-
PDF
- Split View
-
Views
-
Cite
Cite
Christian Zipper, Thomas Fleischmann, Hans-Peter E. Kohler, Aerobic biodegradation of chiral phenoxyalkanoic acid derivatives during incubations with activated sludge, FEMS Microbiology Ecology, Volume 29, Issue 2, June 1999, Pages 197–204, https://doi.org/10.1111/j.1574-6941.1999.tb00611.x
- Share Icon Share
Abstract
The aerobic biodegradation of racemic mixtures of five chiral phenoxyalkanoic acids was studied according to a biodegradation test that was complemented with enantiomer-specific analysis. Both enantiomers of (RS)-2-phenoxypropanoic acid, (RS)-2-(3-chlorophenoxy)propanoic acid, and (RS)-2-(4-chlorophenoxy)propanoic acid, were completely degraded within 25 days when aerobically incubated with activated sludge. During incubations of (RS)-2-phenoxypropanoic acid, the (R) enantiomer was degraded before the (S) enantiomer, whereas during incubations of (RS)-2-(3-chlorophenoxy)propanoic acid the (S) enantiomer was preferentially degraded. The (R) enantiomer of (RS)-2-(2-chlorophenoxy)propanoic acid was removed after 24 days while only 30% of the (S) enantiomer was degraded within 47 days of incubation. (RS)-2-(2,4,5-Trichlorophenoxy)propanoic acid was the most persistent of all the racemic mixtures tested. After 47 days, the concentration of the (S) enantiomer was nearly unchanged, and the concentration of (R)-2-(2,4,5-trichlorophenoxy)propanoic acid had decreased only by about 40%. The differences observed in the length of the lag phases and in the degradation rates of individual enantiomers can lead to accumulations of the more recalcitrant enantiomer in aquatic or terrestrial ecosystems.
1 Introduction
Residues of pesticides and other organic compounds are often found in groundwater and surface waters [1–3] and may dramatically affect the quality of drinking water resources. For assessing the environmental fate of mobile pollutants, it is important to know their potential for biodegradation in natural and in engineered environments such as soils and wastewater treatment plants. In standardized biodegradation tests, the extent of microbial breakdown of organic compounds is usually measured as the elimination of dissolved organic carbon (DOC) during incubation with sewage sludge [4]. In order to test and understand the microbial degradation of racemic mixtures of chiral organic compounds, the DOC measurements need to be complemented by enantiomer-specific analysis, because the length of the lag phases as well as the degradation rates may differ for the enantiomers. We have reported previously the enantioselective degradation of the chiral herbicides mecoprop [(RS)-2-(4-chloro-2-methylphenoxy)propanoic acid] and dichlorprop [(RS)-2-(2,4-dichlorophenoxy)propanoic acid] by Sphingomonas herbicidovorans MH [5,6] and upon incubation with aerobic sewage sludge [7]. Both the (R) and the (S) enantiomers were completely metabolized but the (S) enantiomers were removed from the test medium before the (R) enantiomers. Therefore, it was of interest to determine whether the (S) enantiomers of structurally related compounds were also preferentially degraded during incubations with sewage sludge. This led us to examine the biodegradation of five structural analogues of mecoprop and dichlorprop (see Fig. 1) according to a biodegradability test of the International Organization for Standardization (ISO) [4]. It is of great importance to assess the biodegradability of the enantiomers of racemic mixtures of chiral pollutants for the following reasons. (i) Many chiral pollutants are released into the environment as racemic mixtures [8,9]. (ii) One enantiomer of a racemic mixture may be rapidly and completely degraded, while the other enantiomer may be resistant to microbial attack [10,11]. This can lead to an accumulation of the more recalcitrant enantiomer in the environment [10,12]. (iii) Enantiomers may have very different biological activities as well as toxicities, a fact that is well known for pharmaceutical chemicals [8,13,14].
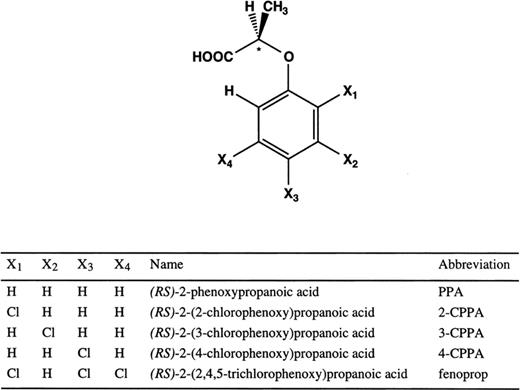
General structure, names and abbreviations of the chiral phenoxyalkanoic acids investigated in this study. An asterisk marks the stereogenic center.
