-
PDF
- Split View
-
Views
-
Cite
Cite
Sonja Selenska-Pobell, Petra Panak, Vanya Miteva, Ivo Boudakov, Gert Bernhard, Heino Nitsche, Selective accumulation of heavy metals by three indigenous Bacillus strains, B. cereus, B. megaterium and B. sphaericus, from drain waters of a uranium waste pile, FEMS Microbiology Ecology, Volume 29, Issue 1, May 1999, Pages 59–67, https://doi.org/10.1111/j.1574-6941.1999.tb00598.x
- Share Icon Share
Abstract
The interaction was studied of three Bacillus isolates, recovered from a uranium waste pile in Saxony, Germany, with different heavy metal ions present in the original ground water of the pile. Using 16S amplified ribosomal DNA restriction and random amplified polymorphic DNA analyses, the three isolates were classified as B. cereus, B. megaterium and B. sphaericus. The ability was investigated of vegetative cells and spores of the indigenous Bacillus strains as well as those of the corresponding reference strains B. cereus ATCC 4415, B. megaterium NRRL B5385, and B. sphaericus NCTC 9602 to accumulate Al, Ba, Cd, Co, Cr, Cs, Cu, Fe, Ga, Mn, Ni, Rb, Pb, Si, Sn, Sr, Ti, U, and Zn. It was clearly shown that all strains studied accumulated selectively large amounts of U, Pb, Cd, Cu and Al. Co, Mn, Ni, Zn, and Ga was only weakly accumulated. The binding of Ba, Ga, Mn, Ni, and Zn was species and in some cases even strain specific.
1 Introduction
This genus Bacillus comprises more than 70 Gram-positive bacterial species [1]. Except the recently described species B. infernus, which is an obligate anaerobe [2], they involve aerobic or facultatively anaerobic spore-forming rod-shaped microorganisms [3,4]. Due to their impressive physiological diversity and the high resistance of their spores to unfavourable conditions, bacilli are widely distributed in a large variety of natural habitats [2,4–5–7]. It was demonstrated that in addition to the most abundant bacteria, the haemolithoautotrophic thiobacilli and leptospirilli, and the heterotrophic acidophili [8], some Bacillus sp. are also present in uranium waste piles [9]. However, nothing was known about the phylogenetic affiliation and the activities of bacilli in such environments. Recently, we have demonstrated the presence of diverse aerobic spore-forming Bacillus groups in a uranium waste pile near the town Johanngeorgenstadt in Saxony, Germany (unpublished observations). In the work presented here, we have classified three of the waste pile isolates as B. cereus, B. megaterium and B. sphaericus using 16S amplified ribosomal DNA restriction analysis (ARDRA) and random amplified polymorphic DNA (RAPD) analysis. The interactions were studied of the vegetative cells and spores of these strains with the heavy metals naturally present in the drain waters of the pile.
2 Materials and methods
2.1 Bacterial strains and growing conditions
The bacterial strains studied are listed in Table 1. They were grown with intensive aeration in liquid nutrient medium (8 g l−1 nutrient broth, Difco) at 30°C.
Strain | Source | Strain | Source |
Bacillus cereus 4415 | ATCCa | Bacillus circulans 2783 | ATCC |
Bacillus sp. JG-A30 | FZRb | Bacillus subtilis (Marburg) 6051T | ATCC |
Bacillus megaterium B5385 | NRRLc | Bacillus stearothermophilus 12980T | ATCC |
Bacillus sp. JG-A22 | FZR | Brevibacillus brevis 8246T | ATCC |
Bacillus sphaericus 9602 | NCTCd | Paenibacillus amylolyticus 9995T | ATCC |
Bacillus sp. JG-A12 | FZR | Paenibacillus macerans 8244T | ATCC |
Bacillus thuringiensis (Berl.) | IEBCe | Paenibacillus polymixa 3020 | IFOf |
Strain | Source | Strain | Source |
Bacillus cereus 4415 | ATCCa | Bacillus circulans 2783 | ATCC |
Bacillus sp. JG-A30 | FZRb | Bacillus subtilis (Marburg) 6051T | ATCC |
Bacillus megaterium B5385 | NRRLc | Bacillus stearothermophilus 12980T | ATCC |
Bacillus sp. JG-A22 | FZR | Brevibacillus brevis 8246T | ATCC |
Bacillus sphaericus 9602 | NCTCd | Paenibacillus amylolyticus 9995T | ATCC |
Bacillus sp. JG-A12 | FZR | Paenibacillus macerans 8244T | ATCC |
Bacillus thuringiensis (Berl.) | IEBCe | Paenibacillus polymixa 3020 | IFOf |
American Type Culture Collection.
