-
PDF
- Split View
-
Views
-
Cite
Cite
M Fischer-Le Saux, E Arteaga-Hernández, Z Mrácek, N.E Boemare, The bacterial symbiont Xenorhabdus poinarii (Enterobacteriaceae) is harbored by two phylogenetic related host nematodes: the entomopathogenic species Steinernema cubanum and Steinernema glaseri (Nematoda: Steinernematidae), FEMS Microbiology Ecology, Volume 29, Issue 2, June 1999, Pages 149–157, https://doi.org/10.1111/j.1574-6941.1999.tb00606.x
- Share Icon Share
Abstract
Xenorhabdus symbionts were isolated from infective juveniles of Steinernema cubanum, an entomopathogenic nematode isolated in western Cuba. A polyphasic approach, including phenotypic tests, restriction polymorphism analysis of PCR-amplified 16S rRNA genes and DNA-DNA hybridizations with determination of the ΔTm, was used to characterize this Xenorhabdus isolate. All methods converged to the conclusion that the isolate was a strain of Xenorhabdus poinarii. Until today, this species was only isolated from Steinernema glaseri and was considered to be specific to this nematode species. From the closely phylogenetic relatedness of S. cubanum and S. glaseri, which is supported by morphological and genotypic similarities, one can assume that the divergence of these two species is relatively recent. The speciation of the respective symbiotic bacteria may be in course, but at the present time, phenotypic and genotypic divergence is not sufficient to delineate two Xenorhabdus species.
1 Introduction
Xenorhabdus species are Gram-negative enterobacteria, symbiotically associated to entomopathogenic nematodes of the family Steinernematidae that are used in the biological control of insect pests. In fundamental terms, the symbiosis between both partners is a fascinating model of co-speciation. The bacteria are carried monoxenically in a differentiated vesicle [1] within the gut of infective juveniles (IJs) and are released into the insect hemocoel after the nematode invasion. Death of the insect occurs within 48 h. The bacteria multiply, establishing favorable conditions for nematode reproduction by providing nutrients and inhibiting growth of other microorganisms [2]. The invading nematodes reproduce within the prey cadaver producing new infective juveniles which escape from the cadaver to infect new hosts. Both symbiotic partners can be cultivated separately: on one hand Steinernematidae are reared axenically on an artificial diet, on the other hand pure cultures of Xenorhabdus bacteria grow on nutrient media. Thereby, gnotobiological experiments have demonstrated in most cases that nematode reproduction is optimal with the native symbiont. In fact, taxonomic studies of symbionts and their host nematodes have defined that almost each species of Steinernema possesses a specific symbiont species. Xenorhabdus nematophilus is the symbiont species of Steinernema carpocapsae, Xenorhabdus poinarii of Steinernema glaseri, Xenorhabdus beddingii of another unnamed Steinernema species [2] and Xenorhabdus japonicus of Steinernema kushidai [3]. Xenorhabdus bovienii is the only species associated with several host species: Steinernema affine, Steinernema intermedium, Steinernema kraussei and Steinernema feltiae. However, recent restriction fragment length polymorphism (RFLP) analysis of PCR-amplified 16S rRNA genes (rDNA) have evidenced genetic polymorphism within this species, each genotype corresponding more or less to one nematode species [4,5].
A novel species of Steinernema [6] was described in Cuba as Steinernema cubanum. Its bacterial symbiont was isolated and preliminary phenotypic characterization [6] has indicated a possible new Xenorhabdus species. However the bacterial strain isolated presented unusual properties for a Xenorhabdus strain as it exhibited catalase and nitrate reductase activities. Usually Xenorhabdus strains are considered as atypical members of Enterobacteriaceae because they are devoid of catalase activity and do not reduce nitrate to nitrite [7]. More detailed phenotypic and genotypic data were needed to accurately characterize the symbiont of S. cubanum.
Isolation of the true symbiont of Steinernema nematodes is a critical step which needs full attention [8]. We re-isolated the bacterial symbiont from IJs of S. cubanum and identified the isolates by using a RFLP analysis of their PCR-amplified 16S rDNA together with reference strains. We found that the S. cubanum symbiont had the same RFLP genotype as the X. poinarii type strain. To confirm this result, a complete phenotypic characterization and DNA-DNA hybridizations were undertaken. So far, X. poinarii species has only been isolated from S. glaseri. Its occurrence in another Steinernema species is discussed through biogeographical and phylogenetic inferences.
2 Materials and methods
2.1 Steinernema cubanum culture
S. cubanum was isolated by E. Arteaga in western Cuba in 1990 and its rearing has been established in the nematode collection of the Institut National de la Recherche Agronomique (INRA) at Antibes (France). Nematodes used for our experiments were multiplied on the greater wax moth larvae, Galleria mellonella.
