-
PDF
- Split View
-
Views
-
Cite
Cite
Lynne Boddy, Interspecific combative interactions between wood-decaying basidiomycetes, FEMS Microbiology Ecology, Volume 31, Issue 3, March 2000, Pages 185–194, https://doi.org/10.1111/j.1574-6941.2000.tb00683.x
- Share Icon Share
Abstract
Competition is the most common type of interaction occurring between wood-decaying higher fungi. Since competition for nutrients in organic resources is effectively brought about by competition for space, the common division into interference and exploitation competition is not very appropriate. Fungal competition can be divided into primary resource capture (obtaining uncolonized resources) and secondary resource capture (combat to obtain resources already colonized by other fungi). Combative mechanisms include antagonism at a distance, hyphal interference, mycoparasitism and gross mycelial contact. Interactions can result in deadlock or replacement, and a hierarchy of combative ability can be discerned amongst fungi that inhabit particular resources, but within this hierarchy there exists intransitivity, modification of outcome by other species and abiotic variables. Interactions can dramatically alter mycelial function, and have potential as biological control agents of fungal pathogens of trees and in service timber.
1 Introduction
At early stages of colonization of virgin organic resources such as wood, fungal mycelia may develop for a brief period in the absence of other organisms. With time, however, as mycelia expand their domains will increasingly overlap and interactions will occur. While wood decay fungi exhibit the whole spectrum of interaction types, including mutualistic interactions and those detrimental to neither but not beneficial to both, competitive interactions are most common. Keddy's [1] general ecological definition of competition as ‘the negative effects which one organism has upon another by consuming, or controlling access to, a resource that is limited in availability’ is applicable to fungi.
Competition is commonly considered by macroecologists as either interference competition or exploitation competition [1]. The former refers to the situation where one organism inhibits another, for example by the production of allelopaths or by shading out of slower growing plants. Exploitation competition, on the other hand, occurs when one organism uses a resource and consequently reduces the availability to another. Competition between fungi has also been discussed in these terms [2,3], however, this distinction is not clear for heterotrophic mycelial fungi that decompose leaf and woody litter. Since these fungi gain access to nutrients by colonizing solid organic resources, competition for nutrients is effectively brought about by competition for space/territory. Thus, in these circumstances interference and exploitation competition cannot sensibly be divorced. Perhaps the only situation in which the distinction might be appropriate is when those fungi which are not restricted to a resource unit grow out in search of new organic resources [4,5]. During passage through soil they sequester nutrients from solution, which might otherwise have been available to other soil microorganisms.
Fungal competition in organic resources can, however, be further divided into primary resource capture and combat recognizing the difference respectively between gaining initial access to, and influence over, an available uncolonized resource and capturing territory from fungi already colonizing a resource or defending territory from potential invaders [6]. Success in primary resource capture is determined by various factors, including good dispersal, rapid spore germination, rapid mycelial extension, and ability to utilize organic compounds available in previously uncolonized resources. Such fungi are said to have r-selected characteristics [6]. Success in combat, on the other hand, depends on antagonistic mechanisms. These combative interactions are the main focus of this review.
2 Mechanisms of combative interactions
Antagonistic interactions can be mediated at a distance, via volatile or diffusible antibiotics or waste products, or following contact. The latter can operate both at the level of individual hyphae and mycelia, though obviously hyphal interactions contribute to the expression of gross mycelial interactions. Two main distinctive types of interspecific hyphal interaction occur: (1) interference and (2) parasitism.
2.1 Antagonism at a distance: volatile and diffusible antibiotics
Antagonism at a distance is usually attributed to diffusible or volatile compounds [7]. Though the hyphae of one mycelium often become degenerate and are replaced by the other mycelium, very commonly, in agar culture, there is mutual inhibition. Moreover, reactions vary depending on species combinations. This suggests a reciprocal exchange of chemical signals and recognition at a distance [8]. In agar culture mutual inhibition for 15 mm or more may result, but the distances over which such mechanisms operate in the natural environment are unknown, though they are likely to be shorter than this in organic substrata.
2.2 Hyphal interference
This occurs when a hypha makes contact with another hypha or a spore, and results in death of the contacted compartment(s) following a programmed sequence of cytoplasmic destruction. It is common amongst wood-decaying basidiomycetes, the most studied example being the interference with Heterobasidion annosum hyphae by Phlebiopsis gigantea (see refs. in [9,10]), because of its application in biocontrol Section 6. The interference is mediated through a non-enzymic diffusible metabolite(s), released only when hyphae are in close proximity. In contrast, other species produce non-enzymic toxins both in the presence and absence of the antagonist, e.g. Hypomyces aurantius (see refs. in [9,10]). Another example is provided by Phanerochaete magnoliae that specifically replaces Datronia mollis, and forms a hymenial fruiting surface through that of the latter. The former produces sparse, rapidly extending hyphae which grow between those of the latter, and when contact is made highly destructive hyphal interference affects both species, but ultimately P. magnoliae emerges as the sole survivor [11].
