-
PDF
- Split View
-
Views
-
Cite
Cite
Keiji Watanabe, Noritaka Takihana, Hideki Aoyagi, Satoshi Hanada, Yoshitomo Watanabe, Naoya Ohmura, Hiroshi Saiki, Hideo Tanaka, Symbiotic association in Chlorella culture, FEMS Microbiology Ecology, Volume 51, Issue 2, January 2005, Pages 187–196, https://doi.org/10.1016/j.femsec.2004.08.004
- Share Icon Share
Abstract
Chlorella sorokiniana IAM C-212 has long been maintained in slant culture as a mixed strain, representing an associated natural microbial consortium. In this study, the consortium was separated and five nonalgal constituents, a fungal strain (CSSF-1), and four bacterial strains (CSSB-1, CSSB-2, CSSB-3, and CSSB-4) were isolated and identified. 16S rDNA sequence analysis revealed that strains CSSB-1, CSSB-2, CSSB-3, and CSSB-4 were close to Ralstonia pickettii (99.8% identity), Sphingomonas sp. DD38 (99.4% identity), Microbacterium trichotecenolyticum (98.6% identity), and Micrococcus luteus (98.6% identity) respectively. 18S rDNA sequence analysis revealed that strain CSSF-1 resembled Acremonium-like hyphomycete KR21–2 (98.8%). The fungal strain CSSF-1 and one of the bacterial strains, CSSB-3, were found to promote the growth of Chlorella while the presence of bacterial strains CSSB-1 and CSSB-2 had no effect. Strain CSSB-4 could not be subcultured so its role was not elucidated. These results show that the interaction between Chlorella and its symbionts under photoautotrophic conditions involved both mutualism and commensalisms. The chlorophyll content of mixed strain was stable in long-term cultivation (7 months) while the chlorophyll content of a pure culture showed a marked decline. Electron microscopic analysis showed the two bacterial strains CSSB-2 and CSSB-3 were harbored on the sheath excreted by Chlorella, while the fungal strain CSSF-1 and the bacterial strain CSSB-1 directly adhered to the Chlorella cell surface.
This report is the first observation of a symbiotic relationship among fungus, bacteria, and Chlorella, and the first observation of direct adhesion of fungus and bacteria to Chlorella in a consortium.
1 Introduction
Symbiotic associations play an important role in natural ecosystems and contribute usefully to industrial microbiology. In natural ecosystems, various symbiotic associations are well known, including rhizobia–legume, termite–enterobacterium, arbuscular mycorrhizal fungi (AMF)–plant, and lichen symbiosis [1–4]. Especially, the lichen symbiosis is well-known for its three dimensional structure where an algae supplies carbohydrate to a fungi by photosynthesis and the fungi shelters the algae from predators in the environment. These associations may have developed as rational survival systems in natural selection.
In industrial microbiology pure cultures are most common but symbiotic associations are being used in the fermented food industries and waste water treatment of activated sludge. The detailed mechanism of these symbioses is not well understood, their utilization is based on empirical knowledge. A thorough understanding of symbiotic mechanisms may allow more effective exploitation of symbiotic association in industrial applications. From this viewpoint, we investigated a symbiotic association between Chlorella sorokiniana and its symbionts as a simple symbiotic association.
Mutualistic associations between microalgae and heterotrophic bacteria have been reported with bacterial uptake of extracellular organic carbon (EOC) released during the microalgal photosynthesis, and microalgal uptake of growth promoting factors produced by heterotrophic bacteria [5–8]. Microalgae often excrete organic matter such as carbohydrate, nitrogen, organic acid, lipid, and vitamins during photosynthesis[9], and biotin, proline, and glycolic acid have also been reported as released extracellular organic matter from Chlorella[9–11]. Previous investigators found little or no benefit in terms of enhanced Chlorella growth from these symbiotic associations but rather, regarded them as microbial contamination [12–14]. In this paper, we provide further information on the symbiotic relationship between C. sorokiniana and its symbionts, including uptake of EOC by symbionts, enhanced microalgal growth, and adhesional interaction.
2 Materials and methods
2.1 Culture of Chlorella and symbionts
C. sorokiniana IAM C-212 was obtained from the culture collection center of the Institute of Applied Microbiology, University of Tokyo (IAM). The strain and its associated symbionts, have been continuously subcultured for more than 30 years. In our laboratory, the culture was routinely subcultured following method of Ogbonna et al.[15]. In brief, every 4 weeks the culture was transferred into a fresh photoautotrophic medium composed of the following salts (per liter of distilled water): 5.0 g of KNO3, 1.25 g of KH2PO4, 0.1 g of K2HPO4, 2.5 g of MgSO4.7H2O, 1.8 g of NaCl, 0.04 g of FeSO4.7H2O, 0.03 g of EDTA, and 1 ml of A5 solution (containing 2.86 g of H3BO4, 1.81 g of MnCl2.4H2O, 0.22 g of ZnSO4.7H2O, 0.08 g of CuSO4.5H2O, and 0.021 g of Na2MoO4.2H2O per liter of distilled water). One liter of this medium was put into a 2 l Roux flask, the pH was adjusted to 6.0 with 1 N NaOH, and it was autoclaved at 121 °C for 15 min. Five ml of subcultured C. sorokiniana was then inoculated to this medium. The cells were incubated at 37 °C under cool-white fluorescent lamps at 360 μmol m−2 s−1 for 4 weeks. Aeration and mixing were achieved by sparging air enriched with 5% CO2 through a glass-ball filter (Kinoshita Rika, Japan) at the rate of 0.3 vol vol−1 min−1 (vvm).
2.2 Isolation and identification of symbiotic microorganisms from Chlorella
After 4 weeks of cultivation, the culture broth contained a high concentration of the symbionts. C. sorokiniana cells were then removed from the culture broth by centrifugation (1500g for 5 min at 4 °C). The symbionts in the supernatant were inoculated into heterotrophic media consisting 10 g of glucose, 5 g of peptone, 2 g of yeast extract, 1 g of MgSO4.7H2O, and 20 g of agar per liter distilled water. The plates were incubated at 30 °C without illumination. After colony formation, single colony isolation was achieved by repeated transfers into the same solid medium. During the single colony isolation, no evidence of C. sorokiniana growth was observed.