2.2 Bacterial isolation and reference strains
The bacterial symbiont of S. cubanum was isolated from infective stages by the hanging drop technique [9]. The origin of the reference strains used in this study is listed in Table 1. All Xenorhabdus strains were cultivated at 28°C on nutrient agar supplemented with 0.004% (w/v) triphenyltetrazolium chloride and 0.0025% (w/v) bromothymol blue (NBTA medium), in order to control the presence of phase I and phase II variants [10].
Strain designationa | Host nematode origin | Geographical origin | Sourceb | 16S rDNA genotypec |
Isolate studied | ||||
CU01 | S. cubanum | Cuba | E. Arteaga | 3 |
Xenorhabdus nematophilus | ||||
AN6 (ATCC 19061)T | S. carpocapsae | USA (Georgia) | R. Akhurst | 1 |
A24 | S. carpocapsae | Russia | R. Akhurst | 2 |
F1 | S. carpocapsae | France | E. Bonifassi | 2 |
Xenorhabdus poinarii | ||||
G6 (ATCC 49121)T | S. glaseri | USA (North Carolina) | R. Akhurst | 3 |
NC33 | S. glaseri | USA (North Carolina) | R. Akhurst | 3 |
SK72 | S. glaseri | USA (Florida) | N. Simões | 3 |
Xenorhabdus beddingii | ||||
Q58 (UQM 2872)T | Steinernema sp.d | Australia (Queensland) | R. Akhurst | 5 |
Xenorhabdus bovienii | ||||
T228 (UQM 2211)T | S. feltiae | Australia (Tasmania) | R. Akhurst | 6 |
USFL52 | S. feltiae | USA (Florida) | G. Smart | 6 |
Si | S. intermedium | USA (South Carolina) | R. Akhurst | 7 |
F3 | S. affine | France | E. Bonifassi | 8 |
SK2 | S. kraussei | Czech Republic | Z. Mrácek | 8 |
F5 | S. feltiae | France | E. Bonifassi | 8 |
Xenorhabdus japonicus | ||||
JP02 | S. kushidai | Japan | N. Ishibashi | 18 |
Xenorhabdus sp. | ||||
SaV | S. arenarium | Russia | R. Akhurst | 9 |
Other Enterobacteriaceae | ||||
Proteus vulgaris CIP 58.60T | Institut Pasteur, Paris | 31 |
Strain designationa | Host nematode origin | Geographical origin | Sourceb | 16S rDNA genotypec |
Isolate studied | ||||
CU01 | S. cubanum | Cuba | E. Arteaga | 3 |
Xenorhabdus nematophilus | ||||
AN6 (ATCC 19061)T | S. carpocapsae | USA (Georgia) | R. Akhurst | 1 |
A24 | S. carpocapsae | Russia | R. Akhurst | 2 |
F1 | S. carpocapsae | France | E. Bonifassi | 2 |
Xenorhabdus poinarii | ||||
G6 (ATCC 49121)T | S. glaseri | USA (North Carolina) | R. Akhurst | 3 |
NC33 | S. glaseri | USA (North Carolina) | R. Akhurst | 3 |
SK72 | S. glaseri | USA (Florida) | N. Simões | 3 |
Xenorhabdus beddingii | ||||
Q58 (UQM 2872)T | Steinernema sp.d | Australia (Queensland) | R. Akhurst | 5 |
Xenorhabdus bovienii | ||||
T228 (UQM 2211)T | S. feltiae | Australia (Tasmania) | R. Akhurst | 6 |
USFL52 | S. feltiae | USA (Florida) | G. Smart | 6 |
Si | S. intermedium | USA (South Carolina) | R. Akhurst | 7 |
F3 | S. affine | France | E. Bonifassi | 8 |
SK2 | S. kraussei | Czech Republic | Z. Mrácek | 8 |
F5 | S. feltiae | France | E. Bonifassi | 8 |
Xenorhabdus japonicus | ||||
JP02 | S. kushidai | Japan | N. Ishibashi | 18 |
Xenorhabdus sp. | ||||
SaV | S. arenarium | Russia | R. Akhurst | 9 |
Other Enterobacteriaceae | ||||
Proteus vulgaris CIP 58.60T | Institut Pasteur, Paris | 31 |
Names are given according to the usual nomenclature of the strains as described in previous works, except for the new designations which indicate first the international code of the home country followed by a number (CU=Cuba; JP=Japan; USFL=USA, Florida).
Abbreviations: ATCC, American Type Culture Collection (Rockville, MD); CIP, Collection de l'Institut Pasteur (Paris, France); UQM, Culture Collection of the University of Queensland, Department of Microbiology (Brisbane, Australia); T, type strain.
