-
PDF
- Split View
-
Views
-
Cite
Cite
Ana Nicolau, Manuel Mota, Nelson Lima, Physiological responses of Tetrahymena pyriformis to copper, zinc, cycloheximide and Triton X-100, FEMS Microbiology Ecology, Volume 30, Issue 3, November 1999, Pages 209–216, https://doi.org/10.1111/j.1574-6941.1999.tb00649.x
- Share Icon Share
Abstract
Protozoa, and particularly ciliates, are essential in aerobic purification processes of wastewaters and have proved to be very sensitive to environmental changes. The physiological response of the ciliate Tetrahymena pyriformis was assessed in terms of mortality, growth and grazing capacity after exposure to four toxicants: copper, zinc, cycloheximide and Triton X-100. In the ranges of concentrations used, mortality, inhibition of growth and inhibition of grazing were observed with all toxicants employed, but in different ways. Copper and zinc showed lower toxicity than observed in other studies with protozoa, though some of the present results are in accordance with those reported by other authors. This supports the importance of the organism tested and the experimental conditions of the bioassays.
1 Introduction
Ciliated protozoa are very common in aquatic environments and in all types of aerobic and anaerobic biological treatment systems. They often reach densities of about 107 cells l−1 of activated sludge [1,2]. They play an essential role in the purification process by removing, through grazing, the majority of dispersed bacteria, which would cause high turbidity in the final effluent. Moreover, it is recognised that changes in the protozoan community may affect the food web of those ecosystems, thus affecting the biological performance of the wastewater treatment plants. The structure of the protozoan community is therefore an effective indicator of the operating conditions of the plants [3–6] and the effects of toxic chemicals, mainly heavy metals, on this community have been described [7–26].
The sensitivity of protozoa to environmental changes suggested a study of the physiological responses arising from exposure to toxic compounds. The organism chosen to carry out the present work was Tetrahymena pyriformis, a ciliated protozoan not very often observed in activated sludge mixed liquor of well-established plants, but having a clear advantage for the present study as it can be grown in the laboratory, in a standard medium, free from bacteria or other organisms, i.e. in axenic cultures.
Previous work has been devoted to separate studies of grazing, mortality or growth. In order to see whether there are interactions between any of these variables, T. pyriformis was exposed to different concentrations of copper, zinc, cycloheximide (an antibiotic) or Triton X-100 (a neutral surfactant) in a series of miniaturised assays in which mortality, growth and grazing were evaluated simultaneously. Grazing assays were carried out with fluorescently labelled latex beads.
2 Materials and methods
2.1 Organisms and media
In all toxicological assays, axenic 18–24 h old cultures of T. pyriformis GL were used; this strain is characterised by the absence of a micronucleus and its duplication time under the control conditions was about 4.85 h. Meyers et al. [24] indicate 3.8 h and Piccinni et al. [21] 7 h for the duplication time of this strain, both in salt enriched 2% proteose peptone medium, at 25°C. The culture medium used, PPY (Proteose Peptone Yeast Extract Medium, Catalogue of Strains of Culture Collection of Algae and Protozoa (CCAP), UK), contains 20.0 g l−1 of proteose peptone and 2.5 g l−1 of yeast extract and has a pH of 6.6–6.8. All tests were performed at 20±1°C.
Cultures used for grazing assays were intentionally more dense than those used for growth/mortality assays: in the first case, high cell numbers favoured rapid counting of grazed particles, whereas in the latter, dense cultures would complicate cell enumeration. Thus, in growth/mortality assays, initial densities reached 200 to 2600 individuals ml−1 and grazing assays departed from initial densities of about 5000 individuals ml−1.
2.2 Toxicants and concentrations
Copper, as CuCl2·2H2O, was used at six concentrations: 50, 100, 200, 300, 400 and 500 mg l−1 and zinc, as ZnCl2, at four: 50, 100, 200 and 300 mg l−1. In both cases, pH was adjusted to 6.6–6.8 by the addition of 0.1 N NaOH. Distilled tap water, with a pH around 5.5, was used to prepare both medium and toxicant solutions.
