-
PDF
- Split View
-
Views
-
Cite
Cite
Gabriele Berg, Annette Krechel, Michaela Ditz, Richard A. Sikora, Andreas Ulrich, Johannes Hallmann, Endophytic and ectophytic potato-associated bacterial communities differ in structure and antagonistic function against plant pathogenic fungi, FEMS Microbiology Ecology, Volume 51, Issue 2, January 2005, Pages 215–229, https://doi.org/10.1016/j.femsec.2004.08.006
- Share Icon Share
Abstract
Differences between endophytic and ectophytic bacterial communities with stress on antagonistic bacteria, were studied by comparing the composition of communities isolated from the rhizosphere, phyllosphere, endorhiza and endosphere of field-grown potato plants using a multiphasic approach. Terminal restriction fragment length polymorphism analysis of 16S rDNA of the bacterial communities revealed discrete microenvironment-specific patterns. To measure the antagonistic potential of potato-associated bacteria, a total of 2648 bacteria were screened by dual testing of antagonism to the soilborne pathogens Verticillium dahliae and Rhizoctonia solani. Composition and diversity of bacterial antagonists were mainly specific for each microenvironment. The rhizosphere and endorhiza were the main reservoirs for antagonistic bacteria and showed the highest similarity in their colonisation by antagonists. The most prominent species of all microenvironments was Pseudomonas putida, and rep-PCR with BOX primers showed that these isolates showed microenvironment-specific DNA fingerprints. P. putida isolates from the rhizosphere and endorhiza gave nearly identical fingerprints confirming the high similarity of bacterial populations. The phlD gene, involved in the production of the antibiotic 2,4-diacetyl-phloroglucinol, was found only among Pseudomonas isolates from the rhizosphere and endorhiza. Evaluation of the bacterial isolates for biocontrol potential based on fungal antagonism and physiological characteristics resulted in the selection of five promising isolates from each microenvironment. The most effective isolate was Serratia plymuthica 3Re4-18 isolated from the endorhiza.
1 Introduction
The knowledge on plant-associated bacteria and their antagonistic potential is essential not only for understanding their ecological role and the interaction with plants, but also for future biotechnological application, e.g., biological control of plant pathogens or the isolation of bioactive compounds [1–3]. Verticillium dahliae Kleb. and Rhizoctonia solani Kühn are important pathogens causing dramatic yield losses worldwide on many crops including potato [4,5]. The universal phase-out of the broad-spectrum fumigant methyl bromide as a control measure for soilborne pathogens, is expected to have a major impact on the frequency of occurrence and level of damage caused by soilborne pathogens[6]. Therefore, alternative control methods for these pathogens are urgently needed for commercial potato production.
An environmentally friendly alternative for the protection of roots against fungal pathogens is antagonist-mediated biological control [1,3,7]. Antagonists are naturally occurring organisms with traits enabling them to interfere with pathogen growth, survival or infection[8]. Mechanisms responsible for antagonistic activity include (i) inhibition of the pathogen by antibiotics, toxins and surface-active compounds called biosurfactants [antibiosis], (ii) competition for colonization sites, nutrients and minerals, and (iii) parasitism that may involve production of extra-cellular cell wall degrading enzymes such as chitinase and β-1,3 glucanase [8–10]. Although several studies have shown discrepancies between the antagonistic effects under in vitro conditions and the corresponding in situ efficacy[3] in vitro selection of biological control agents based on antagonistic mechanisms and activity has been reported [11,12]. Successful biological control on the basis of plant-associated antagonists not only requires a better understanding of the complex regulation of disease suppression by antagonists in response to biotic and abiotic factors, but also knowledge of the dynamics and composition of plant-associated bacterial communities and what triggers plant colonization[13].
Little is known about microenvironment specificity of antagonistic plant-associated bacteria and their biocontrol potential especially for endophytic plant colonizers[14]. Endophytic bacteria have been defined by Hallmann et al.[14] as those bacteria that can be isolated from surface-disinfected plant tissues or extracted from within the plant and do not visibly harm the plant. Endophytic bacteria occur in the endosphere, the internal microenvironment of the shoot and leaves, as well as in the internal microenvironment of the root defined as endorhiza[15]. Recently, it has been demonstrated that bacterial endophytes may have beneficial effects on host plants, such as growth promotion and biological control of pathogens [12,16,17]. It has been suggested that these bacteria might interact more closely with the host plant and therefore could be efficient biological control agents in sustainable crop production[16]. Most information about the occurrence of endophytic bacteria and their community structure has been obtained using cultivation-dependent approaches[14]. The use of cultivation-independent methods can provide additional information as has been shown for the potato-associated community structure [18–21]. However, further characterization of bacterial communities and their antagonistic potential, especially of field grown potatoes, is necessary to fully understand their structure and function in plant health and growth.
The aim of this study was to analyze the structure of bacterial communities, especially those from the important functional group of antagonists. Bacterial communities of the endophytic microenvironments endorhiza and endosphere were compared with those from the ectophytic microenvironments rhizosphere and phyllosphere over a two-year period in the field on the potato cultivar Cilena. The structure of the bacterial community was analyzed with the cultivation-independent method T-RFLP (terminal restriction fragments length polymorphism) of 16S rDNA. Bacterial isolates were obtained by cultivation on R2A agar and then screened for antagonism against the soilborne pathogenic fungi V. dahliae and R. solani. Isolates with antagonistic activity were identified and characterized phenotypically and genotypically (i) to provide basic knowledge on microenvironment-associated diversity, (ii) to analyze the origin of endophytes, and (iii) to find new bacteria able to control soilborne fungal pathogens of potato.
2 Materials and methods
2.1 Isolation of bacteria and determination of colony forming units
Potato plants cv. Cilena were sampled at the experimental station of the Institute for Plant Diseases, Bonn University, in Bonn-Poppelsdorf, Germany. In 2001 and 2002, potato plants were sampled at three sampling times during the vegetation period as described by Krechel et al.[19] corresponding to the growth stages of plants as defined by Hack et al.[22]: (1) young plants, (2) flowering plants, and (3) early senescent plants. Roots with adhering soil and leaves were collected in sterile bags and then transported to the laboratory. For the isolation of plant-associated microorganisms 3 g each of roots and leaves were transferred separately to sterile Erlenmeyer flasks containing 27 ml of a 0.85% NaCl solution. The samples were shaken for 10 min. Roots and leaves were then removed for the isolation of bacteria from the endorhiza and endosphere, respectively. The remaining suspensions were filtered through Stomacher bags prior to isolation of the rhizosphere and phyllosphere colonizers. For the isolation of endophytic bacteria, roots and leaves were surface sterilised in 1.5% sodium hypochlorite (NaOCl) for 3 min followed by three washes in sterile tap water. The plant material was imprinted on nutrient agar as a sterility check. After incubation of 5 days non-sterile samples were discarded and only samples, for which no bacterial growth occurred in the sterility check, were included in further analysis. All samples were homogenised with mortar and pestle, serially diluted with sterile 0.85% NaCl and plated onto R2A medium (Difco, Detroit, MI). Plates were incubated for 5 days at 20 °C. Colony forming units were counted and expressed as colony forming units (CFU) per gram fresh weight. For each microenvironment 120 colonies, with visually different colony morphology, were transferred to nutrient agar, purified and then stored at −70 °C in nutrient broth containing 15% glycerol for further use. Isolated bacteria were encoded using a combination of numbers and letters indicating: (1) sampling time (1 = young plants 2001, 2 = flowering plants 2001, 3 = early senescent plants 2001, 4 = young plants 2002, 5 = flowering plants 2002, 6 = early senescent plants 2002), (2) microenvironment (R = rhizosphere, P = phyllosphere, Re = endorhiza, Pe = endosphere), (3) plant number (1–4), and 4. consecutive number of the isolate per plant.
2.2 DNA extraction and T-RFLP analysis of bacterial communities
Bacterial pellets recovered from different microenvironments were obtained by differential centrifugation and stored at −70 °C. From these pellets total DNA was extracted using FastDNA Spin Kit for Soil (rhizosphere, endorhiza and endosphere) and FastDNA Kit (phyllosphere) (Bio101, Carlsbad, CA). The community DNA obtained from the rhizosphere was additionally cleaned with GENECLEAN Turbo Nucleic Acid Purification Kit (Bio101). 16S rDNA fragments from community DNA were amplified with the eubacterial primers 799f fluorescently-labelled with 6-FAM and 1525r[23]. Each 50 μl reaction mixture contained 1 PCR buffer, 1.75 μM MgCl2, 200 μM each deoxynucleoside triphosphate, 25 pmol of each primer, and 2.5 U of Taq DNA polymerase (Applied Biosystems, Foster City, CA). DNA amplification was performed using the following program: 3 min denaturation at 95 °C followed by 30 cycles of: 20 s at 94 °C, 40 s at 53 °C and 40 s at 72 °C, and a final extension for 7 min at 72 °C. The PCR products were purified with a Qiaquick PCR purification kit (Qiagen, Hilden, Germany). Approximately 100 ng of amplified 16S rDNA was digested with Hha I (New England Biolabs, Beverly, MA) and precipitated with 75% isopropanol. Precipitated DNA was washed twice with 75% isopropanol, vacuum dried and resuspended in 20 μl water. The sample was mixed with 0.4 μl of GeneScan 1000 Rox size standard, denaturated at 95 °C for 2 min, immediately placed on ice, and evaluated following electrophoresis in POP6 polymer with an ABI 310 DNA sequencer. Terminal fragment sizes between 40 and 800 bp were determined using the GeneScan Analytical Software v.3.1.2 (Applied Biosystems). T-RFLP profiles were standardised in a similar way as suggested by Dunbar et al.[24]. First, the sum of peak heights was calculated as an indication of the total DNA quantity in each profile. The fluorescence of each peak was adjusted to the smallest DNA quantity by multiplying with a factor given by the quotient of the smallest and the corresponding DNA quantity. Only peaks above the threshold of 50 fluorescence units were considered. At the end, the identity of peaks was determined by manual grouping based on the size of peaks in base pairs as well as evaluating the pattern of peaks. Computer-assisted comparison of T-RFLP generated fingerprints was made using the GelCompar II program (Kortrijk, Belgium). The cluster analysis was performed using a Pearson Correlation matrix and the UPGMA (unweighted pair-group method using arithmetic averages) algorithm based on the densitometric curves of the T-RFLP profiles.
2.3 Screening of bacteria for antagonism to V. dahlia and R. solani
All bacterial isolates were screened for their activity to the two highly pathogenic isolates of V. dahliae Kleb. ELV 16 and R. solani Kühn RHI SO 325, both isolated from potato and available from the culture collection of the Department of Microbiology, Rostock University [25,26]. Isolates were assessed in a dual culture in vitro on Waksman agar containing 5 g proteose-peptone (Merck, Darmstadt, Germany), 10 g glucose (Merck), 3 g meat extract (Oxoid, Hampshire, England), 5 g NaCl (Merck), 20 g agar (Difco), filled up to 1 l with distilled water and adjusted to pH 6.8. After 5 days of incubation at 20 °C, zones of inhibition were measured according to Berg et al.[25]. The two plant pathogenic fungi were routinely grown on Czapek Dox medium (Gibco).
2.4 Identification of plant-associated bacteria and determination of diversity indices
All isolates were identified based on their cellular fatty acids following fatty acid methyl ester gas chromatography (FAME-GC) as described by Sasser[27] and characterized by the MIDI system (Microbial Identification System, Inc., Newark, DE). Antagonistic isolates were additionally identified by sequencing of about 700 bases of the 16S rRNA gene, covering the variable regions v1–v4. Sequences obtained were aligned with the reference 16S rRNA gene sequence using BLAST algorithm according to Altschul et al.[28]. The general species diversity of the bacterial communities was measured by the Shannon information theory function[29]. Species richness expressed as the number of species (S) as a function (ratio) of the total number of individuals (N) was determined by the index (d) proposed by Menhinick[30].
2.5 Bacterial characterization based on production of hydrolytic enzymes and secondary metabolites
Production of cell wall-degrading enzymes and antifungal secondary metabolites are common mechanism bacteria use to inhibit fungal growth. For a better characterization of the antagonistic bacterial isolates, their potential to produce hydrolytic enzymes and secondary metabolites was studied. Chitinase activity (β-1,4-glucosamine polymer degradation) and pectinase activity (pectin degradation) was tested in minimal medium, according to Chernin et al.[31]. Clearing zones were detected 5 days after incubation at 20 °C. β-Glucanase and cellulase activity was tested using chromogenic azurine-dyed cross-linked (AZCL) and reamazolbrilliant blue R (AZO) substrates (Megazyme, Bray, Ireland), respectively. Formation of blue haloes was recorded until 5 days after incubation. Protease activity indicated by casein degradation was determined from clearing zones in skim milk agar (50 ml sterilized skimmed milk mixed at 55 °C with 50 ml 1/5 TSA and 4% agar) after 5 days of incubation at 20 °C.
The ability of bacterial isolates to produce indole-3-acetic acid (IAA) was determined using the microplate method developed by Sawar and Kremer[32].