For one part, the symbiont cultures were provided by: Dr E. Arteaga, Estación de Sanidad de los Cítricos, Ministerio de Agricultura, Ciudad Habana, Cuba; Dr R. Akhurst, CSIRO, Division of Entomology, Canberra, ACT 2601 Canberra, Australia; Dr E. Bonifassi, INRA, Laboratoire de Biologie des Invertébrés, 06606 Antibes Cedex, France. For the other part, the symbiont strains were isolated by the Montpellier laboratory from the nematodes provided by: Prof. N. Simões, Universidade dos Açores, 9502 Ponta Delgada Codex, Portugal; Prof. G. Smart, University of Florida, Nematology Department PoBox 1106, Building 970, Hull Road, FL 32611-0620, Gainesville, FL, USA; Dr Z. Mrácek, Institute of Entomology, Branisovská, 31, 37005 Ceske Budejovice, Czech Republic; Prof. N. Ishibashi, University of Saga, Konjocho-1, 840 Saga, Japan.
Numbering of the 16S rDNA genotypes was previously defined [5].
New species of Steinernema not yet described.
Strain designationa | Host nematode origin | Geographical origin | Sourceb | 16S rDNA genotypec |
Isolate studied | ||||
CU01 | S. cubanum | Cuba | E. Arteaga | 3 |
Xenorhabdus nematophilus | ||||
AN6 (ATCC 19061)T | S. carpocapsae | USA (Georgia) | R. Akhurst | 1 |
A24 | S. carpocapsae | Russia | R. Akhurst | 2 |
F1 | S. carpocapsae | France | E. Bonifassi | 2 |
Xenorhabdus poinarii | ||||
G6 (ATCC 49121)T | S. glaseri | USA (North Carolina) | R. Akhurst | 3 |
NC33 | S. glaseri | USA (North Carolina) | R. Akhurst | 3 |
SK72 | S. glaseri | USA (Florida) | N. Simões | 3 |
Xenorhabdus beddingii | ||||
Q58 (UQM 2872)T | Steinernema sp.d | Australia (Queensland) | R. Akhurst | 5 |
Xenorhabdus bovienii | ||||
T228 (UQM 2211)T | S. feltiae | Australia (Tasmania) | R. Akhurst | 6 |
USFL52 | S. feltiae | USA (Florida) | G. Smart | 6 |
Si | S. intermedium | USA (South Carolina) | R. Akhurst | 7 |
F3 | S. affine | France | E. Bonifassi | 8 |
SK2 | S. kraussei | Czech Republic | Z. Mrácek | 8 |
F5 | S. feltiae | France | E. Bonifassi | 8 |
Xenorhabdus japonicus | ||||
JP02 | S. kushidai | Japan | N. Ishibashi | 18 |
Xenorhabdus sp. | ||||
SaV | S. arenarium | Russia | R. Akhurst | 9 |
Other Enterobacteriaceae | ||||
Proteus vulgaris CIP 58.60T | Institut Pasteur, Paris | 31 |
Strain designationa | Host nematode origin | Geographical origin | Sourceb | 16S rDNA genotypec |
Isolate studied | ||||
CU01 | S. cubanum | Cuba | E. Arteaga | 3 |
Xenorhabdus nematophilus | ||||
AN6 (ATCC 19061)T | S. carpocapsae | USA (Georgia) | R. Akhurst | 1 |
A24 | S. carpocapsae | Russia | R. Akhurst | 2 |
F1 | S. carpocapsae | France | E. Bonifassi | 2 |
Xenorhabdus poinarii | ||||
G6 (ATCC 49121)T | S. glaseri | USA (North Carolina) | R. Akhurst | 3 |
NC33 | S. glaseri | USA (North Carolina) | R. Akhurst | 3 |
SK72 | S. glaseri | USA (Florida) | N. Simões | 3 |
Xenorhabdus beddingii | ||||
Q58 (UQM 2872)T | Steinernema sp.d | Australia (Queensland) | R. Akhurst | 5 |
Xenorhabdus bovienii | ||||
T228 (UQM 2211)T | S. feltiae | Australia (Tasmania) | R. Akhurst | 6 |
USFL52 | S. feltiae | USA (Florida) | G. Smart | 6 |
Si | S. intermedium | USA (South Carolina) | R. Akhurst | 7 |
F3 | S. affine | France | E. Bonifassi | 8 |
SK2 | S. kraussei | Czech Republic | Z. Mrácek | 8 |
F5 | S. feltiae | France | E. Bonifassi | 8 |
Xenorhabdus japonicus | ||||
JP02 | S. kushidai | Japan | N. Ishibashi | 18 |
Xenorhabdus sp. | ||||
SaV | S. arenarium | Russia | R. Akhurst | 9 |
Other Enterobacteriaceae | ||||
Proteus vulgaris CIP 58.60T | Institut Pasteur, Paris | 31 |
Names are given according to the usual nomenclature of the strains as described in previous works, except for the new designations which indicate first the international code of the home country followed by a number (CU=Cuba; JP=Japan; USFL=USA, Florida).
Abbreviations: ATCC, American Type Culture Collection (Rockville, MD); CIP, Collection de l'Institut Pasteur (Paris, France); UQM, Culture Collection of the University of Queensland, Department of Microbiology (Brisbane, Australia); T, type strain.