Forschungszentrum Rossendorf.
Northern Utilisation Research and Development Division, US Department of Agriculture, Peoria, IL.
National Collection of Type Cultures, London, UK.
International Enthopathogenic Bacillus Centre, Institute Pasteur, France.
Institute of Fermentation, Osaka, Japan.
Strain | Source | Strain | Source |
Bacillus cereus 4415 | ATCCa | Bacillus circulans 2783 | ATCC |
Bacillus sp. JG-A30 | FZRb | Bacillus subtilis (Marburg) 6051T | ATCC |
Bacillus megaterium B5385 | NRRLc | Bacillus stearothermophilus 12980T | ATCC |
Bacillus sp. JG-A22 | FZR | Brevibacillus brevis 8246T | ATCC |
Bacillus sphaericus 9602 | NCTCd | Paenibacillus amylolyticus 9995T | ATCC |
Bacillus sp. JG-A12 | FZR | Paenibacillus macerans 8244T | ATCC |
Bacillus thuringiensis (Berl.) | IEBCe | Paenibacillus polymixa 3020 | IFOf |
Strain | Source | Strain | Source |
Bacillus cereus 4415 | ATCCa | Bacillus circulans 2783 | ATCC |
Bacillus sp. JG-A30 | FZRb | Bacillus subtilis (Marburg) 6051T | ATCC |
Bacillus megaterium B5385 | NRRLc | Bacillus stearothermophilus 12980T | ATCC |
Bacillus sp. JG-A22 | FZR | Brevibacillus brevis 8246T | ATCC |
Bacillus sphaericus 9602 | NCTCd | Paenibacillus amylolyticus 9995T | ATCC |
Bacillus sp. JG-A12 | FZR | Paenibacillus macerans 8244T | ATCC |
Bacillus thuringiensis (Berl.) | IEBCe | Paenibacillus polymixa 3020 | IFOf |
American Type Culture Collection.
Forschungszentrum Rossendorf.
Northern Utilisation Research and Development Division, US Department of Agriculture, Peoria, IL.
National Collection of Type Cultures, London, UK.
International Enthopathogenic Bacillus Centre, Institute Pasteur, France.
Institute of Fermentation, Osaka, Japan.
2.2 16S ARDRA and RAPD analyses
Genomic DNA was isolated using a NucleoSpin kit (Macherey-Nagel, 52313 Düren, Germany) from 10-ml bacterial overnight cultures. 16S rDNA fragments were amplified using approximately 10 ng of the DNA templates and primers: 8F, 5′-AGAGTTTGATCTACTGGC TCAG-3′; and 1492R, 5′-TACGGCTTACCTTGTTACGACTT-3′. The positions of the primers correspond to positions 8–27 and 1513–1492 of the E. coli numbering system [10]. The amplification reactions were performed as described by Selenska-Pobell et al. [11]. The PCR products of the reference strains were digested with ten frequently cutting endonucleases –AluI, BstUI, CfoI, DdeI, HaeIII, HinfI, MspI, NdeII, RsaI, and TaqI (Gibco-BRL, LifeTechnologies, Eggenstein, Germany).
RAPD was performed as described by Selenska-Pobell et al. [11,12] using the primers: AP18, 5′-CACACGCACACGGAAGAA-3′; and AP19, 5′-CAGGCACACGCA GACAGA-3′[13].
The patterns resulting from the RFLP analyses of the PCR amplified 16S rDNA as well as from the RAPD analysis were converted to a two-dimensional binary matrix (1, presence of a band; 0, absence of a band), and average linkage UPGMA dendrograms were derived as described in [12].
2.3 Analysis of the heavy metal binding capability of the Bacillus strains studied
The biomass was separated from the growing medium by centrifugation at 9000×g for 10 min at 4°C and washed four times with 10 ml physiological NaCl solution to remove the ingredients of the medium which may disturb the sorption experiments.
Amounts of 5.2±0.26 mg of pellets of vegetative cells or spores of the corresponding Bacillus strains were resuspended in 10 ml natural drain water samples from the uranium waste pile. The pH of the water samples was 4.6, they were air-saturated and contained different heavy metals in the following concentrations (μg l−1): Al, 1018; Si, 9460; Ti, 4.7; Cr, 1.9; Fe<20; Mn, 1050; Co, 43.2; Ni, 247; Cu, 124; Zn, 3975; Ga, 7.0; As<0.5; Rb, 18.6; Sr, 65; Cd, 15.4; Sn, 1.6; Cs, 1.7; Ba, 17.7; Pb, 18.6; and U, 72.1. The bacterial strains were shaken in the water samples for 48 h at 28°C. Then the bacterial cells and spores were separated from the water samples by centrifugation at 10 200×g for 10 min at 4°C. The amount of the heavy metals in the water solutions was determined by inductive coupled plasma mass spectroscopy (ICP-MS).