The antifungal antibiotic 2,4-diacetyl-phloroglucinol (Phl) is responsible for the antifungal activity of many Pseudomonas strains. To detect the phl D gene a PCR approach was used. Amplification with the gene-specific primers Phl2[33] were performed in the following mix: 1 μl target DNA, 10 pmol of each primer and 17 μl PCR SuperMix High Fidelity (Gibco). PCR was performed under the following conditions: 3 min at 95 °C followed by 29 cycles consisting of 1 min at 94 °C, 45 s at 48 °C and 45 s at 72 °C. PCR was finished by a primer extension step at 72 °C for 5 min. PCR-amplified DNAs were detected by gel electrophoresis using a 1.5% agarose gel. The gels were stained with ethidium bromide for 30 min, and the PCR products were visualized with a UV transilluminator. The reproducibility of the results was verified in two independent experiments.
2.6 BOX-PCR genomic fingerprints
In a final step the antagonistic bacterial isolates were characterized by molecular means. Bacterial DNA was prepared using Qiagen Genomic tips (Qiagen). BOX-PCR was done as described by Rademaker and De Bruijn[34] using the BOXA1R primer 5′-CTA CGG CAA GGC GAC GCT GAC G-3′. Amplification was performed with a Peltier Thermal Cycler PTC-200 (Biozym Diagnostic, Hessisch Oldendorf, Germany) using an initial denaturation step at 95 °C for 6 min, and subsequently 35 cycles of denaturation at 94 °C for 1 min, annealing at 53 °C for 1 min and extension at 65 °C for 8 min followed by a final extension at 65 °C for 16 min. A 10-μl aliquot of amplified PCR product was separated by gel electrophoresis on 1.5% agarose gels in 0.5 × TBE buffer for 4 h, stained with ethidium bromide, and photographed under UV transillumination. The reproducibility of the results was verified in three independent experiments. Computer-assisted evaluation of BOX-PCR generated fingerprints was made using the GelCompar program (Kortrijk, Belgium). The cluster analysis was performed with unweighted pair-group average (UPGMA) algorithm.
2.7 Nucleotide sequence accession numbers
The 16S rRNA gene sequences were deposited in GenBank and given Accession Nos. AJ581118–AJ581119 and AJ581922–AJ581992.
3 Results
3.1 Molecular fingerprinting of potato-associated bacterial communities
Total community DNA was extracted from the microbial pellets recovered from the four microenvironments and amplified 16S rDNA fragments were analyzed by T-RFLP. The highest diversity was found in the rhizosphere as indicated by higher numbers of T-RFs relative to other microenvironments. Common T-RF peaks were found for the rhizosphere and endorhiza, and also for the endorhiza and endosphere. Selected T-RFs, which frequently occurred in all plants were used for further comparison of non-culturable bacteria (Table 1). Nine out of 19 peaks were found in all four microenvironments. Species represented by peak numbers 7, 9 and 19 were the most abundant bacteria in all microenvironments. Three peaks (numbers 13, 14, 15) were found only in the endorhiza and endosphere, while peak numbers 3 and 11 occurred only in the rhizosphere and phyllosphere. Rhizosphere was the only microenvironment with specific bacterial groups (peak numbers 10, 16). The highest number of peaks was found in the rhizosphere (16 peaks) and endosphere (15 peaks) while 13 peaks were found in the endorhiza and 12 in the phyllosphere. During the vegetation period, the number of common peaks increased in the phyllosphere and the rhizosphere as well as in the endosphere and endorhiza. A comparison with 16S rDNA sequences from antagonistic bacteria, which were obtained by cultivation (see below), allowed the identification of some T-RF peaks. For example, peak number 7 (44 bp) contained antagonistic isolates of the species Curtobacterium flaccumfaciens. Peak number 11 (74.3 bp) included more than one genus; the special region of the 16S rRNA gene used in T-RFLP resulted in the same fragment size for species of the genera Agrobacterium, Arthrobacter and Micrococcus. All isolates of the different Pseudomonas species were found together in one peak, i.e., number 12. There were some differences between the real length of the terminal fragments in nucleotides and the detected size. Thus, the terminal fragment with a size of 77 bp produced a reproducible peak at 75.3 bp.
Selected peaks obtained by T-RFLP analysis and their occurrence in four bacterial communities isolated from the rhizosphere, phyllosphere, endorhiza and endosphere of potato
T-RF | Number: | 1 | 2 | 3 | 4 | 5 | 6 | 7 | 8 | 9 | 10 | 11 | 12 | 13 | 14 | 15 | 16 | 17 | 18 | 19 |
Fragment size: | 31.7 | 32.9 | 35.2 | 36.4 | 37.6 | 42.8 | 44 | 51.9 | 54.5 | 57.5 | 74.3 | 75.3 | 84.1 | 85.1 | 107 | 193 | 334 | 336 | 338 | |
Occurrence in | Habitat/growth stagea | |||||||||||||||||||
Rhizosphere | Young plant | ◻ | ◻ | ◻ | ◻ | ◻ | ◻ | ◻ | ◻ | ◻ | ◻ | ◻ | ◻ | ◻ | ||||||
Flowering plant | ◻ | ◻ | ◻ | ◻ | ◻ | ◻ | ◻ | ◻ | ◻ | ◻ | ◻ | ◻ | ◻ | ◻ | ||||||
Early senescent plant | ◻ | ◻ | ◻ | ◻ | ◻ | ◻ | ◻ | ◻ | ◻ | ◻ | ◻ | ◻ | ◻ | ◻ | ◻ | ◻ | ||||
Phyllosphere | Young plant | ◻ | ◻ | ◻ | ◻ | ◻ | ◻ | ◻ | ◻ | ◻ | ◻ | ◻ | ||||||||
Flowering plant | ◻ | ◻ | ◻ | ◻ | ◻ | ◻ | ◻ | ◻ | ◻ | ◻ | ◻ | |||||||||
Early senescent plant | ◻ | ◻ | ◻ | ◻ | ◻ | ◻ | ◻ | ◻ | ◻ | ◻ | ||||||||||
Endorhiza | Young plant | ◻ | ◻ | ◻ | ◻ | ◻ | ◻ | ◻ | ◻ | ◻ | ◻ | ◻ | ||||||||
Flowering plant | ◻ | ◻ | ◻ | ◻ | ◻ | ◻ | ◻ | ◻ | ◻ | ◻ | ◻ | ◻ | ◻ | |||||||
Early senescent plant | ◻ | ◻ | ◻ | ◻ | ◻ | |||||||||||||||
Endosphere | Young plant | ◻ | ◻ | ◻ | ◻ | ◻ | ◻ | ◻ | ◻ | ◻ | ◻ | ◻ | ◻ | |||||||
Flowering plant | ◻ | ◻ | ◻ | ◻ | ◻ | ◻ | ◻ | ◻ | ◻ | ◻ | ||||||||||
Early senescent plant | ◻ | ◻ | ◻ | ◻ | ◻ | ◻ | ◻ | ◻ |
T-RF | Number: | 1 | 2 | 3 | 4 | 5 | 6 | 7 | 8 | 9 | 10 | 11 | 12 | 13 | 14 | 15 | 16 | 17 | 18 | 19 |
Fragment size: | 31.7 | 32.9 | 35.2 | 36.4 | 37.6 | 42.8 | 44 | 51.9 | 54.5 | 57.5 | 74.3 | 75.3 | 84.1 | 85.1 | 107 | 193 | 334 | 336 | 338 | |
Occurrence in | Habitat/growth stagea | |||||||||||||||||||
Rhizosphere | Young plant | ◻ | ◻ | ◻ | ◻ | ◻ | ◻ | ◻ | ◻ | ◻ | ◻ | ◻ | ◻ | ◻ | ||||||
Flowering plant | ◻ | ◻ | ◻ | ◻ | ◻ | ◻ | ◻ | ◻ | ◻ | ◻ | ◻ | ◻ | ◻ | ◻ | ||||||
Early senescent plant | ◻ | ◻ | ◻ | ◻ | ◻ | ◻ | ◻ | ◻ | ◻ | ◻ | ◻ | ◻ | ◻ | ◻ | ◻ | ◻ | ||||
Phyllosphere | Young plant | ◻ | ◻ | ◻ | ◻ | ◻ | ◻ | ◻ | ◻ | ◻ | ◻ | ◻ | ||||||||
Flowering plant | ◻ | ◻ | ◻ | ◻ | ◻ | ◻ | ◻ | ◻ | ◻ | ◻ | ◻ | |||||||||
Early senescent plant | ◻ | ◻ | ◻ | ◻ | ◻ | ◻ | ◻ | ◻ | ◻ | ◻ | ||||||||||
Endorhiza | Young plant | ◻ | ◻ | ◻ | ◻ | ◻ | ◻ | ◻ | ◻ | ◻ | ◻ | ◻ | ||||||||
Flowering plant | ◻ | ◻ | ◻ | ◻ | ◻ | ◻ | ◻ | ◻ | ◻ | ◻ | ◻ | ◻ | ◻ | |||||||
Early senescent plant | ◻ | ◻ | ◻ | ◻ | ◻ | |||||||||||||||
Endosphere | Young plant | ◻ | ◻ | ◻ | ◻ | ◻ | ◻ | ◻ | ◻ | ◻ | ◻ | ◻ | ◻ | |||||||
Flowering plant | ◻ | ◻ | ◻ | ◻ | ◻ | ◻ | ◻ | ◻ | ◻ | ◻ | ||||||||||
Early senescent plant | ◻ | ◻ | ◻ | ◻ | ◻ | ◻ | ◻ | ◻ |
a◻ detected on/in one or two plants; ◻ detected on/in three or more plants.
Selected peaks obtained by T-RFLP analysis and their occurrence in four bacterial communities isolated from the rhizosphere, phyllosphere, endorhiza and endosphere of potato
T-RF | Number: | 1 | 2 | 3 | 4 | 5 | 6 | 7 | 8 | 9 | 10 | 11 | 12 | 13 | 14 | 15 | 16 | 17 | 18 | 19 |
Fragment size: | 31.7 | 32.9 | 35.2 | 36.4 | 37.6 | 42.8 | 44 | 51.9 | 54.5 | 57.5 | 74.3 | 75.3 | 84.1 | 85.1 | 107 | 193 | 334 | 336 | 338 | |
Occurrence in | Habitat/growth stagea | |||||||||||||||||||
Rhizosphere | Young plant | ◻ | ◻ | ◻ | ◻ | ◻ | ◻ | ◻ | ◻ | ◻ | ◻ | ◻ | ◻ | ◻ | ||||||
Flowering plant | ◻ | ◻ | ◻ | ◻ | ◻ | ◻ | ◻ | ◻ | ◻ | ◻ | ◻ | ◻ | ◻ | ◻ | ||||||
Early senescent plant | ◻ | ◻ | ◻ | ◻ | ◻ | ◻ | ◻ | ◻ | ◻ | ◻ | ◻ | ◻ | ◻ | ◻ | ◻ | ◻ | ||||
Phyllosphere | Young plant | ◻ | ◻ | ◻ | ◻ | ◻ | ◻ | ◻ | ◻ | ◻ | ◻ | ◻ | ||||||||
Flowering plant | ◻ | ◻ | ◻ | ◻ | ◻ | ◻ | ◻ | ◻ | ◻ | ◻ | ◻ | |||||||||
Early senescent plant | ◻ | ◻ | ◻ | ◻ | ◻ | ◻ | ◻ | ◻ | ◻ | ◻ | ||||||||||
Endorhiza | Young plant | ◻ | ◻ | ◻ | ◻ | ◻ | ◻ | ◻ | ◻ | ◻ | ◻ | ◻ | ||||||||
Flowering plant | ◻ | ◻ | ◻ | ◻ | ◻ | ◻ | ◻ | ◻ | ◻ | ◻ | ◻ | ◻ | ◻ | |||||||
Early senescent plant | ◻ | ◻ | ◻ | ◻ | ◻ | |||||||||||||||
Endosphere | Young plant | ◻ | ◻ | ◻ | ◻ | ◻ | ◻ | ◻ | ◻ | ◻ | ◻ | ◻ | ◻ | |||||||
Flowering plant | ◻ | ◻ | ◻ | ◻ | ◻ | ◻ | ◻ | ◻ | ◻ | ◻ | ||||||||||
Early senescent plant | ◻ | ◻ | ◻ | ◻ | ◻ | ◻ | ◻ | ◻ |
T-RF | Number: | 1 | 2 | 3 | 4 | 5 | 6 | 7 | 8 | 9 | 10 | 11 | 12 | 13 | 14 | 15 | 16 | 17 | 18 | 19 |
Fragment size: | 31.7 | 32.9 | 35.2 | 36.4 | 37.6 | 42.8 | 44 | 51.9 | 54.5 | 57.5 | 74.3 | 75.3 | 84.1 | 85.1 | 107 | 193 | 334 | 336 | 338 | |
Occurrence in | Habitat/growth stagea | |||||||||||||||||||
Rhizosphere | Young plant | ◻ | ◻ | ◻ | ◻ | ◻ | ◻ | ◻ | ◻ | ◻ | ◻ | ◻ | ◻ | ◻ | ||||||
Flowering plant | ◻ | ◻ | ◻ | ◻ | ◻ | ◻ | ◻ | ◻ | ◻ | ◻ | ◻ | ◻ | ◻ | ◻ | ||||||
Early senescent plant | ◻ | ◻ | ◻ | ◻ | ◻ | ◻ | ◻ | ◻ | ◻ | ◻ | ◻ | ◻ | ◻ | ◻ | ◻ | ◻ | ||||
Phyllosphere | Young plant | ◻ | ◻ | ◻ | ◻ | ◻ | ◻ | ◻ | ◻ | ◻ | ◻ | ◻ | ||||||||
Flowering plant | ◻ | ◻ | ◻ | ◻ | ◻ | ◻ | ◻ | ◻ | ◻ | ◻ | ◻ | |||||||||
Early senescent plant | ◻ | ◻ | ◻ | ◻ | ◻ | ◻ | ◻ | ◻ | ◻ | ◻ | ||||||||||
Endorhiza | Young plant | ◻ | ◻ | ◻ | ◻ | ◻ | ◻ | ◻ | ◻ | ◻ | ◻ | ◻ | ||||||||
Flowering plant | ◻ | ◻ | ◻ | ◻ | ◻ | ◻ | ◻ | ◻ | ◻ | ◻ | ◻ | ◻ | ◻ | |||||||
Early senescent plant | ◻ | ◻ | ◻ | ◻ | ◻ | |||||||||||||||
Endosphere | Young plant | ◻ | ◻ | ◻ | ◻ | ◻ | ◻ | ◻ | ◻ | ◻ | ◻ | ◻ | ◻ | |||||||
Flowering plant | ◻ | ◻ | ◻ | ◻ | ◻ | ◻ | ◻ | ◻ | ◻ | ◻ | ||||||||||
Early senescent plant | ◻ | ◻ | ◻ | ◻ | ◻ | ◻ | ◻ | ◻ |
a◻ detected on/in one or two plants; ◻ detected on/in three or more plants.