All five compounds investigated are mobile and have the potential to contaminate groundwater and drinking water sources. Their physico-chemical properties are similar to those of mecoprop and 2,4-D [2,3].
2 Materials and methods
2.1 Chemicals
Racemic mixtures of 2-phenoxypropanoic acid, 2-(2-chlorophenoxy)propanoic acid, 2-(3-chlorophenoxy)propanoic acid, 2-(4-chlorophenoxy)propanoic acid, and 2-(2,4,5-trichlorophenoxy)propanoic acid (purity=97%) were purchased from Fluka (Buchs, Switzerland). HPLC-grade methanol, acetonitrile and all other chemicals used were of the highest purity commercially available and were obtained from Fluka (Buchs, Switzerland).
2.2 Sewage sludge
The sewage sludge for the degradation experiments was from the aeration tank of the municipal wastewater treatment plant Neugut, Dübendorf, Switzerland. The plant consists of mechanical and biological stages for the treatment of the wastewater from 45 000 inhabitants and some industrial sites. The sludge contained approximately 2% dry matter and had a pH of 7.2. This plant is considered to be typical of many other installations in Switzerland.
2.3 Biodegradation studies
Aerobic incubations were done according to the international standard (ISO 7827) for the evaluation of the ‘ultimate’ aerobic biodegradability of organic compounds [4]. The racemic mixtures of the test compound were added to the medium at initial concentrations of 40–45 mg l−1. The medium consisted of a phosphate buffer (pH 7.4) with additions of MgSO4, CaCl2 and FeCl3. The sewage sludge was diluted and added to the medium to give a concentration of 30 mg l−1 of suspended solids in the final mixture.
2.4 Storage and preparation of samples
Ten-ml samples from incubations of the racemic mixtures of PPA, 2-CPPA, 3-CPPA and 4-CPPA were filtered (0.45 μm, cellulose acetate filters, Nalgene, Rochester, MN, USA), collected in 25-ml teflon-stoppered glass vials and stored at −20°C until analyzed by HPLC. Samples from the experiments with (RS)-fenoprop were treated in the same way, but they were methylated prior to analysis according to the following procedure. The samples were alkalified with 100 μl of sodium hydroxide (50% v/v) and evaporated to dryness at 30°C with a gentle stream of nitrogen. After the addition of 10 ml of methanol and 250 μl of H2SO4 (50% v/v), the extracts were vigorously shaken and left overnight at room temperature and then analyzed by HPLC.
2.5 HPLC analysis
HPLC analyses were performed on a Gynkotek HPLC system with a M480G pump, a Gina 50T autosampler and a UVD340S photodiode array detector (Gynkotek GmbH, Germering, Germany). The system was operated isocratically. Samples (40 μl) were injected and the eluting compounds were detected at a wavelength of 230 nm. Three different columns were used for the enantiomer separation of the five compounds investigated (see Table 1): a Nucleodex-α-PM and a Nucleodex-β-PM column (both 200×4.0 mm, Macherey-Nagel, Düren, Germany), and a Chiralcel OD-R column (250×4.6 mm, Daicel Chemical Industries, Tokyo, Japan). The 4-CPPA enantiomers were separated on the Nucleodex-α-PM column (mobile phase: CH3OH/NaH2PO4-H3PO4 (50 mM, pH 3.0), 45:55 (v/v), flow rate: 0.7 ml min−1), whereas the enantiomers of fenoprop-methylester were resolved on the Nucleodex-β-PM column (CH3OH/NaH2PO4-H3PO4 (50 mM, pH 3.0), 70:30 (v/v), flow rate: 0.7 ml min−1). The Chiralcel OD-R column was used for the enantiomer separation of PPA, 2- and 3-CPPA (CH3CN/NaClO4-HClO4 (0.5 M, pH 2), 40:60 (v/v), flow rate: 0.5 ml min−1). The elution order of the (+) and (−) enantiomers was determined by means of a polarimetric detector (IBZ, Hannover, Germany) that was connected on-line with the HPLC system. The cell was 40 mm in length and had a volume of 300 μl. A chart strip recorder was used for recording the optical rotation. The (R) enantiomers of all compounds investigated have positive specific rotations [15, 16].
