-
PDF
- Split View
-
Views
-
Cite
Cite
Sushma Gupta Sood, Chemotactic response of plant-growth-promoting bacteria towards roots of vesicular-arbuscular mycorrhizal tomato plants, FEMS Microbiology Ecology, Volume 45, Issue 3, August 2003, Pages 219–227, https://doi.org/10.1016/S0168-6496(03)00155-7
- Share Icon Share
Abstract
The chemotactic responses of the plant-growth-promoting rhizobacteria Azotobacter chroococcum and Pseudomonas fluorescens to roots of vesicular-arbuscular mycorrhizal (Glomus fasciculatum) tomato plants were determined. A significantly (P=0.05) greater number of bacterial cells of wild strains were attracted towards vesicular-arbuscular mycorrhizal tomato roots compared to non-vesicular-arbuscular mycorrhizal tomato roots. Substances exuded by roots served as chemoattractants for these bacteria. P. fluorescens was strongly attracted towards citric and malic acids, which were predominant constituents in root exudates of tomato plants. A. chroococcum showed a stronger response towards sugars than amino acids, but the response was weakest towards organic acids. The effects of temperature, pH, and soil water matric potential on bacterial chemotaxis towards roots were also investigated. In general, significantly (P=0.05) greater chemotactic responses of bacteria were observed at higher water matric potentials (0, −1, and −5 kPa), slightly acidic to neutral pH (6, 6.5 and 7), and at 20–30°C (depending on the bacterium) than in other environmental conditions. It is suggested that chemotaxis of P. fluorescens and A. chroococcum towards roots and their exudates is one of the several steps in the interaction process between bacteria and vesicular-arbuscular mycorrhizal roots.
1 Introduction
The success of plant growth promotion by microorganisms depends largely on their timely response to chemical stimuli released by plants, their competition or interaction with other microorganisms, and persistence throughout the growing season. Mycorrhizal fungi are ubiquitous soil inhabitants, and form a symbiotic relationship with roots of most terrestrial plants. The largest group, which is predominantly associated with about 80% of all plant species, consists of vesicular-arbuscular mycorrhizal (VAM) fungi [1]. These AM fungi play important roles in key rhizosphere processes, including plant protection against soil-borne diseases [2–5], nutrient cycling [6], conservation of soil structure [7], and enhancement of nitrogen fixation from rhizobia [8]. In many of these key processes, VAM fungi interact synergistically with other microorganisms such as nitrogen-fixing bacteria [9–12], phosphate-solubilizing bacteria [13–15], biocontrol agents [2–5], and plant-growth-promoting microorganisms [16] to enhance plant growth and survival. AM fungi interact with these bacteria directly by providing niche and/or habitat [17–19] or indirectly by modifying host physiology [20]. Several investigations have indicated that the rhizosphere bacteria also have a strong impact on growth of VAM fungi [21]. The plant-growth-promoting rhizobacteria have been described as facilitating colonization of the plants by AM fungi, improving the development of the mycosymbiont, and reducing damage caused by soil-borne plant pathogens. Paenibacillus strain from the mycorrhizosphere of Glomus mosseae stimulated AM colonization of plants by antagonizing soil-borne plant pathogenic fungi [3]. Bacteria related to the genus Burkholderia were found endosymbiotically within spores of Gigaspora spp., where they possibly support fungal development by means of nitrogen fixation or other factors [17].
In some of these direct and indirect interactions with involvement of motile bacteria, chemotaxis may provide a significant advantage. Chemotaxis is suggested to be the first step of bacterial colonization of roots of several plant species [22–24]. Furthermore, chemotaxis and motility have been found to play a number of important roles in the symbiotic interactions of rhizobia with their hosts [25]. Motility and chemotaxis are also key characteristics of plant-growth-promoting rhizobacteria and could thus play an important role in the interaction process, yet the role of motility and chemotaxis in plant—microbe interaction is poorly understood. Recently, chemotactic responses of plant-growth-promoting bacteria and endophytic bacteria towards root exudates from rice have been reported [26]. However, there is no report on the direct and indirect influence of VAM fungi on the chemotactic response of bacteria towards roots.
This study was conducted to determine whether or not (1) plant-growth-promoting bacteria (Azotobacter chroococcum and Pseudomonas fluorescens) exhibit chemotaxis towards tomato plants colonized by Glomus fasciculatum, (2) G. fasciculatum plays a role in the communication between roots of tomato plants and plant-growth-promoting bacteria by altering amount and/or characteristics of root exudates and (3) abiotic factors (temperature, pH, and soil water matric potential) affect the chemotactic response of plant-growth-promoting bacteria towards VAM and non-VAM tomato roots.
