-
PDF
- Split View
-
Views
-
Cite
Cite
Kevin J. Purdy, Mark A. Munson, David B. Nedwell, T. Martin Embley, Comparison of the molecular diversity of the methanogenic community at the brackish and marine ends of a UK estuary, FEMS Microbiology Ecology, Volume 39, Issue 1, January 2002, Pages 17–21, https://doi.org/10.1111/j.1574-6941.2002.tb00902.x
- Share Icon Share
Abstract
The 16S rRNA sequence diversity of the euryarchaeal community in a predominately freshwater sediment at East Hill Bridge (EHB) on the River Colne estuary, Essex, UK was investigated and compared to that from marine sediments at the mouth of the river (Colne Point). The East Hill Bridge sediments appear to support the full range of methanogen phenotypes with some genotypes similar to those previously detected at Colne Point. However, no Marine Benthic Group D or halophilic archaeal genotypes, both abundant in gene libraries at Colne Point, were detected at East Hill Bridge. Clones related to Methanosarcina and Methanocorpusculum were detected only at East Hill Bridge while clones closely related to Methanoculleus and Methanococcoides were detected only at Colne Point. The most common clones in the East Hill Bridge library were closely related to the obligate acetate-utilising Methanosaeta concilii, suggesting they may be important methanogens in these sediments. Clones that group closely with M. concilii appear to be ubiquitous in freshwater sediments and we suggest that they are prime candidates for a globally important acetoclastic methanogenic group. The distribution of clones in the East Hill Bridge and Colne Point libraries implies that certain methanogen groups are generalists, adapted to the range of conditions within an estuarine environment (e.g. Methanogenium) while others are more specialist (e.g. Methanosaeta).
1 Introduction
Marine and freshwater anaerobic sediments are important sites for biological carbon cycling [1], but relatively little is known about the identities of the organisms responsible for key terminal processes such as methanogenesis and sulphate reduction. This is due in part to the difficulties of culturing representative organisms from natural samples. Molecular methods offer an alternative approach to identifying prokaryotes in nature without the need to culture. We are currently using molecular methods to investigate the community structure of methanogenic archaea in contrasting sites along a salinity gradient in the well studied River Colne estuary, Essex, UK. Along such a gradient, anaerobic terminal organic degradation changes from being dominated by sulphate reduction at the high sulphate, marine end to being methanogenesis-dominated at the low sulphate, freshwater end [2]. Sulfate reducers can out-compete methanogens for both acetate and hydrogen [3–6], so the primary controlling factor deciding which process dominates in nature is thought to be the availability of sulphate [3,7]. However methanogenesis does occur even under sulphate-rich conditions as certain methanogens can exploit C1-compounds that sulphate reducers cannot utilise, such as methylamines [8,9].
We have previously used phylogenetic analysis of 16S rRNA sequences to identify members of the archaeal community participating in methanogenesis in tidal marine sediments at Colne Point, at the mouth of the River Colne [10]. The sequences were from clone libraries made from polymerase chain reaction (PCR) products generated using environmental DNA extracts. In agreement with published hypotheses, we found genotypes closely related to methanogens such as Methanolobus and Methanococcoides, which can utilise C1-compounds such as methylamines. However, we also detected many clone sequences which are closely related to species of methanogens that can use hydrogen for methanogenesis. Indeed representatives of many different methanogen 16S rRNA lineages were detected suggesting that, although methanogenesis is a minor process in these sediments [11], the sediments nevertheless contain a diverse methanogen community.
Here, we have extended our phylogenetic description of the methanogenic archaea in Colne Point sediments, to include occasionally brackish sediments at East Hill Bridge, at the top of the River Colne estuary. Methanogenesis at East Hill Bridge is typically much higher than in the tidal sediments, although sulphate reduction may dominate after particularly high tides when seawater can penetrate far upstream. The new 16S rRNA sequences were analysed together with sequences recovered previously from the marine sediments at Colne Point, sequences from cultured reference methanogens and published sequences from other molecular studies of anaerobic marine and freshwater systems. Our aim was to identify which methanogens are present in brackish East Hill Bridge sediments and to compare them to those found in the nearby marine sediments. Further, by integrating all of the currently available 16S rRNA data on anaerobic marine, brackish and freshwater sediments we aimed to identify any important distribution patterns of archaeal genotypes that might be emerging.