Analytical characteristics of chiral 2-phenoxypropanoic acids as determined by enantiomer-specific high-performance liquid chromatography (HPLC)
Compound | Retention time (min) | Enantiomer resolution Ra | RSDb (n=3) (%) | Column | Recovery in sewage sludge (%) |
(S)-PPA | 11.5 | 2.1 | 91.6 | ||
(R)-PPA | 15.1 | 2.4 | 2.3 | Chiralcel OD-R | 91.0 |
(S)-2-CPPA | 15.1 | 2.4 | 90.2 | ||
(R)-2-CPPA | 16.8 | 1.3 | 2.6 | Chiralcel OD-R | 90.0 |
(S)-3-CPPA | 15.5 | 1.4 | 87.7 | ||
(R)-3-CPPA | 16.9 | 1.0 | 1.8 | Chiralcel OD-R | 88.9 |
(R)-4-CPPA | 16.3 | 0.1 | 92.2 | ||
(S)-4-CPPA | 18.9 | 2.3 | 0.2 | Nucleodex α-PM | 92.4 |
(S)-fenoprop | 12.9 | 0.6 | 83.2 | ||
(R)-fenoprop | 13.9 | 1.3 | 1.7 | Nucleodex β-PM | 83.0 |
Compound | Retention time (min) | Enantiomer resolution Ra | RSDb (n=3) (%) | Column | Recovery in sewage sludge (%) |
(S)-PPA | 11.5 | 2.1 | 91.6 | ||
(R)-PPA | 15.1 | 2.4 | 2.3 | Chiralcel OD-R | 91.0 |
(S)-2-CPPA | 15.1 | 2.4 | 90.2 | ||
(R)-2-CPPA | 16.8 | 1.3 | 2.6 | Chiralcel OD-R | 90.0 |
(S)-3-CPPA | 15.5 | 1.4 | 87.7 | ||
(R)-3-CPPA | 16.9 | 1.0 | 1.8 | Chiralcel OD-R | 88.9 |
(R)-4-CPPA | 16.3 | 0.1 | 92.2 | ||
(S)-4-CPPA | 18.9 | 2.3 | 0.2 | Nucleodex α-PM | 92.4 |
(S)-fenoprop | 12.9 | 0.6 | 83.2 | ||
(R)-fenoprop | 13.9 | 1.3 | 1.7 | Nucleodex β-PM | 83.0 |
Enantiomer resolution was defined as (t2−t1)/(w1+w2); t1 and t2 are the retention times of the earlier- and the later-eluting enantiomer, respectively, and w1 and w2 are the peak widths at half-height of the earlier- and the later-eluting enantiomer, respectively.
Relative standard deviation.
Analytical characteristics of chiral 2-phenoxypropanoic acids as determined by enantiomer-specific high-performance liquid chromatography (HPLC)
Compound | Retention time (min) | Enantiomer resolution Ra | RSDb (n=3) (%) | Column | Recovery in sewage sludge (%) |
(S)-PPA | 11.5 | 2.1 | 91.6 | ||
(R)-PPA | 15.1 | 2.4 | 2.3 | Chiralcel OD-R | 91.0 |
(S)-2-CPPA | 15.1 | 2.4 | 90.2 | ||
(R)-2-CPPA | 16.8 | 1.3 | 2.6 | Chiralcel OD-R | 90.0 |
(S)-3-CPPA | 15.5 | 1.4 | 87.7 | ||
(R)-3-CPPA | 16.9 | 1.0 | 1.8 | Chiralcel OD-R | 88.9 |
(R)-4-CPPA | 16.3 | 0.1 | 92.2 | ||
(S)-4-CPPA | 18.9 | 2.3 | 0.2 | Nucleodex α-PM | 92.4 |
(S)-fenoprop | 12.9 | 0.6 | 83.2 | ||
(R)-fenoprop | 13.9 | 1.3 | 1.7 | Nucleodex β-PM | 83.0 |
Compound | Retention time (min) | Enantiomer resolution Ra | RSDb (n=3) (%) | Column | Recovery in sewage sludge (%) |
(S)-PPA | 11.5 | 2.1 | 91.6 | ||
(R)-PPA | 15.1 | 2.4 | 2.3 | Chiralcel OD-R | 91.0 |
(S)-2-CPPA | 15.1 | 2.4 | 90.2 | ||
(R)-2-CPPA | 16.8 | 1.3 | 2.6 | Chiralcel OD-R | 90.0 |
(S)-3-CPPA | 15.5 | 1.4 | 87.7 | ||
(R)-3-CPPA | 16.9 | 1.0 | 1.8 | Chiralcel OD-R | 88.9 |
(R)-4-CPPA | 16.3 | 0.1 | 92.2 | ||
(S)-4-CPPA | 18.9 | 2.3 | 0.2 | Nucleodex α-PM | 92.4 |
(S)-fenoprop | 12.9 | 0.6 | 83.2 | ||
(R)-fenoprop | 13.9 | 1.3 | 1.7 | Nucleodex β-PM | 83.0 |
Enantiomer resolution was defined as (t2−t1)/(w1+w2); t1 and t2 are the retention times of the earlier- and the later-eluting enantiomer, respectively, and w1 and w2 are the peak widths at half-height of the earlier- and the later-eluting enantiomer, respectively.