2 Materials and methods
2.1 Bacterial strains
Twelve of the most abundant bacteria isolated from the rhizosphere and rhizoplane of tomato plants were tested for their plant-growth-promoting ability and chemotactic response towards tomato root exudates in vitro. Two motile and chemotactic isolates with the most efficient plant growth promotion activity were selected for the study. Selected isolates were identified based on fatty acid methyl ester analysis of total cellular fatty acids using gas chromatography and the MIDI system (Microbial Identification System, Newark, NJ, USA) [27]. P. fluorescens 1013 and A. chroococcum X-50 were maintained on King's B medium [28] and nutrient medium (glucose 20 g, K2HPO4 0.8 g, KH2PO4 0.29 g, MgSO4 0.5 g, FeCl3 0.1 g, CaCl2 20 g, Na2MnO4·2H2O 0.05 g, agar 20 g l−1; pH 7.4–7.6), respectively.
2.2 Soil and tomato seedlings
The sandy loam soil (pH 7, 2.3% organic matter, 73.5% sand, 14.6% silt, and 11.9% clay w/w, 2 μg g−1 NO3− N, 12 μg g−1 NH4 N, 8 μg g−1 P) was collected from the field of the Central Mining Research Institute. The soil was sieved through 2-mm mesh and steam-sterilized for two consecutive days.
Tomato seeds (Lycopersicon esculentum Mill.) were surface-disinfected in 70% ethanol for 20 min, then in 20% (v/v) bleach for 35 min, followed by five washes with sterile distilled water. The seeds (five per treatment) were then aseptically transferred to clay pots containing 250 g of sterilized sandy loam field soil. For VAM plants, 0.5 g pot culture inoculum of G. fasciculatum (isolated from tomato rhizosphere soil and maintained on tomato plants containing 300 MPN (most probable number)) was layered just below the seed. The pots were incubated in a growth chamber with a light–dark cycle consisting of 16 h of light (21°C) and 8 h of darkness (15°C). The chambers were maintained at a relative humidity of 50% and a photon flux density of 550–650 mmol m−2 s−1. After 4 weeks the seedlings were harvested and washed with sterile distilled water. Mycorrhizal infection was assessed by randomly sampling the roots and by staining the sample in chlorazol black E [29]. Assessment of VAM colonization was made by a gridline method of McGonigle et al. [30].
2.3 Root exudates
Root exudates from tomato seedlings were acquired as described by Graham et al. [31]. Three-week-old mycorrhizal and non-mycorrhizal tomato seedlings were removed from the pots and carefully washed to be free of soil. Ten plants were immediately placed in a beaker (500 ml) with the roots submerged in 200 ml of aerated 0.5 mM CaCl2 solution containing 0.05 g l−1 rifampicin and 0.025 g l−1 tetracycline and incubated for 2 h to reduce bacterial contamination. Bacterial contamination was monitored by periodically sampling the solution during exudate collection and plating the sample dilutions in medium containing 10 g casein, 5 g yeast extract, 4 g K2HPO4, and 15 g of agar (Difco) in 1 l water. After antibiotic pretreatment, the roots were rinsed with 500 ml of sterile 0.05 mM CaCl2 solution and immediately submerged in 200 ml of 0.5 mM CaCl2 solution (sterile and aerated) for 22 h in the dark at 25°C. Throughout the process, the beakers were aerated by bubbling fresh air through perforated tubes. The plants were then removed from the beaker and the dry weight of roots was determined. The exudates were immediately passed through a 0.22-μm Millipore filter and stored at 4°C or at −20°C.
2.4 Analysis of root exudates
Root exudates were analyzed to determine the amino acids, organic acids and reducing sugars. Sugars and organic acids in root exudates were determined by high-performance liquid chromatography (Waters, Bedford, MA, USA). Sugars were separated on reverse-phase Guard Pack and Sugar Pack I using distilled water flowing at a rate of 0.5 ml min−1 at 90 °C as solvent, and detected with a refractive index detector (Waters 410). Organic acids were measured by reverse-phase ion suppression on a C18 column with 0.05 M KH2PO4 buffer (pH 2.5). Amino acids were analyzed on a cation exchange column (Biotronik LC-7000, Frankfurt/Main, Germany), using a sodium citrate buffer system as eluent.
2.5 Chemotaxis
2.5.1 Bacterial strains for chemotaxis assays
Rifampicin-resistant wild-type (chemotactic and motile) A. chroococcum and P. fluorescens were selected by incubating them on appropriate agar media supplemented with 100 μg ml−1 rifampicin. Tn5-induced non-motile and non-chemotactic mutants of A. chroococcum and P. fluorescens were compared. To obtain the mutants, bacteria were surface-mated with Escherichia coli WA 803 containing the transmissible plasmid pCU1::Tn5 (Apr, Kmr, Smr, Spr, tra+) [32]. Transcojugants were retrieved in appropriate media containing 100 μg rifampicin and 100 μg kanamycin ml−1 and screened for negative motility and chemotactic attraction in semisolid agar swarm plates.