2 Materials and methods
2.1 Sample collection and analysis of sediment
Sediment cores (10 mm diameter and 10 cm in length) were taken in February 1998 at East Hill Bridge on the River Colne estuary, Colchester, Essex, UK. Cores were returned to the laboratory, the sediment washed with 120 mM sodium phosphate, pH 8.0 buffer to remove extracellular nucleic acids [12] and stored at −20°C until nucleic acid extraction.
The salinity of sediment pore water was determined using a refractive salinometer (model 10419, Reichert-Jung, Buffalo, NY, USA). Pore water sulphate concentrations were measured using ion exchange chromatography (series 2000 i, Dionex Corp., Sunnyvale, CA, USA).
To gain an appreciation of the relative importance of methanogenesis and sulphate reduction at East Hill Bridge in situ process rates were also determined in August 2000 using published methods [2].
2.2 Nucleic acid extraction
Nucleic acids were extracted from the sediments (1 g of the complete depth horizon) by the hydroxyapatite spin-column method [13]. DNA extracts were further purified on a 1.4% Tris–acetate–ethylenediaminetetraacetic acid (EDTA) agarose gel. Gel slices containing DNA fragments of >5000 bp were excised from the gel and the DNA extracted using the Spinbind gel extraction kit (Flowgen, Satffordshire, UK). The extract was then dialysed twice against TE (10 mM Tris, 1 mM EDTA, pH 8.0) using Microcon 30 filtration cartridges (Amicon Ltd., Stonehouse, Gloucestershire, UK).
2.3 PCR and cloning of environmental archaea 16S rDNA gene fragments
A hemi-nested PCR approach was used [10]. Five replicate amplifications were used and the products bulked in each round of amplification to avoid stochastic biases in individual PCR reactions [14]. The PCR product from the secondary amplification was gel purified, ligated into pGem-T easy (Promega, Madison, WI, USA) and transformed into XL1-Blue MFR′ (Stratagene, La Jolla, CA, USA) Escherichia coli cells.
2.4 Sequencing and analysis of environmental clones
182 clones were initially screened by manual A-tracking in order to identify unique environmental clones [15,16]. 72 unique clones were sequenced using the universal sequencing primer 519R [17] using an ABI automatic sequencer (Sequencing Facility, Natural History Museum, London, UK). The resulting sequences were aligned with reference taxa and available environmental clones within the genetics database environment [18] and analysed using PAUP (Version 4.01b [19]). A neighbour joining tree was generated based on about 300 nucleotides and the 72 unique clones grouped. 17 representative clones were chosen and fully sequenced using vector based sequencing primers (Sp6 and T7). An alignment of the complete sequences, reference taxa and available environmental clones gave 940 positions for analysis. Pairwise distances for all alignable sites were calculated using the Logdet/Paralinear distances method [17,20]. Logdet/Paralinear distances method assumes all sites can vary which is not true for most data sets. This was corrected for by estimating the number of variable sites in the alignment using a two state (variable–invariable) maximum likelihood model in PAUP and phylogenetic analysis was limited to only those positions (56% of sites [21,22]). Bootstrap values (1000 replicates) were determined using PAUP. The tree was rooted using Thermoplasma acidophilum as the outgroup. Clone sequences have been submitted to GenBank, accession numbers AF374274–AF374285.
3 Results and discussion
3.1 In situ process rates, sediment pore water sulphate concentrations and salinity
Salinity in the pore water of the East Hill Bridge sediments was <1‰ and pore water sulphate concentrations were <0.5 mM at the sampling time used for molecular analysis. In situ process rates measured in August indicated that methanogenesis represented approximately 6% of anaerobic terminal oxidation in East Hill Bridge sediments (Table 1). However, at the time of these measurements pore water sulphate concentration was high (3.9 mM), resulting from tidal intrusion. In comparison, at the Weir, a few 100 m upstream of East Hill Bridge, sulphate concentrations were 0.9 mM and methanogenesis represented 53% of terminal organic carbon mineralisation (Table 1). This suggested that East Hill Bridge is a dynamic environment where particularly high tides are able to significantly affect the balance between sulphate reduction and methanogenesis. In contrast, methanogenesis represented only 0.6% of terminal oxidation in the permanently high sulphate marine Colne Point sediments, indicating that under most conditions methanogenesis is more important at East Hill Bridge than at Colne Point (Table 1).