Soluble concentrations of heavy metals were obtained after dialysis of the PPY medium through a cellulose membrane of 3500 Da (molecular mass cut-off). For each concentration, two replicates of 10 ml were subjected to dialysis for 24 h in 5 l of de-ionised tap water with 0.02 g l−1 of sodium azide, at 20±1°C. This water was renewed three times, after which both copper and zinc remaining in the medium were determined by photometric Merk Spectroquant Methods, after acid digestion with nitric acid (65%). This was considered to be the amount of metal bound to the dissolved organic matter, allowing determination of the effectively bio-availabel fraction of each metal (Table 1). Less than 1% of total protein was lost during dialysis. Protein was determined by the Sigma Micro Protein Determination Method, based on the biuret and Lowry procedures.
Nominal and soluble concentrations of the heavy metal toxicants used in the present study
Metal nominal concentration (mg l−1) | Soluble metal concentration (mg l−1) | |
Copper | Zinc | |
50 | 30 | 15 |
100 | 65 | 45 |
200 | 145 | 120 |
300 | 200 | 200 |
400 | 275 | – |
500 | 350 | – |
Metal nominal concentration (mg l−1) | Soluble metal concentration (mg l−1) | |
Copper | Zinc | |
50 | 30 | 15 |
100 | 65 | 45 |
200 | 145 | 120 |
300 | 200 | 200 |
400 | 275 | – |
500 | 350 | – |
Nominal and soluble concentrations of the heavy metal toxicants used in the present study
Metal nominal concentration (mg l−1) | Soluble metal concentration (mg l−1) | |
Copper | Zinc | |
50 | 30 | 15 |
100 | 65 | 45 |
200 | 145 | 120 |
300 | 200 | 200 |
400 | 275 | – |
500 | 350 | – |
Metal nominal concentration (mg l−1) | Soluble metal concentration (mg l−1) | |
Copper | Zinc | |
50 | 30 | 15 |
100 | 65 | 45 |
200 | 145 | 120 |
300 | 200 | 200 |
400 | 275 | – |
500 | 350 | – |
Prior to the toxicity assays, a control assay was performed to investigate the influence of the chloride ion present in copper and zinc assays using sodium chloride. No effect was detected in terms of growth, mortality or grazing ability, at the chloride concentrations of the metals assayed.
Triton X-100, a neutral surfactant that disrupts the cell membrane, was used at concentrations of 12.5, 25.0, 37.0, 50.0 and 75.0 mg l−1. Cycloheximide, an antibiotic which inhibits protein synthesis in eukaryotic cells, was used at concentrations of 0.01, 0.05, 0.1, 0.5 and 1.0 mg l−1 in grazing assays and 0.0002, 0.001, 0.002, 0.01 and 0.05 mg l−1 in growth and mortality assays. Two concentration ranges were used since the lower range did not affect grazing capacity and the higher range did not allow growth at any of the concentrations. The pH values of the culture medium were not significantly changed by the addition of Triton X-100 and cycloheximide.
2.3 Toxicity assays
Growth and mortality assays were performed simultaneously in 96-well tissue culture dishes, each well containing 300 μl: 200 μl of PPY with or without the toxicant, and 100 μl of inoculum of T. pyriformis.
For each toxicant, two independent assays were carried out. For each tested concentration and the control with no toxicant, 15 replicates were made (three wells for each of five sampling times). Protozoan density (individuals ml−1) was estimated in three sub-samples of 25 μl according to Madoni [27]. The three sub-samples were taken after 1, 6, 12, 24 and 48 h of treatment, always from different wells.
Total and dead cell numbers were estimated by microscopic observation at 100× magnification in an inverted microscope (NIKON Diaphot 300). For each sub-sample, a drop of 25 μl was taken, as described above, and dead individuals, i.e. non-motile cells, were counted. A drop of 5 μl of formalin (4% v/v in water) was then added and the total number of organisms was counted. Estimates of mortality were determined as the percentage of total individuals.