In order to characterise how stable the heavy metals were bound by the strains studied, the pellets of the bacterial samples were washed with 5 ml saline, and extracted for 3 h with 10 ml 0.01 M EDTA/0.01 M Tris solution (pH 7.2) after their incubation with the contaminated water. The amount of the different heavy metals in the washing solution was also measured by ICP-MS.
3 Results and discussion
Studying diversity and activity of bacteria in uranium mining waste piles may be useful for the development of bacteria-based technologies for bioremediation of environments that are highly contaminated with heavy metals and radionuclides. Recently, we have demonstrated the presence of a large number of aerobic spore-forming bacterial strains related to several Bacillus species in a uranium waste pile in Saxony, Germany (unpublished). Three of these waste pile isolates JG-A12, JG-A22 and JG-A30 (see Table 1) possessing colony, cell, and spore morphology typical for Bacillus sphaericus, Bacillus megaterium and B. cereus, respectively, were chosen for further analysis in order to precisely classify them and to estimate their ability to interact with different heavy metals occurring in original drain waters of the waste.
3.1 16S ARDRA classification of the uranium waste isolates
Applying 16S ARDRA with 10 different four-base-recognising endonucleases (see Section 2) it was easily possible to classify the isolates and to confirm their affiliation to the three above-mentioned species (see Fig. 1 and Fig. 2).
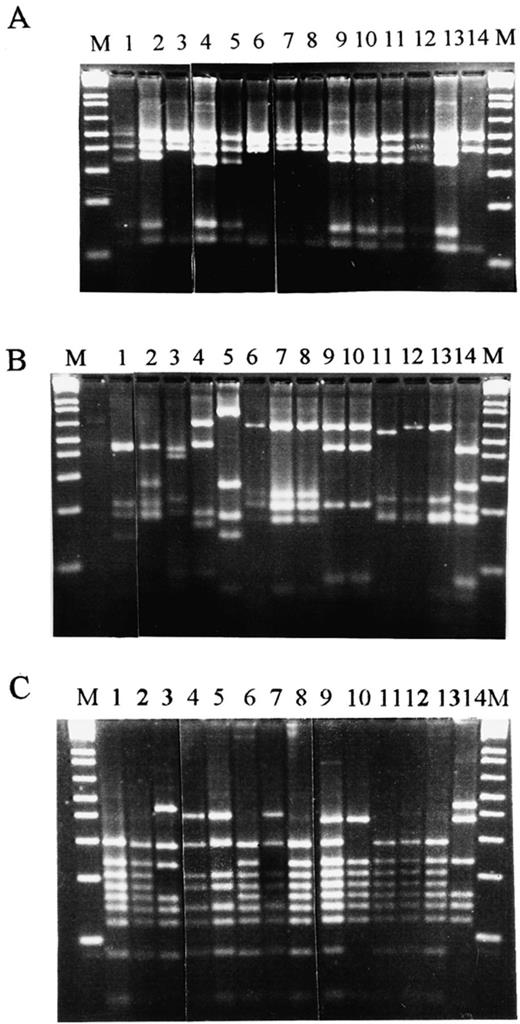
16S-ARDRA analysis. (A) RsaI patterns of the strains: 1, Bacillus subtilis (Marburg); 2, Bacillus thuringiensis (Berl.); 3, Bacillus cereus 4415; 4, Bacillus sp. JG-A30; 5, Bacillus megaterium B5385; 6, Bacillus sp. JG-A22; 7, Bacillus sphaericus 9602; 8, Bacillus sp. JG-A12; 9, Bacillus circulans 2783; 10, Paenibacillus amylolyticus 9995T; 11, Paenibacillus polymixa 3020; 12, Brevibacillus brevis 8246T; 13, Paenibacillus macerans 8244T; 14, Bacillus stearothermophilus 12980T; (B) AluI patterns of the strains: 1, Bacillus subtilis (Marburg); 2, Bacillus thuringiensis (Berl.); 3, Bacillus cereus 4415; 4, Bacillus sp. JG-A30; 5, Bacillus megaterium B5385; 6, Bacillus sp. JG-A22; 7, Bacillus sphaericus 9602; 8, Bacillus sp. JG-A12; 9, Bacillus circulans 2783; 10, Brevibacillus brevis 8246T; 11, Paenibacillus amylolyticus 9995T; 12, Paenibacillus polymixa 3020; 13; Paenibacillus macerans 8244T; 14, Bacillus stearothermophilus 12980T. (C) BstUI patterns of the strains: 1, Bacillus subtilis (Marburg); 2, Bacillus thuringiensis (Berl.); 3, Bacillus cereus 4415; 4, Bacillus sp. JG-A30; 5, Bacillus megaterium B5385; 6, Bacillus sp. JG-A22; 7, Bacillus sphaericus 9602; 8, Bacillus circulans 2783; 9, Bacillus sp. JG-A12; 10, Paenibacillus amylolyticus 9995T; 11, Paenibacillus polymyxa 3020; 12; Paenibacillus macerans 8244T; 13, Brevibacillus brevis 8246T; 14, Bacillus stearothermophilus 12980T.