For one part, the symbiont cultures were provided by: Dr E. Arteaga, Estación de Sanidad de los Cítricos, Ministerio de Agricultura, Ciudad Habana, Cuba; Dr R. Akhurst, CSIRO, Division of Entomology, Canberra, ACT 2601 Canberra, Australia; Dr E. Bonifassi, INRA, Laboratoire de Biologie des Invertébrés, 06606 Antibes Cedex, France. For the other part, the symbiont strains were isolated by the Montpellier laboratory from the nematodes provided by: Prof. N. Simões, Universidade dos Açores, 9502 Ponta Delgada Codex, Portugal; Prof. G. Smart, University of Florida, Nematology Department PoBox 1106, Building 970, Hull Road, FL 32611-0620, Gainesville, FL, USA; Dr Z. Mrácek, Institute of Entomology, Branisovská, 31, 37005 Ceske Budejovice, Czech Republic; Prof. N. Ishibashi, University of Saga, Konjocho-1, 840 Saga, Japan.
Numbering of the 16S rDNA genotypes was previously defined [5].
New species of Steinernema not yet described.
2.3 PCR amplification and RFLP analysis
DNA extractions were performed according to a rapid protocol using the nucleic acid extraction kit Isoquick (ORCA Research, Bothell, USA) [5]. 16S rDNA of each strain was amplified by PCR under conditions previously described [4]. The following two Eubacteria universal primers were used: 5′-GAAGAGTTTGATCATGGCTC-3′ and 5′-AGGAGGTGATCCAGCCGCA-3′[11]. Samples of 6–17 μl of the amplified products were digested separately overnight with 5 U of the six following restriction enzymes: AluI, CfoI, DdeI, HaeIII, HinfI and MspI. Restriction fragments were separated by horizontal electrophoresis at 6 V cm−1 in 3% (w/v) Nusieve or Metaphor agarose gels (Tebu, Le Perray en Yvelines, France) containing 0.5 mg of ethidium bromide per l. Genetic relationships between each pair of strains was evaluated by the presence or absence of DNA restriction fragments of a given length. Dice coefficients were calculated, then genetic distances were estimated by the mathematical model previously defined [12,13] and displayed as a dendrogram using the unweighted pair group method with arithmetic mean (UPGMA).
2.4 DNA hybridizations and thermal stability of duplexes
Total DNA for DNA-DNA hybridizations experiments was extracted from pure cultures of the CU01, NC33 and G6 strains according to the protocol of Brenner et al. [14]. Total DNA from the type strain G6 was labelled in vitro by nick translation using tritium-labelled nucleotides (Amersham International, Amersham, UK). Levels of DNA relatedness were determined by using the S1 nuclease-trichloracetic acid method [15] at the optimal temperature for DNA reassociation (65°C) for 16 h. Each reaction was carried out in duplicate and values obtained were averaged. The 0% control hybridization was obtained from re-association of the labelled DNA with herring sperm DNA and the 100% control from homologous hybridization. Heterologous hybridization values were normalized using 0% and 100% controls, to determine the percentage of relative DNA binding between two bacterial strains.
The thermal stability of re-associated DNAs was estimated by determining the denaturation temperature (Tm, temperature at which 50% of the double-stranded DNA was denatured and lysed by S1 nuclease). The Tm was determined by using the method previously described [16] with the following slight modifications. The temperature of the hybridization mixture was increased from 65°C to 95°C in 5°C increments. The hybridization mixture was not diluted with distilled water (final NaCl concentration 0.42 M) after DNA hybridization was completed. The divergence between DNAs was estimated by calculating the ΔTm value (difference between Tm of homologous and heterologous duplexes).
2.5 Phenotypic characterization
Phenotypic tests were conducted at 28°C by using the same methods as Boemare and Akhurst [10] except for the following tests. Acidification of carbohydrates was determined on API 50 CH strips with API 50 CHE medium (BioMérieux, Craponne, France), using phenol red as pH indicator. Utilization of carbohydrates and of organic and amino acid compounds was tested either on API 50 CH, 50 AO or 50 AA strips with API LRA medium, or on Biotype 100 by using the minimal medium II (BioMérieux). The maximum growth temperature for each strain was determined by inoculating cultures in nutrient broth maintained at a calibrated temperature in a water bath electronically adjusted at 0.1°C (Julabo, Germany) and monitored with an Hg thermometer (graduations 1/20 per °C). Temperatures ranging from 30 to 42°C by 1°C increments were tested. To avoid any pre-selection from the original strains, the inocula for these tests were taken from cultures maintained at 28°C.
The phenotypic relationships among strains were determined by performing a factor analysis using the software Statlab (SLP®, Paris, France) taking into consideration only the physiological and biochemical characters scored as variable.