Grazing assays were performed in 24-well tissue culture dishes, each well containing 1000 μl of PPY, with or without the toxicant, and 500 μl of inoculum of T. pyriformis. For each toxicant, three independent assays were carried out, with three replicates for each concentration tested and for the control, assuming that ciliates do not discriminate between different particles on the basis of properties other than size or shape [28]. In each well, fluorescently labelled latex beads of 0.5 μm diameter were added at a concentration of approximately 1.05×106 ml−1. Previous assays determined which size and concentration ranges enabled the best microscopic observation and the subsequent counting of grazing beads. Experiments of Sherr et al. [31] with estuarine phagotrophic ciliates showed that they ingested fluorescently labelled bacteria at a higher rate than they grazed on latex beads of similar size, leading to the conclusion that this technique may underestimate effective protozoan bacterivory. In the present study, however, precise estimation of protozoan grazing rate was not intended; the objective was to estimate the influence of toxicants on grazing activity.
For each concentration tested, a sub-sample of 50 μl was taken after 1, 10 and 20 min of incubation; 10 μl of formalin (4% v/v in water) was added and the sub-sample was stored in the dark until subsequent microscopic analysis with an epifluorescence microscope (ZEISS Axioskop). Each sub-sample was inspected at a magnification of 100×; when a ciliate was located, the incident light was changed to UV light (EX 450–490 excitation filter, FT 510 mirror and BA 520 barrier filter) and the number of beads ingested was counted at a magnification of 400×.
In each sub-sample, 30 ciliates were inspected and the total number of ingested fluorescently labelled latex beads was determined.
3 Results
Table 1 shows the nominal metal concentrations used and the corresponding concentrations of soluble metal observed after 24 h of incubation. All toxicological data refer to soluble metal concentrations.
3.1 Growth and mortality assays
The results of the two independent assays on growth and mortality are shown in Figs. 1 and 2. Mortality values correspond to the percentage of dead cells observed. For both growth and mortality of T. pyriformis, the results of the two independent assays presented the same pattern.
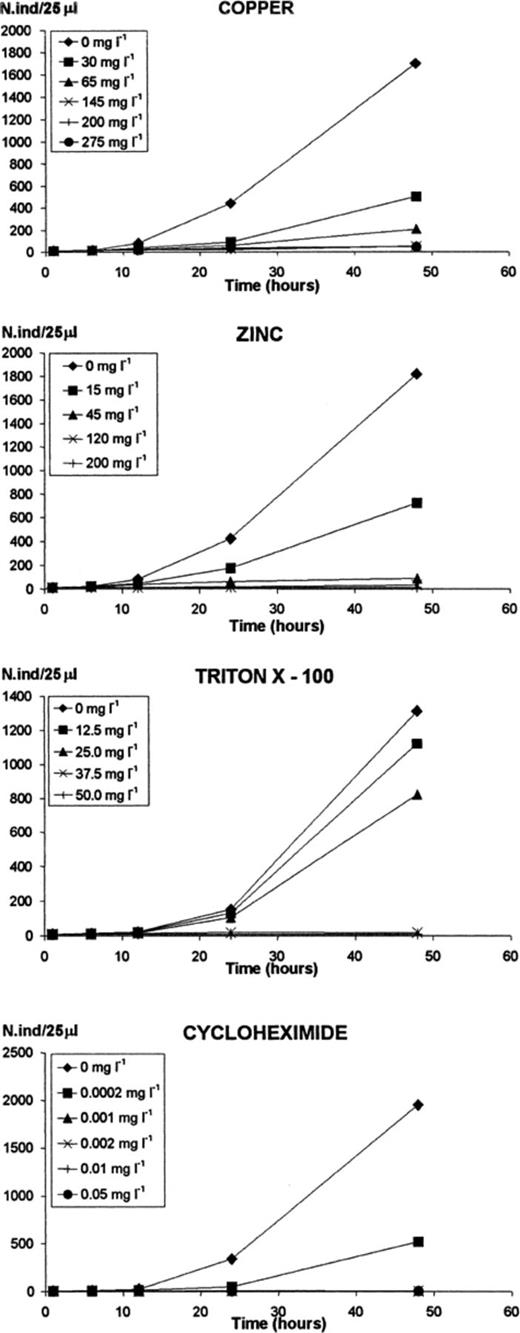
Growth of Tetrahymena pyriformis, exposed to the toxicants for 48 h, in the second of the two independent assays. The results of the first assay presented the same pattern. Values presented are the averages and standard deviations of three sub-samples.