2.3 DNA extraction
A small amount of symbiont cells, either fungal or bacterial, were picked from a colony and suspended in 200 μl distilled water and centrifuged (12,000 × g for 5 min at 4 °C). The pellets were re-suspended in 40 μl distilled water. The suspensions were frozen and thawed two times (−80 and 60 °C) and disrupted five times by sonification (15 W for 2 s) using a SONIFIER 250 (Branson). Proteinase K (10 μl of a 1 mg ml−1 solution) (Takara, Japan) and 50 μl of BL buffer (40 mM Tris (2-amino-2-hydroxymethyl-1, 3-propanediol), 1% (v/v) Tween 20, 0.2% (v/v) Nonidet P-40, 0.2 mM EDTA, pH 8.0) were added to the suspensions and gently mixed. The solutions were incubated for 20 min at 60 °C and 10 min at 95 °C. The suspensions were centrifuged (12,000 × g for 5 min at 4 °C) and 1 μl of supernatants were diluted to 49 μl with distilled water and the OD260 were measured[16].
2.4 Polymerase chain reaction amplification of 16S and 18S rDNA
Amplification of the 16S rDNA was carried out using universal primers 16S-Uni530F and 16S-Eu/Ar1500R. In the case of 18S rDNA, universal primers 18S-Euk1F and 18S-Euk1800R were used (primers are shown in Table 1). Amplification reaction mixtures contained 66.5 μl of distilled water, 10 μl of 10 × PCR buffer, 10 μl of dNTP mixture, 4 μl of each primer (10 pmol), 0.5 μl of AmpliTaq Gold DNA polymerase (Applied Biosystems) and 5 μl of DNA template (100 ng μl−1). Reactions were carried out as follows: 9 min at 95 °C (preheat), 35 cycles of 1 min at 95 °C (denaturation), 1 min at 50 °C (annealing) and 2 min at 72 °C (extension), with a final 4 °C after cycling was completed. PCR products were checked by electrophoresis on 1% agarose gel with TBE electrophoresis buffer (containing 5.4 g of Tris, 2.75 g of boric acid, and 8 ml of 0.5 M EDTA per liter of distilled water).
Primer name | Primer sequence (5′ to 3′) | Position | References |
16S-Uni530F | GTGCCAGCMGCCGCGG | Escherichia coli 530-545 | [18] |
16S-Eu/Ar1500R | GGTTACCTTGTTACGACTT | Escherichia coli 1510-1492 | [17] |
16S-Eu10F | AGAGTTTGATCCTGGCTCAG | Escherichia coli 8–27 | [17] |
16S-Eu500R | GTATTACCGCGGCTGCTGG | Escherichia coli 536-518 | [17] |
16S-Eu800R | CATCGTTTACGGCGTGGAC | Escherichia coli 821-803 | [17] |
16S-Hu1100F | AAGTCCCGCAACGAGCGCA | Escherichia coli 1092-1110 | This study |
16S-Eu1100R | TTGCGCTCGTTGCGGGACT | Escherichia coli 1111-1093 | [17] |
16S-Eu1400R | ACGGGCGGTGTGTACAAG | Escherichia coli 1406-1389 | [17] |
18S-Euk1F | TATCTGGTTGATCCTGCCAG | Saccharomyces cereviciae 1–20 | [19] |
18S-Euk1800R | TGATCCTTCCGCAGGTTCACCT | Saccharomyces cereviciae 1787-1766 | [19] |
18S-Euk500R | CCAGACTTGCCCTCCAATTG | Saccharomyces cereviciae 562-543 | [20] |
18S-Euk900F | GGTGAAATTCTTGGATTTATTG | Saccharomyces cereviciae 900-921 | [20] |
18S-Euk1100F | GGAGTATGGTCGCAAGGCTG | Saccharomvces cereviciae 1108-1127 | [20] |
18S-Euk1100R | CAGCCTTTGCGACCATACTCC | Saccharomyces cereviciae 1119-1099 | [19] |
Primer name | Primer sequence (5′ to 3′) | Position | References |
16S-Uni530F | GTGCCAGCMGCCGCGG | Escherichia coli 530-545 | [18] |
16S-Eu/Ar1500R | GGTTACCTTGTTACGACTT | Escherichia coli 1510-1492 | [17] |
16S-Eu10F | AGAGTTTGATCCTGGCTCAG | Escherichia coli 8–27 | [17] |
16S-Eu500R | GTATTACCGCGGCTGCTGG | Escherichia coli 536-518 | [17] |
16S-Eu800R | CATCGTTTACGGCGTGGAC | Escherichia coli 821-803 | [17] |
16S-Hu1100F | AAGTCCCGCAACGAGCGCA | Escherichia coli 1092-1110 | This study |
16S-Eu1100R | TTGCGCTCGTTGCGGGACT | Escherichia coli 1111-1093 | [17] |
16S-Eu1400R | ACGGGCGGTGTGTACAAG | Escherichia coli 1406-1389 | [17] |
18S-Euk1F | TATCTGGTTGATCCTGCCAG | Saccharomyces cereviciae 1–20 | [19] |
18S-Euk1800R | TGATCCTTCCGCAGGTTCACCT | Saccharomyces cereviciae 1787-1766 | [19] |
18S-Euk500R | CCAGACTTGCCCTCCAATTG | Saccharomyces cereviciae 562-543 | [20] |
18S-Euk900F | GGTGAAATTCTTGGATTTATTG | Saccharomyces cereviciae 900-921 | [20] |
18S-Euk1100F | GGAGTATGGTCGCAAGGCTG | Saccharomvces cereviciae 1108-1127 | [20] |
18S-Euk1100R | CAGCCTTTGCGACCATACTCC | Saccharomyces cereviciae 1119-1099 | [19] |
Primer name | Primer sequence (5′ to 3′) | Position | References |