Relative standard deviation.
3 Results
3.1 Enantiomer-specific analysis by HPLC
The methods employed allowed the separation of the enantiomers of the five phenoxyalkanoic acid derivatives and were based on columns that contain a chiral selector as the stationary phase. The enantiomers of PPA, 2-, and 3-CPPA were separated on a column that contained a cellulose derivative, whereas the stereoisomers of 4-CPPA and fenoprop were resolved on a stationary phase containing permethylated cyclodextrin (Table 1). The enantiomer separation was generally good and the enantiomer resolution of the compounds ranged from 1.0 to 2.4 (Table 1). Samples of the incubations of racemic mixtures of PPA, 2-, 3-, and 4-CPPA with sewage sludge were filtered and directly injected onto the column with compound recoveries in the range of 90% (Table 1). Only fenoprop had to be methylated prior to analysis to achieve good enantiomer resolution and the recovery was slightly lower (83%, Table 1). The detection limits (0.1–0.3 mg l−1) as well as the reproducibilities of repeated injections (0.1–2.6% relative standard deviation, see Table 1) allowed precise and sensitive determinations of the enantiomers of the chiral compounds.
3.2 Biodegradation of test compounds
Both enantiomers of racemic mixtures of PPA were degraded within 12 days during aerobic incubations with sewage sludge (Fig. 2A). The degradation of the enantiomers was sequential. The (R) enantiomer was degraded at first and after a period of 7 days the (S) enantiomer was also removed from the medium (Figs. 2A,3). At the end of such experiments, 85–95% of the DOC was degraded, which indicated the complete breakdown of (R)- and (S)-PPA. Control experiments with sterilized sewage sludge showed no removal of PPA showing that the degradation was biologically mediated. During incubations of racemic mixtures of 2-CPPA with activated sludge we observed preferential breakdown of the (R) enantiomer. The degradation of the (S) enantiomer started only after complete depletion of the (R) enantiomer. At the end of such experiments (47 days), 75% of the initial amount of the (S)-2-CPPA was still present, and only 60% of the DOC was eliminated (Fig. 2B).
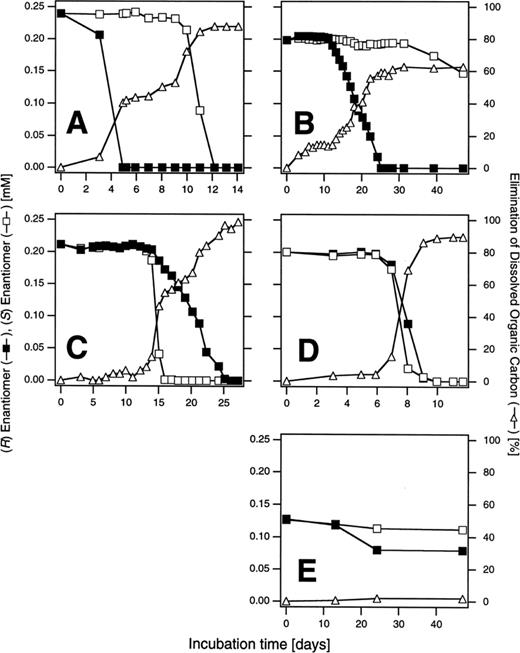
Aerobic degradation of racemic mixtures of (A) 2-phenoxypropanoic acid; (B) 2-(2-chlorophenoxy)propanoic acid; (C) 2-(3-chlorophenoxy)propanoic acid; (D) 2-(4-chlorophenoxy)propanoic acid; and (E) 2-(2,4,5-trichlorophenoxy)propanoic acid during incubations with sewage sludge from the aeration tank of a municipal waste water treatment plant at 25°C. Concentrations (mM) (left-hand axis) and elimination of dissolved organic carbon (%) (right-hand axis) are plotted versus incubation time (days).