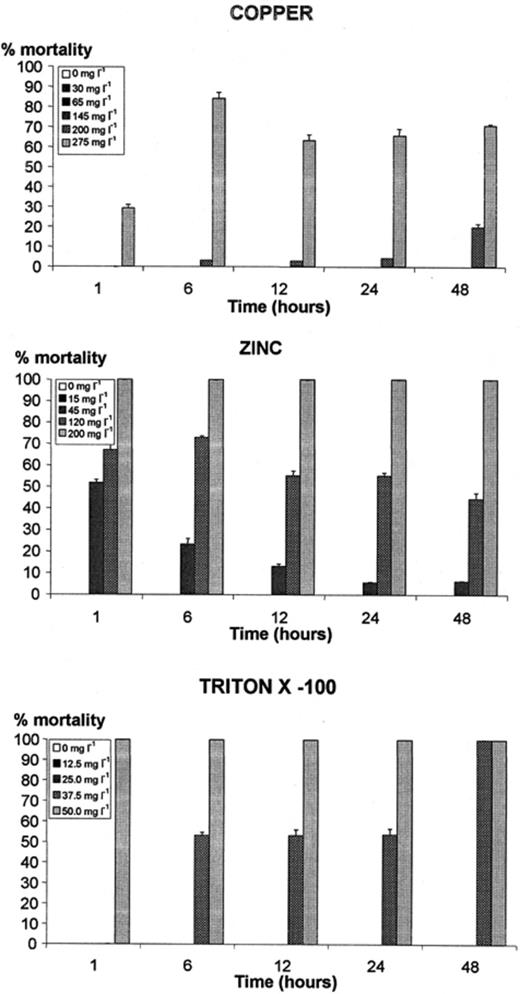
Mortality of Tetrahymena pyriformis, exposed to copper, zinc and Triton X-100 for 48 h, in the second of the two independent assays. Cycloheximide did not cause significant mortality during this period. The results of the first assay presented the same pattern. Values presented correspond to the percentages of dead cells in relation to the total number of individuals (averages and standard deviations of three sub-samples).
Copper inhibited growth significantly above 200 mg l−1 and T. pyriformis cell number increased 3-fold by 48 h in the first assay, and about 4.5-fold in the second (Fig. 1). Copper caused some mortality above 200 mg l−1, with no detectable death after 1 h of incubation and almost 20% after 48 h (Fig. 2). At 275 mg l−1, mortality reached almost 90% after 6 h of incubation, dropping to about 58% after 12 h of incubation, and then increasing slowly and reaching more than 65% (average of the two assays) at 48 h. A concentration of 145 mg l−1 caused death, though not above 1.7%.
Zinc inhibited growth almost completely above 120 mg l−1 (Fig. 1). T. pyriformis did not grow in the first assay and cell number only doubled by 48 h, in the second. Growth was also heavily affected at concentrations of 45 mg l−1 and even 15 mg l−1. Zinc caused the death of all cells after 1 h of incubation at a concentration of 200 mg l−1 (Fig. 2). Even 45 mg l−1 caused 45% of mortality at the beginning of the incubation, dropping to a minimum of 4% after 24 h. At a concentration of 15 mg l−1 of zinc, no mortality was detected.
Triton X-100 inhibited growth of T. pyriformis significantly above 37.5 mg l−1 and cell number only doubled by 48 h (Fig. 1). At a concentration of 12.5 mg l−1, it grew only slightly less than in the control. Triton X-100 caused total mortality from 1 h of incubation at a concentration of 50 mg l−1, and after 48 h at a concentration of 37.5 mg l−1. Lower concentrations did not cause detectable mortality.
Cycloheximide completely inhibited growth at concentrations above 0.001 mg l−1 and gave 75% inhibition at 0.0002 mg l−1 (Fig. 1). Cycloheximide did not cause significant mortality by 48 h.