2.3 Mycoparasitism
Parasitism of one hypha by another is preceded by contact and recognition of the host by a lectin or agglutinin-carbohydrate interaction [10,12]. This is followed by penetration or appression to and continued growth over the hyphal surface of the host. Nutritients can then be obtained from the host biotrophically or necrotrophically. Only a few examples have been reported between wood decay fungi: Lenzites betulina is parasitic on Coriolus species, and Pseudotrametes gibbosa is parasitic on Bjerkandera species. In these cases, rather than parasitism being the sole or major source of nutrition it is a temporary situation facilitating possession by the mycoparasites of the large mycelial domain that the hosts occupy in wood [13]. When the parasites have possession of this domain they obtain their nutrition by decomposing the wood, and they interact aggressively with other fungi by gross mycelial contact (Section 2.3). The situation has been likened to that of strangler figs and temporary social parasitism in ants [13].
2.4 Gross mycelial contact
Gross mycelial contact seems to be a particularly important type of interaction between wood-decaying higher fungi. Commonly, mycelial morphology changes during interactions (Fig. 1). In agar culture this takes the form of stationary ‘barrages’ resistant to invasion, invasive mycelial fronts (Fig. 1a–c,l), mycelial fans (Fig. 1d,j,k) and linear organs – cords and rhizomorphic structures (Fig. 1e), all of which can sometimes be expressed in a single interaction [14]. These ‘phase shifts’ are accompanied by a redistribution of mycelium; distal to the interaction zone there is reduction in mycelial density (Fig. 1d,e,k), rendering the colony more susceptible to invasion if the interfacial zone is breached (Fig. 1j,k). There is also evidence of redistribution of mycelium in wood where, upon direct incubation, mycelial outgrowth is often prolific at the edges of decay columns close to interaction zones and often much sparser in inner regions of decay columns (Fig. 2f). The invasive ‘phases’ are thought to be non-assimilative but driven forward from the rest of the colony which is assimilative [14].
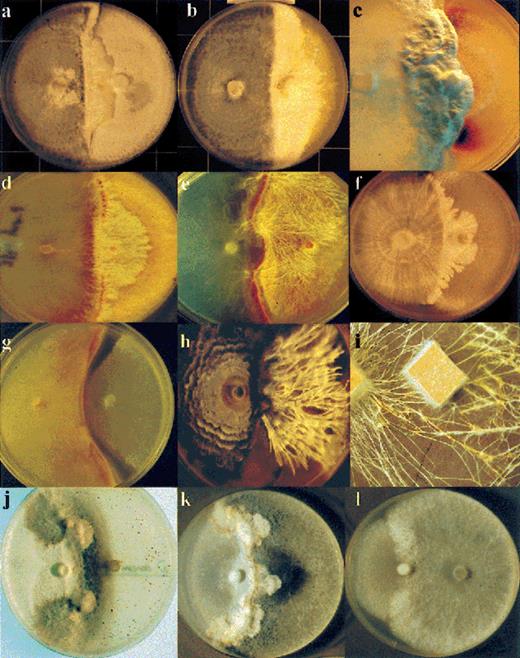
Mycelial interactions in agar plates (9 cm diameter) (a–h), soil (i) at 25°C and ambient gaseous regime unless otherwise stated, the fungus mentioned first being inoculated on the left side of the plate and that mentioned second on the right side: L. betulina replacing Vuilleminia comedens (a), S. hirsutum (b) and the ascomycete Lopadostoma turgidum (elevated CO2) (c); P. velutina replacing S. hirsutum at 25°C (d) and 10°C (e); H. fasciculare replacing C. versicolor (f); Psathyrella hydrophillum replacing S. hirsutum at 10°C – note zone of lysis preceding replacement (g); complete replacement of Armillaria gallica by the ascomycete X. hypoxylon– note the completely different morphologies of the latter on the two sides of the plate (h); mycelial interference of P. velutina by R. bicolor on soil (sides of wood blocks 2 cm) (i). C. versicolor interacting with the ascomycete Daldinia concentrica (j–l), (k) a similar pairing to (j) but several weeks later, (l) under 30% CO2/5% O2 after the same time as (k). (Photographs c, d, e, g, h courtesy of I.H. Chapella, and i courtesy of J.M. Wells and M.J. Harris).