For chemotactic assays, rifampicin-resistant wild-type bacteria and their mutants were grown in 20 ml of their respective liquid medium for 16 h (stationary phase: OD550 1.0–1.2) at 25±2°C on a rotary shaker (125 rpm). To obtain the exponential growth phase of bacteria (OD550 0.15–0.25), a 1-ml aliquot of 16-h-old culture was transferred to 10 ml of respective liquid medium containing 100 μg ml−1 rifampicin and incubated for approximately 1–3 h. Cells were centrifuged (10 000×g at 4°C) and the pellets were washed twice with cold (4°C) 50 mM phosphate buffer (pH 6.8). Cells were resuspended in 10 mM potassium phosphate buffer containing 10 M Na2EDTA and the cell density was adjusted to approximately 1×107 to 1×108 (OD550 0.06–0.12) using a colorimeter (Bausch and Lomb ‘Spectronic’ 20, USA). Before each experiment, the motility of the bacterial cells was checked with a phase contrast microscope (Nikon ‘Optiphot’, Japan).
2.5.2 Chemotaxis towards root exudates
Chemotaxis experiments were done by a capillary method [33,34]. A glass tube of 0.5 cm internal diameter (i.d.) and 2 cm long was cemented near one end, parallel to the long axis of a microscopic glass slide (7.5×2.5 cm). Capillary micropipets (1 μl; 0.2 mm i.d.×3 cm; Dade Division, American Hospital Supply Corporation, USA) were sealed at one end and filled with root exudates (0.05, 0.1, 0.5, 1.0, 1.5, 2.0 and 2.5 mg ml−1) or buffer (pH 6.8; control). The open end of the capillary micropipet was inserted into a glass chamber containing 0.2 ml of bacterial suspension (1×107 cells ml−1). After 30 min of incubation at 25±2°C, the capillary tube was removed and washed exteriorly by a jet of sterile distilled water. The tube was crushed in 10 ml of sterilized 0.9% NaCl solution. This was diluted and 0.2 ml of suspension (10−2 and 10−3 dilutions) was spread over Petri dishes (three dishes per treatment) containing appropriate agar medium supplemented with rifampicin (100 μg ml−1). Plates were incubated at 25±2°C and bacterial colonies were counted after 3–4 days.
2.5.3 Chemotaxis towards carbon substances of root exudates
The chemotactic response of bacteria towards specific amino acids, organic acids, and sugars present in root exudates was determined by the same method as for in vitro studies. Solutions of amino acids, sugars and organic acids were made with 10 mM potassium phosphate buffer. Concentrations of these compounds were made similar to those in root exudates of VAM tomato seedlings. Capillaries filled with these solutions were placed in glass chambers containing bacterial suspension and incubated for 1 h. The results are expressed as a relative chemotactic response, i.e. the ratio of the number of bacterial cells attracted in capillaries containing attractant to the number of cells in capillaries containing potassium phosphate buffer (10 mM; pH 6.8; non-attractant).
2.5.4 Chemotaxis in soil towards roots
Chemotactic responses of bacteria towards roots of VAM and non-VAM tomato seedlings were determined at different distances (10, 15, and 20 mm) at different soil water matric potentials (0, −1, −5, −10, −15, and −20 kPa). We also determined effects of temperature (15, 20, 25, 30, 35 and 40°C) and pH (4, 5, 6, 7, 8, and 9) on chemotactic responses of bacteria towards roots of VAM tomato seedling when seedlings were placed at 10 mm distance and at 0 kPa ψm. The soil matric potential (ψm) was controlled using a tensiometer [35,36] consisting of a 9-cm-diameter Büchner funnel with a sintered glass plate of fine porosity (4.5 μm). A rubber stopper was placed at the stem of each funnel through which a glass tube (7 cm long; 0.5 cm i.d.) was passed. A water column was supported within 0.5 cm i.d. plastic tubing attached at the end of the glass tube. The soil suction could be set at the desired matric potential by adjusting the length of the water column (1.002 cm high; 1 kPa=10 m bar). Fifty grams of unsterilized soil held at 15% moisture (w/w) was placed in a Büchner funnel. A column of 100 cm was maintained for 48 h and then the height of tension plates was adjusted to obtain the desired soil matric potential (Fig. 1).
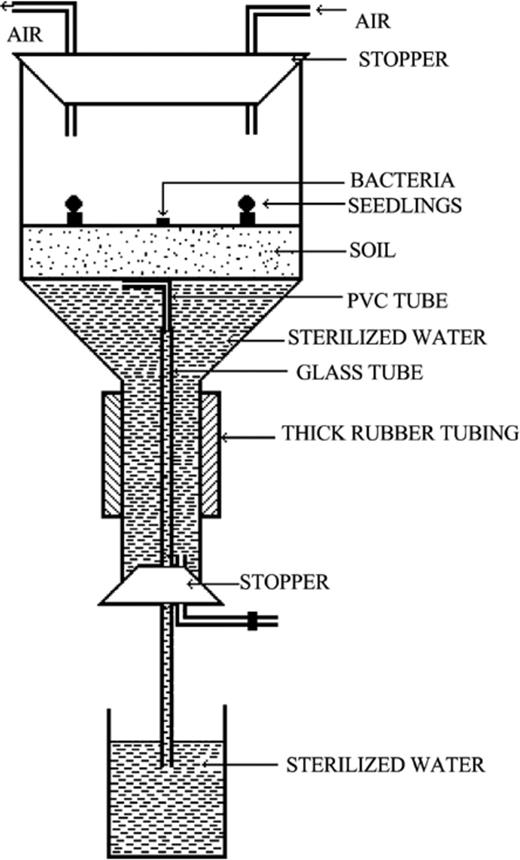
Apparatus used to study bacterial chemotaxis towards roots of tomato seedlings in soil.