The pathogenicity of the isolate CU01 was evaluated against Spodoptera littoralis larvae. Bacteria for injection experiments were grown overnight in nutrient broth. Different bacterial cell concentrations were obtained by dilution in sterile nutrient broth. Colony forming units (CFUs) of dilutions were assessed by inoculation of 100 μl on nutrient agar in three Petri dishes allowing to calculate the average of CFUs/ml of each dilution. To test the pathogenicity of the bacteria, 20 last instar larvae of Spodoptera littoralis were inoculated with 20 μl of each dilution, respectively, and incubated at 23°C with food. Then, the lethal dose to kill 50% of the injected insects (LD50) was estimated.
3 Results and discussion
3.1 Isolation of S. cubanum symbiont
Only one type of individual bacterial colonies was isolated from the infective stages of S. cubanum by the hanging drop technique. This isolate shared the common phenotypic properties with all Xenorhabdus reference strains and therefore belonged to the Xenorhabdus genus (see Section 3.3): it was named strain CU01. In contrast with previous reported data [6], the bacterial symbiont isolated from S. cubanum was devoid of catalase and nitrate reductase activities as most of the Xenorhabdus strains are. It seems that the bacterium isolated previously from S. cubanum was not the Xenorhabdus symbiont but a contaminant. The occurrence of such bacteria is often associated to extended storage periods in a laboratory [17–19]. Another possible explanation is an inadequate disinfection of the nematode tegument microflora before symbiont isolation.
3.2 RFLP analysis of the 16S rDNA
In order to define the phylogenetic position of the Xenorhabdus strain isolated from S. cubanum within the Xenorhabdus genus, a PCR-RFLP study of the 16S rDNA was undertaken with several representatives of each defined species. Proteus vulgaris was included as an outgroup.
PCR amplification of the 16S rDNA of the isolate CU01 with the universal ribosomal primers produced a single band of about 1600 bp as for all the Xenorhabdus strains. The restriction patterns obtained with each of the six endonucleases tested, respectively, were identical to those of the three tested X. poinarii strains SK72, G6 and NC33. This combination of patterns was assigned to genotype 3. The restriction patterns obtained with each endonuclease for all the strains studied are shown in Fischer-Le Saux et al. [5] and in Brunel et al. [4].
In all, by combining the restriction patterns for the six endonucleases used, the 16 Xenorhabdus strains studied could be grouped into nine different 16S rDNA genotypes. Genetic distances between the 16S rDNA genotypes were calculated and displayed as a dendrogram by using the UPGMA algorithm (Fig. 1). Representatives of each Xenorhabdus species formed a separate cluster, clearly delineated from other Xenorhabdus strains. The nearest defined species to X. poinarii, which included the isolate CU01, was X. beddingii. Complete sequence analysis of the 16S rDNA corroborated this latter result [20].
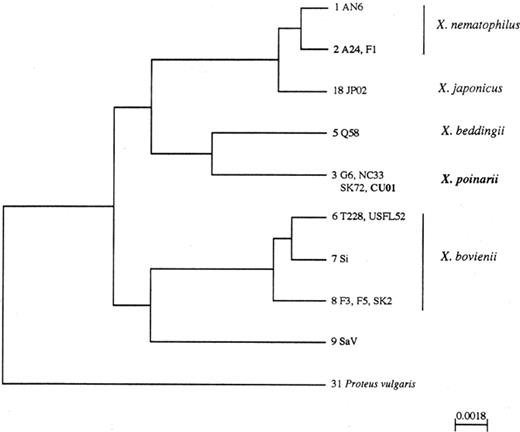
Phylogenetic tree of the Xenorhabdus species inferred from PCR-RFLP analysis of the 16S rDNAs and constructed by the UPGMA method. The genotype number is followed by the name of the strains exhibiting this genotype. Scale bar=substitution per site.
As S. glaseri and S. arenarium belong to the same morphological group [21] and are phylogenetically related as determined by a RFLP analysis of their internal transcribed spacer of the ribosomal DNA repeat unit [22], we investigated the phylogenetic position of their respective symbionts. The bacterial symbiont of S. arenarium, SaV, possessed a specific genotype that did not cluster with any defined species (Fig. 1). Thereby, we found that the symbiotic bacteria of S. arenarium and S. glaseri, respectively, are not phylogenetically closely related within the Xenorhabdus genus.
3.3 Phenotypic analysis
Isolate CU01 shared all the common phenotypic properties of the Xenorhabdus genus. Cells were asporogeneous, rod-shaped and motile by means of peritrichous flagella. Isolate CU01 was negative for catalase and oxidase activities, nitrate to nitrite reduction, Vosges-Proskauer reaction, hydrogen sulfide production, indole production from tryptophane, β-galactosidase activity on o-nitrophenyl-β-d-galactopyranoside and p-nitrophenyl-β-d-galactopyranoside, lysine and ornitine decarboxylase activity, and arginine dihydrolase activity. It hydrolyzed gelatin of the Kohn's method and produced antimicrobial compounds. As other Xenorhabdus species, isolate CU01 produced acid from fructose, glycerol, N-acetylglucosamine, maltose, d-mannose and trehalose, but not from d-adonitol, l-arabinose, cellobiose, dulcitol, lactose, d-mannitol, melibiose, α-methyl-d-glucoside, raffinose, l-rhamnose, d-sorbitol, sucrose and d-xylose. Isolate CU01 was also able to grow on dl-lactate as sole source of carbon but not on l-fucose and d-mannitol.