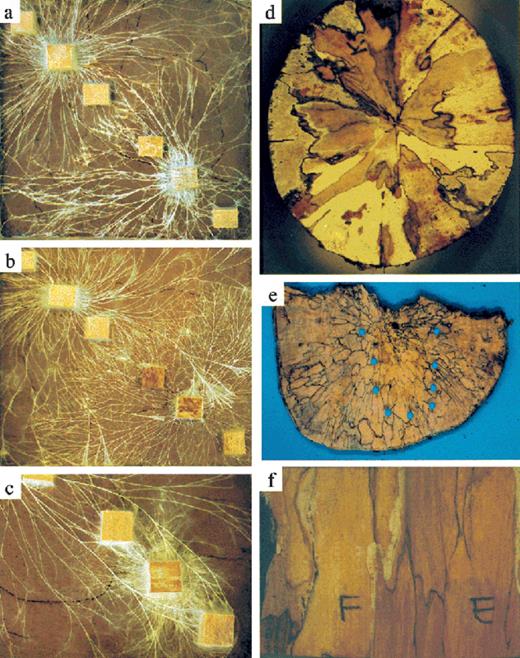
Interactions between higher fungi in soil and wood. (a–c) Mycelial cord systems growing from wood block inocula (sides 2 cm) in trays (24×24 cm) of non-sterile soil at 12.5°C. The wood block inocula were placed in corners diagonally opposite each other. Fresh wood resources were placed on either side of the inocula on the diagonal, and were colonized by the developing mycelial systems. P. velutina (top left) interacting with (a) R. bicolor and (b) P. impudicus and (c) H. fasciculare. Note interference between cords at the junction of P. velutina colonies with R. bicolor and P. impudicus (a, b), and the cords of P. velutina overgrowing those of P. impudicus and H. fasciculare with subsequent colonization of the wood resources. Transverse (d, e) and longitudinal (f) sections through beech (F. sylvatica) logs 4.5 year after felling. Dark lines are ‘interaction zone lines’ demarcating different fungal individuals (of the same or different species). Sections shown in (d) and (f) were incubated in moist conditions in polythene bags allowing mycelium to grow out of some of the decay columns. Faded and disjointed zone lines indicate that the fungus that formed them has been replaced by another species, e.g. in (d) the ‘relic’ zone lines in the region outlined with blue circles were originally formed by the ascomycete X. hypoxylon, but decay columns occupied by numerous individuals of the latter have been replaced by a single individual of a white-rot producing (indicated by lighter colored wood) basidiomycete. The numerous decay columns evident in (e) is indicative of colonization by genetically different spores. (Photographs (a–c) courtesy of J.M. Wells and M.J. Harris, and (d–f) of I.H. Chapela.)
Interactions in agar are commonly accompanied by inter- and intracellular pigment production (e.g. Fig. 1c,d,e,g,j), and changes in phenol-oxidizing activity [15,–18]. There also appears to be a reciprocal relationship between tyrosinase and laccase enzyme activity associated with formation and suppression of aerial mycelium respectively, and marked differences in release of hydrophobic metabolites in extracts from interacting cultures at different stages of development [16,19,20]. Further, exposure of cultures of single species to the uncoupling agent 2,4-dinitrophenol resulted in similar metabolite profiles and developmental morphologies to those seen during interactions, while incorporation of a phenoloxidase inhibitor into the medium resulted in metabolite release [15,16,19]. This has led to the suggestion that there is a relationship between mycelial pattern, hydrophobic extracellular chemistry and phenol-oxidizing activity [14].
In soil/leaf litter, interactions between mycelial cord systems and between mycelial systems of fairy ring-forming basidiomycetes are evident at the gross mycelial level. When cord systems (Fig. 2a–c) make contact there is often a marked yellow or brown discoloration and lytic responses in one or both of the cord segments involved ([21], Fig. 1i). These mycelial responses have been termed ‘mycelial interference’, indicating their affinity with ‘hyphal interference’ between individual hyphae (Section 2.2). Mycelial search patterns and coverage also alter (Section 5).
Gross mycelial interactions also occur in woody resources both when mycelia colonize from well established mycelial cord systems (Fig. 2a–c) and when mycelial domains of fungi established within the resource overlap. Progress of mycelial confrontations within wood cannot, however, be observed directly non-destructively because of the opaque nature of the substratum, but artificial pairings can be made in wood lengths that can be sacrificed over a time course and isolations and observations made from sections along the length to follow the progress of interactions ([22], Fig. 3).