Cluster analysis based on all T-RFLP profiles obtained in 2001 clearly demonstrated that highly specific community composition was found in the different microenvironments (Fig. 1(a)–(c)). Profiles of the same microenvironment though usually clustered together. However, the branches of the microenvironments showed a different relationship to each other depending on the sampling times studied. In young plants, endosphere and phyllosphere were grouped together whereas the endosphere in flowering plants were clearly separated from the other microenvironments. In senescent plants, both endophytic communities displayed similarities and could not be clearly distinguished. At this sampling point the endosphere and endorhiza were separated from the two other microenvironments.
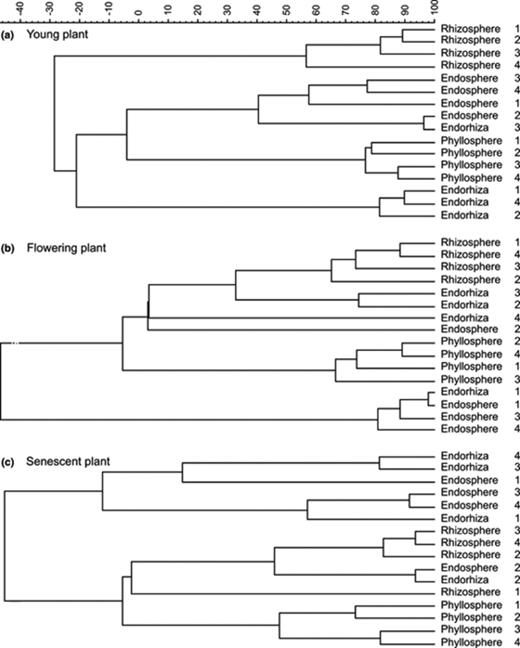
UPGMA dendrogram generated from the T-RFLP community profiles of (a) young plants, (b) flowering plants, and (c) senescent plants. Numbers indicate individual plants representing the four replicates of each habitat and sampling time.
3.2 Population densities of potato-associated bacteria in different microenvironments
The highest abundance of culturable bacteria was found in the potato rhizosphere with 7.6 × 107 CFU g [fresh wt]− in 2001 and 3.6 × 107 CFU g [fresh wt]− in 2002. Following was the endorhiza with 2.0 × 107 CFU g [fresh wt]− in 2001 and 2.3 × 105 CFU g [fresh wt]− in 2002, and the phyllosphere with 1.1 × 106 CFU g [fresh wt]− in 2001, and 9.4 × 104 CFU g [fresh wt]− in 2002. The lowest population density occurred in the endosphere with 6.7 × 105 CFU g [fresh wt]− in 2001, and 1.1 × 103 CFU g [fresh wt]− in 2002. Interestingly, the population densities increased in all microenvironments during the vegetation period with the exception of the endosphere where the population significantly decreased.
3.3 Screening of bacteria for antagonism to V. dahliae and R. solani
A total of 2648 bacterial isolates were screened for their antagonistic ability to suppress growth of V. dahliae and R. solani in an in vitro dual culture assay (Table 2). A total of 349 (= 14.4%) of the isolates were found antagonistic against one or both fungal pathogens. In general, more isolates were antagonistic to R. solani (301 isolates = 8%) than to V. dahliae (208 isolates = 8%). Although similar numbers of isolates were tested from each microenvironment the proportion of antagonists varied depending on microenvironment and sampling time. The lowest variability was found in the rhizosphere; the proportion of antagonists varied between 3% and 13%. In contrast, the highest variability was observed in the phyllosphere. While in the rhizosphere and endosphere antagonistic bacteria occurred in all samples, samples of the phyllosphere and endosphere sometimes yielded no antagonists at all. The number of isolates with antifungal activity against V. dahliae was highest in the endorhiza (124), followed by the rhizosphere (47), phyllosphere (33), and endosphere (4). In contrast, the number of isolates with antifungal activity against R. solani was the highest in the phyllosphere (137), followed by the endorhiza (80), rhizosphere (66), and endosphere (18). Altogether, 126 isolates were antagonistic to both fungal pathogens. The highest number of multitarget antagonists was found in the endorhiza followed by the rhizosphere. In contrast to R. solani, the proportion of antagonists to V. dahliae increased during the vegetation period.
Proportion of bacterial isolates antagonistic towards Verticillium dahliae and/or Rhizoctonia solani
Origin of strains microenvironment | Growth stage of plant | Year | Isolates tested | Antagonistic towardaV.d. | Antagonistic toward R.s. | Toward both | |||
Number | % | Number | % | Number | % | ||||
Rhizosphere | Young plant | 2001 | 105 | 5 | 5 | 12 | 11 | 4 | 4 |
2002 | 120 | 4 | 3 | 10 | 8 | 4 | 3 | ||
Flowering | 2001 | 107 | 9 | 8 | 14 | 13 | 8 | 7 | |
2002 | 150 | 10 | 7 | 11 | 7 | 5 | 3 | ||
Senescent plant | 2001 | 107 | 8 | 7 | 8 | 7 | 5 | 5 | |
2002 | 150 | 11 | 7 | 11 | 7 | 4 | 3 | ||
Phyllosphere | Young plant | 2001 | 93 | 1 | 1 | 3 | 3 | 1 | 1 |
2002 | 119 | 30 | 25 | 87 | 73 | 26 | 22 | ||
Flowering | 2001 | 97 | 0 | 0 | 11 | 11 | 0 | 0 | |
2002 | 126 | 2 | 2 | 34 | 27 | 2 | 2 | ||
Senescent plant | 2001 | 116 | 0 | 0 | 2 | 2 | 0 | 0 | |
2002 | 150 | 0 | 0 | 0 | 0 | 0 | 0 | ||
Endorhiza | Young plant | 2001 | 71 | 1 | 1 | 1 | 1 | 1 | 1 |
2002 | 77 | 2 | 3 | 5 | 6 | 2 | 3 | ||
Flowering | 2001 | 146 | 24 | 16 | 30 | 21 | 20 | 14 | |
2002 | 150 | 23 | 15 | 23 | 15 | 22 | 15 | ||
Senescent plant | 2001 | 118 | 16 | 14 | 10 | 8 | 8 | 7 | |
2002 | 149 | 58 | 39 | 11 | 7 | 10 | 7 | ||
Endosphere | Young plant | 2001 | 64 | 2 | 3 | 4 | 6 | 2 | 3 |
2002 | 39 | 1 | 3 | 1 | 3 | 1 | 3 | ||
Flowering | 2001 | 72 | 1 | 1 | 3 | 4 | 1 | 1 | |
2002 | 112 | 0 | 0 | 2 | 2 | 0 | 0 | ||
Senescent plant | 2001 | 117 | 0 | 0 | 8 | 7 | 0 | 0 | |
2002 | 93 | 0 | 0 | 0 | 0 | 0 | 0 |
Origin of strains microenvironment | Growth stage of plant | Year | Isolates tested | Antagonistic towardaV.d. | Antagonistic toward R.s. | Toward both | |||
Number | % | Number | % | Number | % | ||||
Rhizosphere | Young plant | 2001 | 105 | 5 | 5 | 12 | 11 | 4 | 4 |
2002 | 120 | 4 | 3 | 10 | 8 | 4 | 3 | ||
Flowering | 2001 | 107 | 9 | 8 | 14 | 13 | 8 | 7 | |
2002 | 150 | 10 | 7 | 11 | 7 | 5 | 3 | ||
Senescent plant | 2001 | 107 | 8 | 7 | 8 | 7 | 5 | 5 | |
2002 | 150 | 11 | 7 | 11 | 7 | 4 | 3 | ||
Phyllosphere | Young plant | 2001 | 93 | 1 | 1 | 3 | 3 | 1 | 1 |
2002 | 119 | 30 | 25 | 87 | 73 | 26 | 22 | ||
Flowering | 2001 | 97 | 0 | 0 | 11 | 11 | 0 | 0 | |
2002 | 126 | 2 | 2 | 34 | 27 | 2 | 2 | ||
Senescent plant | 2001 | 116 | 0 | 0 | 2 | 2 | 0 | 0 | |
2002 | 150 | 0 | 0 | 0 | 0 | 0 | 0 | ||
Endorhiza | Young plant | 2001 | 71 | 1 | 1 | 1 | 1 | 1 | 1 |
2002 | 77 | 2 | 3 | 5 | 6 | 2 | 3 | ||
Flowering | 2001 | 146 | 24 | 16 | 30 | 21 | 20 | 14 | |
2002 | 150 | 23 | 15 | 23 | 15 | 22 | 15 | ||
Senescent plant | 2001 | 118 | 16 | 14 | 10 | 8 | 8 | 7 | |
2002 | 149 | 58 | 39 | 11 | 7 | 10 | 7 | ||
Endosphere | Young plant | 2001 | 64 | 2 | 3 | 4 | 6 | 2 | 3 |
2002 | 39 | 1 | 3 | 1 | 3 | 1 | 3 | ||
Flowering | 2001 | 72 | 1 | 1 | 3 | 4 | 1 | 1 | |
2002 | 112 | 0 | 0 | 2 | 2 | 0 | 0 | ||
Senescent plant | 2001 | 117 | 0 | 0 | 8 | 7 | 0 | 0 | |
2002 | 93 | 0 | 0 | 0 | 0 | 0 | 0 |
aDual culture assay against V. d. =Verticillium dahliae and R.s.=Rhizoctonia solani, active isolates with more than 5 mm wide zone of inhibition.