In situ process rates at East Hill Bridge (August 2000) and Colne Point (August 1995)
The Weir (freshwater) | East Hill Bridge (brackish) | Colne Point (marine) | |
Methanogenesis | |||
Rate [S.E.M.] (mmol m−2 day−1) | 2.03 [0.1, n=3] | 0.22 [0.02, n=3] | 0.29 [0.01, n=5] |
% (mol−1 carbon) | 53% | 6% | 0.6% |
Sulfate reduction | |||
Rate [S.E.M.] (mmol m−2 d−1) | 0.92 [0.1] | 1.69 [0.1] | 24.6 [3.1, n=5] |
% (mol−1 carbon) | 47% | 94% | 99.4% |
The Weir (freshwater) | East Hill Bridge (brackish) | Colne Point (marine) | |
Methanogenesis | |||
Rate [S.E.M.] (mmol m−2 day−1) | 2.03 [0.1, n=3] | 0.22 [0.02, n=3] | 0.29 [0.01, n=5] |
% (mol−1 carbon) | 53% | 6% | 0.6% |
Sulfate reduction | |||
Rate [S.E.M.] (mmol m−2 d−1) | 0.92 [0.1] | 1.69 [0.1] | 24.6 [3.1, n=5] |
% (mol−1 carbon) | 47% | 94% | 99.4% |
Standard error was estimated by taking the square of the standard deviation of the mean divided by n for each depth profile (estimated variance). These were then summed over the complete depth (0–10 cm at East Hill Bridge and the Weir and 0–20 cm at Colne Point). The square root of this sum is the estimated S.E.M. for the areal process rate (personal communication, Dr C. Moncreiff).
In situ process rates at East Hill Bridge (August 2000) and Colne Point (August 1995)
The Weir (freshwater) | East Hill Bridge (brackish) | Colne Point (marine) | |
Methanogenesis | |||
Rate [S.E.M.] (mmol m−2 day−1) | 2.03 [0.1, n=3] | 0.22 [0.02, n=3] | 0.29 [0.01, n=5] |
% (mol−1 carbon) | 53% | 6% | 0.6% |
Sulfate reduction | |||
Rate [S.E.M.] (mmol m−2 d−1) | 0.92 [0.1] | 1.69 [0.1] | 24.6 [3.1, n=5] |
% (mol−1 carbon) | 47% | 94% | 99.4% |
The Weir (freshwater) | East Hill Bridge (brackish) | Colne Point (marine) | |
Methanogenesis | |||
Rate [S.E.M.] (mmol m−2 day−1) | 2.03 [0.1, n=3] | 0.22 [0.02, n=3] | 0.29 [0.01, n=5] |
% (mol−1 carbon) | 53% | 6% | 0.6% |
Sulfate reduction | |||
Rate [S.E.M.] (mmol m−2 d−1) | 0.92 [0.1] | 1.69 [0.1] | 24.6 [3.1, n=5] |
% (mol−1 carbon) | 47% | 94% | 99.4% |
Standard error was estimated by taking the square of the standard deviation of the mean divided by n for each depth profile (estimated variance). These were then summed over the complete depth (0–10 cm at East Hill Bridge and the Weir and 0–20 cm at Colne Point). The square root of this sum is the estimated S.E.M. for the areal process rate (personal communication, Dr C. Moncreiff).
3.2 Phylogeny and diversity of East Hill Bridge methanogens
Using a 97% similarity cut-off value [23] East Hill Bridge clone library coverage was estimated at 80% indicating that the library was reasonably well sampled for diversity [24,25]. It is sometimes suggested that 16S rRNA sequences say nothing about prokaryote phenotypes. However, if one accepts that 16S rRNA trees reflect organism phylogeny, then when a clone sequence falls within a group of known and consistent phenotypes, it is reasonable to infer a similar physiology for the organism from which that sequence originated [10]. Thus, East Hill Bridge sediments appear to support the full range of methanogen phenotypes (Fig. 1) including methanogens able to use CO2 and H2 (e.g. Methanogenium and Methanocorpusculum like), acetoclastic methanogens (Methanosaeta and Methanosarcina like) and methylotrophic methanogens (Methanolobus and Methanosarcina like). Our results demonstrate that these brackish sediments contain some similar genotypes to those previously detected in the marine sediments at Colne Point [10], suggesting that some methanogens such as Methanogenium, Methanolobus, Methanosaeta and a novel group, called the River Colne (RC) Group I, related to Methanococcoides (>93% similar) are distributed throughout the salinity gradient.
