REP group A6) could represent new Vibrio species. Biological functions of these vibrios were also determined and it was found that all these isolates have antagonistic activity against phytopathogens, and isolates belonging to REP groups A5 and A6 were positive for nifH gene by PCR. In conclusion, this study for the first time demonstrates the occurrence of genetically diverse groups of antagonistic, diazotrophic red-pigmented vibrios from different mangrove plants and suggests a new ecological role for vibrios as heterotrophic plant associated rhizobacteria.
Introduction
The genus Vibrio encompasses a diverse group of heterotrophic marine bacteria, currently including more than 70 recognized species (Sawabe et al., 2007), of which Vibrio gazogenes (Baumann et al., 1984), Vibrio ruber (Shieh et al., 2003) and Vibrio rhizosphaerae (Kumar & Nair, 2007) are the only known Vibrio species reported to produce red pigments. Unfortunately, their species description was on the basis of a very limited set of isolates (one or two) analysed and, consequently, their environmental distribution, function and ecology are poorly understood. It is well known from many studies on Vibrio ecology using culture-dependent and -independent methods that vibrios appear at particularly high densities in tissues and/or organs of various marine algae and animals, for example abalones, bivalves, corals, fish, shrimp, sponges, squid and zooplankton, where they act beneficially in host organisms as probionts or as facultative symbionts, or as pathogens (Thompson et al., 2004a).
To date, relatively few culture-based studies have shown an association of vibrios with plant rhizospheres, a very limited set of Vibrio isolates being recovered (Shieh et al., 1987; Bagwell et al., 1998; Bagwell & Lovell, 2000; Criminger et al., 2007). On the other hand, many studies confirm the ubiquity of vibrios in plant rhizospheres using culture-independent methods and these studies are mostly carried out in salt marsh rhizospheres, especially Spartina alterniflora (Lovell et al., 2000; Bergholz et al., 2001; Brown et al., 2003) and rarely in other plants (LaRocque et al., 2004; Flores-Mireles et al., 2007). The vibrios reported in these studies were Vibrio diazotrophicus, Vibrio parahaemolyticus, Vibrio anguillarum and Vibrio alginolyticus (Bagwell et al., 1998; Bagwell & Lovell, 2000; Lovell et al., 2000, 2001; LaRocque et al., 2004). However, the occurrence and genetic diversity of vibrios present in the rhizosphere of different mangrove plants (Porteresia coarctata, Avicennia marina and Rhizophora mucronata), especially red-pigmented vibrios and their biological functions, have not been reported.
The present study was a continuation of our previous work in which we isolated and characterized two novel red-pigmented Vibrio isolates, MSSRF3T and MSSRF10, from mangrove-associated wild rice (P. coarctata), which is of interest because both isolates were observed to have antagonistic activity against bacterial phytopathogens when tested. Phenotypic characterization, 16S rRNA gene sequence analysis, and DNA–DNA hybridization suggested that isolate MSSRF3T is a new bacterial species in the genus of Vibrio; its proposed name is V. rhizosphaerae sp. nov. (=LMG 23790T=DSM 18581T) (Kumar & Nair, 2007).
In this study we had three objectives: (1) To investigate the occurrence of these antagonistic red-pigmented vibrios in different mangrove rhizospheres, we selected P. coarctata (mangrove-associated plant), A. marina and R. mucronata (predominant mangrove plants) in Pichavaram mangrove. (2) To study the genetic diversity among these vibrios and identify possible novel genotypes using repetitive element primed-PCR (rep-PCR) analysis with GTG5 and BOX primers, and phylogenetic analysis we used four genetic loci (pyrH, recA, rpoA, 16S rRNA gene). (3) Biological functions such as antagonistic activity against phytopathogens and detection of nifH gene by PCR amplification of nifH gene were determined. The results of these studies are presented.
Materials and methods
Study area and sample collection
The study was conducted in Pichavaram mangroves on the southeast coast of India, situated about 250 km south of Chennai in March 2006. The geographical location was 11°27′N and 79°47′E. It contains about 25–30 ppt soil salinity and maintains a temperature around 28–32 °C. Porteresia coarctata (mangrove-associated plant), A. marina and R. mucronata (true mangrove plants) rhizosphere samples were collected carefully by uprooting the plants along with the soil adhering to the plant roots from Pichavaram mangrove, Tamil Nadu, India. Five rhizosphere samples were collected for each plant. The samples were placed in sterile polyethylene bags, properly labeled, transported to the lab and stored at 4 °C until isolation of bacterial strains.
Isolation
The rhizosphere soil samples were obtained by shaking the roots gently to remove loosely attached soil. The roots with tightly adhering soil were then grouped into three samples, each comprising five root systems and 1 g of soil adhering to the roots (rhizosphere sample) of each sample was then put in a 50-mL test tube containing 10 mL sterile water and vortexed vigorously for 10 min. The resulting solution containing the rhizosphere bacteria was serially diluted, 100-μL aliquots was taken from each dilution and plated onto half-strength trypticase soy agar (TSA, Himedia, India) supplemented with 2% NaCl (TSA+NaCl) and incubated for 3–4 days at room temperature. Red–pink-pigmented colonies appearing on half-strength trypticase soy agar (TSA+NaCl) plates were purified on PYG+NaCl medium (1% peptone, 0.5% yeast extract, 1% glucose, 2% NaCl and 1.8% agar, pH 7.2) and selected for further studies.
Genomic DNA isolation
The genomic DNA was extracted from all 20 pigmented isolates including two type strains V. rhizosphaerae LMG23790T and V. ruber JCM11486T as previously described (Ausubel et al., 1987).
Genomic fingerprinting
The rep-PCR fingerprinting using GTG5 and BOX primers was performed as described previously (Ben-Haim et al., 2003). The primers and the amplification cycling conditions for the rep-PCRs are listed in Table 1. The rep-PCR was carried out in 25 μL reaction volumes. Each reaction mixture contained 2.5 μL of 10 × PCR buffer, 2 μL of 2 mM dNTP mix, 2 μL of primer (30 ng), 0.3 μL of Taq DNA polymerase (3 U μL−1), 2 μL of template DNA (100–200 ng) and made up to final volume of 25 μL using double-sterilized distilled water. The PCR products were separated by electrophoresis of 10 μL of the reaction mixtures in ethidium bromide-stained 1.5% agarose gels in 0.5 × TBE buffer at a constant 40 V voltage for 14 h. A 1-kb DNA ladder (Biotools) was used as a DNA size standard and loaded in the first and sixth lanes. The rep-PCR profiles were visualized under UV light, and the gel images were captured using the gel documentation system (Bio-Rad). Normalization, recognition, and assignment of bands on the gel were performed with the software program fingerprinting ii (Bio-Rad), using the Dice coefficient and the unweighted-pair group method with averages for generation of dendrograms. Strains that had PCR-generated DNA banding patterns with more than one band difference in terms of size or intensity were considered distinct types. Vibrio rhizosphaerae LMG 23790T and V. ruber JCM 11486T were used as reference Vibrio strains (both are red-pigmented vibrios) for this study.