16S-Uni530F | GTGCCAGCMGCCGCGG | Escherichia coli 530-545 | [18] |
16S-Eu/Ar1500R | GGTTACCTTGTTACGACTT | Escherichia coli 1510-1492 | [17] |
16S-Eu10F | AGAGTTTGATCCTGGCTCAG | Escherichia coli 8–27 | [17] |
16S-Eu500R | GTATTACCGCGGCTGCTGG | Escherichia coli 536-518 | [17] |
16S-Eu800R | CATCGTTTACGGCGTGGAC | Escherichia coli 821-803 | [17] |
16S-Hu1100F | AAGTCCCGCAACGAGCGCA | Escherichia coli 1092-1110 | This study |
16S-Eu1100R | TTGCGCTCGTTGCGGGACT | Escherichia coli 1111-1093 | [17] |
16S-Eu1400R | ACGGGCGGTGTGTACAAG | Escherichia coli 1406-1389 | [17] |
18S-Euk1F | TATCTGGTTGATCCTGCCAG | Saccharomyces cereviciae 1–20 | [19] |
18S-Euk1800R | TGATCCTTCCGCAGGTTCACCT | Saccharomyces cereviciae 1787-1766 | [19] |
18S-Euk500R | CCAGACTTGCCCTCCAATTG | Saccharomyces cereviciae 562-543 | [20] |
18S-Euk900F | GGTGAAATTCTTGGATTTATTG | Saccharomyces cereviciae 900-921 | [20] |
18S-Euk1100F | GGAGTATGGTCGCAAGGCTG | Saccharomvces cereviciae 1108-1127 | [20] |
18S-Euk1100R | CAGCCTTTGCGACCATACTCC | Saccharomyces cereviciae 1119-1099 | [19] |
Primer name | Primer sequence (5′ to 3′) | Position | References |
16S-Uni530F | GTGCCAGCMGCCGCGG | Escherichia coli 530-545 | [18] |
16S-Eu/Ar1500R | GGTTACCTTGTTACGACTT | Escherichia coli 1510-1492 | [17] |
16S-Eu10F | AGAGTTTGATCCTGGCTCAG | Escherichia coli 8–27 | [17] |
16S-Eu500R | GTATTACCGCGGCTGCTGG | Escherichia coli 536-518 | [17] |
16S-Eu800R | CATCGTTTACGGCGTGGAC | Escherichia coli 821-803 | [17] |
16S-Hu1100F | AAGTCCCGCAACGAGCGCA | Escherichia coli 1092-1110 | This study |
16S-Eu1100R | TTGCGCTCGTTGCGGGACT | Escherichia coli 1111-1093 | [17] |
16S-Eu1400R | ACGGGCGGTGTGTACAAG | Escherichia coli 1406-1389 | [17] |
18S-Euk1F | TATCTGGTTGATCCTGCCAG | Saccharomyces cereviciae 1–20 | [19] |
18S-Euk1800R | TGATCCTTCCGCAGGTTCACCT | Saccharomyces cereviciae 1787-1766 | [19] |
18S-Euk500R | CCAGACTTGCCCTCCAATTG | Saccharomyces cereviciae 562-543 | [20] |
18S-Euk900F | GGTGAAATTCTTGGATTTATTG | Saccharomyces cereviciae 900-921 | [20] |
18S-Euk1100F | GGAGTATGGTCGCAAGGCTG | Saccharomvces cereviciae 1108-1127 | [20] |
18S-Euk1100R | CAGCCTTTGCGACCATACTCC | Saccharomyces cereviciae 1119-1099 | [19] |
2.5 16S or 18S rDNA sequence, phylogenetic analysis, and microscopic observation
The PCR products were sequenced directly on ABI 377 DNA sequencer using a dRhodamine Dye Terminator cycle sequencing kit (Applied Biosystems). The 16S rDNA sequence primers 16S-Eu10F, 16S-Eu500R, 16S-Eu800R, 16S-Eu1100F, 16S-Eu1100R and 16S-Eu1400R were used for bacterial samples and 18S-Euk500R, 18S-Euk900F, 18S-Euk1100F and 18S-Euk1100R primers were used for fungal samples. Sequences of all primers are shown in Table 1.
The sequences obtained were compared with known sequences using the FASTA service. Sequence data were aligned with the CLUSTAL W package[21]. Phylogenetic trees were constructed by the neighbor-joining method within the MEGA2 package [22,23]. Bootstrap resampling analysis was performed to estimate the confidence of tree topologies (1000 replicates)[24].
Microscopic observation of the fungus morphology was also undertaken to supplement the 18S rDNA analysis.
2.6 Nucleotide sequence accession number
The nucleotide sequences of partial 16S or 18S rDNA genes have been deposited in the DDBJ nucleotide sequences databank under the following accession numbers: CSSB-1: AB167381; CSSB-2: AB167382; CSSB-3: AB16783; CSSB-4: AB167385; CSSF-1: AB167384.
2.7 Purification of Chlorella culture
C. sorokiniana was purified from the consortium by addition of antibiotics. Streptomycin, gentamicin, penicillin G, vancomycin and pimaricin were selected to inhibit bacterial and/or fungal growth. For screening their inhibitory properties, each antibiotic solution was filtered through a 0.2 μm cellulose acetate filter (ADVANTEC, DISMIC-25cs), and combined with heterotrophic medium at a final concentration of 10 mg l−1 (pimaricin), 100 mg l−1 (gentamicin or vancomycin) or 1000 mg l−1 (streptomycin or penicillin G). To each antibiotic-media solution, one of the symbionts (bacteria or the fungus) was inoculated (1 × 107 cells) and incubated in a water bath at 30 °C for three days.