Proportion of bacterial isolates antagonistic towards Verticillium dahliae and/or Rhizoctonia solani
Origin of strains microenvironment | Growth stage of plant | Year | Isolates tested | Antagonistic towardaV.d. | Antagonistic toward R.s. | Toward both | |||
Number | % | Number | % | Number | % | ||||
Rhizosphere | Young plant | 2001 | 105 | 5 | 5 | 12 | 11 | 4 | 4 |
2002 | 120 | 4 | 3 | 10 | 8 | 4 | 3 | ||
Flowering | 2001 | 107 | 9 | 8 | 14 | 13 | 8 | 7 | |
2002 | 150 | 10 | 7 | 11 | 7 | 5 | 3 | ||
Senescent plant | 2001 | 107 | 8 | 7 | 8 | 7 | 5 | 5 | |
2002 | 150 | 11 | 7 | 11 | 7 | 4 | 3 | ||
Phyllosphere | Young plant | 2001 | 93 | 1 | 1 | 3 | 3 | 1 | 1 |
2002 | 119 | 30 | 25 | 87 | 73 | 26 | 22 | ||
Flowering | 2001 | 97 | 0 | 0 | 11 | 11 | 0 | 0 | |
2002 | 126 | 2 | 2 | 34 | 27 | 2 | 2 | ||
Senescent plant | 2001 | 116 | 0 | 0 | 2 | 2 | 0 | 0 | |
2002 | 150 | 0 | 0 | 0 | 0 | 0 | 0 | ||
Endorhiza | Young plant | 2001 | 71 | 1 | 1 | 1 | 1 | 1 | 1 |
2002 | 77 | 2 | 3 | 5 | 6 | 2 | 3 | ||
Flowering | 2001 | 146 | 24 | 16 | 30 | 21 | 20 | 14 | |
2002 | 150 | 23 | 15 | 23 | 15 | 22 | 15 | ||
Senescent plant | 2001 | 118 | 16 | 14 | 10 | 8 | 8 | 7 | |
2002 | 149 | 58 | 39 | 11 | 7 | 10 | 7 | ||
Endosphere | Young plant | 2001 | 64 | 2 | 3 | 4 | 6 | 2 | 3 |
2002 | 39 | 1 | 3 | 1 | 3 | 1 | 3 | ||
Flowering | 2001 | 72 | 1 | 1 | 3 | 4 | 1 | 1 | |
2002 | 112 | 0 | 0 | 2 | 2 | 0 | 0 | ||
Senescent plant | 2001 | 117 | 0 | 0 | 8 | 7 | 0 | 0 | |
2002 | 93 | 0 | 0 | 0 | 0 | 0 | 0 |
Origin of strains microenvironment | Growth stage of plant | Year | Isolates tested | Antagonistic towardaV.d. | Antagonistic toward R.s. | Toward both | |||
Number | % | Number | % | Number | % | ||||
Rhizosphere | Young plant | 2001 | 105 | 5 | 5 | 12 | 11 | 4 | 4 |
2002 | 120 | 4 | 3 | 10 | 8 | 4 | 3 | ||
Flowering | 2001 | 107 | 9 | 8 | 14 | 13 | 8 | 7 | |
2002 | 150 | 10 | 7 | 11 | 7 | 5 | 3 | ||
Senescent plant | 2001 | 107 | 8 | 7 | 8 | 7 | 5 | 5 | |
2002 | 150 | 11 | 7 | 11 | 7 | 4 | 3 | ||
Phyllosphere | Young plant | 2001 | 93 | 1 | 1 | 3 | 3 | 1 | 1 |
2002 | 119 | 30 | 25 | 87 | 73 | 26 | 22 | ||
Flowering | 2001 | 97 | 0 | 0 | 11 | 11 | 0 | 0 | |
2002 | 126 | 2 | 2 | 34 | 27 | 2 | 2 | ||
Senescent plant | 2001 | 116 | 0 | 0 | 2 | 2 | 0 | 0 | |
2002 | 150 | 0 | 0 | 0 | 0 | 0 | 0 | ||
Endorhiza | Young plant | 2001 | 71 | 1 | 1 | 1 | 1 | 1 | 1 |
2002 | 77 | 2 | 3 | 5 | 6 | 2 | 3 | ||
Flowering | 2001 | 146 | 24 | 16 | 30 | 21 | 20 | 14 | |
2002 | 150 | 23 | 15 | 23 | 15 | 22 | 15 | ||
Senescent plant | 2001 | 118 | 16 | 14 | 10 | 8 | 8 | 7 | |
2002 | 149 | 58 | 39 | 11 | 7 | 10 | 7 | ||
Endosphere | Young plant | 2001 | 64 | 2 | 3 | 4 | 6 | 2 | 3 |
2002 | 39 | 1 | 3 | 1 | 3 | 1 | 3 | ||
Flowering | 2001 | 72 | 1 | 1 | 3 | 4 | 1 | 1 | |
2002 | 112 | 0 | 0 | 2 | 2 | 0 | 0 | ||
Senescent plant | 2001 | 117 | 0 | 0 | 8 | 7 | 0 | 0 | |
2002 | 93 | 0 | 0 | 0 | 0 | 0 | 0 |
aDual culture assay against V. d. =Verticillium dahliae and R.s.=Rhizoctonia solani, active isolates with more than 5 mm wide zone of inhibition.
3.4 Diversity of potato-associated antagonistic bacteria in different microenvironments
Of the total 349 antagonistic isolates, 316 were identified to the species or genus level by FAME-GC and/or partial sequencing of the 16S rRNA gene (Table 3). Altogether, 40 bacterial species could be identified. The highest number of species with antagonistic properties was isolated from the rhizosphere (25) and endorhiza (16) while 14 and 10 species were found in the phyllosphere and endosphere, respectively. Richness and diversity indices confirmed the specificity of microenvironments and showed a high diversity of bacterial antagonists in the rhizosphere. The lowest number of species with antagonistic potential was found in the phyllosphere. Two species were obtained from all microenvironments, i.e., Bacillus pumilus and Bacillus subtilis. Microenvironments, which shared the most species were the endorhiza and rhizosphere with ten species, while rhizosphere and phyllosphere shared three species. A low similarity with regard to antagonistic species was found between the endorhiza and the endosphere with only five common bacterial species. These were, in addition to the two Bacillus species occurring in all microenvironments, Agrobacterium tumefaciens, Erwinia persicinus, and Pseudomonas fluorescens. In the ectophytic microenvironments five additional species occurred in common: Bacillus amyloliquefaciens, Pseudomonas chlororaphis, P. putida, Staphylococcus epidermidis, and Staphylococcus pasteuri.
List of bacterial antagonistic species isolated from different microenvironments of potato
Speciesa/microenvironment | Rhizosphere | Phyllosphere | Endorhiza | Endosphere |
Agrobacterium tumefaciens | 1 (0) | 1 (0) | 1 (0) | |
Arthrobacter globiformis | 2 (1) | |||
Arthrobacter ilicis | 1 (1) | |||
Arthobacter oxydans | 1 (1) | |||
Bacillus amyloliquefaciens | 1 (1) | 3 (3) | ||
Bacillus aquamarinus | 1(0) | |||
Bacillus cereus | 3 (3) | |||
Bacillus licheniformis | 1 (1) | 1 (1) | ||
Bacillus mycoides | 1 (1) | |||
Bacillus pumilus | 4 (4) | 56 (54) | 2 (2) | 1 (0) |
Bacillus subtilis | 2 (0) | 32 (28) | 6 (2) | 5 (1) |
Bacillus thuringiensis | 1 (0) | |||
Curtobacterium flaccumfaciens | 4 (3) | |||
Enterobacter taylorae | 1 (1) | |||
Erwinia amylovora | 1 (1) | |||
Erwinia persicinus | 4 (0) | 1 (0) | 1 (0) | |
Erwinia rhapontici | 1 (0) | |||
Lysobacter antibioticus | 1 (0) | |||
Micrococcus kristinae | 1 (1) | |||
Paenibacillus peoriae | 1 (0) | |||
Paenibacillus polymyxa | 2 (2) | 1 (1) | ||
Pseudomonas chlororaphis | 6 (6) | 21 (21) | 1 (1) | |
Pseudomonas corrugata | 2 (2) | 7 (7) | ||
Pseudomonas fluorescens | 13 (5) | 17 (4) | 1 (0) | |
Pseudomonas jessenii | 1 (0) | |||
Pseudomonas marginalis | 2 (2) | |||
Pseudomonas migulae | 2 (0) | |||
Pseudomonas orientalis | 1 (0) | |||
Pseudomonas putida | 12 (12) | 9 (9) | 48 (46) | |
Pseudomonas reactans | 1 (0) | 2 (0) | ||
Pseudomonas savastanoi pv. fraxinus | 2 (2) | 6 (6) | ||
Pseudomonas straminea | 1 (0) | |||
Pseudomonas syringae | 4 (4) | |||
Rhodococcus erythropolis | 1 (1) | |||
Serratia plymuthica | 1 (0) | |||
Shingobacterium spiritovorum | 1 (1) | |||
Staphylococcus epidermidis | 1 (0) | 1 (0) | ||
Staphylococcus pasteuri | 1 (0) | 1 (0) | ||
Streptomyces halstedii | 1 (1) | |||
Variovorax paradoxus | 2 (0) | 1 (1) | ||
Total no. of species | 25 | 14 | 16 | 10 |
Total no of isolates | 64 | 134 | 101 | 17 |
Richness | 3.1 | 1.2 | 1.6 | 2.4 |
Diversity | 2.7 | 1.7 | 1.9 | 2.0 |
Speciesa/microenvironment | Rhizosphere | Phyllosphere | Endorhiza | Endosphere |
Agrobacterium tumefaciens | 1 (0) | 1 (0) | 1 (0) | |
Arthrobacter globiformis | 2 (1) | |||
Arthrobacter ilicis | 1 (1) | |||
Arthobacter oxydans | 1 (1) | |||
Bacillus amyloliquefaciens | 1 (1) | 3 (3) | ||
Bacillus aquamarinus | 1(0) | |||
Bacillus cereus | 3 (3) | |||
Bacillus licheniformis | 1 (1) | 1 (1) | ||
Bacillus mycoides | 1 (1) | |||
Bacillus pumilus | 4 (4) | 56 (54) | 2 (2) | 1 (0) |
Bacillus subtilis | 2 (0) | 32 (28) | 6 (2) | 5 (1) |
Bacillus thuringiensis | 1 (0) | |||
Curtobacterium flaccumfaciens | 4 (3) | |||
Enterobacter taylorae | 1 (1) | |||
Erwinia amylovora | 1 (1) | |||
Erwinia persicinus | 4 (0) | 1 (0) | 1 (0) | |
Erwinia rhapontici | 1 (0) | |||
Lysobacter antibioticus | 1 (0) | |||
Micrococcus kristinae | 1 (1) | |||
Paenibacillus peoriae | 1 (0) | |||
Paenibacillus polymyxa | 2 (2) | 1 (1) | ||
Pseudomonas chlororaphis | 6 (6) | 21 (21) | 1 (1) | |
Pseudomonas corrugata | 2 (2) | 7 (7) | ||
Pseudomonas fluorescens | 13 (5) | 17 (4) | 1 (0) | |
Pseudomonas jessenii | 1 (0) | |||
Pseudomonas marginalis | 2 (2) | |||
Pseudomonas migulae | 2 (0) | |||
Pseudomonas orientalis | 1 (0) | |||
Pseudomonas putida | 12 (12) | 9 (9) | 48 (46) | |
Pseudomonas reactans | 1 (0) | 2 (0) | ||
Pseudomonas savastanoi pv. fraxinus | 2 (2) | 6 (6) | ||
Pseudomonas straminea | 1 (0) | |||
Pseudomonas syringae | 4 (4) | |||
Rhodococcus erythropolis | 1 (1) | |||
Serratia plymuthica | 1 (0) | |||
Shingobacterium spiritovorum | 1 (1) | |||
Staphylococcus epidermidis | 1 (0) | 1 (0) | ||
Staphylococcus pasteuri | 1 (0) | 1 (0) | ||
Streptomyces halstedii | 1 (1) | |||
Variovorax paradoxus | 2 (0) | 1 (1) | ||
Total no. of species | 25 | 14 | 16 | 10 |
Total no of isolates | 64 | 134 | 101 | 17 |
Richness | 3.1 | 1.2 | 1.6 | 2.4 |
Diversity | 2.7 | 1.7 | 1.9 | 2.0 |
aIdentification by FAME analysis of isolates identified with a similarity index of >0.5 and 16S rDNA sequencing with a similarity index of >98% (number in brackets).