The primer sequences and the amplification cycling conditions used in rep-PCR genomic fingerprinting
Sl. no. | Primer | Nucleotide sequence | Amplification cycling conditions |
1 | (GTG)5 | 5′-GTGGTGGTGGTGGTG-3′ | Initial denaturation 95°C for 2 min; 32 cycles of 94°C for 30 s, 47°C for 1 min, and 65°C for 8 min. Final extension at 68°C for 8 min; final 4°C soak |
2 | BOX | 5′-ACGGCAAGGCGACGCTGACG-3′ | Initial denaturation 95°C for 2 min, 94°C for 3 s; 30 cycles of 92°C for 30 s, 50°C for 1 min, and 65°C for 8 min. Final extension at 68°C for 8 min; final 4°C soak |
Sl. no. | Primer | Nucleotide sequence | Amplification cycling conditions |
1 | (GTG)5 | 5′-GTGGTGGTGGTGGTG-3′ | Initial denaturation 95°C for 2 min; 32 cycles of 94°C for 30 s, 47°C for 1 min, and 65°C for 8 min. Final extension at 68°C for 8 min; final 4°C soak |
2 | BOX | 5′-ACGGCAAGGCGACGCTGACG-3′ | Initial denaturation 95°C for 2 min, 94°C for 3 s; 30 cycles of 92°C for 30 s, 50°C for 1 min, and 65°C for 8 min. Final extension at 68°C for 8 min; final 4°C soak |
The primer sequences and the amplification cycling conditions used in rep-PCR genomic fingerprinting
Sl. no. | Primer | Nucleotide sequence | Amplification cycling conditions |
1 | (GTG)5 | 5′-GTGGTGGTGGTGGTG-3′ | Initial denaturation 95°C for 2 min; 32 cycles of 94°C for 30 s, 47°C for 1 min, and 65°C for 8 min. Final extension at 68°C for 8 min; final 4°C soak |
2 | BOX | 5′-ACGGCAAGGCGACGCTGACG-3′ | Initial denaturation 95°C for 2 min, 94°C for 3 s; 30 cycles of 92°C for 30 s, 50°C for 1 min, and 65°C for 8 min. Final extension at 68°C for 8 min; final 4°C soak |
Sl. no. | Primer | Nucleotide sequence | Amplification cycling conditions |
1 | (GTG)5 | 5′-GTGGTGGTGGTGGTG-3′ | Initial denaturation 95°C for 2 min; 32 cycles of 94°C for 30 s, 47°C for 1 min, and 65°C for 8 min. Final extension at 68°C for 8 min; final 4°C soak |
2 | BOX | 5′-ACGGCAAGGCGACGCTGACG-3′ | Initial denaturation 95°C for 2 min, 94°C for 3 s; 30 cycles of 92°C for 30 s, 50°C for 1 min, and 65°C for 8 min. Final extension at 68°C for 8 min; final 4°C soak |
Multilocus sequence and phylogenetic analysis using 16S rRNA, pyrH, recA and rpoA genes
The 16S rRNA gene was amplified and sequenced as described previously by Saha (2005), and the recA, rpoA and pyrH genes as described by Thompson (2005). The sequences of the primers for 16S rRNA, recA, rpoA and pyrH genes used for amplification and sequencing are listed in Table 2. For all the above mentioned genes, PCR amplification was carried out in a total volume of 20 μL containing 2 μL of template DNA (50–100 ng), 0.3 μL of Taq-DNA polymerase (3 U μL−1), 2 μL of 10 × Taq buffer, 2 μL of 2 mM dNTP mix, 2 μL of forward and reverse primer (each 30 ng μL−1) and made up to final volume of 20 μL using double-sterilized distilled water. Amplification was carried out in a MJ Research minicycler. In all the reactions, water was used in place of DNA as a negative control. The correct amplified DNA fragments were separated on a 1% agarose gel and purified using Eppendorf Perfectprep Gel Cleanup Kit (according to the manufacturer's instructions). The purified PCR product was used directly for DNA sequencing using the dideoxy chain termination method with Big Dye Terminator kit (Applied Biosystems). The primers used for sequencing are listed in Table 2. The reaction products were analysed using capillary electrophoresis on an ABI 310 Genetic Analyzer (Applied Biosystems).
Amplification and sequencing primers for 16S rRNA genes (Saha et al., 2005), rpoA, recA, and pyrH (Thompson et al., 2005)
Gene | Primer | Sequence (5′→3′) | Position | PCR conditions |
16S rRNA | fD1 | AGTTTGATCCTGGCTCAG | 26 | Initial denaturation at 95°C for 4 min followed by 30 cycles of denaturation at 95°C for 1 min, annealing at 58°C for 1 min and elongation at 72°C for 1 min and final extension at 72°C for 10 min |
rP2 | ACGGCTACCTTGTTACGACTT | 1494 | ||
357f | CTCCTACGGGAGGCAGCAG | 357 | ||
704f | CTAGCGGTGAAATGCGTAGA | 704 | ||
907r | CCGTCAATTCCTTTGAGTTT | 907 | ||
1220r | TTGTAGCACGTGTGTAGCCC | 1220 | ||
pyrH | pyrH-02-R | GTRAABGCNGMYARRTCCA | 599 | Initial denaturation at 95°C for 4 min followed by 30 cycles of denaturation at 95°C for 1 min, annealing at 52°C for 1 min and elongation at 72°C for 1 min and final extension at 72°C for 10 min |
pyrH-04-F | ATGASNACBAAYCCWAAACC | 1 | ||
rpoA | rpoA-01-F | ATGCAGGGTTCTGTDACAG | 1 | Initial denaturation at 95°C for 4 min followed by 30 cycles of denaturation at 95°C for 1 min, annealing at 55°C for 1 min and elongation at 72°C for 1 min and final extension at 72°C for 10 min |
rpoA-03-R | GHGGCCARTTTTCHARRCGC | 951 | ||
rpoA-05-F | GCAGCDCGTGTWGARCARCG | 568 | ||
rpoA-06-R | CGYTGYTCWACACGHGCTGC | 568 | ||
recA | recA-01-F | TGARAARCARTTYGGTAAAGG | 222 | Initial denaturation at 95°C for 4 min followed by 30 cycles of denaturation at 95°C for 1 min, annealing at 56°C for 1 min and elongation at 72°C for 1 min and final extension at 72°C for 10 min |
recA-02-R | TCRCCNTTRTAGCTRTACC | 1040 | ||
recA-03-F | TYGGBGTGATGTTYGGTAACC | 767 |
Gene | Primer | Sequence (5′→3′) | Position | PCR conditions |
16S rRNA | fD1 | AGTTTGATCCTGGCTCAG | 26 | Initial denaturation at 95°C for 4 min followed by 30 cycles of denaturation at 95°C for 1 min, annealing at 58°C for 1 min and elongation at 72°C for 1 min and final extension at 72°C for 10 min |
rP2 | ACGGCTACCTTGTTACGACTT | 1494 | ||
357f | CTCCTACGGGAGGCAGCAG | 357 | ||
704f | CTAGCGGTGAAATGCGTAGA | 704 | ||
907r | CCGTCAATTCCTTTGAGTTT | 907 | ||
1220r | TTGTAGCACGTGTGTAGCCC | 1220 | ||
pyrH | pyrH-02-R | GTRAABGCNGMYARRTCCA | 599 | Initial denaturation at 95°C for 4 min followed by 30 cycles of denaturation at 95°C for 1 min, annealing at 52°C for 1 min and elongation at 72°C for 1 min and final extension at 72°C for 10 min |
pyrH-04-F | ATGASNACBAAYCCWAAACC | 1 | ||
rpoA | rpoA-01-F | ATGCAGGGTTCTGTDACAG | 1 | Initial denaturation at 95°C for 4 min followed by 30 cycles of denaturation at 95°C for 1 min, annealing at 55°C for 1 min and elongation at 72°C for 1 min and final extension at 72°C for 10 min |
