-
PDF
- Split View
-
Views
-
Cite
Cite
Breugelmans Philip, Pieter-Jan D'Huys, René De Mot, Dirk Springael, Characterization of novel linuron-mineralizing bacterial consortia enriched from long-term linuron-treated agricultural soils, FEMS Microbiology Ecology, Volume 62, Issue 3, December 2007, Pages 374–385, https://doi.org/10.1111/j.1574-6941.2007.00391.x
- Share Icon Share
Abstract
Linuron-mineralizing cultures were enriched from two linuron-treated agricultural soils in the presence and absence of a solid support. The cultures contained linuron-degrading bacteria, which coexisted with bacteria degrading either 3,4-dichloroaniline (3,4-DCA) or N, O-dimethylhydroxylamine (N, O-DMHA), two common metabolites in the linuron degradation pathway. For one soil, the presence of a solid support enriched for linuron-degrading strains phylogenetically related to but different from those enriched without support. Most linuron-degrading consortium members were identified as Variovorax, but a Hydrogenophaga and an Achromobacter strain capable of linuron degradation were also obtained. Several of the linuron-degrading isolates also degraded 3,4-DCA. Isolates that degraded 3,4-DCA but not linuron belonged to the genera Variovorax, Cupriavidus and Afipia. Hyphomicrobium spp. were involved in the metabolism of N, O-DMHA. Whereas several isolates degraded linuron independently, more efficient degradation was achieved by combining linuron and 3,4-DCA-degraders or by adding casamino acids. These data suggest that (1) linuron degradation is performed by a group of metabolically interacting bacteria rather than by individual strains, (2) there are other genera in addition to Variovorax that degrade linuron beyond 3,4-DCA, (3) linuron-degrading consortia of different origins have a similar composition, and (4) interactions between consortium members can be complex and can involve exchange of both metabolites and other nutrients.
Introduction
Since their commercial introduction about 50 years ago, phenylurea herbicides have become one of the most widely used herbicides to control germinating and emerging grasses and broad-leafed weeds in orchards, tree nurseries and crop areas (U.S. EPA, 1995). Linuron [3-(3,4-dichlorophenyl)-1-methoxy-1-methyl urea] is a selective N-methoxy-N-methyl substituted phenylurea that is considered to be moderately to highly toxic to aquatic organisms (Orme & Kegley, 2006). In addition, linuron is suspected to act as an androgen receptor antagonist affecting the male reproductive system, and is therefore also of concern for human health (McIntyre et al., 2002). Although linuron is moderately persistent and relatively immobile in soil, runoff and leaching can result in the migration of the compound to surface- and ground-water bodies, with attendant environmental risks (U.S. EPA, 1995). Therefore, an understanding of the natural attenuation of linuron in the environment is important. Under field conditions, the half-life of linuron varies from 30 to 150 days for different soil types, with an estimated average half-life of c. 60 days (U.S. EPA, 1984).
The main route for the removal of linuron in the environment is biodegradation. Bacterial degradation of linuron involves a stepwise transformation that starts with a direct hydrolysis resulting in the formation of 3,4-dichloroaniline (3,4-DCA) and N, O-dimethylhydroxylamine (N, O-DMHA). Both 3,4-DCA and N, O-DMHA can be further mineralized. At present, only a few reports exist on the isolation and characterization of bacteria involved in linuron degradation. Bacillus sphaericus ATCC12123 and Arthrobacter globiformis D47 transform linuron into 3,4-DCA and N, O-DMHA (Engelhardt et al., 1971; Cullington & Walker, 1999). Complete mineralization of linuron including the mineralization of 3,4-DCA and N, O-DMHA has only recently been reported, by Dejonghe et al. (2003) and Sørensen et al. (2005), and these studies show contrasting findings. Dejonghe et al. (2003) showed that a synergistic consortium was involved in complete linuron mineralization. In this consortium, Variovorax sp. WDL1 is the primary linuron degrader and is responsible for the transformation of linuron into 3,4-DCA and N, O-DMHA. Based on pure cultures, although the strain is able to also metabolize 3,4-DCA, transient accumulation of 3,4-DCA resulted apparently in ineffective linuron degradation. Efficient linuron degradation by Variovorax sp. WDL1 in the consortium was shown to rely on coexisting members, i.e. the 3,4-DCA degraders Delftia acidovorans WDL34 and Comamonas testosteroni WDL7, and the N, O-DMHA degrader Hyphomicrobium sulfonivorans WDL6. In contrast, Sørensen et al. (2005) concluded that a single organism, Variovorax sp. SRS16, mineralized linuron independently of other bacteria. This strain is closely related to Variovorax sp. WDL1, and it was suggested that only members of this genus are able to mineralize linuron. In this study, novel linuron-fed enrichments from two long-term linuron-treated agricultural soils were initiated to investigate (1) whether linuron in soil is commonly degraded by a synergistic consortium rather than by a single strain, (2) whether complete linuron degradation is indeed a property unique to Variovorax spp., and (3) whether linuron-degrading consortia from different origins have similar community compositions. In addition, in analogy with a previous study on the enrichment of polycyclic aromatic hydrocarbon-degrading bacteria (Bastiaens et al., 2000), the impact of the presence of a solid matrix on the composition of the enriched communities was studied.
Materials and methods
Soils
Topsoil samples BEH and BEW were collected in Belgium from two agricultural fields in April 2005. The Danish soil DKE was kindly provided by S.R. Sørensen from the same field from which the linuron-mineralizing strain Variovorax sp. SRS16 was isolated (Sørensen et al., 2005). Soils BEH and DKE are both from agricultural fields that have been treated on a long-term basis with linuron, whereas soil BEW has not received pesticide treatment in the last 25 years. All soils were sieved (<2 mm) and stored in the dark at 4 °C. Table 1 gives an overview of the determined soil properties. Particle size distribution was determined according to ISO 11277: 1998(E) guidelines. Soil pH was measured in a 0.01 M CaCl2 solution (soil/liquid ratio of 1 : 2.5), and total carbon and nitrogen contents were determined after dry combustion with a VarioMAX CN analyser (Elementar Analysensysteme, Hanau, Germany).
Soil | Texture | pH | Moisture content (%) | Total carbon (%) | Total nitrogen (%) |
DKE | Sandy | 5.9 | 19.3 | 1.4 | 0.06 |
BEH | Sandy loam | 4.9 | 19.0 | 0.8 | 0.06 |
BEW | Sandy | 4.5 | 9.9 | 2.3 | 0.21 |
Soil | Texture | pH | Moisture content (%) | Total carbon (%) | Total nitrogen (%) |
DKE | Sandy | 5.9 | 19.3 | 1.4 | 0.06 |
BEH | Sandy loam | 4.9 | 19.0 | 0.8 | 0.06 |
BEW | Sandy | 4.5 | 9.9 | 2.3 | 0.21 |
Soil | Texture | pH | Moisture content (%) | Total carbon (%) | Total nitrogen (%) |
DKE | Sandy | 5.9 | 19.3 | 1.4 | 0.06 |
BEH | Sandy loam | 4.9 | 19.0 | 0.8 | 0.06 |
BEW | Sandy | 4.5 | 9.9 | 2.3 | 0.21 |
Soil | Texture | pH | Moisture content (%) | Total carbon (%) | Total nitrogen (%) |
DKE | Sandy | 5.9 | 19.3 | 1.4 | 0.06 |
BEH | Sandy loam | 4.9 | 19.0 | 0.8 | 0.06 |
BEW | Sandy | 4.5 | 9.9 | 2.3 | 0.21 |
Chemicals
Linuron [3-(3,4-dichlorophenyl)-1-methoxy-1-methyl urea] (99.5%), diuron [3-(3,4-dichlorophenyl)-1,1-dimethyl urea] (99.5%), isoproturon [(3-(4-isopropylphenyl)-1,1-dimethyl urea) (99.0%), metobromuron [3-(4-bromophenyl)-1-methoxy-1-methyl urea] (99.9%) and 3,4-dichloroaniline (99.3%) were purchased from Sigma Aldrich, and N, O-dimethylhydroxylamine (98%) was purchased from Alfa Aesar. [phenyl-U-14C]Linuron (16.93 mCi mmol−1, radio-chemical purity >95%) was obtained from Izotop.