![Outcome of direct mycelial pairings in joined ash (F. excelsior) twig lengths between P. lycii (left) and Phomopsis platanoidis (right) under three different abiotic regimes, after 4 weeks: (a) on open shaded roof; (b) −0.8 MPa, 20°C; (c) −4.1 MPa, 20°C. Diagrams are pictorial representations of actual pairs of joined twig lengths (three replicates). Shadings demarcate volumes of twigs colonized by different species (determined by isolations). Note that P. lycii exhibits highly polar growth, such that as it grows and replaces P. platanoidis it leaves empty the wood that it formerly occupied. Redrawn from [22].](https://oup.silverchair-cdn.com/oup/backfile/Content_public/Journal/femsec/31/3/10.1111_j.1574-6941.2000.tb00683.x/1/m_FEM_185_f3.jpeg?Expires=1750717650&Signature=13oTRedgZ7Yf8vSXDXCKVrDx2kxDba7-4CmB67bPAPRUS0Y38~PkwLzo3vUMcChEmFq46nfxrTY091AIcDTVBREQ1MkGfx63otiCtDxut~0ZaqgGD~VCWsEh8FU-he3Smlq556KDkPyeud5aAQU6A7~cS4d5e~W8dQ8XTYGGGZ3V64HMJgkWOizjLxqUSpyeR1Wc8Fnr8df91aV~084bJNz2TdWiTo54u7rIq-cHaHAOOTC4BCPEgW3I6P5HKpzHGrhwFiumhAo6QFtWxlKpkYp61KJOLWmH9q-oIvHGYCZzAchtxeN93ZG2XN5oKVYFG9SdgjeOBdSBGyYErwW87Q__&Key-Pair-Id=APKAIE5G5CRDK6RD3PGA)
Outcome of direct mycelial pairings in joined ash (F. excelsior) twig lengths between P. lycii (left) and Phomopsis platanoidis (right) under three different abiotic regimes, after 4 weeks: (a) on open shaded roof; (b) −0.8 MPa, 20°C; (c) −4.1 MPa, 20°C. Diagrams are pictorial representations of actual pairs of joined twig lengths (three replicates). Shadings demarcate volumes of twigs colonized by different species (determined by isolations). Note that P. lycii exhibits highly polar growth, such that as it grows and replaces P. platanoidis it leaves empty the wood that it formerly occupied. Redrawn from [22].
3 Outcome of interactions
Gross outcomes of combative interactions can be either replacement, where one fungus gains the territory of the other, or deadlock where neither fungus gains headway. Sometimes partial replacement will result where initially one fungus gains headway but subsequently deadlock ensues, or where both species make some ingress into the territory held by the other (Fig. 1j,k). A complete change from initial replacement of one fungus to complete replacement of the other can sometimes occur, even without apparent changes in environmental conditions. For example, with Phlebia radiata and Phlebia rufa colonies begin initially to be replaced by fungi which are early colonizers of attached branches (e.g. Quercus spp.), however, subsequently the balance shifts and often the outcome is replacement of the opponent [23]. This is associated with the apparent inability of colony margins of these two Phlebia species to recognize other fungi as antagonists, recognition occurring in older regions.
Outcomes of interactions between the same combinations of fungi are not always the same, even under apparently identical conditions. In 20 replicate encounters of Peniophora lycii with Coriolus versicolor three different interaction patterns occurred: C. versicolor replaced P. lycii in two of these, and P. lycii replaced C. versicolor in the other [14].
3.1 Field evidence of mycelial interactions
Sawing wood into sections reveals evidence of interactions in the form of a variety of patterns of discoloration and decay (e.g. Fig. 2d–f). ‘Interaction zone lines’– narrow regions of wood, appearing as lines in cross-section, having a different color (often darker) from adjacent wood – are characteristic of mycelial confrontations between different species or individuals of the same species (Fig. 2d–f). These zone lines comprise pseudosclerotial plates (PSPs), which are commonly longitudinally extensive, surrounding the column of wood occupied by a wood decay fungus. They are crust-like aggregations of either highly branched and anastomosed or pseudoparenchymatous hyphae, often melanized with individual compartments being inflated or distorted. Interaction zone lines should not, however, be confused with reaction zones which have different origins, nor with PSPs formed by some fungi, e.g. Armillaria spp., Xylaria spp. and Ustulina deusta, to maintain a favorable moisture regime in the wood – wet for the former and dry for the last two (e.g. [9,24]). Interestingly, the niche formed by the narrow ‘no man's land’ within double zone lines (one line formed by each of the antagonists) is often occupied by dematiaceous microfungi, e.g. Chaetosphaeria myriocarpa and Rhinocladiella spp. (e.g. [9]).
If the position of these interaction zone lines appears to be permanent, the outcome of the interaction between the species on either side is probably deadlock, but the occurrence of ‘relic’ zone lines (Fig. 2e) within an area of decay suggests replacement of one mycelium by another (see [9,24]). Since zone lines are sometimes characteristic of species, it is sometimes possible to tell not only which fungus is currently present but also which was there before. Presence of more than one decay fungus in a column (as revealed by isolation or direct incubation of wood (Fig. 2d,f) to yield mycelium which can be identified) is often indicative of an ongoing replacement interaction.
3.2 Factors affecting the outcome of interactions
Not surprisingly, in view of the difficulties of observation in wood, interactions have been most commonly studied in agar culture in the laboratory (e.g. [6,7,15,16,19,25–30]). While such an approach can provide valuable information, interpretation of outcomes and extrapolation to the field must be made with caution, since results of interactions in agar culture and natural substrata are not necessarily the same (e.g. [21,22,31]). For example, Hypholoma fasciculare replaced Steccherinum fimbriatum in agar culture under ambient conditions, but deadlocked with it when mycelial cords met in soil, and was itself replaced when paired in wood [21].