The inhibitory effects of the antibiotics on symbionts were monitored by measuring at OD580. The effect of each antibiotic on the growth of C. sorokiniana was also investigated by monitoring the cell density at OD680. To remove the symbionts from Chlorella culture, all the antibiotics were added to 100 ml of autotrophic medium in Erlenmayer flask with a silicon stopper. A small amount of C. sorokiniana consortium was inoculated and incubated at 30 °C for 30 days. After the incubation, the culture broth was observed by microscopy and inoculated on heterotrophic agar plate to confirm the purity of Chlorella strain.
2.8 Analysis of total organic carbon
The purified C. sorokiniana was incubated in Roux flask containing 1 l of the autotrophic medium at 37 °C under illumination (360 μmol m−2 s−1). Aeration was done by sparging air enriched with 5% CO2 through a glass-ball filter at 0.3 vvm. A 10 ml sample of the culture broth was removed every two days for analysis. Chlorella growth was determined by measuring the OD680 of the culture broth. The sample was then centrifuged (5000 × g for 15 min at 4 °C) and filtered with a 0.2 μm filter (to remove algal cells) prior to use for total organic carbon (TOC) analysis. The TOC measurement (TOC-650, Toray Engineering, Tokyo Japan) was taken to represent the EOC in the culture medium.
2.9 Growth of symbionts on EOC
The heterotrophic growth of the symbionts on EOC produced by C. sorokiniana was investigated according to the following method. The culture broth containing EOC was prepared by cultivating the purified C. sorokiniana under photoautotrophic condition for two weeks. The culture broth was centrifuged (5000g, for 15 min at 4 °C) to remove the cells, and then filtered through a sterile 0.2 μm pore size filter. This filtrate was mixed with an equal volume of the fresh photoautotrophic medium for the supplement of inorganic salt. Control culture was done with fresh photoautotrophic medium without EOC. A small amount of the bacteria or the fungus was inoculated in a 500 ml Erlenmayer flask containing 100 ml of this medium. After the inoculation, each culture was incubated at 30 °C without illumination on rotary shaker at 200 rpm. The growth of each symbiont was monitored at OD580.
2.10 Mixed culture
Symbionts and C. sorokiniana were precultured as mono cultures on heterotrophic (for symbionts) or autotrophic (C. sorokiniana) liquid medium before mixing the cultures. Each symbiont culture broth was centrifuged (5000g, for 15 min at 4 °C) to remove heterotrophic liquid medium, washed three times and finally resuspended in autotrophic medium. One ml of each symbiont solution (1 × 108 cells ml−1) was mixed with 1 ml of the purified C. sorokiniana solution (2 × 108 cells ml−1) in 500 ml Erlenmayer flask containing 200 ml photoautotrophic medium sealed with a silicon topper. Mixed cultures of C. sorokiniana with each of the four symbionts were investigated. The mixed cultures were incubated at 30 °C under illumination (360 μmol m−2 s−1) on rotary shaker at 200 rpm. Purified C. sorokiniana was used as a control culture. The time course of the growth of Chlorella was monitored at OD680. All experiments were done five times.


Effects of symbiont addition on growth rate of C. sorokiniana were evaluated with one-way analysis of variance (ANOVA) at day 7 and means were compared by Tukey's HSD (honestly significant difference) multiple comparison procedure with α set at 0.05. All analyses were performed using the SPSS 10.0 for windows.
2.11 Observation of symbiotic conformation using scanning electron microscope
A small quantity of C. sorokiniana cells was taken out from the mixed culture by centrifugation. Each sample was washed 2–3 times with phosphate-buffered saline (PBS, 137 mM NaCl, 3 mM KCl, 20 mM Na2HPO4, 1.5 mM KH2PO4, pH 7.4). Two hundred and fifty microliters of C. sorokiniana cells solution (3 × 107 cells ml−1) were fixed using 250 μl of 0.2 M sodium cacodylate, 250 μl of 4% (v/v) glutaraldehyde, and 250 μl of 0.2 M phosphate buffer (containing 5.28 g of NaH2PO4.H2O and 43.46 g of Na2HPO4.7H2O per liter distilled water, pH 7.4), and once again fixed with 250 μl of 0.2 M sodium cacodylate and 250 μl of 4% osmic acid, and 250 μl of 0.2 M phosphate buffer (pH 7.4). The fixed sample was dehydrated sequentially with 50%, 70%, 80%, 90%, and 100% (v/v) ethanol. Finally 100% ethanol was exchanged with 2-methyl-2-propanol. This sample was dried with a freeze drier (ES-2030, HITACHI, Tokyo, Japan), and coated with Pt–Pd (E-1030, HITACHI, Tokyo, Japan). The sample was observed with scanning electron microscope (S-4200, HITACHI, Tokyo, Japan) at magnifications ranging from × 5000 to × 15,000.
2.12 Long-time cultivation of consortium or purified Chlorella strain and time course of chlorophyll content
Test tubes containing 10 ml of photoautotrophic medium were autoclaved. Consortium and purified C. sorokiniana strains were inoculated to the medium individually and incubated at 30 °C under cool-white fluorescent lamps at 120 μmol m−2 s−1 for 7 months under the slant condition.
After the cultivation, 4 ml of PBS (pH 7.4) was added in the test tubes and Chlorella cells were suspended in PBS using an inoculation loop. The cell concentration was determined by counting under a phase-contrast microscope at 400× magnification. For chlorophyll concentration measurements, the cell suspension was centrifuged (5000g, for 15 min at 4 °C) to collect the cells, and the precipitate was re-suspended in 5 ml of 100% DMF (N, N′-dimethylformamide) and incubated at 4 °C for 24 h. Absorption was measured at two wavelengths, 646.6 and 663.6 nm by spectrophotometer (V-550, JASCO, Tokyo, Japan) zeroed at 750.0 nm. The values obtained at each wavelength were used to calculate the micromolar (μM) concentration of chlorophyll. The equation for determining chlorophyll (Chl) concentration was[25]: Total chlorophyll concentration (Chls a+b) = 19.43A646.6+ 8.05A663.6. The time course of chlorophyll content (μM cell− 1) of Chlorella cell was calculated from cell concentration and chlorophyll concentration every month. All experiments were done in triplicate.