Media
Mineral media MMO and MMN were prepared as described previously (Dejonghe et al., 2003). Medium MMN was derived from medium MMO with the elimination of all nitrogen. For mineralization experiments, the pH of medium MMN was adjusted to 5.8 to allow the formation of volatile CO2. R2A (0.5 g tryptone, 0.5 g yeast extract, 0.5 g casein hydrolysate, 0.5 g glucose d+, 0.5 g soluble starch, 0.3 g sodium pyruvate, 0.3 g KH2PO4 and 0.05 g MgSO4 L−1 distilled water; pH 7.0) was used as heterotrophic medium. When appropriate, linuron, diuron, isoproturon, metobromuron, 3,4-DCA or N, O-DMHA was added at a concentration ranging from 25 to 200 mg L−1. Solid media were obtained by adding 15 g agar L−1 (Select Agar, Invitrogen). All glassware and media were sterilized by autoclaving (121 °C, 15 min).
Enrichment and isolation procedure
Enrichment cultures from the two linuron-treated soils (BEH and DKE) were initiated with 2.5 g of sieved soil (<2 mm) in 25 mL of MMN with linuron (25 mg L−1) as the only source of carbon and nitrogen, and incubated at 25 °C on a rotary shaker (120 r.p.m.). Soil particles present in these cultures served as a solid support for sessile growth. Sterile controls of soils BEH and DKE were incubated simultaneously to monitor abiotic losses of linuron during the enrichment. No abiotic losses of linuron were recorded during the incubation period. Linuron concentrations were monitored with HPLC, and when the concentration of linuron in the medium was below the detection limit (0.5 mg L−1), 50% of the volume of the culture was removed after settlement of the soil particles and replaced with fresh medium containing 50 mg L−1 linuron. After three such refreshments, the concentration of linuron was increased by adding linuron crystals in a final concentration of 100 mg L−1 each time the medium was replaced.
Linuron-fed enrichment cultures without solid support were set up from these cultures after the medium had been refreshed five times. Therefore, 250 μL of the cultures was transferred to 25 mL of MMN supplemented with 25 mg L−1 linuron. To make sure that all soil particles were removed, the cultures were transferred in the same way three times when the linuron concentration had decreased below the detection limit. Then, 50% of the volume of the cultures was replaced with fresh medium, and linuron crystals (100 mg L−1) were added. All linuron-fed enrichment cultures were enriched for 20 weeks (corresponding to 10 medium refreshments) before plating.
Subcultures fed with 3,4-DCA (25 mg L−1) and N, O-DMHA (200 mg L−1) as the sole source of carbon and nitrogen were set up from the linuron-based enrichment cultures without solid support that had been transferred three times. Therefore, 250 μL of the linuron-based enrichments was transferred to 25 mL of 3,4-DCA or N, O-DMHA-containing MMN medium. The 3,4-DCA-fed cultures were transferred in an identical way five times on the basis of complete 3,4-DCA degradation (enrichment of 10 and 8 weeks for BEH and DKE, respectively). The N, O-DMHA-fed cultures was transferred five times in a period of 10 weeks on the basis of visual growth.
To isolate linuron-, 3,4-DCA- and N,O-DMHA-degrading community members, 10-fold dilution series of the cultures enriched on either linuron, 3,4-DCA or N,O-DMHA were plated on R2A agar medium supplemented with respectively linuron, 3,4-DCA or N, O-DMHA. On the basis of colony morphology, individual colonies were picked and transferred at least three times on fresh plates to obtain pure isolates. All isolates were tested for degradation of linuron and 3,4-DCA in batch, and growth on N, O-DMHA. To identify clonally identical isolates, genomic fingerprints based on the repetitive 154-bp BOX element were compared between strains (Koeuth et al., 1995). A representative of each group of strains showing identical genomic fingerprints was retained for further analysis and identification by partial 16S rRNA gene sequencing (Polz & Cavanaugh, 1998).
Batch degradation experiments
Degradation of metobromuron, diuron or isoproturon by the enriched cultures was tested by inoculating 250 μL of the cultures in 25 mL of MMN supplemented with 25 mg L−1 of one of the phenylurea herbicides. Metobromuron, diuron and isoproturon concentrations in the cultures and controls were monitored with HPLC during incubation. Tests were performed in triplicate, and uninoculated vials containing the same media were incubated simultaneously to determine abiotic losses. Batch degradation experiments with individual and mixed isolates were performed in triplicate in 25 mL of MMN supplemented with 25 mg L−1 linuron or 3,4-DCA as the sole source of carbon and nitrogen, either in the absence or in the presence of amino acids (Casamino acids, Difco, 1 g L−1), by inoculating 250 μL of a cell suspension of the appropriate strain(s). Before inoculation, the R2A-grown cells were washed three times in 0.015 M NaCl, and the OD600 nm (Spectronic 21, Bausch & Lomb) was adjusted to 0.25. Ten-fold dilution series were plated on R2A agar medium to determine corresponding viable cell numbers, which were in all cases of the order of 108 CFU mL−1. Vials containing no inoculum served as abiotic controls. Linuron and metabolite concentrations in the cultures and controls were monitored with HPLC during incubation. Utilization of N, O-DMHA as a carbon and/or nitrogen source was determined by following the change in OD600 nm in 8 mL of MMO or MMN supplemented with either 200 mg L−1N, O-DMHA or 1% (v/v) methanol and 200 mg L−1N, O-DMHA at 25 °C. No solid support was provided in these experiments. After each experiment, the cultures were plated on R2A agar medium to rule out possible contamination. All incubations were carried out at 25 °C in the dark on a rotary shaker set at 120 r.p.m.
HPLC analysis
Cultures (including sterile controls) were sampled and analysed by reverse-phase HPLC (LaChrom, Merck Hitachi) after centrifugation at 8500 g for 10 min. The HPLC system was equipped with a LiChroCART 4-4 Purospher STAR RP-18 guard column and a LiChroCART 125-4 Purospher STAR RP-18 endcapped column packed with silica beads (5 μm), and a UV detector set at 210 nm. The mobile phase consisted of CH3OH/0.1% H3PO4 (70/30) with a flow rate of 0.8 mL min−1 (Dejonghe et al., 2003). Identification and quantification were based on comparison to the retention times and peak areas of external standards of linuron, isoproturon, diuron, metobromuron and 3,4-DCA.