Major discriminative characters of Xenorhabdus species are listed in Table 2 with the biochemical and physiological characters specific for X. poinarii strains and isolate CU01. The most relevant were the negative responses to DNase and lecithinase tests and the ability to tolerate temperatures as high as 40°C. The light brown pigmentation and the weak adsorption of bromothymol blue from NBTA medium were also characteristic of X. poinarii strains. Similarly to some X. poinarii strains, isolate CU01 gave a negative response to the Simmons' citrate reaction. In contrast to the X. poinarii strains tested, it hydrolyzed esculin. However, a positive response to this test has previously been noticed for members of this species [23].
Isolate CU01 | X. poinarii | X. beddingii | X. nematophilus | X. japonicus | X. bovienii | |
Number of tested strain | 1 | 3 | 1 | 3 | 1 | 6 |
Isolation from: | ||||||
S. cubanum | + | + | − | − | − | − |
S. glaseri | − | + | − | − | − | − |
Steinernema species | − | − | + | − | − | − |
S. carpocapsae | − | − | − | + | − | − |
S. kushidai | − | − | − | − | + | − |
S. feltiae | − | − | − | − | − | + |
S. kraussei | − | − | − | − | − | + |
S. affine | − | − | − | − | − | + |
S. intermedium | − | − | − | − | − | + |
Maximum growth T (°C) | 40°C | 40°C | 39°C | 35°C | 35°C | 32°C |
Pathogenicity against lepideptoran insects | − | − | + | + | − | + |
Pigmentationa | lb | br | lb | ow | yb | y |
Dye adsorption | +w | +w | + | + | + | + |
DNase | − | − | + | + | + | + |
Lecithinase (egg yolk agar) | − | dw | + | + | + | +w |
Tryptophane deaminase | − | − | − | − | − | d |
Simmons citrate | − | d | + | + | − | d |
Esculin hydrolysis | + | − | + | − | − | − |
Acid production from | ||||||
Myo-inositol | − | − | − | +w | − | dw |
Ribose | − | − | +w | +w | − | +w |
Salicin | − | − | +w | − | − | − |
Gluconate | +w | dw | + | − | − | dw |
Utilization of | ||||||
dl-Gycerate | − | [−] | + | d | − | d |
l-Histidine | + | d | + | − | − | d |
Myo-inositol | − | − | − | +w | − | dw |
Ribose | − | − | + | d | − | [+] |
l-Tyrosine | − | [−]w | + | − | − | d |
Isolate CU01 | X. poinarii | X. beddingii | X. nematophilus | X. japonicus | X. bovienii | |
Number of tested strain | 1 | 3 | 1 | 3 | 1 | 6 |
Isolation from: | ||||||
S. cubanum | + | + | − | − | − | − |
S. glaseri | − | + | − | − | − | − |
Steinernema species | − | − | + | − | − | − |
S. carpocapsae | − | − | − | + | − | − |
S. kushidai | − | − | − | − | + | − |
S. feltiae | − | − | − | − | − | + |
S. kraussei | − | − | − | − | − | + |
S. affine | − | − | − | − | − | + |
S. intermedium | − | − | − | − | − | + |
Maximum growth T (°C) | 40°C | 40°C | 39°C | 35°C | 35°C | 32°C |
Pathogenicity against lepideptoran insects | − | − | + | + | − | + |
Pigmentationa | lb | br | lb | ow | yb | y |
Dye adsorption | +w | +w | + | + | + | + |
DNase | − | − | + | + | + | + |
Lecithinase (egg yolk agar) | − | dw | + | + | + | +w |
Tryptophane deaminase | − | − | − | − | − | d |
Simmons citrate | − | d | + | + | − | d |
Esculin hydrolysis | + | − | + | − | − | − |
Acid production from | ||||||
Myo-inositol | − | − | − | +w | − | dw |
Ribose | − | − | +w | +w | − | +w |
Salicin | − | − | +w | − | − | − |
Gluconate | +w | dw | + | − | − | dw |
Utilization of | ||||||
dl-Gycerate | − | [−] | + | d | − | d |
l-Histidine | + | d | + | − | − | d |
Myo-inositol | − | − | − | +w | − | dw |
Ribose | − | − | + | d | − | [+] |
l-Tyrosine | − | [−]w | + | − | − | d |
All tests were done at 28°C unless otherwise mentioned: +=90–100% of strains are positive; [+]=76–89% are positive; d=26–75% are positive; [−]=11–25% are positive; −=0–10% are positive. The superscript w (e.g. +w) indicates a weak reaction.
lb, light brown; b, brown; ow, off-white; y, yellow; yb, yellowish brown.