3 Results
3.1 Isolation and characterization of symbionts
The heterotrophic microorganisms separated from C. sorokiniana were cultivated on agar plates. In the initial inoculation, four bacterial strains CSSB-1, CSSB-2, CSSB-3, CSSB-4, and one fungal strain CSSF-1 formed colonies. The identification of the isolated bacteria was carried out by 16S rDNA analysis. The strain CSSB-1 showed 99.8% similarity with Ralstonia pickettii (AJ270260) belonging to β-Proteobacteria and related to the genus Ralstonia (Fig. 1(a)). The strain CSSB-2 was 99.4% similar to Sphingomonas sp. DD38 (AF105023) belonging to α-Proteobacteria. It was affiliated to the genus Sphingomonas (Fig. 1(b)). The strain CSSB-3 showed 98.6% similarity with Microbacterium trichotecenolyticum (Y17240), while strain CSSB-4 showed 98.6% similarity with Micrococcus luteus (AJ409096), and the two strains belong to high G + C Gram-positive bacteria. The strains CSSB-3 and CSSB-4 were assigned to the genus Microbacterium and the genus Micrococcus respectively (Figs. 1(c) and (d)). Although CSSB-4 formed colonies in the first inoculations, it could not be subcultured in subsequent inoculations. For this reason, its symbiotic role, if any, could not be elucidated.
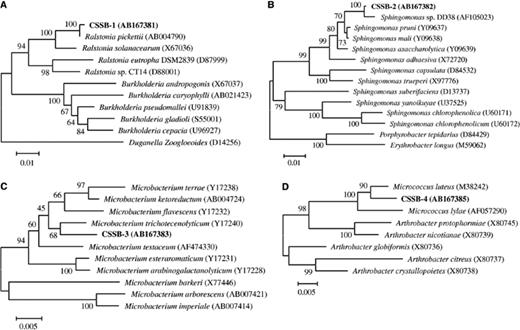
Neighbor-joining phylogenetic tree based on bacterial 16S rDNA sequence. The numbers at nodes represent bootstrap values resampled 1000 times. These trees were based on: (A) CSSB-1, (B) CSSB-2, (C) CSSB-3, and (D) CSSB-4 sequences.
The fungal strain CSSF-1 was investigated by both 18S rDNA and microscopic analysis. The 18S rDNA of strain CSSF-1 showed 98.8% similarity with Acremonium-like hyphomycete KR21–2 (AB108787), 95.8% similarity with Cordyceps sinensis (AB067700), 95.7% similarity with Nectria cinnabarina (AB003949), 95.7% similarity with Geosmithia putterillii (AB031390), 95.6% similarity with Gibberella pulicaris (AF081467) and Chaetopsina fulva (AB003786), 95.2% similarity with Geosmithia lavendula (D14405), and 95.0% similarity with Acremonium alternatum (AY083232). C. sinensis, N. cinnabarina, and G. pulicaris are known to belong to the order Hypocreales, and C. fulva and V. lecanii are related to the anamorphic Hypocreaceae. About half of the strains on phylogenetic tree based on fungal 18S rDNA belonged to the order Hypocreales or the family anamorphic Hypocreaceae (Fig. 2). On the other hand, microscopic observation showed that the fungus produced phialoconidium. Monocellular columniform conidium formed on the top of the phialide and dropped one by one. These morphological characteristics agreed with the general description for the genus Acremonium. It is well known that the teleomorph of the genus Acremonium belong to Ascomycota, and in general, is related to the order Hypocreales (for example the genus Nectria).
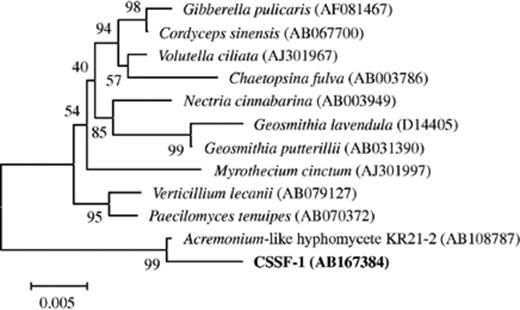
Neighbor-joining phylogenetic tree based on fungal 18S rDNA sequence. The numbers at nodes represent bootstrap values resampled 1000 times.
On the bases of both the 18S rDNA analysis and the microscopic observation, we tentatively identify this fungal strain CSSF-1 as an Acremonium sp.
3.2 Purification of Chlorella culture
Pure culture of C. sorokiniana was isolated from the consortium by the addition of antibiotics. Pimaricin, penicillin G, streptomycin, gentamicin, and vancomycin were used to inhibit bacterial and/or fungal growth (Table 2). As shown in the table, addition of the various antibiotics or fungicide affected the growth of the various species to differing degrees, but none of them inhibited algal growth completely. Therefore, purification of C. sorokiniana was affected by adding all the antibiotics simultaneously to the consortium. After 30 days of cultivation, the culture broth was observed by microscopy and inoculated into heterotrophic medium (no growth was observed) to confirm that the symbionts had been removed from the Chlorella strain.
Antibiotics | CSSB-1 | CSSB-2 | CSSB-3 | CSSF-1 | C. sorokiniana |
Streptomycin | 0.003 ± 0.005 | 0.019 ± 0.002 | NDa | ND | 0.371 ± 0.021 |
Gentamycin | ND | 0.008 ± 0.005 | 0.015 ± 0.007 | ND | 0.371 ± 0.026 |
Penicillin G | ND | ND | 0.013 ± 0.008 | ND | 1.767 ± 0.404 |
Vancomycin | ND | ND | 0.016 ± 0.018 | ND | 1.810 ± 0.173 |
Pimaricin | ND | ND | ND | 0.008 ± 0.005 | 0.652 ± 0.041 |
Antibiotics | CSSB-1 | CSSB-2 | CSSB-3 | CSSF-1 | C. sorokiniana |
Streptomycin | 0.003 ± 0.005 | 0.019 ± 0.002 | NDa | ND | 0.371 ± 0.021 |
Gentamycin | ND | 0.008 ± 0.005 | 0.015 ± 0.007 | ND | 0.371 ± 0.026 |
Penicillin G | ND | ND | 0.013 ± 0.008 | ND | 1.767 ± 0.404 |
Vancomycin | ND | ND | 0.016 ± 0.018 | ND | 1.810 ± 0.173 |
Pimaricin | ND | ND | ND | 0.008 ± 0.005 | 0.652 ± 0.041 |
The values are mean OD580± SD (n= 3).
aND, not determined.