14C-linuron mineralization experiments
Mineralization of 14C-linuron was examined in triplicate in 15 mL Pyrex tubes as described previously (Uyttebroek et al., 2006). Mineralization by the bulk soil was assayed by adding 250 mg (dry weight) of sieved soil (<2 mm) to 5 mL of medium. The linuron mineralization capacity of the enrichment cultures was assessed with 20-week-old cultures that had been refreshed 10 times. The inocula were prepared by washing the cultures once with 0.015 M NaCl, and 1 mL of this washed suspension was added to 4 mL of medium. In the case of the enrichments with solid support, this included transfer of soil particles to the mineralization vials. The inoculum size for all cultures was of the order of 107 CFU mL−1, based on the plating of serial dilution series. In the case of the enrichments with solid support, this number may not take into account cells firmly attached to soil particles. The total linuron concentration (labelled and unlabelled) in the MMN medium (pH 5.8) was 25 mg L−1, and the total radioactivity was 0.009 μCi mL−1. The vials were incubated at 25 °C in the dark on a rotary shaker set at 120 r.p.m., and cumulative mineralization curves were established. Maximal mineralization rates were calculated as the slope of the linear regression line between two of the first three successive points of the mineralization curve at which the amount of 14CO2 showed the largest increase. The Tukey test (SAS® 9.1, Cary, NC) (P<0.05) was used for all statistical comparisons of maximal mineralization rate and extent.
DNA extraction
Total DNA was extracted from bulk soil (0.4 g) or enrichment cultures and pure cultures (400 μL) as described previously (Uyttebroek et al., 2006). The crude DNA extracts were purified with a Wizard DNA clean-up kit with vacuum manifold, according to the manufacturer's instructions (Promega). Purified DNA was recovered in 50 μL of Molecular Biology Water (Cambrex) and stored at −20 °C.
PCR
All PCR reactions were performed in a Mastercycler from Eppendorf (Hamburg, Germany). Eubacterial 16S rRNA gene fragments were PCR-amplified using forward primer 63F with a GC clamp 5′ attached and reverse primer 518R (El-Fantroussi et al., 1999). For sequencing, 16S rRNA gene fragments of the pure isolates were amplified using eubacterial primers 27F and 1492R (Polz & Cavanaugh, 1998). In both cases, the PCR mixture contained 1 μL of DNA extract, 5 μL of 10 × PCR buffer (Qiagen), 5 μL of 1% bovine serum albumin (BSA) (Amersham Biosciences), 4 μL of dNTPs (2.5 mM each) (Invitrogen), 0.25 μL of each primer (0.1 mM) (Operon), and 0.25 μL Taq DNA polymerase (5 U μL−1) (Qiagen) in a total volume of 50 μL. The boxA element was amplified using primer BOXA1R as described previously (Koeuth et al., 1995) in a 50 μL mixture containing 2 μL of DNA extract, 5 μL of 10 × PCR buffer (Qiagen), 0.8 μL of 1% BSA, 5 μL of 100% dimethyl sulfoxide (Sigma Aldrich), 2.5 μL of dNTPs (2.5 mM each) (Invitrogen), 0.85 μL of primer BOXA1R (0.1 mM) (Operon) and 0.25 μL of Taq DNA polymerase (5 U μL−1). Amplification was checked by electrophoresis on 1.5% agarose gels stained with ethidium bromide (0.2 mg L−1).
Denaturing gradient gel electrophoresis (DGGE)
DGGE analysis of the PCR products amplified with eubacterial primers 63F and 518R was performed as described previously (Uyttebroek et al., 2006). gelcompar II version 3.5 (Applied Maths) was used for cluster analysis of DGGE fingerprints. The similarity matrix for the fingerprints was calculated based on the Pearson product–moment correlation coefficient (Pearson, 1926), and the unweighed pair group method with arithmetic mean (UPGMA) (Sneath & Sokal, 1973) was used as a clustering algorithm to calculate the dendrogram. The SD of a dendrogram branch was obtained by reconstructing the similarity values from the branch and comparing the values with the original similarity values.
Sequence analysis and accession numbers
Amplified 16S rRNA gene fragments with primers 27F and 1492R were purified from impurities with a QIAquick PCR purification kit according to the manufacturer's instructions (Qiagen). Sequencing reactions were performed with a BigDye Terminator cycle sequencing kit (Applied Biosystems) using the eubacterial primer 27F, and analysed on an automatic sequencer (ABI PRISM 3100-Avant, Applied Biosystems). The resulting 16S rRNA gene sequences were compared with sequences deposited in GenBank by performing a blastn search (Altschul et al., 1997). The partial 16S rRNA gene sequence data have been deposited in the EMBL nucleotide sequence database (Kulikova et al., 2007) under accession numbers AM502915–AM502926.
The phylogenetic relationship between linuron-degrading and nonlinuron-degrading Variovorax spp. was studied with sequences obtained from GenBank and this study. Sequences were aligned with mega version 3.1 (Kumar et al., 2004) based on the clustalw algorithm (Thompson et al., 1994) using default parameters, and phylogenetic trees were generated with a neighbour-joining (NJ) algorithm. Confidence values for NJ trees were generated by bootstrapping, based on 1000 replicates.
Results
Linuron mineralization capacity of soil communities and enrichment cultures
The indigenous microbial communities of the two soils BEH and DKE were able to mineralize linuron (data not shown). In contrast, untreated soil BEW did not show 14C-linuron mineralization even after an incubation period of 9 weeks (data not shown). No 14C-linuron mineralization was observed with sterile controls of soils BEH, BEW and DKE (data not shown). From soils BEH and DKE, linuron-degrading enrichment cultures were obtained, both in the presence (BEH-LWS and DKE-LWS) and in the absence (BEH-LNS and DKE-LNS) of a solid support. All four enrichment cultures showed linuron mineralization immediately after inoculation (data not shown). Although originating from different sources and enriched under different conditions, no significant difference (significance level α=0.05) was observed between these cultures regarding maximal mineralization extent (c. 50%) and rate (c. 0.037 μg L−1 day−1). Throughout the enrichments, no accumulation of 3,4-DCA or other metabolites could be detected using HPLC. In addition to linuron, the cultures were capable of degrading metobromuron, but no activity was observed towards diuron and isoproturon (data not shown).
Community composition analysis by DGGE fingerprinting
The eubacterial community compositions of the linuron enrichment cultures derived from soils BEH and DKE were compared by means of 16S rRNA gene-based PCR-DGGE community fingerprinting (Fig. 1). The community fingerprints constituted two distinct clusters reflecting the soil of origin. The community structure of the enrichment culture of soil BEH in the presence of a solid support was clearly different from that of the enrichment culture obtained without solid support, with a similarity (±SD) of only 37%±12%. In contrast, for soil DKE the fingerprints of the enrichments in the presence and absence of a solid support grouped together.
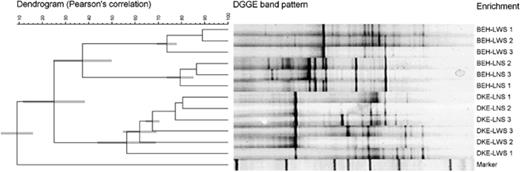
UPGMA-dendrogram of 16S rRNA gene-based PCR-DGGE community profiles of linuron-mineralizing cultures with (LWS) and without (LNS) solid support derived from soil BEH and soil DKE. The scale indicates the similarity level (0–100%). DNA samples originated from cultures that had been refreshed three times (8 weeks) (1), six times (14 weeks) (2), and 10 times (20 weeks) (3). Denaturant concentrations increase from left to right.