3.2 Grazing assays
The effects of the four toxicants on grazing capacity are shown in Fig. 3. Copper inhibited grazing above 145 mg l−1, but at concentrations of 45 and 65 mg l−1, grazing capacity was stimulated. Zinc significantly inhibited grazing above 15 mg l−1. Triton X-100 inhibited grazing above 37.5 mg l−1 and, at 25.0 and 12.5 mg l−1, reduced grazing to about 50% and slightly less than 50%, respectively. In the presence of 0.01 mg l−1 of cycloheximide, inhibition was only slight, but at 0.50 mg l−1 grazing was about half the grazing in control conditions. Above this level, inhibition was greater but never complete, in the range of concentrations tested.
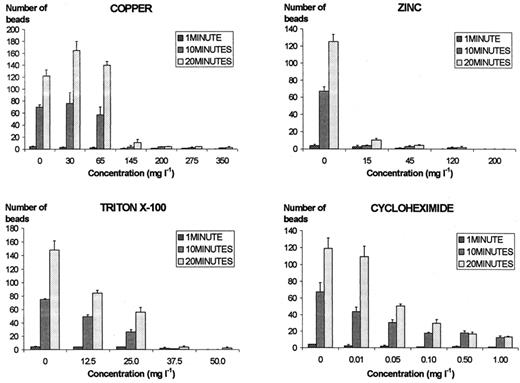
Number of beads ingested by 30 individuals of Tetrahymena pyriformis exposed to different concentrations of toxicants for 20 min. Values presented are the averages and standard deviations of three independent assays.
4 Discussion
Copper affected T. pyriformis above a concentrations of 200 mg l−1 with 3- or 4.5-fold increases in cell number in 48 h, mortality of almost 20% and significant inhibition of grazing. At a concentration of 275 mg l−1, the drop of mortality from almost 90% at 6 h of incubation to less than 60% at 12 h of incubation seems to indicate adaptation of T. pyriformis after an initial shock, but mortality then increased slowly but steadily until the end of the 48 h period. In contrast, at a concentration of 200 mg l−1, mortality constantly increased from 6 h of incubation until the end of the experiment. At a concentration of 145 mg l−1, there was almost no mortality, but growth and grazing were still heavily inhibited. Lower concentrations inhibited growth but stimulated grazing activity and caused no mortality. Consistently lower growth in the first assay is perhaps explained by higher cell densities.
Zinc caused inhibition of growth and grazing and high mortality of T. pyriformis above 45 mg l−1. In all cases, except for 200 mg l−1 where all the individuals died within the first hour of incubation, mortality decreased throughout the 48 h period, indicating a true adaptation of T. pyriformis to zinc, in contrast to copper. Acclimatisation of microorganisms to heavy metals is described by several authors [8,9,20,21,23] and may result from replacement of damaged enzymes by others or by an alternate metabolic pathway. Increased amounts of toxicant will cause more damage and the system may not recover [8,9]. At a concentration of 15 mg l−1, zinc inhibited growth by more than 50% and inhibited grazing capacity almost completely, but did not cause mortality.