rpoA-03-R | GHGGCCARTTTTCHARRCGC | 951 | ||
rpoA-05-F | GCAGCDCGTGTWGARCARCG | 568 | ||
rpoA-06-R | CGYTGYTCWACACGHGCTGC | 568 | ||
recA | recA-01-F | TGARAARCARTTYGGTAAAGG | 222 | Initial denaturation at 95°C for 4 min followed by 30 cycles of denaturation at 95°C for 1 min, annealing at 56°C for 1 min and elongation at 72°C for 1 min and final extension at 72°C for 10 min |
recA-02-R | TCRCCNTTRTAGCTRTACC | 1040 | ||
recA-03-F | TYGGBGTGATGTTYGGTAACC | 767 |
Amplification and sequencing primers for 16S rRNA genes (Saha et al., 2005), rpoA, recA, and pyrH (Thompson et al., 2005)
Gene | Primer | Sequence (5′→3′) | Position | PCR conditions |
16S rRNA | fD1 | AGTTTGATCCTGGCTCAG | 26 | Initial denaturation at 95°C for 4 min followed by 30 cycles of denaturation at 95°C for 1 min, annealing at 58°C for 1 min and elongation at 72°C for 1 min and final extension at 72°C for 10 min |
rP2 | ACGGCTACCTTGTTACGACTT | 1494 | ||
357f | CTCCTACGGGAGGCAGCAG | 357 | ||
704f | CTAGCGGTGAAATGCGTAGA | 704 | ||
907r | CCGTCAATTCCTTTGAGTTT | 907 | ||
1220r | TTGTAGCACGTGTGTAGCCC | 1220 | ||
pyrH | pyrH-02-R | GTRAABGCNGMYARRTCCA | 599 | Initial denaturation at 95°C for 4 min followed by 30 cycles of denaturation at 95°C for 1 min, annealing at 52°C for 1 min and elongation at 72°C for 1 min and final extension at 72°C for 10 min |
pyrH-04-F | ATGASNACBAAYCCWAAACC | 1 | ||
rpoA | rpoA-01-F | ATGCAGGGTTCTGTDACAG | 1 | Initial denaturation at 95°C for 4 min followed by 30 cycles of denaturation at 95°C for 1 min, annealing at 55°C for 1 min and elongation at 72°C for 1 min and final extension at 72°C for 10 min |
rpoA-03-R | GHGGCCARTTTTCHARRCGC | 951 | ||
rpoA-05-F | GCAGCDCGTGTWGARCARCG | 568 | ||
rpoA-06-R | CGYTGYTCWACACGHGCTGC | 568 | ||
recA | recA-01-F | TGARAARCARTTYGGTAAAGG | 222 | Initial denaturation at 95°C for 4 min followed by 30 cycles of denaturation at 95°C for 1 min, annealing at 56°C for 1 min and elongation at 72°C for 1 min and final extension at 72°C for 10 min |
recA-02-R | TCRCCNTTRTAGCTRTACC | 1040 | ||
recA-03-F | TYGGBGTGATGTTYGGTAACC | 767 |
Gene | Primer | Sequence (5′→3′) | Position | PCR conditions |
16S rRNA | fD1 | AGTTTGATCCTGGCTCAG | 26 | Initial denaturation at 95°C for 4 min followed by 30 cycles of denaturation at 95°C for 1 min, annealing at 58°C for 1 min and elongation at 72°C for 1 min and final extension at 72°C for 10 min |
rP2 | ACGGCTACCTTGTTACGACTT | 1494 | ||
357f | CTCCTACGGGAGGCAGCAG | 357 | ||
704f | CTAGCGGTGAAATGCGTAGA | 704 | ||
907r | CCGTCAATTCCTTTGAGTTT | 907 | ||
1220r | TTGTAGCACGTGTGTAGCCC | 1220 | ||
pyrH | pyrH-02-R | GTRAABGCNGMYARRTCCA | 599 | Initial denaturation at 95°C for 4 min followed by 30 cycles of denaturation at 95°C for 1 min, annealing at 52°C for 1 min and elongation at 72°C for 1 min and final extension at 72°C for 10 min |
pyrH-04-F | ATGASNACBAAYCCWAAACC | 1 | ||
rpoA | rpoA-01-F | ATGCAGGGTTCTGTDACAG | 1 | Initial denaturation at 95°C for 4 min followed by 30 cycles of denaturation at 95°C for 1 min, annealing at 55°C for 1 min and elongation at 72°C for 1 min and final extension at 72°C for 10 min |
rpoA-03-R | GHGGCCARTTTTCHARRCGC | 951 | ||
rpoA-05-F | GCAGCDCGTGTWGARCARCG | 568 | ||
rpoA-06-R | CGYTGYTCWACACGHGCTGC | 568 | ||
recA | recA-01-F | TGARAARCARTTYGGTAAAGG | 222 | Initial denaturation at 95°C for 4 min followed by 30 cycles of denaturation at 95°C for 1 min, annealing at 56°C for 1 min and elongation at 72°C for 1 min and final extension at 72°C for 10 min |
recA-02-R | TCRCCNTTRTAGCTRTACC | 1040 | ||
recA-03-F | TYGGBGTGATGTTYGGTAACC | 767 |
The sequences of these genes were compared with the sequences available from GenBank using the blast program (Altschul et al., 1990), Ribosomal Database Project II (Cole et al., 2005) and Eztaxon databases (Chun et al., 2007). Then they were aligned using clustal w software (Thompson et al., 1994). The concatenated sequences represented 80% of the full-length 16S rRNA gene, and 71%, 78% and 85% of the coding region for recA, rpoA and pyrH, respectively. Distances were calculated according to Kimura's two-parameter correction (Kimura, 1980). Phylogenetic trees were inferred using the neighbour-joining method (Saitou & Nei, 1987). Bootstrap analysis was based on 1000 resamplings. The mega3 package (Kumar et al., 2004) was used for all analyses.
nifH amplification and sequencing
The nifH gene was amplified by PCR using primers 19F (5′-GCIWTYTAYGGIAARGGIGG-3′) and 407R (5′-AAICCRCCRCAIACIACRTC-3′) as described by Ueda (1995). PCR amplification was carried out in a total volume of 20 μL containing 2 μL of template DNA (50–100 ng), 0.3 μL of Taq-DNA polymerase (3 U μL−1), 2 μL of 10 × Taq buffer, 2 μL of 2 mM dNTP mix, 2 μL of forward and reverse primer (each 30 ng μL−1) and made up to final volume of 20 μL using double-sterilized distilled water. Amplification was carried out in a MJ Research minicycler. PCR conditions were as follows: initial denaturation at 95 °C for 4 min followed by 30 cycles of denaturation at 95 °C for 1 min, annealing at 50 °C for 1 min and elongation at 72 °C for 1 min and final extension at 72 °C for 10 min. In all the reactions, water was used in place of DNA as a negative control. The correct amplified DNA fragments were separated on a 1% agarose gel and purified using Eppendorf Perfectprep Gel Cleanup Kit (according to the manufacturer's instructions). The purified fragment was used directly for DNA sequencing with the dideoxy chain termination method with the BigDye terminator kit (Applied Biosystems) using the above mentioned nifH primers, and the reaction products were analysed by capillary electrophoresis on an ABI 310 Genetic Analyzer (Applied Biosystems). For phylogenetic analysis, partial nifH nucleotide sequences were aligned, and a neighbour-joining phylogeny was constructed in the mega3 version using pairwise deletion of gaps and missing data and Kimura's two-parameter correction (Kumar et al., 2004).