Characterization of the linuron- and metabolite-degrading consortium members
To unravel the composition of the linuron-mineralizing enrichment cultures, subcultures fed with reported potential metabolites of the linuron degradation pathway, namely 3,4-DCA and N, O-DMHA, were set up. From both the linuron-mineralizing cultures and the metabolite-fed subcultures, single strains were obtained by plating on R2A agar medium containing, respectively, linuron, 3,4-DCA or N, O-DMHA, and further purification on the same medium. A total of 48 and 52 isolates were recovered from the enrichment cultures from soils BEH and DKE, respectively. From each soil, c. 50% of the strains were able to degrade linuron or one of the metabolites. After evaluation of the degradation capabilities of each individual strain, clonally identical isolates were identified with BOX-PCR. Representatives of each group of strains showing identical BOX-PCR genomic fingerprints were identified by partial 16S rRNA gene analysis (Table 2). To examine whether the isolates represented dominant strains in the enrichment cultures, the 16S rRNA gene DGGE fingerprints of representative isolates were compared with those of the enrichment cultures from which they originated (Fig. 2).
Overview of linuron-, 3,4-DCA- and N, O-DMHA-degrading isolates recovered from the linuron-mineralizing cultures enriched from soil BEH and soil DKE
Isolate | Enrichment culture | No. of identical isolates (based on BOX-PCR) | Closest relative according to blast (% 16S rRNA gene similarity) (Genbank accession no.) | Degradation capability | ||
Linuron | 3,4-DCA | N, O-DMHA | ||||
PBS-H4 | BEH-LWS | 3 | Variovorax sp. WDL1 (99%) (AF538929) | + | − | − |
PBL-H3 | BEH-LNS | 3 | Hydrogenophaga sp. clone J-11 (99%) (AF523049) | + | + | − |
PBL-H6 | BEH-LNS* | 4 | Variovorax sp. WDL1 (98%) (AF538929) | + | + | − |
PBL-H7 | BEH-LWS; BEH-LNS* | 5 | Achromobacter xylosoxidans 2MN-2 (99%) (DQ659433) | +† | +† | − |
PBD-H1 | BEH-LNS*‡ | 5 | Variovorax sp. 3A_12 (99%) (AY689027) | − | + | − |
PBN-H4 | BEH-LWS; BEH-LNS‡ | 4 | Hyphomicrobium sp. DM2 (98%) (AJ854113) | − | − | − |
PBL-E5 | DKE-LWS; DKE-LNS* | 6 | Variovorax sp. SRS16 (98%) (AY621157) | + | + | − |
PBD-E5 | DKE-LNS* | 4 | Variovorax paradoxusP1 (98%) (AJ491705) | − | + | − |
PBS-E1 | DKE-LWS | 4 | Cupriavidus sp. WBF7 (99%) (DQ777737) | − | + | − |
PBD-E37 | DKE-LNS§ | 2 | Variovorax sp. C59 (99%) (AB167220) | − | + | − |
PBD-E87 | DKE-LNS§¶ | 3 | Afipia genosp. 14 (100%) (U87785) | − | +† | − |
PBN-E9 | DKE-LWS; DKE-LNS‡ | 5 | Hyphomicrobium sp. MW1 (98%) (AY934495) | − | − | + |
Isolate | Enrichment culture | No. of identical isolates (based on BOX-PCR) | Closest relative according to blast (% 16S rRNA gene similarity) (Genbank accession no.) | Degradation capability | ||
Linuron | 3,4-DCA | N, O-DMHA | ||||
PBS-H4 | BEH-LWS | 3 | Variovorax sp. WDL1 (99%) (AF538929) | + | − | − |
PBL-H3 | BEH-LNS | 3 | Hydrogenophaga sp. clone J-11 (99%) (AF523049) | + | + | − |
PBL-H6 | BEH-LNS* | 4 | Variovorax sp. WDL1 (98%) (AF538929) | + | + | − |
PBL-H7 | BEH-LWS; BEH-LNS* | 5 | Achromobacter xylosoxidans 2MN-2 (99%) (DQ659433) | +† | +† | − |
PBD-H1 | BEH-LNS*‡ | 5 | Variovorax sp. 3A_12 (99%) (AY689027) | − | + | − |
PBN-H4 | BEH-LWS; BEH-LNS‡ | 4 | Hyphomicrobium sp. DM2 (98%) (AJ854113) | − | − | − |
PBL-E5 | DKE-LWS; DKE-LNS* | 6 | Variovorax sp. SRS16 (98%) (AY621157) | + | + | − |
PBD-E5 | DKE-LNS* | 4 | Variovorax paradoxusP1 (98%) (AJ491705) | − | + | − |
PBS-E1 | DKE-LWS | 4 | Cupriavidus sp. WBF7 (99%) (DQ777737) | − | + | − |
PBD-E37 | DKE-LNS§ | 2 | Variovorax sp. C59 (99%) (AB167220) | − | + | − |
PBD-E87 | DKE-LNS§¶ | 3 | Afipia genosp. 14 (100%) (U87785) | − | +† | − |
PBN-E9 | DKE-LWS; DKE-LNS‡ | 5 | Hyphomicrobium sp. MW1 (98%) (AY934495) | − | − | + |
Isolated from both initial linuron-fed culture and 3,4-DCA-fed subculture.
Requires the addition of amino acids.
Isolated from both initial linuron-fed culture and N, O-DMHA-fed subculture.
Isolated directly from 3,4-DCA-fed subculture.
Isolated directly from N, O-DMHA-fed subculture.
LWS, linuron enrichment culture with solid support; LNS, linuron enrichment culture without solid support.