Isolate CU01 | X. poinarii | X. beddingii | X. nematophilus | X. japonicus | X. bovienii | |
Number of tested strain | 1 | 3 | 1 | 3 | 1 | 6 |
Isolation from: | ||||||
S. cubanum | + | + | − | − | − | − |
S. glaseri | − | + | − | − | − | − |
Steinernema species | − | − | + | − | − | − |
S. carpocapsae | − | − | − | + | − | − |
S. kushidai | − | − | − | − | + | − |
S. feltiae | − | − | − | − | − | + |
S. kraussei | − | − | − | − | − | + |
S. affine | − | − | − | − | − | + |
S. intermedium | − | − | − | − | − | + |
Maximum growth T (°C) | 40°C | 40°C | 39°C | 35°C | 35°C | 32°C |
Pathogenicity against lepideptoran insects | − | − | + | + | − | + |
Pigmentationa | lb | br | lb | ow | yb | y |
Dye adsorption | +w | +w | + | + | + | + |
DNase | − | − | + | + | + | + |
Lecithinase (egg yolk agar) | − | dw | + | + | + | +w |
Tryptophane deaminase | − | − | − | − | − | d |
Simmons citrate | − | d | + | + | − | d |
Esculin hydrolysis | + | − | + | − | − | − |
Acid production from | ||||||
Myo-inositol | − | − | − | +w | − | dw |
Ribose | − | − | +w | +w | − | +w |
Salicin | − | − | +w | − | − | − |
Gluconate | +w | dw | + | − | − | dw |
Utilization of | ||||||
dl-Gycerate | − | [−] | + | d | − | d |
l-Histidine | + | d | + | − | − | d |
Myo-inositol | − | − | − | +w | − | dw |
Ribose | − | − | + | d | − | [+] |
l-Tyrosine | − | [−]w | + | − | − | d |
Isolate CU01 | X. poinarii | X. beddingii | X. nematophilus | X. japonicus | X. bovienii | |
Number of tested strain | 1 | 3 | 1 | 3 | 1 | 6 |
Isolation from: | ||||||
S. cubanum | + | + | − | − | − | − |
S. glaseri | − | + | − | − | − | − |
Steinernema species | − | − | + | − | − | − |
S. carpocapsae | − | − | − | + | − | − |
S. kushidai | − | − | − | − | + | − |
S. feltiae | − | − | − | − | − | + |
S. kraussei | − | − | − | − | − | + |
S. affine | − | − | − | − | − | + |
S. intermedium | − | − | − | − | − | + |
Maximum growth T (°C) | 40°C | 40°C | 39°C | 35°C | 35°C | 32°C |
Pathogenicity against lepideptoran insects | − | − | + | + | − | + |
Pigmentationa | lb | br | lb | ow | yb | y |
Dye adsorption | +w | +w | + | + | + | + |
DNase | − | − | + | + | + | + |
Lecithinase (egg yolk agar) | − | dw | + | + | + | +w |
Tryptophane deaminase | − | − | − | − | − | d |
Simmons citrate | − | d | + | + | − | d |
Esculin hydrolysis | + | − | + | − | − | − |
Acid production from | ||||||
Myo-inositol | − | − | − | +w | − | dw |
Ribose | − | − | +w | +w | − | +w |
Salicin | − | − | +w | − | − | − |
Gluconate | +w | dw | + | − | − | dw |
Utilization of | ||||||
dl-Gycerate | − | [−] | + | d | − | d |
l-Histidine | + | d | + | − | − | d |
Myo-inositol | − | − | − | +w | − | dw |
Ribose | − | − | + | d | − | [+] |
l-Tyrosine | − | [−]w | + | − | − | d |
All tests were done at 28°C unless otherwise mentioned: +=90–100% of strains are positive; [+]=76–89% are positive; d=26–75% are positive; [−]=11–25% are positive; −=0–10% are positive. The superscript w (e.g. +w) indicates a weak reaction.
lb, light brown; b, brown; ow, off-white; y, yellow; yb, yellowish brown.
By applying a factor analysis to the phenotypic data, the different Xenorhabdus species were well-separated and the three species X. nematophilus, X. bovienii and X. poinarii, which encompassed several strains, formed three separate clusters (Fig. 2). The species X. nematophilus corresponded to a tight cluster and strains of X. bovienii and X. poinarii were more distant. The isolate CU01 was located near the X. poinarii strains indicating that it was phenotypically related to this species. One of the determinative characters of factor analysis was the maximum growth temperature. Members of the X. bovienii species did not tolerate temperatures higher than 32°C. X. nematophilus and X. japonicus strains were more tolerant and could grow up to 35°C. At the opposite, X. beddingii and X. poinarii species were resistant to higher temperatures and could multiply up to 39 and 40°C, respectively.