Antibiotics | CSSB-1 | CSSB-2 | CSSB-3 | CSSF-1 | C. sorokiniana |
Streptomycin | 0.003 ± 0.005 | 0.019 ± 0.002 | NDa | ND | 0.371 ± 0.021 |
Gentamycin | ND | 0.008 ± 0.005 | 0.015 ± 0.007 | ND | 0.371 ± 0.026 |
Penicillin G | ND | ND | 0.013 ± 0.008 | ND | 1.767 ± 0.404 |
Vancomycin | ND | ND | 0.016 ± 0.018 | ND | 1.810 ± 0.173 |
Pimaricin | ND | ND | ND | 0.008 ± 0.005 | 0.652 ± 0.041 |
Antibiotics | CSSB-1 | CSSB-2 | CSSB-3 | CSSF-1 | C. sorokiniana |
Streptomycin | 0.003 ± 0.005 | 0.019 ± 0.002 | NDa | ND | 0.371 ± 0.021 |
Gentamycin | ND | 0.008 ± 0.005 | 0.015 ± 0.007 | ND | 0.371 ± 0.026 |
Penicillin G | ND | ND | 0.013 ± 0.008 | ND | 1.767 ± 0.404 |
Vancomycin | ND | ND | 0.016 ± 0.018 | ND | 1.810 ± 0.173 |
Pimaricin | ND | ND | ND | 0.008 ± 0.005 | 0.652 ± 0.041 |
The values are mean OD580± SD (n= 3).
aND, not determined.
3.3 Extracellular organic carbon excreted by Chlorella
A pure strain of C. sorokiniana was aerobically cultured on basal salt medium with 5% CO2 enriched air under light illumination. The time course of TOC produced by Chlorella extracellularly was traced during the entire cultivation. The concentration of TOC increased with increase in the concentration of algae in the medium. After 12 days of cultivation, the growth of Chlorella reached its stationary phase, while TOC concentration also reached a plateau at 750 mg l−1 (Fig. 3). These observations clearly indicated that Chlorella released organic carbon into the medium during photoautotrophic growth. The sheath production by C. sorokiniana was also observed microscopically (data not shown).
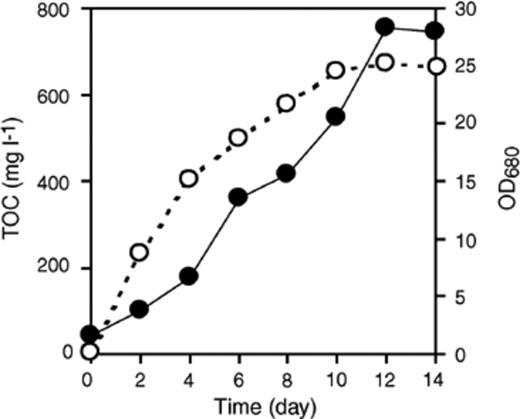
Growth of C. sorokiniana and EOC production. A pure culture of C. sorokiniana was grown under autotrophic conditions for 14 days. Growth of C. sorokiniana (OD680, ●) and total organic carbon (O) were measured daily, and reached plateaus by the 12th day.
The growth of symbionts in EOC was examined. When the symbionts were cultivated on EOC from the pure C. sorokiniana culture, two bacterial strains, CSSB-1 and CSSB-2 grew well (up to three days), and reached their stationary phase. CSSB-3 grew very well up to six days, and reached its stationary phase (Fig. 4). On the other hand, CSSF-1 grew and reached stationary phase within 30 h (Fig. 5). None of the microorganisms showed any growth in the absence of EOC in the medium. This indicated that Chlorella provides a usable carbon source for heterotrophic growth of both bacteria and fungus under photoautotrophic conditions.
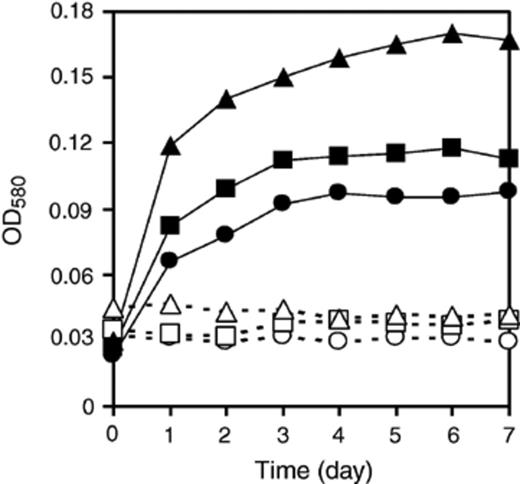
Growth of symbiotic bacteria on EOC. All three bacteria are able to use the C. sorokiniana EOC for growth. Open symbols (O, δ, ◻) are control cultures without EOC, closed symbols (●, Δ, ◻) are with EOC. CSSB-1 (O, ●), CSSB-2 (δ, Δ), and CSSB-3 (◻, ◻).