Overview of linuron-, 3,4-DCA- and N, O-DMHA-degrading isolates recovered from the linuron-mineralizing cultures enriched from soil BEH and soil DKE
Isolate | Enrichment culture | No. of identical isolates (based on BOX-PCR) | Closest relative according to blast (% 16S rRNA gene similarity) (Genbank accession no.) | Degradation capability | ||
Linuron | 3,4-DCA | N, O-DMHA | ||||
PBS-H4 | BEH-LWS | 3 | Variovorax sp. WDL1 (99%) (AF538929) | + | − | − |
PBL-H3 | BEH-LNS | 3 | Hydrogenophaga sp. clone J-11 (99%) (AF523049) | + | + | − |
PBL-H6 | BEH-LNS* | 4 | Variovorax sp. WDL1 (98%) (AF538929) | + | + | − |
PBL-H7 | BEH-LWS; BEH-LNS* | 5 | Achromobacter xylosoxidans 2MN-2 (99%) (DQ659433) | +† | +† | − |
PBD-H1 | BEH-LNS*‡ | 5 | Variovorax sp. 3A_12 (99%) (AY689027) | − | + | − |
PBN-H4 | BEH-LWS; BEH-LNS‡ | 4 | Hyphomicrobium sp. DM2 (98%) (AJ854113) | − | − | − |
PBL-E5 | DKE-LWS; DKE-LNS* | 6 | Variovorax sp. SRS16 (98%) (AY621157) | + | + | − |
PBD-E5 | DKE-LNS* | 4 | Variovorax paradoxusP1 (98%) (AJ491705) | − | + | − |
PBS-E1 | DKE-LWS | 4 | Cupriavidus sp. WBF7 (99%) (DQ777737) | − | + | − |
PBD-E37 | DKE-LNS§ | 2 | Variovorax sp. C59 (99%) (AB167220) | − | + | − |
PBD-E87 | DKE-LNS§¶ | 3 | Afipia genosp. 14 (100%) (U87785) | − | +† | − |
PBN-E9 | DKE-LWS; DKE-LNS‡ | 5 | Hyphomicrobium sp. MW1 (98%) (AY934495) | − | − | + |
Isolate | Enrichment culture | No. of identical isolates (based on BOX-PCR) | Closest relative according to blast (% 16S rRNA gene similarity) (Genbank accession no.) | Degradation capability | ||
Linuron | 3,4-DCA | N, O-DMHA | ||||
PBS-H4 | BEH-LWS | 3 | Variovorax sp. WDL1 (99%) (AF538929) | + | − | − |
PBL-H3 | BEH-LNS | 3 | Hydrogenophaga sp. clone J-11 (99%) (AF523049) | + | + | − |
PBL-H6 | BEH-LNS* | 4 | Variovorax sp. WDL1 (98%) (AF538929) | + | + | − |
PBL-H7 | BEH-LWS; BEH-LNS* | 5 | Achromobacter xylosoxidans 2MN-2 (99%) (DQ659433) | +† | +† | − |
PBD-H1 | BEH-LNS*‡ | 5 | Variovorax sp. 3A_12 (99%) (AY689027) | − | + | − |
PBN-H4 | BEH-LWS; BEH-LNS‡ | 4 | Hyphomicrobium sp. DM2 (98%) (AJ854113) | − | − | − |
PBL-E5 | DKE-LWS; DKE-LNS* | 6 | Variovorax sp. SRS16 (98%) (AY621157) | + | + | − |
PBD-E5 | DKE-LNS* | 4 | Variovorax paradoxusP1 (98%) (AJ491705) | − | + | − |
PBS-E1 | DKE-LWS | 4 | Cupriavidus sp. WBF7 (99%) (DQ777737) | − | + | − |
PBD-E37 | DKE-LNS§ | 2 | Variovorax sp. C59 (99%) (AB167220) | − | + | − |
PBD-E87 | DKE-LNS§¶ | 3 | Afipia genosp. 14 (100%) (U87785) | − | +† | − |
PBN-E9 | DKE-LWS; DKE-LNS‡ | 5 | Hyphomicrobium sp. MW1 (98%) (AY934495) | − | − | + |
Isolated from both initial linuron-fed culture and 3,4-DCA-fed subculture.
Requires the addition of amino acids.
Isolated from both initial linuron-fed culture and N, O-DMHA-fed subculture.
Isolated directly from 3,4-DCA-fed subculture.
Isolated directly from N, O-DMHA-fed subculture.
LWS, linuron enrichment culture with solid support; LNS, linuron enrichment culture without solid support.
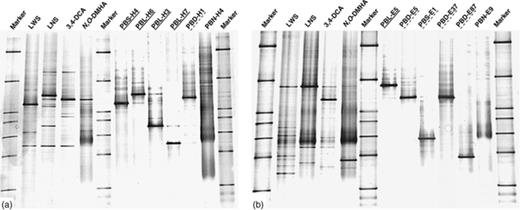
16S rRNA gene PCR-DGGE fingerprints of enrichment cultures and selected isolates of (a) soil BEH and (b) soil DKE. Marker: 16S rRNA gene marker; LWS: linuron culture with solid support (refreshed 10 times); LNS: linuron culture without solid support (refreshed 10 times); 3,4-DCA: 3,4-DCA-fed subculture (transferred five times), and N,O-DMHA: N,O-DMHA-fed subculture (transferred five times). Linuron- and 3,4-DCA-degrading isolates are underlined with a continuous or a dashed line, respectively. Denaturant concentrations increase from top to bottom.
From soil BEH, four unique linuron-degrading strains were isolated, belonging to the genera Variovorax (PBS-H4 and PBL-H6), Hydrogenophaga (PBL-H3) and Achromobacter (PBL-H7). Variovorax sp. PBS-H4 was a dominant member in the linuron-fed culture with solid support (BEH-LWS), and was only recovered from that culture. Isolate PBS-H4 degraded linuron with 3,4-DCA as end product. In contrast, Variovorax sp. PBL-H6, which was a dominant member in the linuron-fed culture without solid support (BEH-LNS), degraded both linuron and 3,4-DCA. This organism could not be isolated from the BEH-LWS culture and apparently did not belong to the dominant strains in that culture. Although showing different DGGE migration patterns, both PBS-H4 and PBL-H6 were phylogenetically most closely related to the linuron degrader Variovorax sp. WDL1 (Dejonghe et al., 2003) (Fig. 3). Hydrogenophaga sp. PBL-H3 was only recovered from the BEH-LNS culture and apparently represented a dominant strain in that culture. Achromobacter sp. PBL-H7 was a prominent member in both linuron-fed cultures. These latter two strains degraded linuron beyond 3,4-DCA. One isolate from soil BEH, Variovorax sp. PBD-H1, degraded 3,4-DCA but not linuron. The strain was a dominant member in the BEH-LNS culture, the 3,4-DCA-fed subculture and the N, O-DMHA-fed subculture.
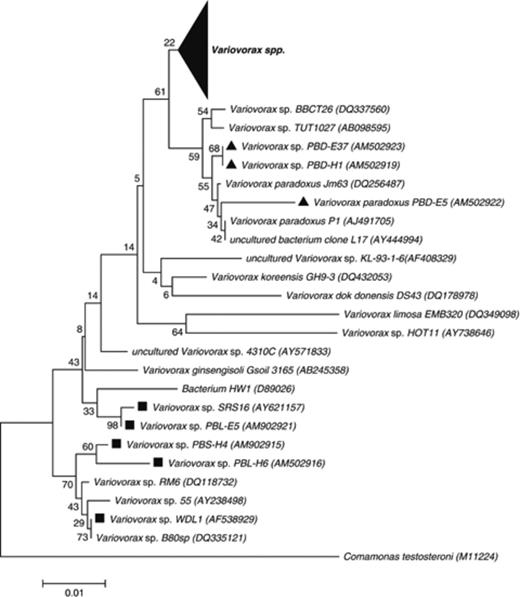
Neighbour-joining phylogenetic tree based on 16S rRNA gene fragments showing the relationship between linuron-degrading and nonlinuron-degrading Variovorax spp. from this study and the literature. Linuron-degrading isolates are indicated with a black square; and 3,4-DCA-degrading isolates, with a black triangle. The large black triangle covers 67 randomly selected sequences of Variovorax spp. The Comamonas testosteroni type strain M11224 was used as outgroup. GenBank accession numbers are indicated in parentheses. The statistics based on bootstrapping with 1000 replications are indicated at the nodes of each branch.
From soil DKE, one linuron-degrading isolate (PBL-E5) was obtained. It was a dominant member in the linuron-fed enrichment cultures both with (DKE-LWS) and without (DKE-LNS) solid support. Isolate PBL-E5 was phylogenetically most closely related to Variovorax sp. SRS16, a linuron-mineralizing strain previously isolated from the same field (Sørensen et al., 2005) (Fig. 3). As was the case with strain SRS16, PBL-E5 degraded both linuron and 3,4-DCA. The genomic BOX-PCR fingerprint of PBL-E5 was very similar to the fingerprint of SRS16, but not identical to it (data not shown). In addition, four strains that degraded 3,4-DCA but not linuron were isolated from soil DKE. They belonged to the genera Variovorax (PBD-E37 and PBD-E5), Cupriavidus (PBS-E1) or Afipia (PBD-E87). Strains PBD-37 and PBD-E87 were only detected in the metabolite-fed subcultures, whereas strains PBD-E5 and PBS-E1 were members of the linuron-fed cultures. Although PBD-E37 and PBD-E5 showed similar migration patterns on DGGE (Fig. 2b), they proved to be different strains based on BOX-PCR analysis (data not shown).