In vitro antagonism against phytopathogens
The antagonistic activities of 20 pigmented Vibrio isolates against bacterial phytopathogens were assayed on TSB (Himedia) containing 1.8% (w/v) agar (TSA) by Spot-on-lawn assay as described (Kumar & Nair, 2007). Briefly, the bacterial phytopathogen Xanthomonas oryzae (a rice pathogen known to cause bacterial blight), was grown separately in TSB for 72 h at 28 °C. From this culture, 100 μL (at OD600 nm, c. 106 CFU mL−1) was pipetted onto (TSA) plates and spread plated. After 3 h drying at room temperature, 5 μL of a 24-h culture of pigmented Vibrio isolates were spotted on the target organism lawn, and the spots allowed to dry. These plates were incubated at 28 °C and examined every day for the appearance of zones of inhibition of microbial growth. Activity was measured as the diameter (in millimeters) of the zones of inhibition.
In vitro antagonism against fungal plant pathogens was assessed on potato dextrose agar [PDA; 20% (w/v) peeled potato, 2% (w/v) glucose, 1.8% (w/v) agar] supplemented with 0.5% (w/v) NaCl (PDA+NaCl). Briefly, strains of Fusarium oxysporum (known to cause wilt disease in plants) and Magnaporthe grisea (a rice pathogen known to cause blast disease) were grown on the PDA medium for 72 h before the test. A loopful of pigmented Vibrio isolates grown overnight was streaked onto one side of each PDA+NaCl plate (pH 7.0) and incubated at 28 °C for 48 h. Then, a 0.45-cm-diameter agar plug of fungal mycelium was transferred onto the center of the other side of each PDA+NaCl plate. Fungal plugs were also placed on uninoculated PDA+NaCl plates separately as uninhibited controls. Cultures were incubated at 28 °C for 2–5 days, and the plates were examined for inhibition of growth.
Phenotypic characterization
The cells of all red-pigmented Vibrio strains were grown in TSB+NaCl for 24 h and were examined for both morphology and motility using a phase contrast microscope. Classical phenotypic tests were performed as described previously (Baumann et al., 1984). In vitro pigment analysis using a spectrophotometer was performed as described (Shieh et al., 2003). Growth in thiosulfate citrate bile salts sucrose (TCBS) agar (Oxoid, Merck, Eiken) was monitored for 2 days of incubation at 28 °C. Vibrio rhizosphaerae LMG 7894T and V. ruber LMG 7910Twere used as references.
Nucleotide sequence accession numbers
Sequences of 16S rRNA gene
Strains MSSRF2 (EU295424), MSSRF3T (DQ847123), MSSRF5 (EU295429), MSSRF6 (EU295430), MSSRF7 (DQ847122), MSSRF8 (EU295425), MSSRF9 (EU295426), MSSRF10 (DQ273663), MSSRF11 (EU295436), MSSRF12 (EU295431), MSSRF13 (EU295432), MSSRF14 (EU295437), MSSRF18 (EU295434), MSSRF22 (EU295427), MSSRF24 (EU295435), MSSRF28 (EU295428), MSSRF29 (EU295438), MSSRF32 (EU295439), MSSRF33 (EU295433), MSSRF38 (EU144014), MSSRF39 (EU314670).
Sequences of recA
Strains MSSRF28 (EU295441), MSSRF3T (EF523232), MSSRF6 (EU295442), MSSRF7 (EU295445), MSSRF10 (EF523239), MSSRF38 (EU144016), MSSRF39 (EU314672), and V. ruber VR1T (EF523236).
Sequences of rpoA
Strains MSSRF28 (EU295440), MSSRF3T (EF523233), MSSRF6 (EU295443), MSSRF10 (EF523240), MSSRF7 (EU295444), MSSRF38 (EU144015), MSSRF39 (EU314671), and V. ruber VR1T (EF523235).
Sequences of pyrH
Strains MSSRF28 (EU426843), MSSRF3T (EF523234), MSSRF6 (EU620233), MSSRF10 (EF523238), MSSRF7 (EU426844), MSSRF38 (EU620231), MSSRF39 (EU620232) and V. ruber VR1T (EF523237).
Sequences of nifH
Strains MSSRF38 (EU155079) and MSSRF39 (EU620234).
Results and discussion
Isolation and identification
A total of 20 red–pink pigmented isolates were isolated from the rhizosphere region of three different mangrove plants collected from Pichavaram mangrove, Tamil Nadu, India (Table 3). Based on the heterotrophic plate count, the total population of culturable aerobic bacteria and red-pigmented Vibrio strains associated with the rhizosphere was 6 × 105 and 8 × 102 CFU g−1 soil (P. coarctata), 4 × 105 and 5 × 102 CFU g−1 soil (A. marina), 3.6 × 105 and 2 × 102 CFU g−1 soil (R. mucronata), respectively. Notably, we could observe different pigmented colonies appearing during isolation; on repeated subculturing these colonies retained their original colony colour and morphology (data not shown). The taxonomic identities of these 20 red–pink pigmented isolates were determined initially based on partial 16S rRNA gene sequencing. Comparison of these 16S rRNA gene sequences with the databases (see Materials and methods) enabled us to assign each isolate to a taxonomic group at the family or genus level. These results indicate that all the pigmented isolates studied are members of the genus Vibrio and formed close relationships with species known to produce red pigments. Vibrio rhizosphaerae LMG 23790T (Kumar & Nair, 2007) and V. ruber JCM 11486T (Shieh et al., 2003) shared 98.3–99.5% sequence similarity and moderate sequence similarities (93.0–95.0%), which were found towards other selected species of the genus Vibrio. A neighbour-joining tree showing this relationship is shown in Fig. 1.