Water (solute) potential (ψ), gaseous regime and to a lesser extent temperature considerably affect outcomes of interactions in agar culture (Table 1; Fig. 1j–l; [22,25–27]). Different species are affected differently, but some general trends related to ecological and taxonomic groups emerge. Wood decay basidiomycetes cannot grow at much below −4.4 MPa [32], and some are even more sensitive to reduced water potential, but xylariaceous ascomycetes can grow at much lower ψ (L. Boddy, unpublished). The antagonistic ability of basidiomycetes seems to be lessened at the lower end of their range of tolerance to reduced ψ. The antagonistic ability of cord-forming basidiomycetes declines with elevated CO2/reduced O2[27]. Temperature can affect the form, rate, extent (Fig. 1d, e) and outcomes of interactions [33].
Effect of water potential and gaseous regime on outcome of interactions between D. concentrica and other wood decay fungi from ash (F. excelsior) in agar culture (modified from [26])
Opposing species | Malt agar | −1.3 MPa | −3.1 MPa | −4.4 MPa | 5% O2, 30% CO2 | 5% O2, 60% CO2 | 20% O2, 30% CO2 | 20% O2, 60% CO2 |
C. versicolor | D to partial R of Dc by Cv | partial to complete R of Dc by Cv | D, some lysis of Dc | no growth of Cv | D to slight R of Cv by Dc | D to slight R of Cv by Dc | partial R of Cv by Dc | R of CV by Dc |
Hypoxylon rubiginosum | R of Hr by Dc | D to slight R of Hr by Dc | D | colonies did not meet | D to slight R of Dc by Hr | D to slight R of Hr by Dc | D | D to slight R of Hr by Dc |
Mycacia uda | R of Mu by Dc | R of Mu by Dc | no growth of Mu | no growth of Mu | R of Dc by Mu | R of Dc by Mu | R of Dc by Mu | R of Dc by Mu |
P. radiata | partial to complete R of Pr by Dc | R of Pr by Dc | no growth of Pr | no growth of Pr | D to slight R of Pr by Dc | D to slight R of Pr by Dc | R of Pr by Dc | R of Pr by Dc |
Abbreviations: D, deadlock; R, replacement; Cv, Coriolus versicolor; Dc, Daldinia concentrica; Hr, Hypoxylon rubiginosum; Mu, Mycacia uda; Pr, Phlebia radiata. |
Opposing species | Malt agar | −1.3 MPa | −3.1 MPa | −4.4 MPa | 5% O2, 30% CO2 | 5% O2, 60% CO2 | 20% O2, 30% CO2 | 20% O2, 60% CO2 |
C. versicolor | D to partial R of Dc by Cv | partial to complete R of Dc by Cv | D, some lysis of Dc | no growth of Cv | D to slight R of Cv by Dc | D to slight R of Cv by Dc | partial R of Cv by Dc | R of CV by Dc |
Hypoxylon rubiginosum | R of Hr by Dc | D to slight R of Hr by Dc | D | colonies did not meet | D to slight R of Dc by Hr | D to slight R of Hr by Dc | D | D to slight R of Hr by Dc |
Mycacia uda | R of Mu by Dc | R of Mu by Dc | no growth of Mu | no growth of Mu | R of Dc by Mu | R of Dc by Mu | R of Dc by Mu | R of Dc by Mu |
P. radiata | partial to complete R of Pr by Dc | R of Pr by Dc | no growth of Pr | no growth of Pr | D to slight R of Pr by Dc | D to slight R of Pr by Dc | R of Pr by Dc | R of Pr by Dc |
Abbreviations: D, deadlock; R, replacement; Cv, Coriolus versicolor; Dc, Daldinia concentrica; Hr, Hypoxylon rubiginosum; Mu, Mycacia uda; Pr, Phlebia radiata. |
Effect of water potential and gaseous regime on outcome of interactions between D. concentrica and other wood decay fungi from ash (F. excelsior) in agar culture (modified from [26])
Opposing species | Malt agar | −1.3 MPa | −3.1 MPa | −4.4 MPa | 5% O2, 30% CO2 | 5% O2, 60% CO2 | 20% O2, 30% CO2 | 20% O2, 60% CO2 |
C. versicolor | D to partial R of Dc by Cv | partial to complete R of Dc by Cv | D, some lysis of Dc | no growth of Cv | D to slight R of Cv by Dc | D to slight R of Cv by Dc | partial R of Cv by Dc | R of CV by Dc |
Hypoxylon rubiginosum | R of Hr by Dc | D to slight R of Hr by Dc | D | colonies did not meet | D to slight R of Dc by Hr | D to slight R of Hr by Dc | D | D to slight R of Hr by Dc |
Mycacia uda | R of Mu by Dc | R of Mu by Dc | no growth of Mu | no growth of Mu | R of Dc by Mu | R of Dc by Mu | R of Dc by Mu | R of Dc by Mu |
P. radiata | partial to complete R of Pr by Dc | R of Pr by Dc | no growth of Pr | no growth of Pr | D to slight R of Pr by Dc | D to slight R of Pr by Dc | R of Pr by Dc | R of Pr by Dc |
Abbreviations: D, deadlock; R, replacement; Cv, Coriolus versicolor; Dc, Daldinia concentrica; Hr, Hypoxylon rubiginosum; Mu, Mycacia uda; Pr, Phlebia radiata. |
Opposing species | Malt agar | −1.3 MPa | −3.1 MPa | −4.4 MPa | 5% O2, 30% CO2 | 5% O2, 60% CO2 | 20% O2, 30% CO2 | 20% O2, 60% CO2 |
C. versicolor | D to partial R of Dc by Cv | partial to complete R of Dc by Cv | D, some lysis of Dc | no growth of Cv | D to slight R of Cv by Dc | D to slight R of Cv by Dc | partial R of Cv by Dc | R of CV by Dc |