Finally, Hyphomicrobia were isolated from both soils. They were dominant in the N, O-DMHA-fed subcultures and were also present in the linuron-fed cultures with and without solid support. Hyphomicrobium sp. PBN-E9, recovered from soil DKE, was able to utilize N, O-DMHA as the sole source of carbon and nitrogen, whereas Hyphomicrobium sp. PBN-H4, obtained from soil BEH, did not show growth (data not shown).
Metabolic interactions between isolated consortium members
To examine whether cross-feeding interactions were of importance for linuron degradation in the original consortia, relevant linuron- and 3,4-DCA-degrading isolates derived from the same soil were combined and evaluated for their linuron degradation efficiency compared with single-strain linuron-degrading cultures. In addition, the influence of amino acids on linuron degradation was determined with individual linuron degraders. Table 3 summarizes the results for all tested strains, and Figs 4 and5 show the linuron degradation curves for two representative examples.
Linuron degradation by pure linuron-degrading cultures, in the presence of amino acids and in combination with a 3,4-DCA-degrading isolate
Time to complete linuron removal (days) | Maximal 3,4-DCA accumulation (% of theoretical yield) | Time to remove maximal accumulated 3,4-DCA concentration (days) | |
PBL-H6 | 22 | 37 (± 2.4) | 14 |
+Amino acids | 4 | 3 (<1) | ND |
+PBD-H1 | 8 | 7 (± 2.1) | ND |
PBL-H3 | 50 | 100 (± 2.7) | 30 |
+Amino acids | 28 | 74 (± 2.6) | 18 |
+PBD-H1 | 1 | 2 (<1) | ND |
PBS-H4 | 4 | 100 (<1) | ∞ |
+Amino acids | 6 | 100 (<1) | ∞ |
+PBD-H1 | 3 | 6 (± 2.6) | ND |
PBL-E5 | 15 | 34 (± 1.5) | 10 |
+Amino acids | 3 | 14 (<1) | 2 |
+PBD-E5 | 5 | 4 (<1) | ND |
+PBD-E37 | 12 | 5 (<1) | ND |
+PBS-E1 | 18 | 7 (<1) | ND |
+PBD-E87 | 20 | 24 (± 2.2) | 15 |
Time to complete linuron removal (days) | Maximal 3,4-DCA accumulation (% of theoretical yield) | Time to remove maximal accumulated 3,4-DCA concentration (days) | |
PBL-H6 | 22 | 37 (± 2.4) | 14 |
+Amino acids | 4 | 3 (<1) | ND |
+PBD-H1 | 8 | 7 (± 2.1) | ND |
PBL-H3 | 50 | 100 (± 2.7) | 30 |
+Amino acids | 28 | 74 (± 2.6) | 18 |
+PBD-H1 | 1 | 2 (<1) | ND |
PBS-H4 | 4 | 100 (<1) | ∞ |
+Amino acids | 6 | 100 (<1) | ∞ |
+PBD-H1 | 3 | 6 (± 2.6) | ND |
PBL-E5 | 15 | 34 (± 1.5) | 10 |
+Amino acids | 3 | 14 (<1) | 2 |
+PBD-E5 | 5 | 4 (<1) | ND |
+PBD-E37 | 12 | 5 (<1) | ND |
+PBS-E1 | 18 | 7 (<1) | ND |
+PBD-E87 | 20 | 24 (± 2.2) | 15 |
Values are means of triplicate measurements (SD is given between parentheses). ND, not determined due to low 3,4-DCA accumulation (<10%); ∞, no 3,4-DCA degradation.
Linuron degradation by pure linuron-degrading cultures, in the presence of amino acids and in combination with a 3,4-DCA-degrading isolate
Time to complete linuron removal (days) | Maximal 3,4-DCA accumulation (% of theoretical yield) | Time to remove maximal accumulated 3,4-DCA concentration (days) | |
PBL-H6 | 22 | 37 (± 2.4) | 14 |
+Amino acids | 4 | 3 (<1) | ND |
+PBD-H1 | 8 | 7 (± 2.1) | ND |
PBL-H3 | 50 | 100 (± 2.7) | 30 |
+Amino acids | 28 | 74 (± 2.6) | 18 |
+PBD-H1 | 1 | 2 (<1) | ND |
PBS-H4 | 4 | 100 (<1) | ∞ |
+Amino acids | 6 | 100 (<1) | ∞ |
+PBD-H1 | 3 | 6 (± 2.6) | ND |
PBL-E5 | 15 | 34 (± 1.5) | 10 |
+Amino acids | 3 | 14 (<1) | 2 |
+PBD-E5 | 5 | 4 (<1) | ND |
+PBD-E37 | 12 | 5 (<1) | ND |
+PBS-E1 | 18 | 7 (<1) | ND |
+PBD-E87 | 20 | 24 (± 2.2) | 15 |
Time to complete linuron removal (days) | Maximal 3,4-DCA accumulation (% of theoretical yield) | Time to remove maximal accumulated 3,4-DCA concentration (days) | |
PBL-H6 | 22 | 37 (± 2.4) | 14 |
+Amino acids | 4 | 3 (<1) | ND |
+PBD-H1 | 8 | 7 (± 2.1) | ND |
PBL-H3 | 50 | 100 (± 2.7) | 30 |
+Amino acids | 28 | 74 (± 2.6) | 18 |
+PBD-H1 | 1 | 2 (<1) | ND |
PBS-H4 | 4 | 100 (<1) | ∞ |
+Amino acids | 6 | 100 (<1) | ∞ |
+PBD-H1 | 3 | 6 (± 2.6) | ND |
PBL-E5 | 15 | 34 (± 1.5) | 10 |
+Amino acids | 3 | 14 (<1) | 2 |
+PBD-E5 | 5 | 4 (<1) | ND |
+PBD-E37 | 12 | 5 (<1) | ND |
+PBS-E1 | 18 | 7 (<1) | ND |
+PBD-E87 | 20 | 24 (± 2.2) | 15 |
Values are means of triplicate measurements (SD is given between parentheses). ND, not determined due to low 3,4-DCA accumulation (<10%); ∞, no 3,4-DCA degradation.
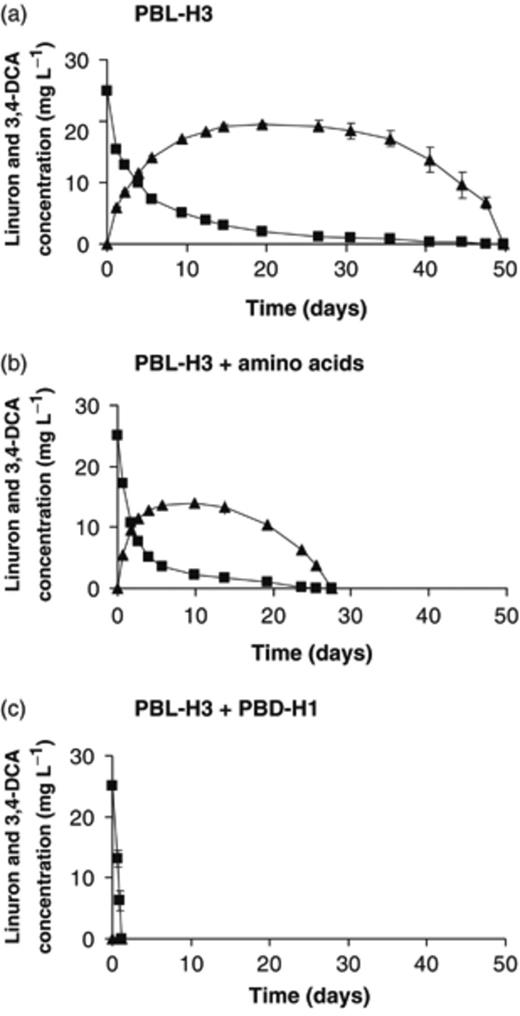
Linuron degradation by Hydrogenophaga sp. PBL-H3 (a) individually, (b) in the presence of amino acids, and (c) in combination with Variovorax sp. PBD-H1. Linuron and 3,4-DCA concentrations are represented by squares and triangles, respectively. Data points and error bars represent the means and SDs of triplicate measurements. Where error bars are not visible, they are hidden behind the symbols.