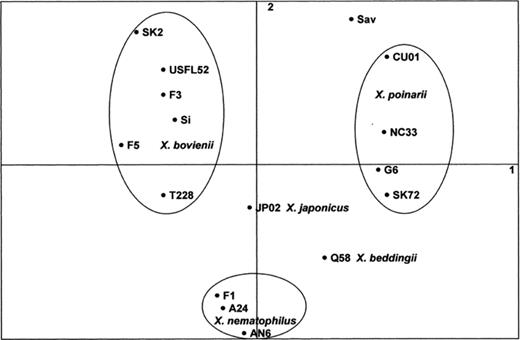
Schematic representation of the strains in the mathematical space defined by the two first components of a factor analysis (see Section 2) using the phenotypic data (only variable characters are retained). Groups corresponding to a Xenorhabdus species are encircled.
Spodoptera larvae were injected with 100–10 000 cells of isolate CU01. Injection of approximately 1000 cells was necessary to reach the LD50. In comparison with other Xenorhabdus strains, this value is higher: usually less than 100 cells are able to kill more than 50% of the injected insects (only one cell for strain F1 of X. nematophilus). The pathogenic pattern of isolate CU01 corresponded to those of X. poinarii strains whose LD50 is in a range of 1000–10 000 cells [24] and are considered weakly pathogenic for Lepidoptera.
3.4 DNA-DNA hybridizations
PCR-RFLP analysis of the 16S rDNA and phenotypic studies both indicated the close relatedness between isolate CU01 and representative strains of X. poinarii. In order to confirm these results by DNA-DNA hybridizations, the DNA of the X. poinarii type strain (G6) was labelled and respectively hybridized with the total genomic DNA of the isolate CU01 and of strain NC33.
The unstandardized re-association values for homoduplexes (100% control) ranged from 71 to 80%. The levels of re-association in negative control tubes (0%) ranged from 2 to 4%. The Tm value of homoduplexes in 0.42 M NaCl was 90.1°C. The DNA relatedness between strain G6 and isolate CU01 from S. cubanum was 68%, with a ΔTm value of 3°C. The percentage of relative DNA relatedness between strain G6 and strain NC33, another strain from X. poinarii species used as positive control, was 96% with a ΔTm value of 2.4°C. The ad hoc committee on reconciliation of approaches to bacterial systematics defined a bacterial species as a group of strains sharing approximatively 70% or greater DNA relatedness with 5°C or less ΔTm[25]. According to these rules the strain CU01 could be considered as belonging to X. poinarii species because of its low divergence with the type strain and its relatively high DNA relatedness.
3.5 Conclusion
Our three different approaches converged to the same conclusion. The Xenorhabdus strain isolated from S. cubanum belongs to X. poinarii. The high DNA relatedness with the type strain associated to a low ΔTm value was corroborated by a close phylogenetic relationship at the level of the ribosomal gene and common specific phenotypic characters.
From a technical point of view, this study confirmed the validity of the PCR-RFLP of 16S rDNA approach to rapidly identify Xenorhabdus isolates at the species level.
The results of this study indicate that the bacterial species X. poinarii is associated with two nematode species: S. glaseri and S. cubanum. Usually, the symbiosis between entomopathogenic nematodes and bacteria is so specific that almost each species of Steinernema possesses a specific symbiont species. However, X. bovienii represents another Xenorhabdus species associated to several host species. In this case, different 16S rDNA genotypes more or less specific to a Steinernema species have been identified. Phenotypic data also reflect the heterogeneity of this bacterial species (Fig. 2). Molecular studies of host ribosomal genes indicate that the different associated nematodes are not phylogenetically closely related [22]. This particular symbiosis needs more data to elucidate the relation between the diversity of the symbiont and those of their host.
More comprehensive data are available to interpret the association of X. poinarii with two nematode species. When S. cubanum was described, it was considered as a related species of S. glaseri because some morphological characters were closely related [6]. These morphological similarities make it difficult to distinguish the two species [26]. The restriction analysis of the intergenic transcribed spacer of the ribosomal genes revealed high molecular similarities between S. cubanum and S. glaseri[26] and the construction of a dendrogram evidenced their close phylogenetic relatedness [22]. A ‘recent’ common ancestor could explain high morphological and molecular similarities between these two species. The geographical isolation of the fauna from Cuba could have lead to their speciation, which may be relatively recent. However, the species concepts for the two types of organisms are different and a relatively larger degree of evolutionary change on the DNA level may be required to allow the creation of a bacterial species compared to a nematode species. Although the bacterial symbionts of the two nematodes at present belong to the same species, the phenomenon of co-speciation, usually observed between Steinernema and Xenorhabdus species, may be in course.
Acknowledgements
The technical assistance of Eliane Bonifassi, Anne Lanois and Christine Laroui is gratefully acknowledged. This work was supported by the French MENRT Grant 95-5-10697, the INRA-CNRS Grant 1998 number 24I06660 and by the Czech Grant A6007608/96 from the Grant Agency of the Czech Academy of Sciences.
References