Hypoxylon rubiginosum | R of Hr by Dc | D to slight R of Hr by Dc | D | colonies did not meet | D to slight R of Dc by Hr | D to slight R of Hr by Dc | D | D to slight R of Hr by Dc |
Mycacia uda | R of Mu by Dc | R of Mu by Dc | no growth of Mu | no growth of Mu | R of Dc by Mu | R of Dc by Mu | R of Dc by Mu | R of Dc by Mu |
P. radiata | partial to complete R of Pr by Dc | R of Pr by Dc | no growth of Pr | no growth of Pr | D to slight R of Pr by Dc | D to slight R of Pr by Dc | R of Pr by Dc | R of Pr by Dc |
Abbreviations: D, deadlock; R, replacement; Cv, Coriolus versicolor; Dc, Daldinia concentrica; Hr, Hypoxylon rubiginosum; Mu, Mycacia uda; Pr, Phlebia radiata. |
In environmentally more realistic systems, reduced ψ also sometimes changes outcomes, as seen when pairings were made in twig lengths ([22]; Fig. 3), and between cord-forming fungi meeting in soil (D.P. Donnelly and L. Boddy, unpublished). Again outcomes were not necessarily the same as in agar at the same ψ. Even if the ultimate outcome is not affected by environmental regime, the rate of replacement of one species by another can be altered [22].
Size and quality of the resources that the combatants have access to is another factor influencing outcome of interactions. Fungi occupying large wood resources commonly have a relatively higher success in combat than do those occupying smaller resources when challenged with the same species [34]. As decomposition proceeds resource quality changes, and state of decay can influence outcome of interactions, as revealed in experiments in which fungi were grown in wood blocks either for 4 weeks or 6 months and then placed on soil, where mycelia grew out and interacted (L. Boddy and M. Warren, unpublished). For example, H. fasciculare extending from wood colonized for 6 months partially replaced P. radiata in wood colonized for 6 months. However, when the former was extending from 4 week wood blocks it completely replaced the latter. When both were extending from 4 week wood blocks the outcome was deadlock. Initial spatial configuration of inoculum in complex tesselations of more than two species considerably influences community development regardless of inoculum quantity or proportion [30].
Clearly, microclimate, resource size and quality can all shift the balance between opponents. Thus, in the natural environment, the outcome of interactions between species is variable, leading to differences in community structure and dynamics.
3.3 Intransitivity
To use general ecological parlance, within the spectrum of outcomes of combative interactions there are transitive and intransitive hierarchies. A transitive hierarchy refers to the situation where species A is more combative than species B, and both are more combative than species C (i.e. A>B>C). For example, Phanerochaete velutina replaced C. versicolor, and both replaced Stereum hirsutum in agar culture [26]. On the other hand, the situation where species A is more combative than species B, species B is more combative than species C, but species C is more combative than species A (i.e. A>B, B>C, C>A) is termed intransitive. For example, in agar culture, Phallus impudicus replaced Megacollybia platyphylla, M. platyphylla replaced Psathyrella hydrophilum, but P. hydrophilum replaced P. impudicus[27].
Intransitive hierarchies are another factor that makes exact prediction of community change in the natural environment impossible.
3.4 Indirect interaction effects and interaction modifications
In general ecological theory, indirect effects are considered to occur as a result of the effect that one species (A) has on another (C) via a change in density of a third species (B) [35]. Thus, if in pairwise interactions species A outcompetes species B and C, and species B outcompetes species C (i.e. A>B; A>C; B>C), when all three compete simultaneously species A reduces the population size (numbers) of species C, but it also reduces the population size of species B, the latter then having less effect on species C. Such effects are said to be non-additive, since the summed results of paired interactions cannot predict the outcome of three-way or higher interactions. It is difficult to relate these ideas directly to wood decay fungi since the emphasis is on population size, whereas because of the indeterminate nature of mycelia it is usually more appropriate to consider outcome of interactions at the level of the individual. In saying that, however, fungi arising as airborne spores might appropriately be considered in these terms, as initially there will be many hundreds of microscopic mycelia competing to colonize the wood resource from the surface [36].