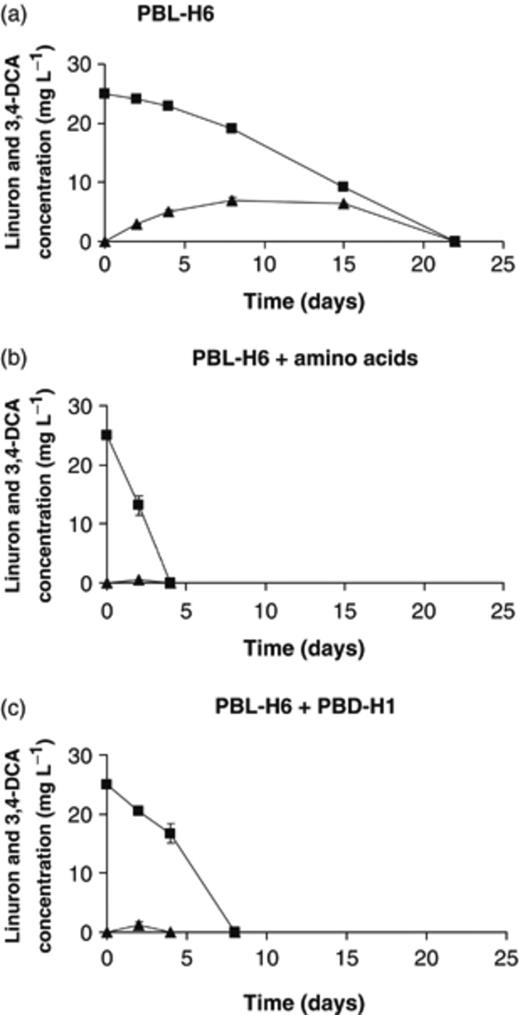
Linuron degradation by Variovorax sp. PBL-H6 (a) individually, (b) in the presence of amino acids, and (c) in combination with Variovorax sp. PBD-H1. Linuron and 3,4-DCA concentrations are represented by squares and triangles, respectively. Data points and error bars represent the means and SDs of triplicate measurements. Where error bars are not visible, they are hidden behind the symbols.
In most cases, combinations of a linuron degrader and a 3,4-DCA degrader from soil BEH degraded linuron faster with less accumulation of 3,4-DCA. The combination of the linuron degraders Variovorax sp. PBL-H6 and Hydrogenophaga sp. PBL-H3 with the 3,4-DCA degrader Variovorax sp. PBD-H1 resulted in a significantly faster linuron degradation with minimal release of 3,4-DCA compared with results from the individual strains. Moreover, the combination of Variovorax sp. PBS-H4, which could not degrade 3,4-DCA, with Variovorax sp. PBD-H1 proved to be very efficient in complete linuron degradation. With isolates from soil DKE, similar results were obtained when coinoculating linuron degrader Variovorax sp. PBL-E5 with 3,4-DCA degraders Variovorax sp. PBD-E37 or Variovorax sp. PBD-E5. However, when PBL-E5 was coinoculated with the 3,4-DCA degraders Cupriavidus sp. PBS-E1 or Afipia sp. PBD-E87, linuron degradation was retarded. Nevertheless, less 3,4-DCA accumulated compared with the case for linuron degradation by PBL-E5 only.
Hydrogenophaga sp. PBL-H3 and Variovorax spp. PBL-H6 and PBL-E5 degraded linuron more efficiently when amino acids were added. For strains PBL-H6 and PBL-E5, the addition of amino acids resulted in even faster linuron degradation compared with the mixed cultures. Moreover, strain PBL-H6 did not accumulate 3,4-DCA in the presence of amino acids. In contrast, strain PBL-H3 still accumulated 3,4-DCA to 74% of its theoretical maximum when amino acids were added and clearly performed better when combined with the 3,4-DCA degrader. Isolate PBS-H4, which is not able to degrade 3,4-DCA, did not benefit from the addition of amino acids.
Discussion
This paper reports on the characterization of novel linuron-mineralizing cultures enriched from two long-term linuron-treated soils. Efficient linuron-mineralizing communities were enriched by successive transfers to fresh medium in the absence and presence of soil particles. 16S rRNA gene-based DGGE community fingerprinting and plating revealed that the cultures with and without solid support enriched from soil BEH were dominated by different strains. Bands dominant in cultures containing soil particles possibly represent bacteria that proliferate preferentially in association with a solid support but are outcompeted when the solid support is removed. Classical enrichments involving transfers to fresh medium tend to select for bacteria that grow rapidly in suspension and that might outcompete slow-growing bacteria. A solid phase can function as a substratum to which slow-growing bacteria attach, meaning that the bacteria are retained in the system (Dunbar et al., 1997; Bastiaens et al., 2000). Interestingly, the dominant linuron-degrading strain in the BEH-LWS culture, namely Variovorax sp. PBS-H4, grew as flocs or as a biofilm on the wall of the recipient flask in R2A broth (data not shown). In contrast, Variovorax sp. PBL-H6, the dominant linuron-degrading member in the BEH-LNS culture, grew as suspended cells. Nevertheless, both strains were phylogenetically closely related. It suggests that Variovorax is an important linuron degrader also under conditions of enrichment in which slow-growing bacteria can remain in the system through attachment.
The linuron-mineralizing cultures degraded, in addition to linuron, the N-methoxy-N-methyl urea herbicide metobromuron, but not the dimethyl-substituted phenylureas diuron and isoproturon. Previously reported linuron-degrading cultures showed variable activity towards different phenylureas (Engelhardt et al., 1971; Roberts et al., 1993; El-Fantroussi et al., 2000; Turnbull et al., 2001), indicating that the transformation of different urea herbicides involves different enzymes, and that substituents on both the aromatic ring and the urea side chain determine catalytic enzyme specificity, or that, alternatively, specificity is determined at the level of gene expression and/or transport into the cell. Interestingly, as in the cultures of this study, the culture described by El-Fantroussi et al. (2000) degraded linuron and metobromuron but not diuron or isoproturon, and contained a Variovorax strain as primary linuron degrader (Dejonghe et al., 2003), suggesting that the phenylurea substrate range of Variovorax is limited to linuron and metobromuron.