Three-week-old VAM or non-VAM (control) tomato seedlings (four seedlings per funnel) were placed in the soil held in Büchner funnels. Seedlings were arranged in a circle at 10, 15, or 20 mm from the bacteria and were equilibrated for 24 h in the soil held at different soil water matric potentials. After 24 h, 330 μl (approx. 1–1.5×107 cells) cell suspension of rifampicin-resistant bacteria or their non-motile and non-chemotactic mutants was placed in the center of the funnel over the soil surface with a micropipet. Care was taken that the bacterial cells did not splash or run across the soil surface. All the experiments were run in triplicate. After 1 h incubation, the seedlings were removed from the soil and roots were washed in a sterilized glass test tube with 10 ml of 0.9% (w/v) NaCl solution. This was diluted and 0.2-ml aliquots of 10−3 and 10−4 dilutions were spread over the Petri dishes containing appropriate agar media supplemented with 100 μg ml−1 rifampicin. Colonies were counted after 3–4 days of incubation at 25±2°C. Different soil temperatures were maintained by an electric heating mantle fitted around the Büchner funnel. The heating mantle was connected to a capillary thermostat to regulate temperature. To determine the effect of pH the soil pH was adjusted using weak solutions (2%) of either NaOH or H2SO4[37]. Similar solutions were also added into soil water supply.
2.6 Statistical analysis
All experiments were performed in triplicate and repeated twice. Data were subjected to analysis of variance or to Student's t-test. A difference between means was distinguished by Duncan's new multiple-range test.
3 Results
3.1 Bacterial strains
Both nitrogen-fixing A. chroococcum and phosphate-solubilizing P. fluorescens isolated from the tomato rhizospheres were the most efficient plant-growth-promoting bacteria among all the isolated motile and chemotactic bacteria. Treatment of the seeds with these two bacteria resulted in significantly higher (P=0.05) root and shoot dry weight of tomato plants than other isolates (data not shown).
3.2 Tomato seedling
Colonization of roots of tomato seedlings by G. fasciculatum was 71% after 3 weeks.
3.3 Analysis of root exudates
Amount and composition of sugars, amino acids and organic acids in exudates from VAM tomato roots were significantly (P=0.05) different from those from non-VAM roots (Table 1). Root exudates of VAM tomato plants had higher concentrations of total amino acids, organic acids and sugars. Cysteine, glutamic acid, isoleucine, leucine, and threonine in exudates from non-VAM roots were found in very small quantities. Proline was not identified in the root exudates from VAM tomato seedlings (Table 1). A difference in concentrations of histidine, lysine, phenylalanine, and fumaric acid in root exudates from VAM and non-VAM tomato plants was not significant (P=0.05) (Table 1).
Relative chemotactic response of bacteria towards amino acids, sugars, and organic acids present in root exudates of VAM tomato seedlings
Compound | Concentration (μg ml−1) | Relative chemotactic response of bacteriaa | ||
VAM | non-VAM | Ac | Pf | |
Amino acids | ||||
Alanine | 2.6 | 1 | 44±2 | 59±4 |
Arginine | 4.1 | 2.5 | – | 76±2 |
Aspartic acid | 10.5 | 3.9 | – | 29±2 |
Cysteine | 3.8 | 0.2 | – | 23±1 |
Glutamic acid | 6.8 | 0.7 | 40±1 | 15±1 |
Glycine | 3.6 | 2.4 | 59±3 | 36±2 |
Histidine | 2.2b | 1.8b | 45±2 | 52±3 |
Isoleucine | 1.3 | 0.5 | 51±2 | 57±3 |
Leucine | 2 | 0.3 | 43±2 | 15±2 |
Lysine | 0 | 1.8 | 40±2 | – |
Phenylalanine | 1b | 1.2b | 22±1 | 29±4 |
Proline | 0 | 2.8 | 44±2 | – |
Serine | 0 | 8.8 | 46±4 | 50±2 |
Threonine | 0 | 0.8 | – | |
TOTAL | 51.5 | 30.6 | ||
Sugars | ||||
Fructose | 16.9 | 10.7 | 39±2 | 18±4 |
Glucose | 53.7 | 42.8 | 54±3 | 29±5 |
Sucrose | 11 | 15.2 | 26±2 | – |