List of bacterial antagonistic species isolated from different microenvironments of potato
Speciesa/microenvironment | Rhizosphere | Phyllosphere | Endorhiza | Endosphere |
Agrobacterium tumefaciens | 1 (0) | 1 (0) | 1 (0) | |
Arthrobacter globiformis | 2 (1) | |||
Arthrobacter ilicis | 1 (1) | |||
Arthobacter oxydans | 1 (1) | |||
Bacillus amyloliquefaciens | 1 (1) | 3 (3) | ||
Bacillus aquamarinus | 1(0) | |||
Bacillus cereus | 3 (3) | |||
Bacillus licheniformis | 1 (1) | 1 (1) | ||
Bacillus mycoides | 1 (1) | |||
Bacillus pumilus | 4 (4) | 56 (54) | 2 (2) | 1 (0) |
Bacillus subtilis | 2 (0) | 32 (28) | 6 (2) | 5 (1) |
Bacillus thuringiensis | 1 (0) | |||
Curtobacterium flaccumfaciens | 4 (3) | |||
Enterobacter taylorae | 1 (1) | |||
Erwinia amylovora | 1 (1) | |||
Erwinia persicinus | 4 (0) | 1 (0) | 1 (0) | |
Erwinia rhapontici | 1 (0) | |||
Lysobacter antibioticus | 1 (0) | |||
Micrococcus kristinae | 1 (1) | |||
Paenibacillus peoriae | 1 (0) | |||
Paenibacillus polymyxa | 2 (2) | 1 (1) | ||
Pseudomonas chlororaphis | 6 (6) | 21 (21) | 1 (1) | |
Pseudomonas corrugata | 2 (2) | 7 (7) | ||
Pseudomonas fluorescens | 13 (5) | 17 (4) | 1 (0) | |
Pseudomonas jessenii | 1 (0) | |||
Pseudomonas marginalis | 2 (2) | |||
Pseudomonas migulae | 2 (0) | |||
Pseudomonas orientalis | 1 (0) | |||
Pseudomonas putida | 12 (12) | 9 (9) | 48 (46) | |
Pseudomonas reactans | 1 (0) | 2 (0) | ||
Pseudomonas savastanoi pv. fraxinus | 2 (2) | 6 (6) | ||
Pseudomonas straminea | 1 (0) | |||
Pseudomonas syringae | 4 (4) | |||
Rhodococcus erythropolis | 1 (1) | |||
Serratia plymuthica | 1 (0) | |||
Shingobacterium spiritovorum | 1 (1) | |||
Staphylococcus epidermidis | 1 (0) | 1 (0) | ||
Staphylococcus pasteuri | 1 (0) | 1 (0) | ||
Streptomyces halstedii | 1 (1) | |||
Variovorax paradoxus | 2 (0) | 1 (1) | ||
Total no. of species | 25 | 14 | 16 | 10 |
Total no of isolates | 64 | 134 | 101 | 17 |
Richness | 3.1 | 1.2 | 1.6 | 2.4 |
Diversity | 2.7 | 1.7 | 1.9 | 2.0 |
Speciesa/microenvironment | Rhizosphere | Phyllosphere | Endorhiza | Endosphere |
Agrobacterium tumefaciens | 1 (0) | 1 (0) | 1 (0) | |
Arthrobacter globiformis | 2 (1) | |||
Arthrobacter ilicis | 1 (1) | |||
Arthobacter oxydans | 1 (1) | |||
Bacillus amyloliquefaciens | 1 (1) | 3 (3) | ||
Bacillus aquamarinus | 1(0) | |||
Bacillus cereus | 3 (3) | |||
Bacillus licheniformis | 1 (1) | 1 (1) | ||
Bacillus mycoides | 1 (1) | |||
Bacillus pumilus | 4 (4) | 56 (54) | 2 (2) | 1 (0) |
Bacillus subtilis | 2 (0) | 32 (28) | 6 (2) | 5 (1) |
Bacillus thuringiensis | 1 (0) | |||
Curtobacterium flaccumfaciens | 4 (3) | |||
Enterobacter taylorae | 1 (1) | |||
Erwinia amylovora | 1 (1) | |||
Erwinia persicinus | 4 (0) | 1 (0) | 1 (0) | |
Erwinia rhapontici | 1 (0) | |||
Lysobacter antibioticus | 1 (0) | |||
Micrococcus kristinae | 1 (1) | |||
Paenibacillus peoriae | 1 (0) | |||
Paenibacillus polymyxa | 2 (2) | 1 (1) | ||
Pseudomonas chlororaphis | 6 (6) | 21 (21) | 1 (1) | |
Pseudomonas corrugata | 2 (2) | 7 (7) | ||
Pseudomonas fluorescens | 13 (5) | 17 (4) | 1 (0) | |
Pseudomonas jessenii | 1 (0) | |||
Pseudomonas marginalis | 2 (2) | |||
Pseudomonas migulae | 2 (0) | |||
Pseudomonas orientalis | 1 (0) | |||
Pseudomonas putida | 12 (12) | 9 (9) | 48 (46) | |
Pseudomonas reactans | 1 (0) | 2 (0) | ||
Pseudomonas savastanoi pv. fraxinus | 2 (2) | 6 (6) | ||
Pseudomonas straminea | 1 (0) | |||
Pseudomonas syringae | 4 (4) | |||
Rhodococcus erythropolis | 1 (1) | |||
Serratia plymuthica | 1 (0) | |||
Shingobacterium spiritovorum | 1 (1) | |||
Staphylococcus epidermidis | 1 (0) | 1 (0) | ||
Staphylococcus pasteuri | 1 (0) | 1 (0) | ||
Streptomyces halstedii | 1 (1) | |||
Variovorax paradoxus | 2 (0) | 1 (1) | ||
Total no. of species | 25 | 14 | 16 | 10 |
Total no of isolates | 64 | 134 | 101 | 17 |
Richness | 3.1 | 1.2 | 1.6 | 2.4 |
Diversity | 2.7 | 1.7 | 1.9 | 2.0 |
aIdentification by FAME analysis of isolates identified with a similarity index of >0.5 and 16S rDNA sequencing with a similarity index of >98% (number in brackets).
The most frequently isolated bacteria were P. putida (69 isolates), B. pumilus (63 isolates), and B. subtilis (45 isolates). With regard to microenvironment, the most commonly isolated species in the rhizosphere and endosphere were P. putida and P. fluorescens, in the phyllosphere it was B. pumilus, and B. subtilis in the endosphere. A high number of species (26 species = 57%) was found exclusively in one microenvironment, i.e., in the rhizosphere.
Differences between microenvironments were also expressed by species composition. The rhizosphere and endorhiza were dominated by Pseudomonas species whereas in the phyllosphere, many gram-positive bacteria were found. Bacterial species identified in all microenvironments belonged to seven phylogenetical groups: α-, β-, and γ-proteobacteria, Actinobacteria, Enterobacteria, Firmicutes and Sphingobacteria (Fig. 2). The rhizosphere and endorhiza were dominated by γ-protebacteria and the phyllosphere and endosphere by Firmicutes, respectively. β-Proteobacteria were found exclusively in the rhizosphere and endorhiza.

Composition of phylogenetic groups of antagonistic bacteria in different microenvironments of potatoes.
3.5 Characterization of P. putida isolates
Pseudomonas putida was the most dominant antagonistic species found at all sampling times, and in all microenvironments, except in the endosphere. In addition, it is a well known plant-associated antagonistic species. All 68 P. putida isolates obtained during this study were therefore phenotypically and genotypically characterized. Genotypic characterization was performed with BOX-PCR fingerprinting of genomic DNA. Analysis of the BOX-PCR fingerprints using UPGMA and GelCompar showed a high genotypic diversity. At a cutoff level of 90% they could be divided into 26 genotypic groups (G1-G26), 14 of them represented a single isolate (Table 4). The majority of isolates (52) could be clustered into 12 groups, which contained between two and 13 isolates. Eleven of these 12 groups (91%) comprised only isolates from one microenvironment. Seven groups (G1, G2, G5, G9, G11, G12, G23) were formed by isolates from the endorhiza while two groups were formed by isolates from the rhizosphere (G4, G8) and phyllosphere (G18, G19). Only group G3 contained P. putida isolates from two microenvironments, the rhizosphere and endorhiza, as well as from two growth stages flowering and senescent plants. BOX fingerprints of all 13 P. putida isolates belonging to group G3 were nearly identical and were 98% similar to each other.
Antagonistic and plant growth promoting mechanisms of Pseudomonas putida isolates in vitro
Groupa | Strain | Origin | Antagonistic activity towardc | Lytic enzymesd | Metabolites | |||||
Habitat | Stageb | V.d. | R.s. | Cellulases | Glucanases | Proteases | DAPGe | IAAf | ||
G1 | 2Re4–1 | Endorhiza | f | ++ | + | – | – | + | + | + |
2Re4–4 | Endorhiza | f | ++ | + | – | – | + | + | + | |
G2 | 2Re4–9 | Endorhiza | f | ++ | + | – | – | + | + | – |
2Re4-10 | Endorhiza | f | ++ | – | – | – | + | + | + | |
2Re4-11 | Endorhiza | f | ++ | + | – | – | + | + | + | |
2Re4–6 | Endorhiza | f | ++ | + | – | – | + | + | + | |
2Re4–7 | Endorhiza | f | ++ | + | – | – | + | + | – | |
G3 | 5Re4-27 | Endorhiza | f | ++ | – | – | – | + | + | – |
5R2–4 | Rhizosphere | f | +++ | + | – | – | + | + | + | |
5Re2-21 | Endorhiza | f | ++ | + | – | – | + | + | – | |
5Re4–2 | Endorhiza | f | ++ | + | – | – | + | + | – | |
5R2-27 | Rhizosphere | f | +++ | ++ | – | – | + | + | – | |
5Re4-19 | Endorhiza | f | ++ | + | – | – | + | + | + | |
5Re2-27 | Endorhiza | f | +++ | + | – | – | + | + | – | |
5Re4–3 | Endorhiza | f | ++ | + | – | – | + | + | – | |
5Re1-25 | Endorhiza | f | +++ | ++ | – | – | + | + | – | |
6Re2–8 | Endorhiza | s | + | – | – | – | + | + | + | |
6Re2-20 | Endorhiza | s | +++ | – | – | – | + | + | – | |
6Re3–9 | Endorhiza | s | +++ | – | – | – | + | + | – | |
6Re4-27 | Endorhiza | s | +++ | – | – | – | + | + | – | |
G4 | 6R5-19 | Rhizosphere | s | +++ | – | – | – | + | + | – |
6R5–4 | Rhizosphere | s | +++ | – | – | – | + | + | + | |
6R5–2 | Rhizosphere | s | +++ | – | – | – | + | + | – | |
6R5-24 | Rhizosphere | s | +++ | ++ | – | – | + | + | + | |
G5 | 6Re2-14 | Endorhiza | s | ++ | – | – | – | + | + | + |
6Re2-16 | Endorhiza | s | ++ | – | – | – | + | + | + | |
6Re2–5 | Endorhiza | s | ++ | – | – | – | + | + | + | |
G6 | 6Re2-22 | Endorhiza | s | +++ | + | – | – | + | + | + |
G7 | 4R2-10 | Rhizosphere | y | – | + | – | – | + | – | – |
G8 | 3R1-19 | Rhizosphere | s | +++ | ++ | – | – | + | + | + |
3R2-12 | Rhizosphere | s | +++ | + | – | – | + | + | + | |
G9 | 2Re4-27 | Endorhiza | f | ++ | – | – | – | + | + | – |
2Re4-32 | Endorhiza | f | ++ | + | – | – | + | + | + | |
2Re4-30 | Endorhiza | f | – | + | – | – | + | + | + | |
2Re4-23 | Endorhiza | f | ++ | + | – | – | + | + | + | |
2Re4-24 | Endorhiza | f | ++ | + | – | – | + | + | – | |
2Re4-26 | Endorhiza | f | + | + | – | – | + | + | + | |
G10 | 2Re4-28 | Endorhiza | f | ++ | + | – | – | + | + | – |
G11 | 2Re4-31 | Endorhiza | f | + | + | – | – | + | + | + |
2Re4-33 | Endorhiza | f | + | + | – | – | + | + | – | |
2Re4-35 | Endorhiza | f | + | + | – | – | + | + | + | |
2Re4-36 | Endorhiza | f | + | + | – | – | + | + | – | |
G12 | 2Re4-16 | Endorhiza | f | + | + | – | – | + | + | – |
2Re4-18 | Endorhiza | f | + | + | – | – | + | + | – | |
2Re4-19 | Endorhiza | f | + | + | – | – | + | + | – | |
G13 | 6Re2–4 | Endorhiza | s | +++ | – | – | – | + | + | + |
G14 | 6R2–2 | Rhizosphere | s | – | ++ | – | – | + | + | + |
G15 | 3R4-17 | Rhizosphere | s | ++ | – | – | – | – | + | – |
G16 | 3Re4–3 | Rhizosphere | s | + | – | + | – | – | + | – |
G17 | 3Re4-21 | Endorhiza | s | +++ | ++ | + | – | + | + | + |
G18 | 5P2-29 | Phyllosphere | f | – | +++ | – | – | + | – | – |
5P2-15 | Phyllosphere | f | – | ++ | – | – | + | – | + | |
5P2-24 | Phyllosphere | f | – | ++ | – | – | + | – | – | |
5P2-20 | Phyllosphere | f | – | ++ | – | – | + | – | + | |
5P2-21 | Phyllosphere | f | – | ++ | – | – | + | – | + | |
G19 | 5P2-16 | Phyllosphere | f | – | ++ | – | – | + | – | – |
5P2-22 | Phyllosphere | f | – | ++ | – | – | + | – | – | |
G20 | 5P2-12 | Phyllosphere | f | – | ++ | – | – | + | – | – |