Plating enabled the isolation of the key members of the linuron- and metabolite-fed enrichment cultures. Although PCR-based analysis might result in biases (Suzuki & Giovannoni, 1996), comparison of the 16S rRNA gene DGGE fingerprints of the isolates with those of the enrichment cultures of origin suggested that the isolates belonged to the dominant strains in the enrichment cultures. On the other hand, for some bands, no corresponding strains could be isolated. Because several strains were isolated that degraded neither linuron, 3,4-DCA nor N, O-DMHA, and since we only focused on linuron-, 3,4-DCA- and N, O-DMHA-degrading strains, these bands might represent nondegrading community members. Previously, Dejonghe et al. (2003) identified in a linuron-degrading consortium a Pseudomonas strain that apparently was not involved in the direct degradation of linuron or its pathway intermediates. Alternatively, these members could not be cultured on R2A broth or they depended for growth on a solid support or physical interactions with other bacteria. From both soils, organisms were obtained that degraded linuron, 3,4-DCA and/or N, O-DMHA, suggesting that the degradation of linuron in the enrichment cultures and in the soils followed the canonical pathway through hydrolysis of linuron into 3,4-DCA and N, O-DMHA and further 3,4-DCA and N, O-DMHA mineralization. In addition, it suggests that, in agreement with the study of Dejonghe et al. (2003), linuron in the enrichment cultures is degraded by a group of metabolically cooperating bacteria. Indeed, from all enrichments, next to organisms that could degrade both linuron and 3,4-DCA, isolates were obtained that degraded N, O-DMHA or 3,4-DCA and not linuron, indicating that these organisms proliferated on N, O-DMHA or 3,4-DCA leaking from linuron degraders. The 3,4-DCA-degrading strains were recovered from the linuron-fed cultures by plating and purification on a medium containing linuron, suggesting that their inability to degrade linuron was not the result of the loss of a linuron-degrading capacity through genetic instability. In contrast, Sørensen et al. (2005) hypothesized that Variovorax sp. SRS16 mineralized linuron independently. However, they made no attempts to isolate metabolite-degrading members, and DGGE fingerprints of the enrichment culture from which SRS16 originated showed several dominant bands in addition to the band corresponding to strain SRS16. Soil DKE, used in this study, was derived from the same field from which strain SRS16 was isolated, and this study clearly shows that this field also contains linuron-degrading consortia. From this soil, Variovorax sp. PBL-E5 was isolated as the primary linuron degrader. Of all Variovorax strains, this strain was apparently phylogenetically most strongly related to strain SRS16. The two strains showed very similar BOX-PCR genomic fingerprints, with only small differences in banding pattern, indicating that they might have a close ancestral relationship.
Most linuron-degrading strains were phylogenetically related to previously described linuron-degrading Variovorax strains (Dejonghe et al., 2003; Sørensen et al., 2005). Clearly, members of the genus Variovorax play a crucial role in linuron degradation. However, to the best of our knowledge, we show for the first time here that strains belonging to genera other than Variovorax, namely Hydrogenophaga and Achromobacter strains, can degrade linuron beyond 3,4-DCA. Other linuron-degrading isolates such as B. sphaericus ATCC12123 and A. globiformis D47 only hydrolyzed linuron into 3,4-DCA, which accumulated as an end product (Engelhardt et al., 1971; Cullington & Walker, 1999). All linuron-degrading Variovorax strains isolated up to now from linuron-treated soils seem to belong to two distinct clusters that are separated from the main cluster of Variovorax strains (Fig. 3). The two clusters seem to reflect the country of origin. The isolates that degraded 3,4-DCA and not linuron were identified as Variovorax, Cupriavidus and Afipia. Interestingly, in contrast to the linuron-degrading Variovorax strains, the 3,4-DCA-degrading Variovorax strains did not group phylogenetically with the linuron-degrading Variovorax strains, but rather grouped with the bulk of known Variovorax strains. It emphasizes that the capacity for hydrolytic cleavage of linuron into 3,4-DCA and N, O-DMHA is inherent to a specific group of Variovorax strains. The identification of the consortia members further shows that the compositions of all linuron-degrading consortia recovered up to now, although enriched from physico-chemically different and geographically separated soils, show strong resemblances to each other and to the consortium described by Dejonghe et al. (2003). In all consortia, Variovorax spp. seem to fulfil a crucial role in metabolizing both linuron and 3,4-DCA. Most 3,4-DCA-degrading community members belong to the order of Burkholderiales within the Betaproteobacteria. Finally, members of the genus Hyphomicrobium (Alphaproteobacteria) were found in all linuron-degrading consortia and are apparently responsible for N, O-DMHA degradation. Members of this genus are so far the only cultured N, O-DMHA-degrading bacteria (Dejonghe et al., 2003).
Further evidence that synergistic metabolic interactions between consortium members are involved in efficient linuron mineralization in the obtained consortia was derived from experiments with individual linuron-degrading strains and relevant combinations of linuron and 3,4-DCA degraders. Mixed cultures degraded linuron more rapidly than individual strains did, although most tested linuron degraders also have the capability to degrade 3,4-DCA. In contrast to the case for the individual strains, most mixed cultures did not accumulate 3,4-DCA. Therefore, the positive effect on linuron degradation in the cocultures and in the consortia can probably be attributed to the fast removal of 3,4-DCA leaking from the linuron degraders. Similar observations have been reported by Dejonghe et al. (2003). 3,4-DCA has been shown to inhibit the aryl acylamidase enzyme that is responsible for linuron hydrolysis in B. sphaericus ATCC12123 (Engelhardt et al., 1973). Moreover, it was observed that a high concentration of 3,4-DCA retards the growth of Variovorax spp. in R2A broth (P. Breugelmans, unpublished results). On the other hand, the addition of amino acids resulted in many cases in an increased linuron degradation rate. Moreover, in the case of strain PBL-H6, the addition of amino acids resulted in a strongly reduced accumulation of 3,4-DCA and in a linuron-degradation rate higher than that found when strain PBL-H6 was mixed with strain PBD-H1. This indicates that, in addition to a metabolic interaction involving the removal of leaking 3,4-DCA, synergistic interactions in the linuron-degrading cultures can also involve the exchange of amino acids. Sørensen et al. (2002) reported previously on such interactions in an enrichment culture degrading isoproturon.
Finally, initial inhibition of linuron degradation was observed when strains were mixed; that is, when the linuron degrader Variovorax sp. PBL-E5 was combined with Cupriavidus sp. PBS-E1 or Afipia sp. PBD-E87. Notably, members of the genus Cupriavidus have been reported to feed on other bacteria (Makkar & Casida, 1987). Clearly, the interactions between consortium members are complex, and more work is needed to elucidate this interplay. The similarities in community composition between different linuron-degrading consortia from different linuron-treated fields strongly suggest the ecological relevance of the identified bacterial species in the in situ biodegradation of linuron in soil. Monitoring the abundance and dynamics of these species during linuron degradation in the field is the next step in understanding whether or not they are actually acting as a consortium in the soil itself, and, if so, in elucidating their respective roles in the natural attenuation of linuron in treated soils.
Acknowledgements
This study was financially supported by the Research Fund of K.U. Leuven (OT/2003/39) and the Institute for the Promotion of Innovation through Science and Technology in Flanders (LBO/IWT/040727). The authors thank S.R. Sørensen for supplying the linuron-treated soil DKE, and G. Schoofs for assistance with 16S rRNA gene sequence analysis.
References
Author notes
Present address: Pieter-Jan D'Huys, Chemical and Biochemical Process Technology and Control Section, Catholic University of Leuven, Willem de Croylaan 46, B-3001 Leuven, Belgium.
Editor: Max Häggblom