Genotype group | Strain | Plant source | Colony colour | Presence of nifH gene | Activity against | References | ||
Xanthomonas oryzae | Magnaporthe grisea | Fusarium oxysporum | ||||||
A1 | MSSRF2 | Porteresia coarctata | RS | − | ++ | +++ | +++ | This study |
MSSRF8 | Porteresia coarctata | RS | − | +++ | +++ | +++ | This study | |
MSSRF9 | Avicennia marina | LRF | − | + | ++++ | +++ | This study | |
MSSRF22 | Rhizophora mucronata | RS | − | ++ | ++ | ++ | This study | |
MSSRF28 | Rhizophora mucronata | PR | − | +++ | +++ | ++ | This study | |
A2 | MSSRF5 | Avicennia marina | RS | − | + | +++ | ++ | This study |
MSSRF6 | Porteresia coarctata | RP | − | +++ | ++++ | ++++ | This study | |
MSSRF12 | Porteresia coarctata | RP | − | ++ | ++ | +++ | This study | |
MSSRF13 | Avicennia marina | PR | − | + | +++ | +++ | This study | |
MSSRF18 | Avicennia marina | RP | − | + | ++ | ++ | This study | |
MSSRF24 | Rhizophora mucronata | RP | − | ++ | ++++ | +++ | This study | |
MSSRF33 | Rhizophora mucronata | RS | − | +++ | ++ | +++ | This study | |
Vibrio rhizosphaerae MSSRF3T | Porteresia coarctata | RP | − | +++ | ++++ | +++ | Kumar & Nair (2007) | |
A3 | MSSRF10 | Porteresia coarctata | DRM | − | ++++ | +++ | ++ | Kumar & Nair (2007) |
MSSRF11 | Avicennia marina | RS | − | ++ | ++ | ++ | This study | |
MSSRF14 | Porteresia coarctata | DRM | − | + | ++ | ++ | This study | |
A4 | MSSRF7 | Porteresia coarctata | PR | − | +++ | ++ | ++ | This study |
A5 | MSSRF38 | Porteresia coarctata | LRF | + | ++ | +++ | ++ | This study |
MSSRF29 | Porteresia coarctata | RS | + | + | ++ | ++ | This study | |
MSSRF32 | Porteresia coarctata | LRF | + | + | ++ | + | This study | |
A6 | MSSRF39 | Porteresia coarctata | LRF | + | ++ | ++ | ++ | This study |
A7 | Vibrio ruber JCM11486T | Sea sediment | DRF | Not tested | Not tested | Not tested | Not tested | Shieh (2003 |
Genotype group | Strain | Plant source | Colony colour | Presence of nifH gene | Activity against | References | ||
Xanthomonas oryzae | Magnaporthe grisea | Fusarium oxysporum | ||||||
A1 | MSSRF2 | Porteresia coarctata | RS | − | ++ | +++ | +++ | This study |
MSSRF8 | Porteresia coarctata | RS | − | +++ | +++ | +++ | This study | |
MSSRF9 | Avicennia marina | LRF | − | + | ++++ | +++ | This study | |
MSSRF22 | Rhizophora mucronata | RS | − | ++ | ++ | ++ | This study | |
MSSRF28 | Rhizophora mucronata | PR | − | +++ | +++ | ++ | This study | |
A2 | MSSRF5 | Avicennia marina | RS | − | + | +++ | ++ | This study |
MSSRF6 | Porteresia coarctata | RP | − | +++ | ++++ | ++++ | This study | |
MSSRF12 | Porteresia coarctata | RP | − | ++ | ++ | +++ | This study | |
MSSRF13 | Avicennia marina | PR | − | + | +++ | +++ | This study | |
MSSRF18 | Avicennia marina | RP | − | + | ++ | ++ | This study | |
MSSRF24 | Rhizophora mucronata | RP | − | ++ | ++++ | +++ | This study | |
MSSRF33 | Rhizophora mucronata | RS | − | +++ | ++ | +++ | This study | |
Vibrio rhizosphaerae MSSRF3T | Porteresia coarctata | RP | − | +++ | ++++ | +++ | Kumar & Nair (2007) | |
A3 | MSSRF10 | Porteresia coarctata | DRM | − | ++++ | +++ | ++ | Kumar & Nair (2007) |
MSSRF11 | Avicennia marina | RS | − | ++ | ++ | ++ | This study | |
MSSRF14 | Porteresia coarctata | DRM | − | + | ++ | ++ | This study | |
A4 | MSSRF7 | Porteresia coarctata | PR | − | +++ | ++ | ++ | This study |
A5 | MSSRF38 | Porteresia coarctata | LRF | + | ++ | +++ | ++ | This study |
MSSRF29 | Porteresia coarctata | RS | + | + | ++ | ++ | This study | |
MSSRF32 | Porteresia coarctata | LRF | + | + | ++ | + | This study | |
A6 | MSSRF39 | Porteresia coarctata | LRF | + | ++ | ++ | ++ | This study |
A7 | Vibrio ruber JCM11486T | Sea sediment | DRF | Not tested | Not tested | Not tested | Not tested | Shieh (2003 |
Grouping was done based on rep-PCR analysis using GTG5 and BOX primers.
Colony morphologies of pigmented vibrios after 48 h of incubation at 28°C on PYG+NaCl agar plates: RS, red smooth round colony; RP, red–pink smooth round colony; DRM, dark red mucoid colony; PR, pink–rose smooth round colony; LRF, light red flat smooth colony; DRF, dark red flat smooth round colony.
Detection of nifH gene in pigmented vibrios by PCR amplification and sequencing of nifH primers (Ueda et al., 1995).
Antagonism towards these three phytopathogens was determined as mentioned in Materials and methods. Results indicate the zone of inhibition as follows: +, 0–5 mm; ++, 5–10 mm; +++, 10–15 mm; ++++, >15 mm.
Genotype group | Strain | Plant source | Colony colour | Presence of nifH gene | Activity against | References | ||
Xanthomonas oryzae | Magnaporthe grisea | Fusarium oxysporum | ||||||
A1 | MSSRF2 | Porteresia coarctata | RS | − | ++ | +++ | +++ | This study |
MSSRF8 | Porteresia coarctata | RS | − | +++ | +++ | +++ | This study | |
MSSRF9 | Avicennia marina | LRF | − | + | ++++ | +++ | This study | |
MSSRF22 | Rhizophora mucronata | RS | − | ++ | ++ | ++ | This study | |
MSSRF28 | Rhizophora mucronata | PR | − | +++ | +++ | ++ | This study | |
A2 | MSSRF5 | Avicennia marina | RS | − | + | +++ | ++ | This study |
MSSRF6 | Porteresia coarctata | RP | − | +++ | ++++ | ++++ | This study | |
MSSRF12 | Porteresia coarctata | RP | − | ++ | ++ | +++ | This study | |
MSSRF13 | Avicennia marina | PR | − | + | +++ | +++ | This study | |
MSSRF18 | Avicennia marina | RP | − | + | ++ | ++ | This study | |
MSSRF24 | Rhizophora mucronata | RP | − | ++ | ++++ | +++ | This study | |
MSSRF33 | Rhizophora mucronata | RS | − | +++ | ++ | +++ | This study | |
Vibrio rhizosphaerae MSSRF3T | Porteresia coarctata | RP | − | +++ | ++++ | +++ | Kumar & Nair (2007) | |
A3 | MSSRF10 | Porteresia coarctata | DRM | − | ++++ | +++ | ++ | Kumar & Nair (2007) |
MSSRF11 | Avicennia marina | RS | − | ++ | ++ | ++ | This study | |
MSSRF14 | Porteresia coarctata | DRM | − | + | ++ | ++ | This study | |
A4 | MSSRF7 | Porteresia coarctata | PR | − | +++ | ++ | ++ | This study |
A5 | MSSRF38 | Porteresia coarctata | LRF | + | ++ | +++ | ++ | This study |
MSSRF29 | Porteresia coarctata | RS | + | + | ++ | ++ | This study | |
MSSRF32 | Porteresia coarctata | LRF | + | + | ++ | + | This study | |
A6 | MSSRF39 | Porteresia coarctata | LRF | + | ++ | ++ | ++ | This study |
A7 | Vibrio ruber JCM11486T | Sea sediment | DRF | Not tested | Not tested | Not tested | Not tested | Shieh (2003 |
Genotype group | Strain | Plant source | Colony colour | Presence of nifH gene | Activity against | References | ||
Xanthomonas oryzae | Magnaporthe grisea | Fusarium oxysporum | ||||||
A1 | MSSRF2 | Porteresia coarctata | RS | − | ++ | +++ | +++ | This study |
MSSRF8 | Porteresia coarctata | RS | − | +++ | +++ | +++ | This study | |
MSSRF9 | Avicennia marina | LRF | − | + | ++++ | +++ | This study | |
MSSRF22 | Rhizophora mucronata | RS | − | ++ | ++ | ++ | This study | |
MSSRF28 | Rhizophora mucronata | PR | − | +++ | +++ | ++ | This study | |
A2 | MSSRF5 | Avicennia marina | RS | − | + | +++ | ++ | This study |
MSSRF6 | Porteresia coarctata | RP | − | +++ | ++++ | ++++ | This study | |
MSSRF12 | Porteresia coarctata | RP | − | ++ | ++ | +++ | This study | |
MSSRF13 | Avicennia marina | PR | − | + | +++ | +++ | This study | |
MSSRF18 | Avicennia marina | RP | − | + | ++ | ++ | This study | |
MSSRF24 | Rhizophora mucronata | RP | − | ++ | ++++ | +++ | This study | |
MSSRF33 | Rhizophora mucronata | RS | − | +++ | ++ | +++ | This study | |
Vibrio rhizosphaerae MSSRF3T | Porteresia coarctata | RP | − | +++ | ++++ | +++ | Kumar & Nair (2007) | |
A3 | MSSRF10 | Porteresia coarctata | DRM | − | ++++ | +++ | ++ | Kumar & Nair (2007) |
MSSRF11 | Avicennia marina | RS | − | ++ | ++ | ++ | This study | |
MSSRF14 | Porteresia coarctata | DRM | − | + | ++ | ++ | This study | |
A4 | MSSRF7 | Porteresia coarctata | PR | − | +++ | ++ | ++ | This study |
A5 | MSSRF38 | Porteresia coarctata | LRF | + | ++ | +++ | ++ | This study |
MSSRF29 | Porteresia coarctata | RS | + | + | ++ | ++ | This study | |
MSSRF32 | Porteresia coarctata | LRF | + | + | ++ | + | This study | |
A6 | MSSRF39 | Porteresia coarctata | LRF | + | ++ | ++ | ++ | This study |
A7 | Vibrio ruber JCM11486T | Sea sediment | DRF | Not tested | Not tested | Not tested | Not tested | Shieh (2003 |
Grouping was done based on rep-PCR analysis using GTG5 and BOX primers.