Interaction modification refers to the situation where the outcome of interaction between two species is altered by the presence of a third species [35]. Again this is a non-additive situation where results of pairwise interactions cannot successfully predict that of a three- or higher-way interaction. For example, in agar Poria placenta replaced Coniophora marmorata, C. marmorata replaced the imperfect fungus Paecilomyces variotii, while P. placenta deadlocked with P. variotii[30]. Extrapolation to three species interactions might suggest that P. variotii would be replaced by C. marmorata, which would in turn replace P. placenta. However, tessellations of three species resulted in variable outcomes [30]. In another example, outcome of interactions between pairs of wood decay basidiomycetes in agar culture often differed in the presence of diffusible metabolites of the non-wood decay fungus Trichoderma harzianum[33].
The degree of ease/difficulty with which a fungus replaces one species relative to another can determine which of these is replaced in higher-way interactions. For example, when P. velutina growing from a wood resource through soil encountered three new resources precolonized by S. hirsutum, S. rugosum and U. deusta, P. velutina preferentially replaced the latter, while the other two resources were effectively ‘ignored’[37]. When encountered individually P. velutina replaces all three species.
4 Hierarchy of combative ability and effects of interactions on fungal community development
Within fungal communities which develop in particular resources, e.g. attached ash (Fraxinus excelsior) branches [25], attached oak (Quercus robur) branches [38], felled beech (Fagus sylvatica) logs [27,36] and Norway spruce (Picea abies) logs [39,40], clear hierarchies of combative ability have been revealed. In view of the foregoing, it is not surprising that these hierarchies are rather like a sports league in that teams at the top of the table are sometimes beaten by those lower down, and the outcome of a confrontation can be considerably affected by abiotic conditions.
Also, as with sports teams, some fungi are generally poor and others are very good at both attack and defence, on the other hand some are good at attack but poor in defence, while others even though poor at attack are good at defending territory that they hold. This is somewhat analogous to the concept in plant ecology where competition is divided into competitive effect and competitive response, the former being the ability to suppress resource levels for other species, and the latter the ability to tolerate suppression [41]. For example, in wood, though not necessarily in agar culture, the early colonizing ascomycete Xylaria hypoxylon tends not to be able to extend its territory once no uncolonized resource remains. It can, however, remain in felled beech logs for 4.5 years or more [27]. In artificially inoculated wood discs, Cytostereum murraii and Phlebia centrifuga were good in defence, but not particularly good at attack against other basidiomycetes from Norway spruce [38].
These hierarchies are broadly, though not completely, correlated with position of the fungi in the succession. The primary colonizers, effective in primary resource capture, tend either already to be present in functional sapwood or bark as latent propagules [42] or to be prolific spore producers that arrive rapidly, germinate and grow rapidly but with limited combative ability (i.e. have r-selected characteristics) [9,18,24,42]. Fungi that arrive once the community is closed have to rely largely on combative ability. However, why some extremely combative species, e.g. Junghuhnia collabens in Norway spruce, tend to be found only at very late stages despite being able to colonize undecayed wood in laboratory tests remains unclear [40]. This may relate to the fact that combative ability of mycelia may not necessarily by reflected by ability to establish from spores landing on wood colonized by active mycelia, and also spore production and dispersal of these fungi may be low. Selective parasitism of one species/genus negates this problem (Section 2.3). Also, some fungi appear selectively to follow others using non-parasitic antagonistic mechanisms. More than 20 basidiomycetes show strong dependencies on certain specific preceding decay fungi, based on extensive field surveys of fruit bodies in northwestern Europe [43]. In artificially inoculated wood discs in the laboratory, ‘late-stage’ fungi were more successful in ‘natural’ predecessor-successor pairings than in pairings that do not commonly occur in nature [44]. This may be at least partly related to changes in abiotic conditions (e.g. lignocellulosic composition, inhibitory extractives, water relations) in the wood brought about by the preceding fungus.
When abiotic stresses and disturbance events are imposed tolerance of these is more important than combative ability. For example, at later stages of decay of oak branches in the canopy bark is lost and wood becomes more porous and prone to desiccation and invertebrate invasion. These conditions favor Shizopora paradoxa, which though it has poor combative ability is able to survive by virtue of its production of thick-walled chlamydospores, and Hyphoderma setigerum that is tolerant of the disturbance caused by wood boring beetles [38]. Invertebrate activity is inimical to the majority of wood-decaying basidiomycetes, resulting at very late stages of decomposition in a shift to non-combative r-selected soil fungi [9,24].
Clearly, the exact pattern of community structure and development will be different for every single woody resource unit. Nonetheless, general trends and driving forces for change can be discerned and general schemes for community development pathways produced [9,24,36].
5 Effect of interactions on mycelial function
As well as the changes in mycelial morphology and enzyme production already described for interactions occurring in agar culture (Section 2.4), recent studies have revealed that interspecific interactions can alter the functioning of mycelia, including mycelial search patterns, distribution and reallocation of nutrients within mycelia, and respiration.