TOTAL | 115.4 | 94.9 | ||
Organic acids | ||||
Citric acid | 3.8 | 1 | 8±2 | 76±3 |
Fumaric acid | 2.5b | 2b | 19±6 | 29±3 |
Malic acid | 4.2 | 1.5 | – | 94±4 |
Succinic acid | 2.9 | 1.2 | 6±2 | 56±4 |
TOTAL | 26.1 | 12.8 |
Compound | Concentration (μg ml−1) | Relative chemotactic response of bacteriaa | ||
VAM | non-VAM | Ac | Pf | |
Amino acids | ||||
Alanine | 2.6 | 1 | 44±2 | 59±4 |
Arginine | 4.1 | 2.5 | – | 76±2 |
Aspartic acid | 10.5 | 3.9 | – | 29±2 |
Cysteine | 3.8 | 0.2 | – | 23±1 |
Glutamic acid | 6.8 | 0.7 | 40±1 | 15±1 |
Glycine | 3.6 | 2.4 | 59±3 | 36±2 |
Histidine | 2.2b | 1.8b | 45±2 | 52±3 |
Isoleucine | 1.3 | 0.5 | 51±2 | 57±3 |
Leucine | 2 | 0.3 | 43±2 | 15±2 |
Lysine | 0 | 1.8 | 40±2 | – |
Phenylalanine | 1b | 1.2b | 22±1 | 29±4 |
Proline | 0 | 2.8 | 44±2 | – |
Serine | 0 | 8.8 | 46±4 | 50±2 |
Threonine | 0 | 0.8 | – | |
TOTAL | 51.5 | 30.6 | ||
Sugars | ||||
Fructose | 16.9 | 10.7 | 39±2 | 18±4 |
Glucose | 53.7 | 42.8 | 54±3 | 29±5 |
Sucrose | 11 | 15.2 | 26±2 | – |
TOTAL | 115.4 | 94.9 | ||
Organic acids | ||||
Citric acid | 3.8 | 1 | 8±2 | 76±3 |
Fumaric acid | 2.5b | 2b | 19±6 | 29±3 |
Malic acid | 4.2 | 1.5 | – | 94±4 |
Succinic acid | 2.9 | 1.2 | 6±2 | 56±4 |
TOTAL | 26.1 | 12.8 |
Ac: A. chroococcum; Pf: P. fluorescens. Values are means of three replicates.
aRelative chemotactic response is the ratio of the number of bacteria in capillaries containing attractant to the number of bacteria in capillaries containing phosphate buffer.
bValues are not significantly different (P=0.05) by Duncan's multiple range test.
Relative chemotactic response of bacteria towards amino acids, sugars, and organic acids present in root exudates of VAM tomato seedlings
Compound | Concentration (μg ml−1) | Relative chemotactic response of bacteriaa | ||
VAM | non-VAM | Ac | Pf | |
Amino acids | ||||
Alanine | 2.6 | 1 | 44±2 | 59±4 |
Arginine | 4.1 | 2.5 | – | 76±2 |
Aspartic acid | 10.5 | 3.9 | – | 29±2 |
Cysteine | 3.8 | 0.2 | – | 23±1 |
Glutamic acid | 6.8 | 0.7 | 40±1 | 15±1 |
Glycine | 3.6 | 2.4 | 59±3 | 36±2 |
Histidine | 2.2b | 1.8b | 45±2 | 52±3 |
Isoleucine | 1.3 | 0.5 | 51±2 | 57±3 |
Leucine | 2 | 0.3 | 43±2 | 15±2 |
Lysine | 0 | 1.8 | 40±2 | – |
Phenylalanine | 1b | 1.2b | 22±1 | 29±4 |
Proline | 0 | 2.8 | 44±2 | – |
Serine | 0 | 8.8 | 46±4 | 50±2 |
Threonine | 0 | 0.8 | – | |
TOTAL | 51.5 | 30.6 | ||
Sugars | ||||
Fructose | 16.9 | 10.7 | 39±2 | 18±4 |
Glucose | 53.7 | 42.8 | 54±3 | 29±5 |
Sucrose | 11 | 15.2 | 26±2 | – |
TOTAL | 115.4 | 94.9 | ||
Organic acids | ||||
Citric acid | 3.8 | 1 | 8±2 | 76±3 |
Fumaric acid | 2.5b | 2b | 19±6 | 29±3 |
Malic acid | 4.2 | 1.5 | – | 94±4 |
Succinic acid | 2.9 | 1.2 | 6±2 | 56±4 |
TOTAL | 26.1 | 12.8 |
Compound | Concentration (μg ml−1) | Relative chemotactic response of bacteriaa | ||
VAM | non-VAM | Ac | Pf | |
Amino acids | ||||
Alanine | 2.6 | 1 | 44±2 | 59±4 |
Arginine | 4.1 | 2.5 | – | 76±2 |
Aspartic acid | 10.5 | 3.9 | – | 29±2 |
Cysteine | 3.8 | 0.2 | – | 23±1 |
Glutamic acid | 6.8 | 0.7 | 40±1 | 15±1 |
Glycine | 3.6 | 2.4 | 59±3 | 36±2 |
Histidine | 2.2b | 1.8b | 45±2 | 52±3 |
Isoleucine | 1.3 | 0.5 | 51±2 | 57±3 |
Leucine | 2 | 0.3 | 43±2 | 15±2 |
Lysine | 0 | 1.8 | 40±2 | – |
Phenylalanine | 1b | 1.2b | 22±1 | 29±4 |
Proline | 0 | 2.8 | 44±2 | – |
Serine | 0 | 8.8 | 46±4 | 50±2 |
Threonine | 0 | 0.8 | – | |
TOTAL | 51.5 | 30.6 | ||
Sugars | ||||
Fructose | 16.9 | 10.7 | 39±2 | 18±4 |
Glucose | 53.7 | 42.8 | 54±3 | 29±5 |
Sucrose | 11 | 15.2 | 26±2 | – |
TOTAL | 115.4 | 94.9 | ||
Organic acids | ||||
Citric acid | 3.8 | 1 | 8±2 | 76±3 |
Fumaric acid | 2.5b | 2b | 19±6 | 29±3 |
Malic acid | 4.2 | 1.5 | – | 94±4 |
Succinic acid | 2.9 | 1.2 | 6±2 | 56±4 |
TOTAL | 26.1 | 12.8 |
Ac: A. chroococcum; Pf: P. fluorescens. Values are means of three replicates.