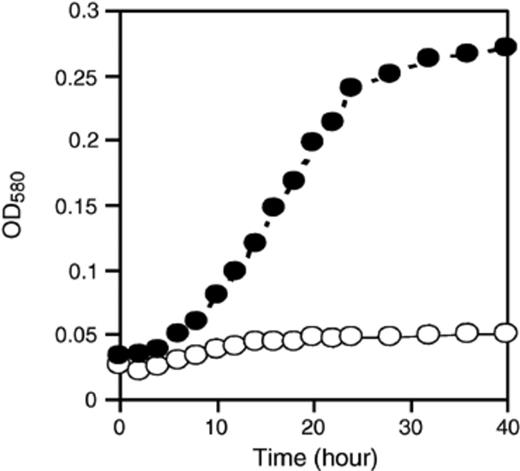
3.4 Effect of symbionts on Chlorella growth
A mixture of pure strain of C. sorokiniana and each of the four successfully subcultured symbionts was cultivated to investigate the effect of each symbiont on the C. sorokiniana growth. Time courses of OD680 of mixed cultures (average of five replicate data) are shown in Fig. 6(a). Effects of symbiont addition on growth rate of C. sorokiniana at day 7 are shown in Fig. 6(b). Tukey's HSD multiple comparison procedure showed significant growth-promotion effects (vs. pure culture) for mixed culture with CSSB-3 (P= 0.011) or CSSF-1 (P= 0.031). However, there was no significant growth promotion effect for CSSB-1 (P= 0.957) or CSSB-2 (P= 0.977).
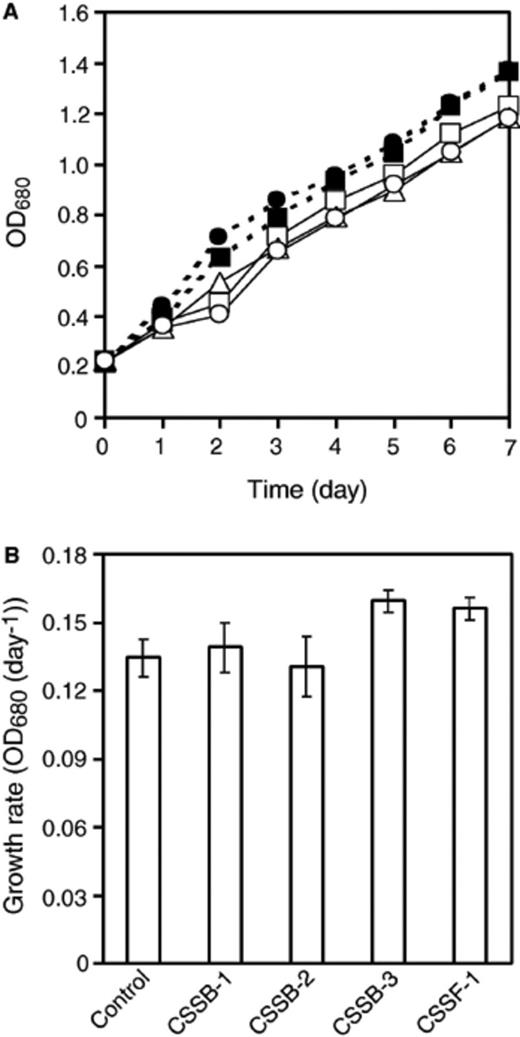
Growth of C. sorokiniana in mixed culture with symbionts (panel A) and growth rate at day 7 (panel B). Control culture (no symbionts) (O), with CSSB-1 (◻), with CSSB-2 (δ), with CSSB-3 (●) and, with CSSF-1 (◻). Error bars in panel B are ±SD (n= 5).
3.5 Observation of symbiotic conformation using scanning electron microscope
Four different samples of symbionts and C. sorokiniana from the mixed cultures described above were observed using scanning electron microscopy at magnification ranges of 5000–15,000. Microscopic observation showed that CSSB-1 and CSSF-1 adhered directly to the C. sorokiniana cell surface (Fig. 7(a) and (d)). On the other hand, CSSB-2 and CSSB-3 tend to exist on the sheath excreted by C. sorokiniana and direct adhesion could not be observed (Fig. 7(b) and (c)). Sheath excretion of C. sorokiniana was observed in every culture although it dose not appear in appear in every photograph, e.g. Fig. 7(a).
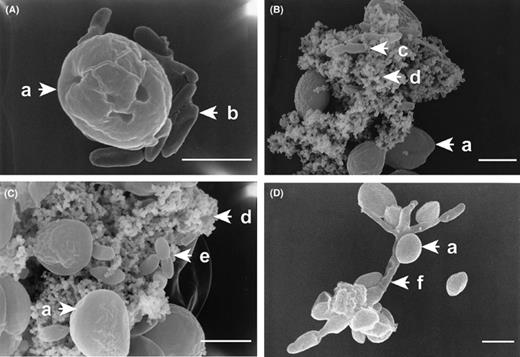
Observation of symbiotic conformation by SEM. Mixed culture of C. sorokiniana with CSSB-1 (panel A), with CSSB-2 (panel B), with CSSB-3 (panel C), with CSSF-1 (panel D). Symbols: C. sorokiniana (a), CSSB-1 (b), CSSB-2 (c), sheath (d), CSSB-3 (e) and CSSF-1 (f). The size of the white bar is 2 μm.
3.6 Long-time cultivation of consortium or purified Chlorella strain and time course of chlorophyll content
When consortium and pure cultured C. sorokiniana strain were cultivated respectively in slants for 7 months, it was observed that consortium colonies were stable even after 7 months, while pure cultured C. sorokiniana colonies had turned from dark green to yellow green (Fig. 8). The chlorophyll content was measured monthly and can be seen (Fig. 9) to decrease markedly from the 5th to the 7th month for the pure culture. As shown in Fig. 9, the chlorophyll content of the pure culture at the 7th month was half of the 3rd month.