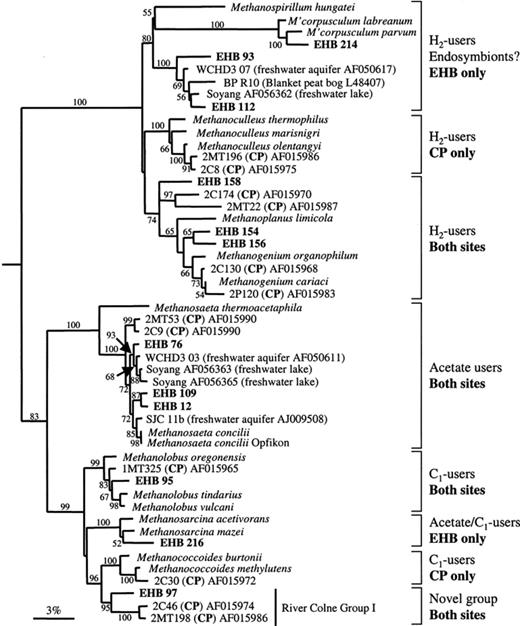
Inferred phylogenetic relationships between environmental sequences from East Hill Bridge (EHB) and Colne Point (CP) sediments, reference taxa and available environmental clones. Logdet/Paralinear distances tree based on 940 alignable nucleotides using 56% of the sites, rooted using T. acidophilum (not shown on tree). Bootstrap support values (1000 replicates) above 50% are shown at nodes.
Our data also suggest that there were differences in the archaeal communities at the marine and brackish ends of the estuary. We failed to detect clones related to the Marine Benthic Group D which, since their initial discovery in Colne Point, have been detected in marine sediments worldwide [10,26]. Nor did we detect clones related to the halophilic archaea which were abundant in the gene libraries made from the marine sediments. That these two groups were not detected at East Hill Bridge (or any other published freshwater study) is consistent with them representing predominantly marine species.
There were also differences in the detected methanogenic community. Clones related to Methanosarcina, Methanocorpusculum and a blanket peat clone (BP R10, [27]) were detected only at the East Hill Bridge site while clones closely related to Methanoculleus and Methanococcoides were detected only at Colne Point. The group of clones related to the blanket peat clone BP R10 [27] are all derived from freshwater habitats [28,29] suggesting a brackish/freshwater role for these widely distributed genotypes. Both this group and the Methanocorpusculum cluster are related to H2-utilising endosymbiotic methanogens (none of which are shown in Fig. 1 as the published sequences were too short for this analysis [30]) and may indicate the presence of a substantial protozoan linked endosymbiotic methanogen community at this site as has been hypothesised for the methanogen community in a freshwater lake in Switzerland [31]. The distribution of H2-utilising methanogen groups suggests that while Methanogenium are found along the estuary, others are limited to either marine environments (Methanoculleus at Colne Point) or freshwater/brackish conditions (Methanocorpusculum and the BP R10 group at East Hill Bridge).
Clones closely related to Methanosaeta concilii (>98% sequence similarity) were the most common clones detected in the East Hill Bridge library, suggesting that close relatives of this species may be important methanogens in East Hill Bridge sediments. Cultured representatives of Methanosaeta sp. are all obligate acetate utilisers so we suggest that the methanogens from which the East Hill Bridge clones originate also share this phenotype. Clones related to Methanosarcina, which are also able to utilise acetate, were also found in the East Hill Bridge sediments, supporting the supposition that acetate degradation is an important component of methanogenesis in these sediments. Acetate is reported to be the primary substrate for methanogenesis in freshwater sediments, accounting for up to 80% of methanogenesis [32–35]. Interestingly, clone sequences that group closely with M. concilii have been found in all freshwater sediments and environments investigated so far using 16S rRNA gene clone libraries (Fig. 1 and others not included in this tree from rice paddy soils and a freshwater lake [31,36]). This suggests that organisms closely related to M. concilii are ubiquitous in freshwater sediments and we suggest that they are prime candidates for a globally important acetoclastic methanogenic group. The inclusion of the marine clones (2P53 and 2C9) in this group indicates that some M. concilii strains may be able to tolerate the saline conditions at Colne Point, although these clones may also be derived from inactive cells washed downstream from the freshwater end of the river.
The distribution of clones in the two River Colne libraries would imply that within the methanogen community certain groups survive by being generalists that are adapted to the range of conditions within an estuarine environment (e.g. Methanogenium) while others are more specialist (e.g. Methanosaeta). We are currently probing rRNA extracted directly from both the freshwater and marine sediments to determine whether the hypotheses about the distribution and dominance of the in situ methanogenic communities inferred from 16S rDNA clone libraries are supported by the relative abundance of these groups in situ.
References
Author notes
Present address: Department of Oral Medicine and Pathology, KingGuy's College London, Guy's Hospital, London, UK.