aRelative chemotactic response is the ratio of the number of bacteria in capillaries containing attractant to the number of bacteria in capillaries containing phosphate buffer.
bValues are not significantly different (P=0.05) by Duncan's multiple range test.
3.4 Chemotaxis towards root exudates
The chemotactic response of A. chroococcum and P. fluorescens to exudates of VAM tomato roots was significantly stronger (P=0.05; 2.2–12-fold) than the response to non-VAM roots and increased with an increase in exudate concentration (Fig. 2).
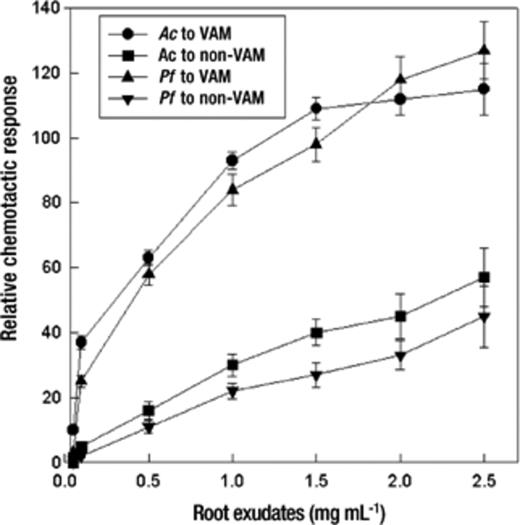
Relative chemotactic responses of bacteria to varying concentrations of root exudates (rehydrated after freezing in 10 mM phosphate buffer, pH 6.8). Mean values±S.E.M. from five replicates are indicated. Relative chemotactic response is the ratio of colony-forming units of the treatment to that of the control (capillaries were filled with 10 mM phosphate buffer). The assay time was 60 min. Ac: A. chroococcum, Pf: P. fluorescens.
3.5 Chemotaxis towards carbon substances of root exudates
Bacteria showed positive chemotaxis towards some specific carbon substances in root exudates (Table 1). Apart from arginine, aspartic acid, cysteine, threonine and malic acid, all amino acids, sugars, and organic acids in root exudates were able to attract a significant number (P=0.05) of A. chroococcum cells in capillaries. P. fluorescens showed no significant response (P=0.05) to lysine, proline, and sucrose. Sugars stimulated a strong response in A. chroococcum, but a poor response in P. fluorescens. In contrast to A. chroococcum, P. fluorescens exhibited strong chemotaxis towards organic acids (Table 1).
3.6 Chemotaxis in soil towards roots
Rifampicin-resistant wild-type A. chroococcum (Fig. 3a) and P. fluorescens (Fig. 3b) exhibited positive chemotaxis towards roots of VAM and non-VAM tomato plants. However, their chemotactic response was significantly stronger (P=0.05, 2.2–5-fold) towards roots of VAM tomato plants than to non-VAM tomato plants. The non-motile non-chemotactic mutants of A. chroococcum and P. fluorescens showed no significant response (P=0.05) towards roots of tomato plants.
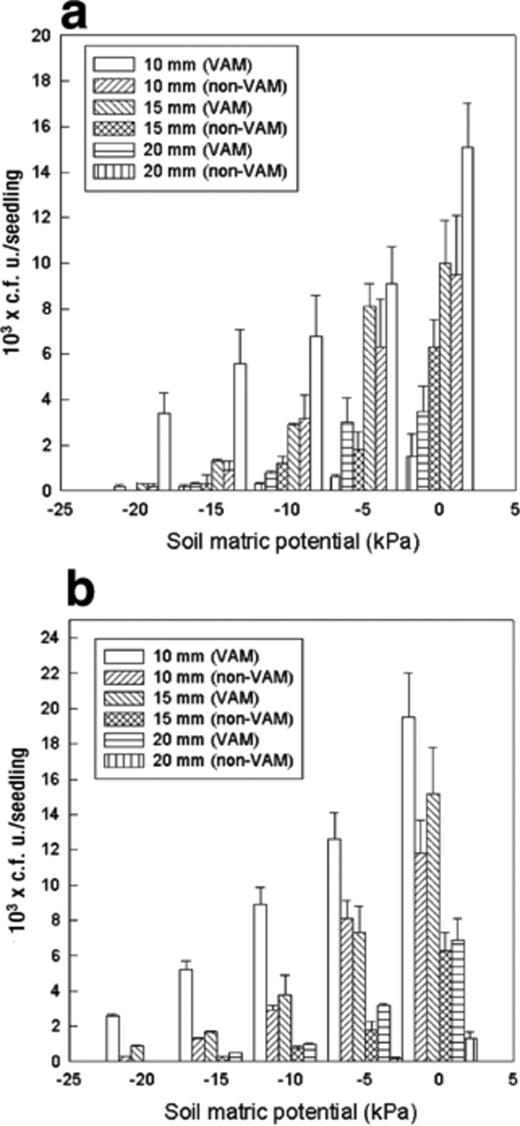
Chemotactic response of (a) A. chroococcum and (b) P. fluorescens towards VAM and non-VAM roots when kept at different distances from tomato seedlings at different soil matric potentials. Mean values±S.E.M. from five replicates are indicated. The assay time was 60 min. Ac: A. chroococcum, Pf: P. fluorescens.