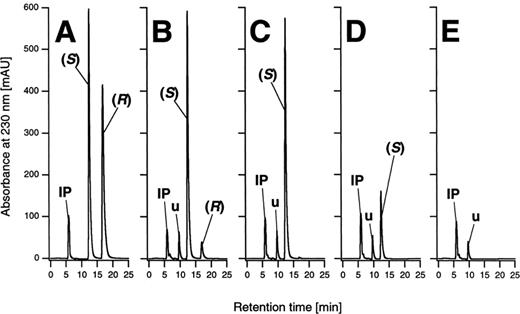
HPLC chromatograms of samples, which were taken from an aerobic degradation experiment of a racemic mixture of 2-phenoxypropanoic with sewage sludge. The (R) and (S) enantiomers were resolved on a column that contained cellulose tris(3,5-dimethylphenyl)carbamate as the chiral selector. Chromatograms represent samples taken at: (A) the start of the experiment; (B) 4.5 days; (C) 7.5 days; (D) 11 days; (E) 12 days. Designation of peaks: IP, injection peak; u, unidentified peak; (S), (S)-2-phenoxypropanoic acid; (R), (R)-2-phenoxypropanoic acid.
The breakdown of the enantiomers of meta and para chlorinated 2-phenoxypropanoic acids was also biologically mediated. But, in contrast to the sequential biodegradation of the enantiomers of PPA and 2-CPPA (Fig. 2A,B), we observed the concomitant removal of the 3- and 4-CPPA enantiomers (Fig. 2C,D) with (S)-3-CPPA being faster degraded than (R)-3-CPPA (Fig. 2C). The specific degradation rates for the 4-CPPA enantiomers were nearly identical (Table 2,Fig. 2D).
Lag phase and maximum degradation rate for the degradation of several phenoxyalkanoic acids incubated with activated sludge from a municipal wastewater treatment plant
Compound | Lag phasea (days) | Maximum degradation rate (μmol h−1 (g dry weight)−1) |
(R)-PPA | 2 | 140–160 |
(S)-PPA | 10 | 140–160 |
(R)-2-CPPA | 12 | 20 |
(S)-2-CPPA | 32 | 4b |
(R)-3-CPPA | 16 | 12 |
(S)-3-CPPA | 16 | 18 |
(R)-4-CPPA | 6 | 100–120 |
(S)-4-CPPA | 6 | 100–120 |
(R)-fenoprop | 10 | 6c |
(S)-fenoprop | 10 | <1 |
Compound | Lag phasea (days) | Maximum degradation rate (μmol h−1 (g dry weight)−1) |
(R)-PPA | 2 | 140–160 |
(S)-PPA | 10 | 140–160 |
(R)-2-CPPA | 12 | 20 |
(S)-2-CPPA | 32 | 4b |
(R)-3-CPPA | 16 | 12 |
(S)-3-CPPA | 16 | 18 |
(R)-4-CPPA | 6 | 100–120 |
(S)-4-CPPA | 6 | 100–120 |
(R)-fenoprop | 10 | 6c |
(S)-fenoprop | 10 | <1 |
The lag phase was defined as the time interval from the start of the incubation to the time point at which the substrate concentration decreased to 95%.
The microbial degradation of the (S)-2-CPPA was not completed at the end of the experiment (47 days). The degradation rate listed is the maximum (S)-2-CPPA degradation rate that was achieved during the experiment.
The degradation rate of (R)-fenoprop was determined from two measurements only. After 24 days of incubation, degradation of (R)-fenoprop did not continue (Fig. 2E).
Lag phase and maximum degradation rate for the degradation of several phenoxyalkanoic acids incubated with activated sludge from a municipal wastewater treatment plant
Compound | Lag phasea (days) | Maximum degradation rate (μmol h−1 (g dry weight)−1) |
(R)-PPA | 2 | 140–160 |
(S)-PPA | 10 | 140–160 |
(R)-2-CPPA | 12 | 20 |
(S)-2-CPPA | 32 | 4b |
(R)-3-CPPA | 16 | 12 |
(S)-3-CPPA | 16 | 18 |
(R)-4-CPPA | 6 | 100–120 |
(S)-4-CPPA | 6 | 100–120 |
(R)-fenoprop | 10 | 6c |
(S)-fenoprop | 10 | <1 |
Compound | Lag phasea (days) | Maximum degradation rate (μmol h−1 (g dry weight)−1) |
(R)-PPA | 2 | 140–160 |
(S)-PPA | 10 | 140–160 |
(R)-2-CPPA | 12 | 20 |
(S)-2-CPPA | 32 | 4b |
(R)-3-CPPA | 16 | 12 |
(S)-3-CPPA | 16 | 18 |
(R)-4-CPPA | 6 | 100–120 |
(S)-4-CPPA | 6 | 100–120 |
(R)-fenoprop | 10 | 6c |
(S)-fenoprop | 10 | <1 |
The lag phase was defined as the time interval from the start of the incubation to the time point at which the substrate concentration decreased to 95%.