Colony morphologies of pigmented vibrios after 48 h of incubation at 28°C on PYG+NaCl agar plates: RS, red smooth round colony; RP, red–pink smooth round colony; DRM, dark red mucoid colony; PR, pink–rose smooth round colony; LRF, light red flat smooth colony; DRF, dark red flat smooth round colony.
Detection of nifH gene in pigmented vibrios by PCR amplification and sequencing of nifH primers (Ueda et al., 1995).
Antagonism towards these three phytopathogens was determined as mentioned in Materials and methods. Results indicate the zone of inhibition as follows: +, 0–5 mm; ++, 5–10 mm; +++, 10–15 mm; ++++, >15 mm.
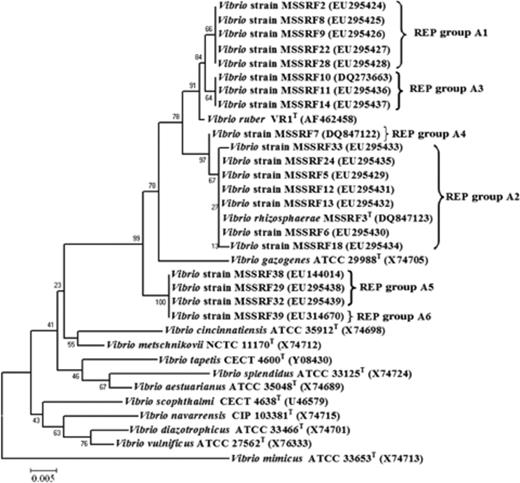
Neighbour-joining tree based on partial 16S rRNA gene sequences of red-pigmented Vibrio strains and other closely related Vibrio strains. The evolutionary distances were computed using the Kimura two-parameter method and are in units of the number of base substitutions per site. All positions containing gaps and missing data were eliminated from the dataset (complete deletion option). There were a total of 527 positions in the final dataset.
Phenotypic analysis
All the pigmented isolates had the main phenotypic features of the genus Vibrio– straight to slightly curved rods, motile, facultative anaerobe, gram-negative, catalase-positive, no growth in the absence of NaCl and stimulation of growth by NaCl, indicating that all these pigmented strains were probably a species of Vibrio in the family Vibrionaceae (Baumann et al., 1984). All strains produced nondiffusible, cellular red pigments, regardless of the presence of light. Acetone/methanol extracts of the red pigments of all isolates showed maximal absorption at about 535 nm, which is identical to the absorption spectrum of prodigiosin (Shieh et al., 2003). It is worth noting that these Vibrio strains possess some phenotypic characteristics quite uncommon among the vibrios, including production of gas from glucose, negative for oxidase, resistance to O/129, and no growth in TCBS agar. Furthermore, these pigmented Vibrio strains were phenotypically very similar to each other and to V. rhizosphaerae LMG 23790T and V. ruber LMG 23124T. It was found that several vibrios have nearly similar phenotypic features (Thompson et al., 2004a) and the techniques that are essential for reliable species identification in the genus Vibrio were based on genomic data (Thompson et al., 2004a).
Genomic fingerprinting
The genetic diversity among 20 red–pink pigmented strains of the genus Vibrio, including two type strains V. rhizosphaerae LMG 23790T and V. ruber JCM 11486T, were determined. The rep-PCR technique using GTG5 and BOX primers was chosen because these techniques are simple, can differentiate between closely related strains of bacteria, and can assign bacteria potentially up to the strain level (Versalovic et al., 1991; Rademaker et al., 1998). Moreover, it has been shown to be a useful technique in the subtyping of Vibrio species (Sawabe et al., 2002; Thompson et al., 2004a; Kumar & Nair, 2007). The rep-PCR analysis using GTG5 and BOX primers had similar groupings, and segregated the 20 red–pink pigmented Vibrio strains including two type strains into seven unique genotypic groups (REP groups A1–A7) (Table 3, Fig. 2). The cluster analyses of the Rep profiles of all isolates and their groups are shown in Fig. 3. The two largest REP groups, REP groups A1 (five isolates) and A2 (seven isolates), were found in all rhizospheres of plants studied (Table 3), indicating these groups may represent predominant strains. However, some genotypes were found only in some plants and not in others. For instance, REP group A3 (three isolates) was found in P. coarctata and A. marina but not found in R. mucronata, whereas REP groups A4 (one isolate), A5 (three isolates) and A6 (one isolate) were found only in P. coarctata (Table 3). Moreover, multiple genotypic groups were obtained from rhizospheres of P. coarctata (REP groups A1–A6), A. marina (REP groups A1–A3) and R. mucronata (REP groups A1 and A2). This seems to reflect that there is more than one strain growing in the rhizospheres of all mangrove plants. In addition, the rhizosphere of P. coarctata harbours highly genetically diverse groups of red-pigmented vibrios compared with other plants. The probable explanation for these findings might be differences in root exudation released in rhizosphere of different plants and abiotic environmental parameters, where several studies have indicated that structural and functional diversities of microorganisms in the rhizosphere differ among plant species (Brimecombe et al., 2001; LaRocque et al., 2004). Moreover, it shows the substantial capability of these red-pigmented Vibrio isolates for colonizing various mangrove plants. However, no correlation was made between the isolates and their source as we found that similar genotypes (REP groups A1 and A2) were obtained in all plants (Table 3). However, some REP groups (A4, A5, A6) were found only in P. coarctata as these REP groups represent small numbers of strains (<4 strains), so we cannot come to a direct conclusion that these genotypes are plant specific. Similarly, no correlation was made between the genotypes and their colony colour (Table 3).