The data indicate that zinc is more toxic than copper for T. pyriformis, under the conditions tested, in contrast to other studies, where copper is systematically considered more toxic than zinc to protozoa [15–17], to bacterial communities of activated sludge [29] or to algae. Madoni et al. [16] define the sequence Cu>Hg>Cd>Pb>Cr>Zn to order the toxicity of heavy metals to a series of species of protozoa taken from a community of activated sludge tested in vitro and not fed during the tests. They define a slightly different sequence for the toxicity of metals to a community of protozoa studied as a whole under field conditions: Cd, Cu>Pb>Zn>Cr [17]. In the present study, T. pyriformis was cultured as the sole species in a nutrient rich medium and the tests were performed under the same conditions, without additional stress from starvation, competition for food or change of environmental conditions. This may explain partially the great differences in the concentrations that proved to be toxic to the organisms involved. Protection of organic growth medium towards toxic effects of heavy metals on Tetrahymena is ascribed to the binding or chelating of heavy metals to organic compounds, thus leaving little in ionic form. This would explain the 100-fold difference in the concentration of copper tolerated by cells in the presence and absence of organic matter [21,23]. Results of dosing after dialysis (Table 1) seem to indicate that this may not be the only cause and toxicity will depend also on the physiological state of the organisms. Nilsson [20] reports that cells forming granules are resistant to higher concentrations of copper and states that these granules can be regarded as structures involved in detoxification or metal ion regulation. In the present experiment, granules were not initially looked for, and confirmation of this detoxification mechanism cannot be given. Madoni et al. [17] found LC50 values of 0.31–2.05 mg l−1 of copper and 0.57–50.0 mg l−1 of zinc for protozoa in an activated sludge community. These values are even lower for cell suspensions protozoa not being fed. In the present study, LC50 was not determined but mortality around 50% in 24 h was determined for much higher values, e.g. above 200 mg l−1 copper or 45 mg l−1 zinc. Yamaguchi et al. [30] observe complete inhibition of growth of T. pyriformis in axenic cultures at 1 mM copper (approximately 63.5 mg l−1). In the present study, this occurred at 275 mg l−1, though at a concentration of 145 mg l−1 growth was reduced. In contrast, Piccinni et al. [22] observed no effect on growth following addition of 10 mg l−1 copper to axenic cultures of T. pyriformis. Nilsson [20] states that the addition of 100 mg l−1 copper (nominal concentration) to the normal 2% proteose peptone medium is tolerated by Tetrahymena and stimulates phagocytosis; cell proliferation is observed to resume after a lag period, but at a decreased rate. These results are in accordance with those of the present work.
Triton X-100 at concentrations above 37.5 mg l−1 killed T. pyriformis after 48 h of incubation, allowing it only to duplicate during this period; grazing capacity was significantly inhibited. Below this concentration, there was no mortality but growth and grazing were partially inhibited. As a surfactant, Triton X-100 disrupts all cell membranes above a certain concentration, 50 mg l−1 in the present study. At concentrations around 37.5 mg l−1, effects on individual cells varied, with growth of the more resistant cells, but long exposure times finally killed all cells. Below 25 mg l−1, Triton X-100 affected only growth and grazing. There is no other study on the toxicity of surfactants on T. pyriformis or on other ciliates, with which these results can be compared.
Cycloheximide did not cause mortality, but, above 0.05 mg l−1, inhibited growth completely and at least 50% of grazing capacity of T. pyriformis. At a concentration of 0.01 mg l−1, growth was also completely inhibited, but grazing was only slightly affected. At the highest concentration (1 mg l−1), grazing was 85% inhibited. Cycloheximide inhibits protein synthesis, which affects both growth and grazing. Roberts and Orias [22] state that 10.0 mg l−1 of cycloheximide causes complete inhibition of grow and eventual lysis of Tetrahymena cells, but do not refer to the effects of lower concentration.
Studies on the influence of different toxicants on grazing capacity of ciliates have not been made recently, but some authors [19,20] state that heavy metals at low concentrations may stimulate grazing, which, in the present study, is supported for copper but not for zinc. Additional assays were carried out to test the possible influence of such low concentrations of zinc on grazing, but at 25.0 and 12.5 mg l−1, no stimulation of grazing was observed.
Recently, the use of ciliates in toxicological tests has been investigated and their potential in standard bioassays has been demonstrated in aquatic environments [32,33] and elsewhere [34]. In this work, their importance as biological indicators of water quality is emphasised and the results obtained encourage further research on the study of patterns of responses to other kinds of toxic compounds to characterise responses to toxicants or groups of toxicants.
The grazing activity test is, by far, the least labour-intensive and time-consuming of the three performed in the present study, but even studies of mortality and/or growth do not require technical expertise or operational expenses that are required by some of the standardised bioassays carried out by regulatory agencies to determine the effects of toxic compounds on higher trophic levels such as fish. In this aspect, miniaturisation and the use of protozoa seem a good alternative to other toxicity tests.
Acknowledgements
The authors acknowledge the financial support provided by the Instituto de Biotecnologia e Química Fina (IBQF) and the Fundação para a Ciência e Tecnologia (Projecto Praxis XXI - 2/2.1/BIO/1118/95). A.N. was supported by grant BD/5080/95 from PRAXIS XXI.
References