Different abiotic factors significantly influenced the response. Soil water matric potential (ψm) played a major role in bacterial chemotaxis towards root exudates. A greater response was observed at 0 kPa. The chemotactic response of bacteria towards the roots of VAM tomato plants was 1.6–2.3-fold greater than the response towards non-VAM tomato plants at 0 kPa. P. fluorescens exhibited significantly greater (P=0.05) chemotactic response than A. chroococcum towards roots with VAM association at all soil matric potentials. No significant difference (P=0.05) between the response of A. chroococcum and P. fluorescens was observed towards the roots without VAM association at 0 and −20 kPa soil matric potentials (Fig. 3a,b). In general, VAM roots were able to attract significantly more (P=0.05) bacteria from a longer distance and in drier conditions. VAM roots attracted 0.9×103P. fluorescens cells from 15 mm distance at −20 kPa ψm, whereas no significant accumulation of P. fluorescens was observed on the roots of non-VAM tomato seedlings.
Chemotaxis was also influenced by soil temperature and hydrogen ion concentration. It increased with an increase in temperature until 25–30°C and decreased with further increases in temperature (Fig. 4). A. chroococcum exhibited greatest chemotaxis at 25 °C. However, P. fluorescens showed a greater response at 30 °C. At 30, 35, and 40°C P. fluorescens showed approximately 1.4-, 1.8-, and 8-fold greater responses, respectively, than A. chroococcum. Similarly, the chemotactic response of A. chroococcum was significantly greater (P=0.05) than that of P. fluorescens at 20 and 25°C (Fig. 4). A greater chemotactic response of both bacteria was observed at pH 7 (Fig. 4). The response was decreased with an increase or a decrease in (neutral) pH. The response of A. chroococcum decreased sharply (5.3-fold) at pH 8.0. A. chroococcum showed a greater response than P. fluorescens at slightly acidic pH (i.e. pH 5 and 6). However, P. fluorescens exhibited a greater response at an alkaline pH (Fig. 5).
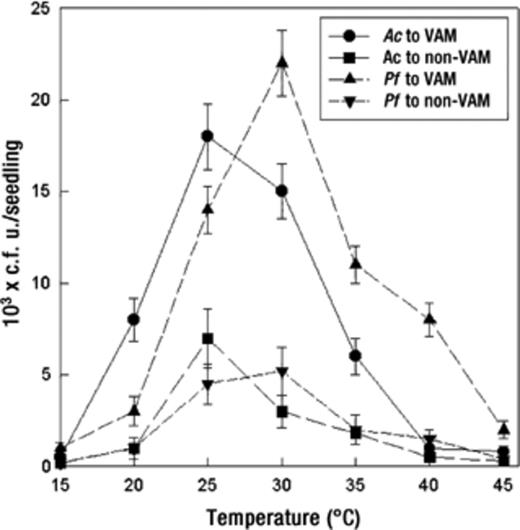
Effect of temperature on bacterial chemotactic response towards VAM and non-VAM roots of tomato seedlings. Mean values±S.E.M. from five replicates are indicated. Assays were done for 60 min. Ac: A. chroococcum, Pf: P. fluorescens.
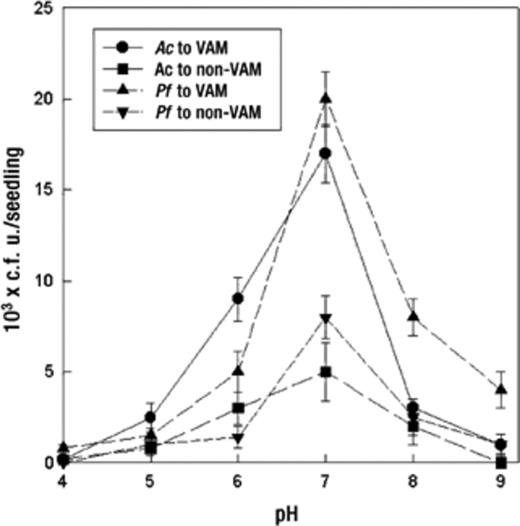
Effect of pH on bacterial chemotactic response towards VAM and non-VAM roots of mycorrhizal tomato seedlings. Mean values±S.E.M. from five replicates are indicated. Assays were done for 60 min. Ac: A. chroococcum, Pf: P. fluorescens.