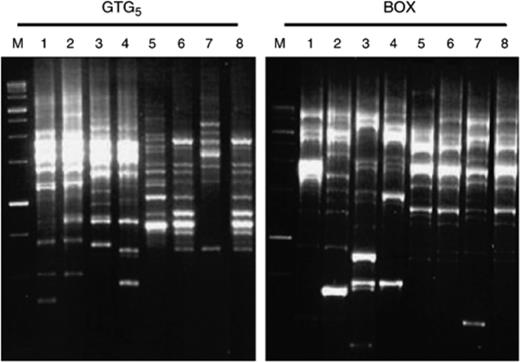
Representative Rep-PCR profiles of red-pigmented Vibrio isolates. Lane M, 1-kb ladder; lane 1, isolates MSSRF28 (REP group A1); lane 2, MSSRF10 (REP group A3); lane 3, MSSRF38 (REP group A5); lane 4, MSSRF39 (REP group A6); lane 5, MSSRF7 (REP group A4); lane 6, MSSRF6 (REP group A2); lane 7, Vibrio ruber JCM 11486T (REP group A7); lane 8, Vibrio rhizosphaerae LMG 23790T (REP group A2).
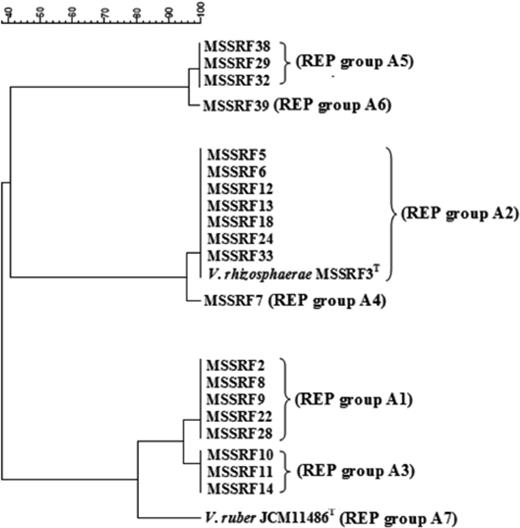
Composite dendrogram generated from data for rep-PCR profiles of all red-pigmented Vibrio strains. Vibrio ruber JCM 11486T and Vibrio rhizosphaerae LMG 23790T were used as reference strains.
It is worth noting that the first isolate of V. rhizosphaerae, including the type strain MSSRF3T, was recovered from the rhizosphere of P. coarctata (Kumar & Nair, 2007). In the present study, the isolates of this species were recovered from the rhizospheres of A. marina and R. mucronata, indicating that V. rhizosphaerae is a widely distributed strain in all mangrove rhizospheres studied. This is supported by the fact that the banding patterns of REP group A2 (the largest REP group) are identical to those of type strain V. rhizosphaerae LMG 23790T (Table 3, Figs 2 and 3). However, none of the isolates showed identical patterns to that of type strain V. ruber JCM 11486T (Table 3, Figs 2 and 3). Several studies on the genetic diversity of vibrios have been reported from different sources, for example, fish, mollusc, shrimp, rotifers, artemia, and their aquaculture water (Thompson et al., 2001, 2004a), but not from studies on plant rhizosphere. However, most studies on Vibrio ecology to date have focused on specific members of the genus (especially pathogenic Vibrio strains), leading to an extensive body of literature on their genetics and ecology. Nevertheless, the diversity and dynamics of co-occurring Vibrio populations have rarely been addressed (Rehnstam et al., 1993; Caldini et al., 1997; Barbieri et al., 1999; Eilers et al., 2000a, b; Heidelberg et al., 2002a, b; Thompson et al., 2004b) and more rarely still using quantitative culture-independent methods (Rehnstam et al., 1993; Eilers et al., 2000a; Heidelberg et al., 2002a, b). This is the first report of the genetic diversity of red-pigmented vibrios in different mangrove rhizospheres. Furthermore, we also report the occurrence of four more new genotypes in addition to the one reported in our previous paper (Kumar & Nair, 2007). This reflects a high degree of genetic diversity among the red-pigmented Vibrio strains isolated from different mangrove rhizospheres.
Sequence analysis of housekeeping genes using pyrH, recA and rpoA genes
Although the 16S rRNA gene is generally used as a framework for modern bacterial classification, it often shows limited variation for the discrimination of closely related taxa and several vibrios have nearly identical 16S rRNA gene sequences (Thompson et al., 2005). Protein-coding genes exhibit a higher genetic variation, which can be used for the classification and identification of closely related taxa (Thompson et al., 2005). Sequencing of the pyrH, recA and rpoA genes has been found to be useful in discriminating species of the closely related Vibrio species (Thompson et al., 2005; Kumar & Nair, 2007). The pyrH, recA and rpoA gene sequence analysis of representative isolates of each genotype (Table 4) obtained from molecular typing studies also supported the phylogenetic position of each isolate within the genus Vibrio, which were phylogenetically closely related to the type strains of V. rhizosphaerae and V. ruber (Fig. 4). Pairwise sequence similarities (pyrH, recA and rpoA genes) of representative isolates of each genotype with the type strains of V. rhizosphaerae, V. ruber, and V. gazogenes are given in Table 4. The remaining species of the genus Vibrio showed <92.3% sequence similarity with these three genes.
Results of pairwise sequence similarities (pyrH, recA and rpoA genes) of representative isolates of each genotype with the type strains of Vibrio rhizosphaerae LMG23790T, Vibrio ruber JCM 11486T, and Vibrio gazogenes ATCC 29988T
Type strains.
†Respective REP groups (identified by rep-PCR analysis).
Results of pairwise sequence similarities (pyrH, recA and rpoA genes) of representative isolates of each genotype with the type strains of Vibrio rhizosphaerae LMG23790T, Vibrio ruber JCM 11486T, and Vibrio gazogenes ATCC 29988T
Type strains.
†Respective REP groups (identified by rep-PCR analysis).
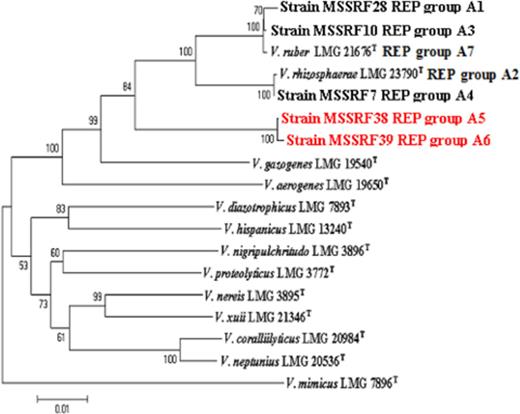
Neighbour-joining phylogeny of concatenated sequences of 16S rRNA, pyrH, recA, and rpoA genes from species of Vibrio. A neighbour-joining tree was constructed using Kimura's two-parameter correction. Bootstrap values represent 1000 replications.