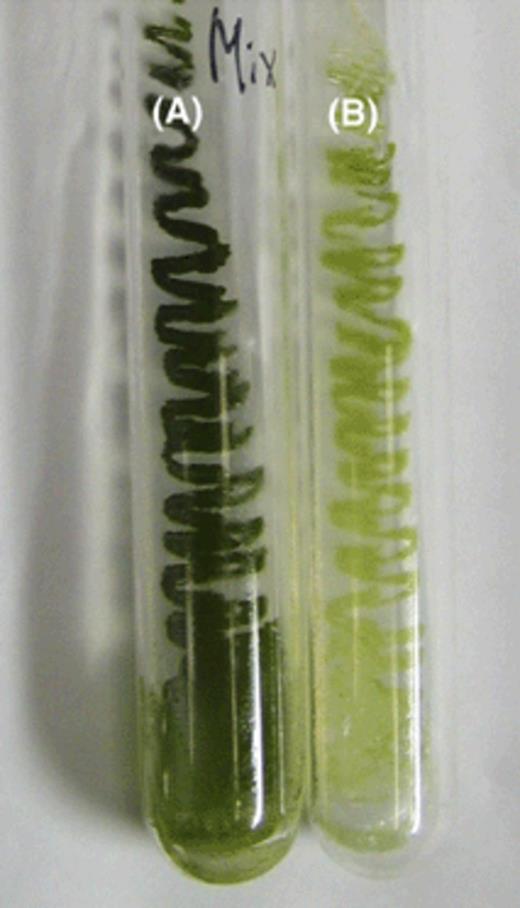
Consortium and pure culture slant of C. sorokiniana after 5 months cultivation. Consortium (A) and pure culture C. sorokiniana (B).
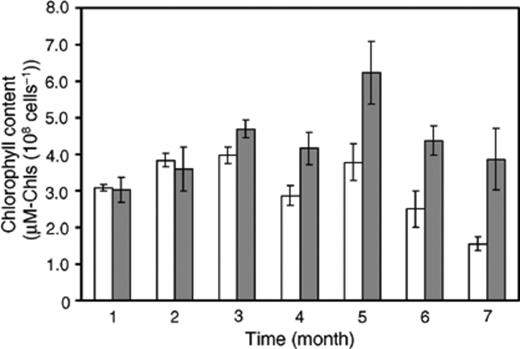
Time course of chlorophyll contents from consortium (gray bars) and pure strain of C. sorokiniana (white bars). Error bars are ±SD (n= 3).
4 Discussion
From the microbial consortium of mixed C. sorokiniana IAM C-212, four species of bacteria (CSSB-1, CSSB-2, CSSB-3 and CSSB-4) and one species of fungus (CSSF-1) were isolated under heterotrophic conditions and identified. Strain CSSB-4 could not be subcultured on the media, and it is also possible that additional heterotrophs, autotrophs, or anaerobes exist in the consortium in addition to the heterotrophs isolated here. For these reasons, the symbionts isolated in this study may represent a subset of a larger symbiotic consortium. Even if this is so, the species isolated are interesting subset. To the best of our knowledge, this is the first report on the symbiotic interaction among Chlorella, bacteria, and fungus.
The symbionts totally depend on EOC from C. sorokiniana under the photoautotrophic conditions. However, in another experiment, addition of 1% glucose into mixed cultures of C. sorokiniana promoted the growth of fungal strain CSSF-1 and inhibited the growth of C. sorokiniana significantly (data not shown). Based on these findings, it is apparent that nutritional balance is important for formation of the consortium between the Chlorella and CSSF-1. Under eutrophic conditions, the interaction between C. sorokiniana and CSSF-1 was not symbiotic but competitive.
Under the photoautotrophic condition, the growth of C. sorokiniana was not significantly affected by addition of CSSB-1 or CSSB-2. However, in mixed culture of CSSB-3 or CSSF-1, growth rate of C. sorokiniana at day 7 was significantly promoted. These results imply that CSSB-1 and CSSB-2 show commensalism with C. sorokiniana, receiving nutrients from the microalgae without affecting its growth. On the other hand, CSSB-3 and CSSF-1 exhibit mutualism, receiving nutrients from the microalgae while promoting its growth.
During long-term cultivation, the consortium was stable and maintained a healthy dark green color even after 7 months. While the chlorophyll content of consortium was stable, over 7 months, the chlorophyll content of the pure strain of C. sorokiniana declined markedly. This phenomenon may be explained by the supplement of an unidentified growth promoting substance, the small supply of carbon dioxide from the symbionts to C. sorokiniana, or bacterial removal (consumption) of oxygen resulting in more favorable conditions[26] for the photosynthetic algae. On the other hand, it has been reported that discoloration of Chlorella can be caused by nitrogen-deficient or nitrogen limitation [27,28]. Nitrogen supply to plants and animals from bacteria as N2 fixation is a very important natural symbiotic mechanism. Various species of N2 fixing bacteria have been reported in symbiotic interaction with plants or animals (for example legume[1], grass[29], and termite [30,31]). However, the separated bacterial and fungal strains identified here, have never been reported as N2 fixing microorganisms.
Two kinds of symbiotic conformation were observed in C. sorokiniana consortium. One of them consisted of symbionts that were harbored on the sheath excreted from C. sorokiniana. This symbiotic conformation has been reported between planktonic algae and epiphytic community of the surface of macrophyte leaves[14]. A second symbiotic conformation was observed when bacterial strain CSSB-1 and fungal strain CSSF-1 directly adhered to C. sorokiniana cell surface. The adhesion of the microorganism to C. sorokiniana reduces the diffusion distance, which may allow more efficient exchange of nutrient sources.
Interaction based on direct adhesion is also known for the lichen symbiosis[4]. The symbiotic interaction between C. sorokiniana and fungal strain CSSF-1 is thus similar except that it does not form a lichenous structure. Furthermore, in general, the symbiotic relationship of lichen is irreversible, if the constituents are separated and cultured independently they cannot spontaneously recombine. However, C. sorokiniana and CSSF-1 cultures could be re-mixed after separation to reestablish symbiotic association. It seems plausible that the reversible algal–fungal symbiosis described here may be more widespread in nature than the more complicated lichenous structure.
In laboratories, bacterial contamination has often been observed in cultures of microalgae and we speculate that this might be partly associated with symbiotic association in nature.
Acknowledgements
This work was supported by the 21st Century COE Program from Ministry of Education, Culture, Sports, Science, and Technology (MEXT). We would like to thank Dr. Akira Nakagiri, Prof. James C. Ogbonna, Thomas R. Glass for technical advice, and the Institute of Applied Microbiology, University of Tokyo, for providing C. sorokiniana IAM C-212.
References