G21 | 5P2-28 | Phyllosphere | f | – | +++ | – | – | + | – | + |
G22 | 5Re5-12 | Endorhiza | f | + | + | – | – | + | – | – |
G23 | 5Re2–6 | Endorhiza | f | +++ | ++ | – | – | + | – | – |
5Re4-23 | Endorhiza | f | +++ | + | – | – | + | + | + | |
5Re2-10 | Endorhiza | f | +++ | ++ | – | – | + | + | – | |
5Re2-17 | Endorhiza | f | +++ | ++ | – | – | + | + | – | |
5Re2-28 | Endorhiza | f | +++ | ++ | – | – | + | + | + | |
G24 | 5Re4-16 | Endorhiza | f | – | + | – | – | + | + | – |
G25 | 6Re4–2 | Endorhiza | s | +++ | – | – | +++ | + | + | + |
G26 | 2Re2-31 | Endorhiza | f | – | + | + | +++ | + | – | – |
Groupa | Strain | Origin | Antagonistic activity towardc | Lytic enzymesd | Metabolites | |||||
Habitat | Stageb | V.d. | R.s. | Cellulases | Glucanases | Proteases | DAPGe | IAAf | ||
G1 | 2Re4–1 | Endorhiza | f | ++ | + | – | – | + | + | + |
2Re4–4 | Endorhiza | f | ++ | + | – | – | + | + | + | |
G2 | 2Re4–9 | Endorhiza | f | ++ | + | – | – | + | + | – |
2Re4-10 | Endorhiza | f | ++ | – | – | – | + | + | + | |
2Re4-11 | Endorhiza | f | ++ | + | – | – | + | + | + | |
2Re4–6 | Endorhiza | f | ++ | + | – | – | + | + | + | |
2Re4–7 | Endorhiza | f | ++ | + | – | – | + | + | – | |
G3 | 5Re4-27 | Endorhiza | f | ++ | – | – | – | + | + | – |
5R2–4 | Rhizosphere | f | +++ | + | – | – | + | + | + | |
5Re2-21 | Endorhiza | f | ++ | + | – | – | + | + | – | |
5Re4–2 | Endorhiza | f | ++ | + | – | – | + | + | – | |
5R2-27 | Rhizosphere | f | +++ | ++ | – | – | + | + | – | |
5Re4-19 | Endorhiza | f | ++ | + | – | – | + | + | + | |
5Re2-27 | Endorhiza | f | +++ | + | – | – | + | + | – | |
5Re4–3 | Endorhiza | f | ++ | + | – | – | + | + | – | |
5Re1-25 | Endorhiza | f | +++ | ++ | – | – | + | + | – | |
6Re2–8 | Endorhiza | s | + | – | – | – | + | + | + | |
6Re2-20 | Endorhiza | s | +++ | – | – | – | + | + | – | |
6Re3–9 | Endorhiza | s | +++ | – | – | – | + | + | – | |
6Re4-27 | Endorhiza | s | +++ | – | – | – | + | + | – | |
G4 | 6R5-19 | Rhizosphere | s | +++ | – | – | – | + | + | – |
6R5–4 | Rhizosphere | s | +++ | – | – | – | + | + | + | |
6R5–2 | Rhizosphere | s | +++ | – | – | – | + | + | – | |
6R5-24 | Rhizosphere | s | +++ | ++ | – | – | + | + | + | |
G5 | 6Re2-14 | Endorhiza | s | ++ | – | – | – | + | + | + |
6Re2-16 | Endorhiza | s | ++ | – | – | – | + | + | + | |
6Re2–5 | Endorhiza | s | ++ | – | – | – | + | + | + | |
G6 | 6Re2-22 | Endorhiza | s | +++ | + | – | – | + | + | + |
G7 | 4R2-10 | Rhizosphere | y | – | + | – | – | + | – | – |
G8 | 3R1-19 | Rhizosphere | s | +++ | ++ | – | – | + | + | + |
3R2-12 | Rhizosphere | s | +++ | + | – | – | + | + | + | |
G9 | 2Re4-27 | Endorhiza | f | ++ | – | – | – | + | + | – |
2Re4-32 | Endorhiza | f | ++ | + | – | – | + | + | + | |
2Re4-30 | Endorhiza | f | – | + | – | – | + | + | + | |
2Re4-23 | Endorhiza | f | ++ | + | – | – | + | + | + | |
2Re4-24 | Endorhiza | f | ++ | + | – | – | + | + | – | |
2Re4-26 | Endorhiza | f | + | + | – | – | + | + | + | |
G10 | 2Re4-28 | Endorhiza | f | ++ | + | – | – | + | + | – |
G11 | 2Re4-31 | Endorhiza | f | + | + | – | – | + | + | + |
2Re4-33 | Endorhiza | f | + | + | – | – | + | + | – | |
2Re4-35 | Endorhiza | f | + | + | – | – | + | + | + | |
2Re4-36 | Endorhiza | f | + | + | – | – | + | + | – | |
G12 | 2Re4-16 | Endorhiza | f | + | + | – | – | + | + | – |
2Re4-18 | Endorhiza | f | + | + | – | – | + | + | – | |
2Re4-19 | Endorhiza | f | + | + | – | – | + | + | – | |
G13 | 6Re2–4 | Endorhiza | s | +++ | – | – | – | + | + | + |
G14 | 6R2–2 | Rhizosphere | s | – | ++ | – | – | + | + | + |
G15 | 3R4-17 | Rhizosphere | s | ++ | – | – | – | – | + | – |
G16 | 3Re4–3 | Rhizosphere | s | + | – | + | – | – | + | – |
G17 | 3Re4-21 | Endorhiza | s | +++ | ++ | + | – | + | + | + |
G18 | 5P2-29 | Phyllosphere | f | – | +++ | – | – | + | – | – |
5P2-15 | Phyllosphere | f | – | ++ | – | – | + | – | + | |
5P2-24 | Phyllosphere | f | – | ++ | – | – | + | – | – | |
5P2-20 | Phyllosphere | f | – | ++ | – | – | + | – | + | |
5P2-21 | Phyllosphere | f | – | ++ | – | – | + | – | + | |
G19 | 5P2-16 | Phyllosphere | f | – | ++ | – | – | + | – | – |
5P2-22 | Phyllosphere | f | – | ++ | – | – | + | – | – | |
G20 | 5P2-12 | Phyllosphere | f | – | ++ | – | – | + | – | – |
G21 | 5P2-28 | Phyllosphere | f | – | +++ | – | – | + | – | + |
G22 | 5Re5-12 | Endorhiza | f | + | + | – | – | + | – | – |
G23 | 5Re2–6 | Endorhiza | f | +++ | ++ | – | – | + | – | – |
5Re4-23 | Endorhiza | f | +++ | + | – | – | + | + | + | |
5Re2-10 | Endorhiza | f | +++ | ++ | – | – | + | + | – | |
5Re2-17 | Endorhiza | f | +++ | ++ | – | – | + | + | – | |
5Re2-28 | Endorhiza | f | +++ | ++ | – | – | + | + | + | |
G24 | 5Re4-16 | Endorhiza | f | – | + | – | – | + | + | – |
G25 | 6Re4–2 | Endorhiza | s | +++ | – | – | +++ | + | + | + |
G26 | 2Re2-31 | Endorhiza | f | – | + | + | +++ | + | – | – |
aGrouping according to BOX-PCR fingerprinting and clustering at a similarity level of 90%.
by, Young plants; f, flowering plants; s, senescent plants.
cAntagonism toward V.d.=Verticillium dahliae, R.s.=Rhizoctonia solani, dual culture assay: + represents 0–5 mm wide zone, ++ represents 5–10 mm wide zone, +++ represents >10 mm wide zone of inhibition.
d+ represents production on plate assay.
eDetection of the phl gene by PCR.
fIAA = indole-3-acetic acid production in vitro.
Antagonistic and plant growth promoting mechanisms of Pseudomonas putida isolates in vitro
Groupa | Strain | Origin | Antagonistic activity towardc | Lytic enzymesd | Metabolites | |||||
Habitat | Stageb | V.d. | R.s. | Cellulases | Glucanases | Proteases | DAPGe | IAAf | ||
G1 | 2Re4–1 | Endorhiza | f | ++ | + | – | – | + | + | + |
2Re4–4 | Endorhiza | f | ++ | + | – | – | + | + | + | |
G2 | 2Re4–9 | Endorhiza | f | ++ | + | – | – | + | + | – |
2Re4-10 | Endorhiza | f | ++ | – | – | – | + | + | + | |
2Re4-11 | Endorhiza | f | ++ | + | – | – | + | + | + | |
2Re4–6 | Endorhiza | f | ++ | + | – | – | + | + | + | |
2Re4–7 | Endorhiza | f | ++ | + | – | – | + | + | – | |
G3 | 5Re4-27 | Endorhiza | f | ++ | – | – | – | + | + | – |
5R2–4 | Rhizosphere | f | +++ | + | – | – | + | + | + | |
5Re2-21 | Endorhiza | f | ++ | + | – | – | + | + | – | |
5Re4–2 | Endorhiza | f | ++ | + | – | – | + | + | – | |
5R2-27 | Rhizosphere | f | +++ | ++ | – | – | + | + | – | |
5Re4-19 | Endorhiza | f | ++ | + | – | – | + | + | + | |
5Re2-27 | Endorhiza | f | +++ | + | – | – | + | + | – | |
5Re4–3 | Endorhiza | f | ++ | + | – | – | + | + | – | |
5Re1-25 | Endorhiza | f | +++ | ++ | – | – | + | + | – | |
6Re2–8 | Endorhiza | s | + | – | – | – | + | + | + | |
6Re2-20 | Endorhiza | s | +++ | – | – | – | + | + | – | |
6Re3–9 | Endorhiza | s | +++ | – | – | – | + | + | – | |
6Re4-27 | Endorhiza | s | +++ | – | – | – | + | + | – | |
G4 | 6R5-19 | Rhizosphere | s | +++ | – | – | – | + | + | – |
6R5–4 | Rhizosphere | s | +++ | – | – | – | + | + | + | |
6R5–2 | Rhizosphere | s | +++ | – | – | – | + | + | – | |
6R5-24 | Rhizosphere | s | +++ | ++ | – | – | + | + | + | |
G5 | 6Re2-14 | Endorhiza | s | ++ | – | – | – | + | + | + |
6Re2-16 | Endorhiza | s | ++ | – | – | – | + | + | + | |
6Re2–5 | Endorhiza | s | ++ | – | – | – | + | + | + | |
G6 | 6Re2-22 | Endorhiza | s | +++ | + | – | – | + | + | + |
G7 | 4R2-10 | Rhizosphere | y | – | + | – | – | + | – | – |
G8 | 3R1-19 | Rhizosphere | s | +++ | ++ | – | – | + | + | + |
3R2-12 | Rhizosphere | s | +++ | + | – | – | + | + | + | |
G9 | 2Re4-27 | Endorhiza | f | ++ | – | – | – | + | + | – |
2Re4-32 | Endorhiza | f | ++ | + | – | – | + | + | + | |
2Re4-30 | Endorhiza | f | – | + | – | – | + | + | + | |
2Re4-23 | Endorhiza | f | ++ | + | – | – | + | + | + | |
2Re4-24 | Endorhiza | f | ++ | + | – | – | + | + | – | |
2Re4-26 | Endorhiza | f | + | + | – | – | + | + | + | |
G10 | 2Re4-28 | Endorhiza | f | ++ | + | – | – | + | + | – |
G11 | 2Re4-31 | Endorhiza | f | + | + | – | – | + | + | + |
2Re4-33 | Endorhiza | f | + | + | – | – | + | + | – | |
2Re4-35 | Endorhiza | f | + | + | – | – | + | + | + | |
2Re4-36 | Endorhiza | f | + | + | – | – | + | + | – | |
G12 | 2Re4-16 | Endorhiza | f | + | + | – | – | + | + | – |
2Re4-18 | Endorhiza | f | + | + | – | – | + | + | – | |
2Re4-19 | Endorhiza | f | + | + | – | – | + | + | – | |
G13 | 6Re2–4 | Endorhiza | s | +++ | – | – | – | + | + | + |
G14 | 6R2–2 | Rhizosphere | s | – | ++ | – | – | + | + | + |
G15 | 3R4-17 | Rhizosphere | s | ++ | – | – | – | – | + | – |
G16 | 3Re4–3 | Rhizosphere | s | + | – | + | – | – | + | – |
G17 | 3Re4-21 | Endorhiza | s | +++ | ++ | + | – | + | + | + |
G18 | 5P2-29 | Phyllosphere | f | – | +++ | – | – | + | – | – |
5P2-15 | Phyllosphere | f | – | ++ | – | – | + | – | + | |
5P2-24 | Phyllosphere | f | – | ++ | – | – | + | – | – | |
5P2-20 | Phyllosphere | f | – | ++ | – | – | + | – | + | |
5P2-21 | Phyllosphere | f | – | ++ | – | – | + | – | + | |
G19 | 5P2-16 | Phyllosphere | f | – | ++ | – | – | + | – | – |
5P2-22 | Phyllosphere | f | – | ++ | – | – | + | – | – | |
G20 | 5P2-12 | Phyllosphere | f | – | ++ | – | – | + | – | – |
G21 | 5P2-28 | Phyllosphere | f | – | +++ | – | – | + | – | + |
G22 | 5Re5-12 | Endorhiza | f | + | + | – | – | + | – | – |
G23 | 5Re2–6 | Endorhiza | f | +++ | ++ | – | – | + | – | – |
5Re4-23 | Endorhiza | f | +++ | + | – | – | + | + | + | |
5Re2-10 | Endorhiza | f | +++ | ++ | – | – | + | + | – | |
5Re2-17 | Endorhiza | f | +++ | ++ | – | – | + | + | – | |
5Re2-28 | Endorhiza | f | +++ | ++ | – | – | + | + | + | |
G24 | 5Re4-16 | Endorhiza | f | – | + | – | – | + | + | – |
G25 | 6Re4–2 | Endorhiza | s | +++ | – | – | +++ | + | + | + |
G26 | 2Re2-31 | Endorhiza | f | – | + | + | +++ | + | – | – |
Groupa | Strain | Origin | Antagonistic activity towardc | Lytic enzymesd | Metabolites | |||||
Habitat | Stageb | V.d. | R.s. | Cellulases | Glucanases | Proteases | DAPGe | IAAf | ||
G1 | 2Re4–1 | Endorhiza | f | ++ | + | – | – | + | + | + |
2Re4–4 | Endorhiza | f | ++ | + | – | – | + | + | + | |
G2 | 2Re4–9 | Endorhiza | f | ++ | + | – | – | + | + | – |
2Re4-10 | Endorhiza | f | ++ | – | – | – | + | + | + | |
2Re4-11 | Endorhiza | f | ++ | + | – | – | + | + | + | |
2Re4–6 | Endorhiza | f | ++ | + | – | – | + | + | + | |
2Re4–7 | Endorhiza | f | ++ | + | – | – | + | + | – | |
G3 | 5Re4-27 | Endorhiza | f | ++ | – | – | – | + | + | – |
5R2–4 | Rhizosphere | f | +++ | + | – | – | + | + | + | |