Based on the study of Thompson (2005), strains of the same Vibrio species have at least 98%, 94% and 94%rpoA, pyrH and recA gene sequence similarity, respectively. But in this study we found that isolates MSSRF38 (REP group A5) and MSSRF39 (REP group A6) showed <98%, 94% and 94%rpoA, pyrH and recA gene sequence similarity with the type strains of V. rhizosphaerae, V. gazogenes and V. ruber (Table 4) and with the remaining species of the genus Vibrio. However, they shared 100% sequence similarity between each other with rpoA, pyrH and recA genes, respectively, indicating that they are conspecific. Furthermore, phylogenetic analysis of the four genetic loci (pyrH, recA, rpoA, 16S rRNA gene) showed evidence that strains MSSRF38 (REP group A5) and MSSRF39 (REP group A6) formed a distinct group outward from the V. rhizosphaerae and V. ruber group (Fig. 4), which probably indicates a new species in the genus Vibrio, especially in the gazogenes clade as described by Sawabe (2007). Further studies on DNA–DNA hybridization will have to be carried out to come to a conclusion on the taxonomic status of the Vibrio strains MSSRF38 (REP group A5) and MSSRF39 (REP group A6). Although several studies have shown that Vibrio strains which have <98%, 94% and 94%rpoA, pyrH and recA gene sequence similarity will have <70% DNA–DNA similarity (Thompson et al., 2004a, 2005; Kumar & Nair, 2007); a threshold value of 70% DNA–DNA similarity is recommended for bacterial species definition (Wayne et al., 1987).
Biological functions
Antagonistic activity against phytopathogens
In our earlier study we found that two red-pigmented vibrios have antimicrobial properties (Kumar & Nair, 2007). Therefore, we tested these properties in the present study. Our results demonstrate that all red-pigmented Vibrio species studied here were capable of inhibiting both bacterial and fungal phytopathogens (Table 3). The antimicrobial activity of all strains did not require the presence of living cells, because antimicrobial activity was demonstrated by filter-sterilized cell-free culture supernatants, indicating that the antimicrobial compound produced by the strains was extracellular (data not shown). There are several reports pertaining to the production of inhibitory substances by vibrios (Sugita et al., 1999; Long & Azam, 2001; Hjelm et al., 2004). Most of these vibrios were isolated from the surface or gastrointestinal tract of fish or fish larvae or shrimp, where they have been evaluated in vitro or in vivo for their potential to inhibit fish-pathogenic organisms and to overcome infections in fish and larvae in aquaculture (Irianto & Austin, 2002; Gram & Ringø, 2005). However, there have been no reports from mangrove rhizosphere and, in addition, antifungal activity was observed in these pigmented Vibrio isolates, which was not reported in any vibrios earlier (Sugita et al., 1999; Long & Azam, 2001; Hjelm et al., 2004).
PCR amplifications of nifH genes
Several studies have shown that diazotrophic activity in salt marsh ecosystems is closely associated with plant roots (Bagwell et al., 1998; Bagwell & Lovell, 2000; Lovell et al., 2000; LaRocque et al., 2004). As all the isolates studied here are from rhizosphere, the strains were subjected to an nifH-specific PCR amplification using the primers of Ueda (1995); the expected 390-bp amplification product was observed in only four isolates (MSSRF38, MSSRF29, MSSRF32 and MSSRF39), whereas negative results were observed in all isolates (data not shown). The amplified 390-bp fragment of strains MSSRF38 (EU155079) and MSSRF39 (EU620234) was then sequenced and the strains showed 100% sequence similarity with each other and <83.0% sequence similarity with V. parahaemolyticus, Vibrio porteresiae, V. diazotrophicus and Vibrio natriegens. However, similarities with the other nifH sequences from the NCBI database were 76.0–90.0%. Phylogenetic analysis showed a clear affiliation of the MSSRF38 and MSSRF39 nifH sequences with sequences from other N2-fixing Vibrio species and also clearly differentiated the MSSRF38 and MSSRF39 nifH sequences from those of other N2-fixing species (Fig. 5).
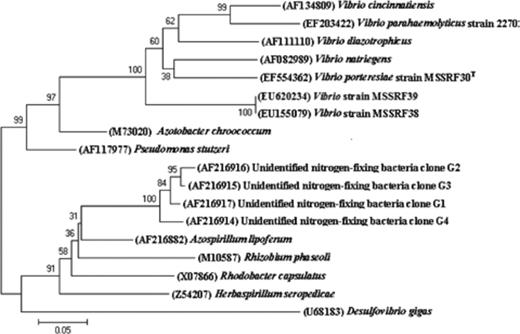
Neighbour-joining tree based on partial nifH gene sequences of strains MSSRF38 and MSSRF39 and other nifH sequences of related organisms and environmental clones.
It has been observed that nitrogen fixation is not a common property among the known species of the genus Vibrio. The Vibrio species known to fix atmospheric N2 are V. diazotrophicus, V. natriagens, Vibrio cincinnatiansis (Guerinot & Patriquin, 1981; Urdaci et al., 1988), V. parahaemolyticus (Criminger et al., 2007) and V. porteresiae (Rameshkumar et al., 2008). However, many culture-dependent and -independent studies on diazotrophs of S. alterniflora, Juncus roemerianus, and Salicornia virginica have shown that vibrios are abundant on the roots and may play a substantial role in N2 fixation in the salt marsh ecosystem. Most of the vibrios reported in these studies are V. diazotrophicus, V. parahaemolyticus, V. anguillarum and V. alginolyticus (Bagwell et al., 1998; Bagwell & Lovell, 2000; Lovell et al., 2000, 2001; LaRocque et al., 2004). However, this study has isolated red-pigmented Vibrio strains MSSRF38 and MSSRF39, positive for nifH gene, which has not been reported in any study (Bagwell et al., 1998; Bagwell & Lovell, 2000; Lovell et al., 2000, 2001; LaRocque et al., 2004), and this actually illustrates the abundance of uncharacterized diazotrophic Vibrio species associated with different mangrove plant rhizospheres.
In conclusion, to the best of our knowledge this is the first report showing the occurrence, functionality and genetic diversity of red-pigmented vibrios colonizing the rhizosphere of different mangrove plants (P. coarctata, A. marina and R. mucronata). We provide strong evidence that some of the strains may represent new species in the genus Vibrio. Thus, understanding the taxonomy and ecological distribution of Vibrio species associated with previously untested plants or from new environments may lead to identification of novel Vibrio strains and this will help in understanding the ecological role of these vibrios in their natural habitats. The limited number of pigmented Vibrio strains observed in this study may be due to the isolation medium, which may not be selective enough to support the growth of these pigmented vibrios when compared with other rhizosphere bacterial populations. Moreover, studies have shown that culture-based recovery of isolates may underestimate the environmental populations and do not assess the nonculturable fractions of the rhizosphere soil microbiota (van Elsas et al., 1998). Hence, further studies have been undertaken to determine the relative abundance and distribution of these red-pigmented vibrios in the rhizosphere of different mangrove plants by designing specific primers for 16S rRNA and recA genes obtained from this study and perform a culture-independent method as described (Muyzer & Smalla, 1998). This would allow analysis of the diversity and ecological distribution of Vibrio species associated with different mangrove plants and to enhance knowledge in the field of Vibrio biology with respect to Vibrio–plant symbiotic interactions.
Acknowledgements
This work was carried out with financial support from the Department of Biotechnology, Government of India. The authors are grateful to the two reviewers and the editor for their valuable comments for improving the manuscript. We are grateful to Dr Alavandi and N. Dinesh Kumar (senior research fellow), Central Institute of Brackish Aquaculture (CIBA), Chennai, for their lab support in cluster analysis.
References
Author notes
Editor: Kornelia Smalla