4 Discussion
This study clearly demonstrates that communication exists between motile bacteria and arbuscular mycorrhizal roots in soil. Positive interactions of plant-growth-promoting rhizobacteria and VAM fungi have been reported previously [38]. It appears that translocation and exudation of metabolites from the VAM roots diffusing outward attracts bacteria. It is also evident from the results that bacteria can detect changes in attractant concentrations as they exhibited different chemotactic responses to different concentrations of root exudates (Fig. 2). The increased response of bacteria with an increase in exudate concentration suggests that this may enable bacteria to detect a concentration gradient of attractant and to reach the immediate vicinity of the roots. The quantity and quality of the root exudates from VAM and non-VAM tomato plants is varied (Table 1). Root exudates from VAM tomato plants contained higher amounts of amino acids, organic acids, and sugars than non-VAM plants (Table 1), confirming earlier findings [5,39]. It is suggested that the formation of VAM might result in changes in plant metabolism [40]. Rambelli [20] and Linderman [41] further point out that mycorrhizal infection alters the physiology of plants by increasing photosynthetic rates, changing the position of photosynthates in shoots and roots and changing the mineral uptake from soil, which consequently changes the nutritional composition of host tissues. This change in tissues in turn brings structural and biochemical changes in root cells and alters membrane permeability, thus, the quality and quantity of root exudates.
Amino acids and organic acid fractions of root exudates were important for P. fluorescens chemotaxis. Similarly, amino acids and sugar fractions were important for enhanced chemotactic response of A. chroococcum (Table 1). The enhanced chemotaxis of P. fluorescens towards amino acids and not to sugars was consistent with the findings of other studies [42–44]. However, in this study, organic acids were stronger attractants to P. fluorescens than amino acids. A stronger chemotactic response towards specific compounds may indicate a greater importance of one type of sensory receptors over another [45]. Specific chemotaxis to an attractant present in the root exudate might provide a competitive and selective advantage in the interaction process. It has been shown that arbuscular mycorrhizal roots influence the species composition of the soil microbial community by stimulating some groups and decreasing another [11,46]. It has also been suggested that certain plant-growth-promoting rhizobacteria are plant-specific [47].
The bacterial chemotaxis towards VAM roots can be affected by several environmental factors such as soil water matric potential, temperature, and pH. One of the most important factors affecting the chemotaxis is soil water matric potential and it may affect either bacterial movement or flow of root exudates, or both. Soils held at 0, −1 and −5 kPa were favorable for chemotaxis of bacteria towards VAM roots. Most likely, this is due to the availability of free water for flagellar motility and a greater rate of diffusion of root exudates in water-filled soil pores. The presence of VAM fungi in soil (−0.49 to −3.92 kPa) has been reported to increase small pores [48]. The significantly greater (P=0.05) chemotaxis towards VAM roots than non-VAM roots in soils held at −15 and −20 kPa matric potentials suggests that VAM fungi play a role in bacterial movement in soil, through hyphae serving as migration pathways and probably increasing bacterial mobility along the growing roots [49]. It was also observed that in the drier soil (−15 and −20 kPa), VAM hyphal length density was significantly (P=0.05) higher than in wet soil (0, −5 and −10 kPa) (data not shown). Movement of bacteria was restricted to only 10 mm towards non-VAM roots (Fig. 3a,b). This movement in soil is expected to be limited to distances of only a few centimeters because the soil matrix acts as a filter for suspended particles [50,51]. The greater response of bacteria towards roots of VAM tomato plants 15 and 20 mm away in soils held at 0 and −5 kPa than at −10, −15 and −20 kPa might be the result of a passive movement in water-filled pores (Fig. 2a,b) [52–54].
Temperature and pH both influenced chemotaxis of the bacteria towards VAM roots of tomato plants (Figs. 4 and 5). The reason for this is not understood because temperature and pH affect so many other soil factors. Thus, it is often difficult to determine the effects that changes in pH and temperature will have on chemotaxis. However, these factors have a great impact on the swimming speed and tumbling frequency of bacterial cells [35,55], and the amount of exudation and diffusion of exudates from roots of tomato seedlings. Hayman [56] reported that the effect of temperature on plant growth of onion was more pronounced than mycorrhizal infection.
The enhanced chemotactic response of P. fluorescens and A. chroococcum towards VAM tomato roots suggests that G. fasciculatum may play an important role in attracting specific bacteria. On the other hand chemotactic responses of these bacteria may play roles in recognizing their host and in their relationship with VAM fungi. Further studies are needed to evaluate the role of chemotaxis in the interaction of VAM fungi and other beneficial soil bacteria.
Acknowledgements
I am grateful to Dr. Mary C. Curran for critical reading of the manuscript.
References