The microbial degradation of the (S)-2-CPPA was not completed at the end of the experiment (47 days). The degradation rate listed is the maximum (S)-2-CPPA degradation rate that was achieved during the experiment.
The degradation rate of (R)-fenoprop was determined from two measurements only. After 24 days of incubation, degradation of (R)-fenoprop did not continue (Fig. 2E).
Fenoprop was the most persistent of all the tested compounds. After 47 days of aerobic incubation, the concentration of the (S) enantiomer was nearly unchanged, and the concentration of the (R) enantiomer had decreased only by about 40%. The DOC was eliminated by only 2% (Fig. 2E) and not by 20% as was expected from the 40% decrease of (R)-fenoprop. This discrepancy may be due to incomplete degradation resulting in the formation of metabolites.
4 Discussion
In this report, we present the results of aerobic biodegradation studies with five chiral phenoxyalkanoic acids. The experiments were performed according to a DOC biodegradability test that was complemented with enantiomer-selective HPLC to assess the fate of the enantiomers of the racemic mixtures incubated with sewage sludge. The removal of the enantiomers of four racemic mixtures differed with respect to the duration of the lag phases and with respect to the values of the specific degradation rates (Table 2). Such differences in degradation characteristics can lead to accumulation of the more recalcitrant enantiomers in aquatic and terrestrial ecosystems. Furthermore, accumulation of enantiomers may increase the toxicity of enantiomer mixtures, due to different toxicities of the single enantiomers.
Enantioselective biodegradation of stereoisomers is based on (i) the selective uptake of enantiomers by microorganisms as reported for the xylose enantiomers [17] and for (R)- and (S)-dichlorprop [6]; (ii) the selective intracellular metabolism of stereoisomers as demonstrated for the initial metabolism of (R)- and (S)-mecoprop by soil isolates [18,19]; (iii) different rates of biologically mediated enantiomerization for the (R) and the (S) enantiomers as shown for the stereoisomers of mecoprop and dichlorprop in soil [20,21]. Extracellular enantiomerization could be excluded in our study since the concentrations of (S)-PPA, (S)-2-CPPA, and (S)-fenoprop did not increase during biodegradation of (R)-PPA, (R)-2-CPPA, and (R)-fenoprop (Fig. 2A,B,E). Enantiomerization of 3- and 4-CPPA cannot be excluded because the enantiomers of these racemic mixtures were concomitantly biodegraded.
The (S) enantiomers of mecoprop and dichlorprop, structural analogues of the compounds investigated in this study, are biodegraded before or faster than the corresponding (R) enantiomers in soil and sewage sludge [5,7,20,22]. In one of the studies [7], it was shown that sewage sludge that was acclimatized to pure (R)-mecoprop preferentially degraded the (R) but not the (S) enantiomer within 20 h of incubation; this indicated a selective enrichment of specific populations or regulatory changes in specific strains. In the present study microorganisms in sewage sludge preferentially degraded the (S) enantiomer of 3-CPPA but preferred the (R) enantiomers of PPA, 2-CPPA and fenoprop. These results suggest that the presence and the position of chlorine atoms on the aromatic ring of 2-phenoxypropanoic acids influence which enantiomer is preferentially biodegraded. Due to the unavailability of pure enantiomers of the compounds tested here, acclimatization studies could not be performed.
Our study demonstrated that the fate of each enantiomer must be investigated when testing the biodegradability of racemic mixtures. This can be done by complementing well-established biodegradability tests with enantiomer-specific analysis, which is available for many chiral compounds [23]. Furthermore, this study showed that, with respect to enantiomer preference, the biodegradation of a chiral compound might differ from the biodegradation of a chiral analogue. This indicates that structural analogues need to be tested separately to assess the biodegradability of their enantiomers.
Acknowledgements
We thank Mary Power, Markus D. Müller, Sol Resnick and Stefano F. Simoni for critically reading the manuscript.
References
Author notes
Present address: BMG Engineering, Ifangstrasse 11, CH-8952 Schlieren, Switzerland.