Fungi that are able to grow out of the resource which they are colonizing in search of new resources exhibit different foraging patterns depending on species, carbon and mineral nutrient status of the mycelial system and surrounding soil [5]. Their mycelial extension rates (both towards and away from an antagonist) and extra-resource mycelial biomass production often decrease following encounter with another fungus (D.P. Donnelly and L. Boddy, unpublished). Further, mycelial distribution is also dramatically altered: the fractal dimension ([5]; a measure of space-filling) was often lowered (indicating less branching) following encounter with other mycelia.
Though there has been little study so far of how interacting fungi reallocate resources within their mycelia, changes in mycelial cord systems seem to be dramatic. Carbon and mineral nutrients are translocated to different parts of mycelial cord systems depending upon supply and demand [4,5]. During interactions in soil, rates and patterns of translocation alter and there is considerable loss of nutrients in the interaction zone (J.M. Wells, M.J. Harris and L. Boddy, unpublished).
Changes in metabolic activity of mycelia might be expected during combative interactions, in order to defend against or overcome an opponent. Laccase and cellobiase activities certainly change in mixed culture [45]. Increases and decreases in CO2 evolution have been detected during both deadlock and replacement interactions in wood [46]. Decay rate of beech logs decreased when spores of single species were applied at high concentrations to cut surfaces [36]. The intense intraspecific interactions that resulted even prevented formation of decay columns of individual species.
6 Applied aspects – biological control
Aggressive saprotrophs have potential as biological control agents of pathogenic and nuisance wood decay fungi. Probably the most successful example is the inoculation of stump surfaces of pine (Pinus sylvestris) and Norway spruce (P. abies), immediately after felling, with a suspension of asexual spores of P. gigantea[47]. This protects against the establishment of H. annosum arriving as spores at the cut surface, at least partly as a result of hyphal interference (Section 2.2). Other wood decay fungi, such as Bjerkandera adusta, Fomitopsis pinicola and Hirschioporus abietinus, have also been suggested as possible biocontrol agents. Since, there is inevitably a delay before saprotrophs colonizing from the stump surface reach pathogens established in the roots, fungi that colonize from the soil would be appropriate; aggressive saprotrophic cord-forming fungi, e.g. H. fasciculare, P. velutina and Resinicium bicolor, can be readily established in forest soils by seeding with colonized wood blocks [4]. In Australia, both Hypholoma australe and Phanerochaete filamentosa were successful in controlling Armillaria luteobubalina, a serious root pathogen transmitted mainly via root contact, if stump surfaces were also treated with 40% aqueous ammonium sulfamate solution [48].
Biological control has also been tried as a means of preventing establishment of wood decay fungi in wounds caused by pruning. Here antagonistic non-wood decay organisms, e.g. Trichoderma spp., have been applied. However, in view of the roles of these fungi in both early and late stages of community development, it is not surprising that, although some successes have been reported, there have been negative effects, i.e. decay has been initiated [9].
Wood of freshly felled trees and in service timber is prone to rapid colonization by a wide range of wood decay fungi, and control agents in these situations would have to have broad spectrum inhibitory activity [33]. Trichoderma spp. have again been tested as biocontrol agents in transmission poles, but although some progress has been made success is a fair way from being realized. Control of Neolentinus (=Lentinus) lepideus in creosote treated poles was limited by incomplete colonization by the biocontrol fungus, though the latter was still present 6 years after inoculation [49]. Trichoderma species have also been suggested as having potential for control of the dry rot fungus of buildings, Serpula lacrimans; the presence of the former restricting colonization by the latter but not spread of it, nor decay of wood initially colonized by it [50].
7 Conclusions
Combat, effected by production of volatile and diffusible chemicals, hyphal interference, mycoparasitism and gross mycelial contact, results in deadlock or replacement. In the former no headway is gained over the opponent, whereas in the latter territory is gained. The outcome of interactions varies depending on the size and quality of the resource and on microclimate. Presence of other interacting fungi can also modify outcome, though more research is required in the area of higher-way interactions. Amongst fungi that inhabit a particular resource a hierarchy of combative ability can be discerned, though within this there exists intransitivities under a particular set of conditions and because of differences in interaction outcome under different abiotic and biotic regimes, as just mentioned. Consequently, it is impossible to predict exactly which fungus will follow another during community development. Nonetheless, general patterns of community development and the driving forces for change can be discerned. Interspecific interactions not only effect changes in fungal community composition but also in community functioning, including reallocation of nutrients to different parts of mycelia and release for sequestration by other organisms, and changes in rate of CO2 evolution and decay rate. As in other branches of ecology the relationship between community structure and functioning is a very important but under-researched area. Interspecific interactions not only play a key role in fungal community development and decomposition processes but they also have potential as biological control agents of tree pathogens and fungi that cause decay of in service timber, though apart from the use of P. gigantea to control the cause of Fomes-root rot, there has been little success to date.
References