5Re2-21 | Endorhiza | f | ++ | + | – | – | + | + | – | |
5Re4–2 | Endorhiza | f | ++ | + | – | – | + | + | – | |
5R2-27 | Rhizosphere | f | +++ | ++ | – | – | + | + | – | |
5Re4-19 | Endorhiza | f | ++ | + | – | – | + | + | + | |
5Re2-27 | Endorhiza | f | +++ | + | – | – | + | + | – | |
5Re4–3 | Endorhiza | f | ++ | + | – | – | + | + | – | |
5Re1-25 | Endorhiza | f | +++ | ++ | – | – | + | + | – | |
6Re2–8 | Endorhiza | s | + | – | – | – | + | + | + | |
6Re2-20 | Endorhiza | s | +++ | – | – | – | + | + | – | |
6Re3–9 | Endorhiza | s | +++ | – | – | – | + | + | – | |
6Re4-27 | Endorhiza | s | +++ | – | – | – | + | + | – | |
G4 | 6R5-19 | Rhizosphere | s | +++ | – | – | – | + | + | – |
6R5–4 | Rhizosphere | s | +++ | – | – | – | + | + | + | |
6R5–2 | Rhizosphere | s | +++ | – | – | – | + | + | – | |
6R5-24 | Rhizosphere | s | +++ | ++ | – | – | + | + | + | |
G5 | 6Re2-14 | Endorhiza | s | ++ | – | – | – | + | + | + |
6Re2-16 | Endorhiza | s | ++ | – | – | – | + | + | + | |
6Re2–5 | Endorhiza | s | ++ | – | – | – | + | + | + | |
G6 | 6Re2-22 | Endorhiza | s | +++ | + | – | – | + | + | + |
G7 | 4R2-10 | Rhizosphere | y | – | + | – | – | + | – | – |
G8 | 3R1-19 | Rhizosphere | s | +++ | ++ | – | – | + | + | + |
3R2-12 | Rhizosphere | s | +++ | + | – | – | + | + | + | |
G9 | 2Re4-27 | Endorhiza | f | ++ | – | – | – | + | + | – |
2Re4-32 | Endorhiza | f | ++ | + | – | – | + | + | + | |
2Re4-30 | Endorhiza | f | – | + | – | – | + | + | + | |
2Re4-23 | Endorhiza | f | ++ | + | – | – | + | + | + | |
2Re4-24 | Endorhiza | f | ++ | + | – | – | + | + | – | |
2Re4-26 | Endorhiza | f | + | + | – | – | + | + | + | |
G10 | 2Re4-28 | Endorhiza | f | ++ | + | – | – | + | + | – |
G11 | 2Re4-31 | Endorhiza | f | + | + | – | – | + | + | + |
2Re4-33 | Endorhiza | f | + | + | – | – | + | + | – | |
2Re4-35 | Endorhiza | f | + | + | – | – | + | + | + | |
2Re4-36 | Endorhiza | f | + | + | – | – | + | + | – | |
G12 | 2Re4-16 | Endorhiza | f | + | + | – | – | + | + | – |
2Re4-18 | Endorhiza | f | + | + | – | – | + | + | – | |
2Re4-19 | Endorhiza | f | + | + | – | – | + | + | – | |
G13 | 6Re2–4 | Endorhiza | s | +++ | – | – | – | + | + | + |
G14 | 6R2–2 | Rhizosphere | s | – | ++ | – | – | + | + | + |
G15 | 3R4-17 | Rhizosphere | s | ++ | – | – | – | – | + | – |
G16 | 3Re4–3 | Rhizosphere | s | + | – | + | – | – | + | – |
G17 | 3Re4-21 | Endorhiza | s | +++ | ++ | + | – | + | + | + |
G18 | 5P2-29 | Phyllosphere | f | – | +++ | – | – | + | – | – |
5P2-15 | Phyllosphere | f | – | ++ | – | – | + | – | + | |
5P2-24 | Phyllosphere | f | – | ++ | – | – | + | – | – | |
5P2-20 | Phyllosphere | f | – | ++ | – | – | + | – | + | |
5P2-21 | Phyllosphere | f | – | ++ | – | – | + | – | + | |
G19 | 5P2-16 | Phyllosphere | f | – | ++ | – | – | + | – | – |
5P2-22 | Phyllosphere | f | – | ++ | – | – | + | – | – | |
G20 | 5P2-12 | Phyllosphere | f | – | ++ | – | – | + | – | – |
G21 | 5P2-28 | Phyllosphere | f | – | +++ | – | – | + | – | + |
G22 | 5Re5-12 | Endorhiza | f | + | + | – | – | + | – | – |
G23 | 5Re2–6 | Endorhiza | f | +++ | ++ | – | – | + | – | – |
5Re4-23 | Endorhiza | f | +++ | + | – | – | + | + | + | |
5Re2-10 | Endorhiza | f | +++ | ++ | – | – | + | + | – | |
5Re2-17 | Endorhiza | f | +++ | ++ | – | – | + | + | – | |
5Re2-28 | Endorhiza | f | +++ | ++ | – | – | + | + | + | |
G24 | 5Re4-16 | Endorhiza | f | – | + | – | – | + | + | – |
G25 | 6Re4–2 | Endorhiza | s | +++ | – | – | +++ | + | + | + |
G26 | 2Re2-31 | Endorhiza | f | – | + | + | +++ | + | – | – |
aGrouping according to BOX-PCR fingerprinting and clustering at a similarity level of 90%.
by, Young plants; f, flowering plants; s, senescent plants.
cAntagonism toward V.d.=Verticillium dahliae, R.s.=Rhizoctonia solani, dual culture assay: + represents 0–5 mm wide zone, ++ represents 5–10 mm wide zone, +++ represents >10 mm wide zone of inhibition.
d+ represents production on plate assay.
eDetection of the phl gene by PCR.
fIAA = indole-3-acetic acid production in vitro.
In addition, the 68 antagonistic P. putida isolates were characterized based on their production of hydrolytic enzymes and secondary metabolites (Table 4). In general, P. putida isolates from all microenvironments showed higher antifungal activity against V. dahliae (54 isolates) than against R. solani (51 isolates). None of the isolates produced chitinases or pectinases (data not shown). Protease production was shown for all isolates except two from the rhizosphere belonging to G15 and G16. Siderophores were produced by all isolates (data not shown). Using PCR, presence of the phl D gene encoding for the antifungal antibiotic 2,4-diacetyl-phloroglucinol (DAPG) was confirmed for Pseudomonas isolates from the rhizosphere and endorhiza, but not for isolates from the phyllosphere. As indicator for plant growth promotion, production of indole-3-acetic acid was studied in vitro and found to be expressed by nearly half of isolates.
3.6 Assessing the best candidates for biological control
An assessment scheme was developed and employed to all 346 antagonistic isolates in order to better select bacterial isolates with high antagonistic potential. The following points were given for each of the bacterial traits: up to three points for antagonistic activity to V. dahliae and R. solani and one point each for production of potential fungal cell wall degrading enzymes (chitinases, cellulases, glucanases, pectinases and proteinases), siderophores, and auxin. A total number of 13 points was possible. The five best isolates of each microenvironment received between 7 (endosphere) and 12 points (endorhiza) (Table 5). All 20 isolates showed antagonistic activity to R. solani and 17 isolates to V. dahliae. Eighteen out of these 20 antagonistic isolates expressed proteolytic activity and 17 isolates expressed cellulytic activity and siderophore production, whereas only three isolates showed chitinolytic activity. The highest score, that is 12, was achieved by Serratia plymuthica 3Re4-18 originally isolated from the endorhiza.
Assessment of potato-associated strains for biological control and list of the most promising candidates from each microenvironment
Origin/micro-environment | Strain | Identificationa | Antagonistic activity towardb | Production of: | Assessment points | |||||||
V.d. | R.s. | Hydrolytic enzymec | Metabolite | |||||||||
Cellulases | Chitinases | Glucanases | Pectinases | Proteases | Siderophoresd | IAAe | ||||||
Rhizosphere | 1R1-22 | Micrococcus kristinae* | 3 | 3 | 1 | – | – | – | 1 | 1 | – | 9 |
2R2-11 | Arthrobacter oxydans* | 3 | 3 | 1 | – | 1 | – | 1 | 1 | – | 10 | |
2R2-15 | Bacillus licheniformis | 2 | 2 | 1 | – | 1 | – | 1 | 1 | – | 8 | |
5R3-11 | Streptomyces rochei* | 3 | 3 | 1 | – | 1 | – | – | – | – | 8 | |
6R4-28 | Pseudomonas chlororaphis* | 2 | 3 | – | – | – | – | 1 | 1 | 1 | 8 | |
Phyllosphere | 1P1–2 | Paenibacillus peoriae | 2 | 3 | 1 | – | 1 | 1 | 1 | 1 | – | 11 |
2P3-18 | Staphylococcus epidermidis | – | 2 | 1 | 1 | 1 | 1 | 1 | 1 | – | 8 | |
2P3-25 | Arthrobacter sulfonivorans | – | 2 | 1 | 1 | 1 | 1 | 1 | 1 | 1 | 9 | |
4P2–4 | Bacillus amyloliquefaciens* | 2 | 2 | 1 | – | 1 | 1 | 1 | 1 | – | 9 | |
5P1–8 | n.d. | 3 | 3 | 1 | – | 1 | 1 | 1 | – | – | 10 | |
Endorhiza | 3Re2–7 | Pseudomonas reactans | 3 | 3 | – | – | – | – | 1 | 1 | 1 | 8 |
3Re2-26 | Pseudomonas fluorescens | 3 | 2 | – | – | – | – | 1 | 1 | 1 | 8 | |
3Re4-18 | Serratia plymuthica | 3 | 3 | 1 | 1 | 1 | 1 | 1 | 1 | – | 12 | |
3Re4-21 | Pseudomonas putida | 3 | 2 | 1 | – | – | – | 1 | 1 | 1 | 9 | |
6Re5–7 | n.d. | 2 | 3 | 1 | – | – | – | 1 | 1 | 1 | 9 | |
Endosphere | 1Pe1-14 | Erwinia rhapontici | 3 | 3 | 1 | – | 1 | – | 1 | 1 | 1 | 11 |
1Pe4-13 | Bacillus subtilis | 3 | 3 | 1 | – | 1 | – | 1 | – | – | 9 | |
2Pe3–3 | Bacillus subtilis | 1 | 2 | 1 | – | 1 | – | 1 | 1 | – | 7 | |
4Pe1–3 | Paenibacillus polymyxa* | 3 | 1 | 1 | – | 1 | 1 | – | 1 | – | 8 | |
5Pe4-14 | Bacillus subtilis* | – | 2 | 1 | – | 1 | 1 | 1 | 1 | – | 7 |
Origin/micro-environment | Strain | Identificationa | Antagonistic activity towardb | Production of: | Assessment points | |||||||
V.d. | R.s. | Hydrolytic enzymec | Metabolite | |||||||||
Cellulases | Chitinases | Glucanases | Pectinases | Proteases | Siderophoresd | IAAe | ||||||
Rhizosphere | 1R1-22 | Micrococcus kristinae* | 3 | 3 | 1 | – | – | – | 1 | 1 | – | 9 |
2R2-11 | Arthrobacter oxydans* | 3 | 3 | 1 | – | 1 | – | 1 | 1 | – | 10 | |
2R2-15 | Bacillus licheniformis | 2 | 2 | 1 | – | 1 | – | 1 | 1 | – | 8 | |
5R3-11 | Streptomyces rochei* | 3 | 3 | 1 | – | 1 | – | – | – | – | 8 | |
6R4-28 | Pseudomonas chlororaphis* | 2 | 3 | – | – | – | – | 1 | 1 | 1 | 8 | |
Phyllosphere | 1P1–2 | Paenibacillus peoriae | 2 | 3 | 1 | – | 1 | 1 | 1 | 1 | – | 11 |
2P3-18 | Staphylococcus epidermidis | – | 2 | 1 | 1 | 1 | 1 | 1 | 1 | – | 8 | |
2P3-25 | Arthrobacter sulfonivorans | – | 2 | 1 | 1 | 1 | 1 | 1 | 1 | 1 | 9 | |
4P2–4 | Bacillus amyloliquefaciens* | 2 | 2 | 1 | – | 1 | 1 | 1 | 1 | – | 9 | |
5P1–8 | n.d. | 3 | 3 | 1 | – | 1 | 1 | 1 | – | – | 10 | |
Endorhiza | 3Re2–7 | Pseudomonas reactans | 3 | 3 | – | – | – | – | 1 | 1 | 1 | 8 |
3Re2-26 | Pseudomonas fluorescens | 3 | 2 | – | – | – | – | 1 | 1 | 1 | 8 | |
3Re4-18 | Serratia plymuthica | 3 | 3 | 1 | 1 | 1 | 1 | 1 | 1 | – | 12 | |
3Re4-21 | Pseudomonas putida | 3 | 2 | 1 | – | – | – | 1 | 1 | 1 | 9 | |
6Re5–7 | n.d. | 2 | 3 | 1 | – | – | – | 1 | 1 | 1 | 9 | |
Endosphere | 1Pe1-14 | Erwinia rhapontici | 3 | 3 | 1 | – | 1 | – | 1 | 1 | 1 | 11 |
1Pe4-13 | Bacillus subtilis | 3 | 3 | 1 | – | 1 | – | 1 | – | – | 9 | |
2Pe3–3 | Bacillus subtilis | 1 | 2 | 1 | – | 1 | – | 1 | 1 | – | 7 | |
4Pe1–3 | Paenibacillus polymyxa* | 3 | 1 | 1 | – | 1 | 1 | – | 1 | – | 8 | |
5Pe4-14 | Bacillus subtilis* | – | 2 | 1 | – | 1 | 1 | 1 | 1 | – | 7 |
aSpecies were identified by FAME analysis (marked by asterics) and/or 16S rDNA sequencing.
bAntagonism toward V. d. =Verticillium dahliae, R.s.=Rhizoctonia solani, dual culture assay: one point represents 0–5 mm wide zone, two points represents 5–10 mm wide zone, three points represents >10 mm wide zone of inhibition.
cOne point represents hydrolysis on plate assay.
dOne point represents production on plate assay.
eOne point represents IAA = indole-3-acetic acid production in vitro.
Assessment of potato-associated strains for biological control and list of the most promising candidates from each microenvironment
Origin/micro-environment | Strain | Identificationa | Antagonistic activity towardb | Production of: | Assessment points | |||||||
V.d. | R.s. | Hydrolytic enzymec | Metabolite | |||||||||
Cellulases | Chitinases | Glucanases | Pectinases | Proteases | Siderophoresd | IAAe | ||||||
Rhizosphere | 1R1-22 | Micrococcus kristinae* | 3 | 3 | 1 | – | – | – | 1 | 1 | – | 9 |
2R2-11 | Arthrobacter oxydans* | 3 | 3 | 1 | – | 1 | – | 1 | 1 | – | 10 | |
2R2-15 | Bacillus licheniformis | 2 | 2 | 1 | – | 1 | – | 1 | 1 | – | 8 | |
5R3-11 | Streptomyces rochei* | 3 | 3 | 1 | – | 1 | – | – | – | – | 8 | |
6R4-28 | Pseudomonas chlororaphis* | 2 | 3 | – | – | – | – | 1 | 1 | 1 | 8 | |
Phyllosphere | 1P1–2 | Paenibacillus peoriae | 2 | 3 | 1 | – | 1 | 1 | 1 | 1 | – | 11 |
2P3-18 | Staphylococcus epidermidis | – | 2 | 1 | 1 | 1 | 1 | 1 | 1 | – | 8 | |
2P3-25 | Arthrobacter sulfonivorans | – | 2 | 1 | 1 | 1 | 1 | 1 | 1 | 1 | 9 | |
4P2–4 | Bacillus amyloliquefaciens* | 2 | 2 | 1 | – | 1 | 1 | 1 | 1 | – | 9 | |
5P1–8 | n.d. | 3 | 3 | 1 | – | 1 | 1 | 1 | – | – | 10 | |
Endorhiza | 3Re2–7 | Pseudomonas reactans | 3 | 3 | – | – | – | – | 1 | 1 | 1 | 8 |
3Re2-26 | Pseudomonas fluorescens | 3 | 2 | – | – | – | – | 1 | 1 | 1 | 8 | |
3Re4-18 | Serratia plymuthica | 3 | 3 | 1 | 1 | 1 | 1 | 1 | 1 | – | 12 | |
3Re4-21 | Pseudomonas putida | 3 | 2 | 1 | – | – | – | 1 | 1 | 1 | 9 | |
6Re5–7 | n.d. | 2 | 3 | 1 | – | – | – | 1 | 1 | 1 | 9 | |
Endosphere | 1Pe1-14 | Erwinia rhapontici | 3 | 3 | 1 | – | 1 | – | 1 | 1 | 1 | 11 |
1Pe4-13 | Bacillus subtilis | 3 | 3 | 1 | – | 1 | – | 1 | – | – | 9 | |
2Pe3–3 | Bacillus subtilis | 1 | 2 | 1 | – | 1 | – | 1 | 1 | – | 7 | |
4Pe1–3 | Paenibacillus polymyxa* | 3 | 1 | 1 | – | 1 | 1 | – | 1 | – | 8 | |
5Pe4-14 | Bacillus subtilis* | – | 2 | 1 | – | 1 | 1 | 1 | 1 | – | 7 |
Origin/micro-environment | Strain | Identificationa | Antagonistic activity towardb | Production of: | Assessment points | |||||||
V.d. | R.s. | Hydrolytic enzymec | Metabolite | |||||||||
Cellulases | Chitinases | Glucanases | Pectinases | Proteases | Siderophoresd | IAAe | ||||||
Rhizosphere | 1R1-22 | Micrococcus kristinae* | 3 | 3 | 1 | – | – | – | 1 | 1 | – | 9 |
2R2-11 | Arthrobacter oxydans* | 3 | 3 | 1 | – | 1 | – | 1 | 1 | – | 10 | |
2R2-15 | Bacillus licheniformis | 2 | 2 | 1 | – | 1 | – | 1 | 1 | – | 8 | |
5R3-11 | Streptomyces rochei* | 3 | 3 | 1 | – | 1 | – | – | – | – | 8 | |
6R4-28 | Pseudomonas chlororaphis* | 2 | 3 | – | – | – | – | 1 | 1 | 1 | 8 | |
Phyllosphere | 1P1–2 | Paenibacillus peoriae | 2 | 3 | 1 | – | 1 | 1 | 1 | 1 | – | 11 |
2P3-18 | Staphylococcus epidermidis | – | 2 | 1 | 1 | 1 | 1 | 1 | 1 | – | 8 | |
2P3-25 | Arthrobacter sulfonivorans | – | 2 | 1 | 1 | 1 | 1 | 1 | 1 | 1 | 9 | |
4P2–4 | Bacillus amyloliquefaciens* | 2 | 2 | 1 | – | 1 | 1 | 1 | 1 | – | 9 | |
5P1–8 | n.d. | 3 | 3 | 1 | – | 1 | 1 | 1 | – | – | 10 | |
Endorhiza | 3Re2–7 | Pseudomonas reactans | 3 | 3 | – | – | – | – | 1 | 1 | 1 | 8 |
3Re2-26 | Pseudomonas fluorescens | 3 | 2 | – | – | – | – | 1 | 1 | 1 | 8 | |
3Re4-18 | Serratia plymuthica | 3 | 3 | 1 | 1 | 1 | 1 | 1 | 1 | – | 12 | |
3Re4-21 | Pseudomonas putida | 3 | 2 | 1 | – | – | – | 1 | 1 | 1 | 9 | |
6Re5–7 | n.d. | 2 | 3 | 1 | – | – | – | 1 | 1 | 1 | 9 | |
Endosphere | 1Pe1-14 | Erwinia rhapontici | 3 | 3 | 1 | – | 1 | – | 1 | 1 | 1 | 11 |
1Pe4-13 | Bacillus subtilis | 3 | 3 | 1 | – | 1 | – | 1 | – | – | 9 | |
2Pe3–3 | Bacillus subtilis | 1 | 2 | 1 | – | 1 | – | 1 | 1 | – | 7 | |
4Pe1–3 | Paenibacillus polymyxa* | 3 | 1 | 1 | – | 1 | 1 | – | 1 | – | 8 | |
5Pe4-14 | Bacillus subtilis* | – | 2 | 1 | – | 1 | 1 | 1 | 1 | – | 7 |
aSpecies were identified by FAME analysis (marked by asterics) and/or 16S rDNA sequencing.
bAntagonism toward V. d. =Verticillium dahliae, R.s.=Rhizoctonia solani, dual culture assay: one point represents 0–5 mm wide zone, two points represents 5–10 mm wide zone, three points represents >10 mm wide zone of inhibition.
cOne point represents hydrolysis on plate assay.
dOne point represents production on plate assay.
eOne point represents IAA = indole-3-acetic acid production in vitro.
4 Discussion
The endophytic and ectophytic potato-associated bacterial communities of field-grown potatoes were analyzed using a multiphasic approach to provide new data on microenvironment-dependent diversity and the occurrence of antagonistic bacteria within each microenvironment.
Our results demonstrated that all four potato microenvironments were colonized by a specific bacterial community. This was shown by cultivation-dependent and cultivation-independent techniques. Cluster analysis of T-RFLP profiles resulted in common clusters for the rhizospheres and phyllospheres. The sampling time throughout the vegetation period had a major effect on bacterial communities. Similar effect was described also by Smalla et al.[34] for bacterial communities of Verticillium host plants and by Heuer et al.[35] for potato plants using denaturing gradient gel electrophoresis (DGGE) of the 16S rDNA. Cultivation-dependent methods were based on the isolation of bacteria from different microenvironments on R2A. Densities of culturable bacteria in endophytic microenvironments confirmed previous reports, which found between 102 and 106 CFU per g plant material as summarized in Hallmann et al.[14]. Proportion of bacterial isolates with antagonistic properties to V. dahliae and R. solani were estimated based on dual culture tests. Although highly variable, the lowest proportion of antagonists was always found in the endosphere, whereas the highest proportion of antagonistic bacteria was detected in the endorhiza. This phenomenon might be explained by the fact that both fungal pathogens are soilborne, attacking primarily the roots and only at later stages the endosphere. The highest variability in the proportion of antagonists over time and between plants was found in the phyllosphere. This variability might be caused by abiotic factors such as drought, rainfall and UV radiation, which cause enormous stress on bacterial survival.
The specificity of bacterial communities for certain microenvironments was additionally confirmed by the diversity and composition of antagonistic bacterial isolates. The highest diversity and richness of antagonistic bacteria was found in the rhizosphere, the lowest in the phyllosphere. However, specific selection of different colony morphologies could lead to increased diversity indices in general compared to random selection. In our study we used this method to obtain a broad and, if possible, complete spectrum of antagonists. Although Pseudomonas species occurred in all microenvironments they were most common in the rhizosphere. Pseudomonas species are known for their beneficial association with the host plants and for their antagonistic activity against plant pathogens [1,31]. A similar degree of colonization with antagonistic bacteria was found in the endorhiza, however, bacterial diversity and richness here was generally lower. It was therefore assumed that antagonistic bacteria in the endorhiza represent a subset of the antagonistic bacterial community in the rhizosphere. Molecular characterization of P. putida isolates indicated that different microenvironments harbored distinct bacterial populations. Only in the rhizosphere and endorhiza we detected isolates of P. putida with nearly identical fingerprints. Furthermore, only in the rhizosphere and endorhiza Pseudomonas contained the phlD gene, but none of the Pseudomonas isolates in the phyllosphere. It was assumed, that this special group of fluorescent pseudomonads occurring in the endorhiza and rhizosphere are part of the antagonistic potential within these microenvironments. A correlation between the antagonistic mechanisms in vitro and the observed antagonistic activity to the two fungi on plates was not found.
Although both the cultivation-dependent and cultivation-independent approaches gave a complementary representation of the bacterial communities of potato plants and confirmed specificity for microenvironments, they also showed differences among the microenvironments. Cultivation-independent approach suggested a high similarity between the endorhiza and endosphere especially towards the end of the vegetation period. In contrast, cultivation-dependent techniques showed, at least for antagonistic bacteria, high similarities between the rhizosphere and endorhiza. Antagonistic bacteria in the endorhiza seem not to be as well distributed throughout the plant as other endophytic bacteria as indicated by microscopic observations of introduced endophytes [36,37].
In a second part of this study mutitarget bacterial antagonists were characterized and identified. A total of 349 isolates exhibiting antagonism to V. dahliae and/or R. solani were identified. Strains that are known as facultative pathogens of humans such as Bacillus cereus, Staphylococcus epidermis and S. pasteuri, were detected. In addition, 16S rRNA gene sequences of some isolates showed high homology to plant pathogenic bacteria, e.g., C. flaccumfaciens and Erwinia amylovora. Both groups of pathogenic bacteria were also found as endophytes of potato [20,21] showing that the distinction between plant-beneficial endophytes and pathogens can be unclear. Isolates with potentially pathogenic characters would have to be excluded from applications in biocontrol. For bacterial isolates with antagonistic properties against fungal pathogens and therefore potential as biocontrol agents, an assessment scheme was developed to enhance screening of efficacious isolates. This assessment scheme included information on biocontrol activity against fungal pathogens and physiological reactions. Production of antibiotics was excluded from the assessment. The use of antibiotic-producing biological control agents can be problematic due to possible development of resistance fungal strains. Furthermore, any risk of antibiotics being released into the plant tissue and affect food quality or human health has to be excluded. Based on this assessment scheme, 20 isolates were considered good biocontrol candidates with broad-spectrum control against different fungal pathogens. These isolates included species of the genera Streptococcus, Streptomyces, Pseudomonas and Serratia and included several isolates of species previously unknown as antagonists. The antagonistic activity of the most efficient in vitro antagonist S. plymuthica 3Re4-18 could be confirmed in another study using the pathosystems R. solani– potato and lettuce under greenhouse and field conditions[26].
In conclusion, a high degree of microenvironment specificity was evidenced in field-grown potatoes. All plant microenvironments were colonized by a distinguishable antagonistic bacterial population. In addition, the study led to the collection of a broad spectrum of antagonistic bacteria, which now need to be further exploited for future applications as biological control agents of plant pathogens or for plant growth enhancement.
Acknowledgements
The authors thank Annekathrin Faupel (Bonn, Stade) for help during samplings and FAME analysis, Hella Goschke (Rostock) for her valuable technical assistance in the lab, and Susann Schmidt (Rostock) for analyzing phl D gene in Pseudomonas strains. This study was partially funded by the Deutsche Forschungsgemeinschaft.
References