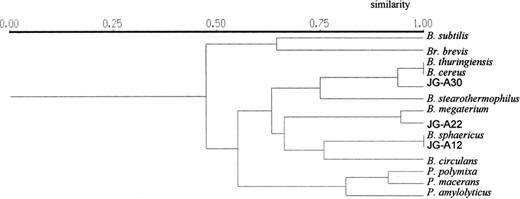
UPGMA dendrogram showing the relationship between the strains studied as determined by 16S-ARDRA with AluI, BstUI, CfoI, DdeI, HaeIII, HinfI, MspI, NdeII, RsaI, and TaqI.
The patterns of strain JG-A12, obtained with all ten enzymes, were identical to those of the reference strain Bacillus sphaericus NCTC 9602. Interestingly, the isolate JG-A12 as well as the reference strain B. sphaericus NCTC 9602 possess an S-layer protein with identical structure [14].
The isolate JG-A30 is representing the largest group of the uranium waste pile isolates, which was related to B. cereus/B. thuringiensis. With all ten enzymes used, it was not possible to discriminate the reference strains of the very closely related species B. cereus and B. thuringiensis. This result reflects the almost identical structure of the 16S rRNA genes of these two species as analysed in [3]. The 16S ARDRA patterns of strain JG-A30 were identical to those of the reference strains B. cereus/B. thuringiensis with only one exception which was obtained using AluI (see Fig. 1B). Strain JG-A30, in contrast to some other isolates of this group, did not possess parasporal crystals (typical for B. thuringensis), and for this reason we considered it to be related to B. cereus. Heyndrickx et al. [15] also found slight differences between the ARDRA patterns of two other B. cereus strains.
It is not excluded, however, that the uranium waste pile isolate JG-A30 represents some other species very closely related to B. cereus in the so-called ‘Bacillus cereus group’ [3,16] or even some novel species which might belong to this group.
The isolate JG-A22 is a member of a minor group of waste isolates which were related to B. megaterium. This strain has very large vegetative cells, colony, and spore morphology, characteristic of the species B. megaterium. Some of the 16S ARDRA patterns of strain JG-A22, however, have been slightly different than those of B. megaterium B5385 (see the example in Fig. 1C). The exact classification of this isolate as well as those of the isolate JG-A30 will be possible after performing sequence analyses of their 16S rDNAs (work in progress in our laboratory). The results of 16S ARDRA are summarised in Fig. 2.
3.2 RAPD analysis of the uranium waste isolates
In Fig. 3A, the RAPD patterns of the strains studied are presented. As seen in the figure, all strains analysed possess strain-specific profiles. This is in agreement with the RAPD-results published for some other groups of bacteria [11–12,13] and in particular with the results published recently for the strains belonging to the species B. cereus and B. licheniformis[16].
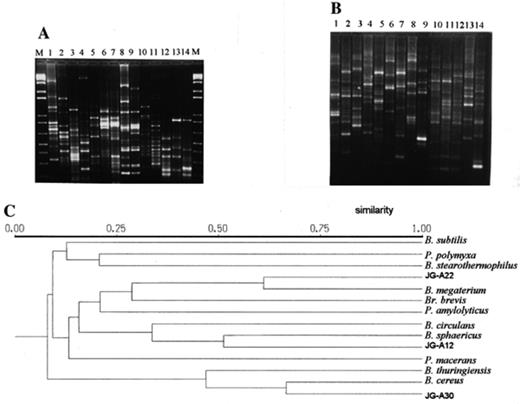
RAPD-PCR analysis. (A) RARD-patterns with the primer AP18 of the strains: 1, Brevibacillus brevis 8246T; 2, Paenibacillus amylolyticus 9995T; 3, Paenibacillus polymyxa 3020; 4, Paenibacillus macerans 8244T; 5, Bacillus circulans 2783; 6, Bacillus sphaericus 9602; 7, Bacillus sp. JG-A12; 8, Bacillus megaterium B5385; 9, Bacillus sp. JG-A22; 10, Bacillus subtilis (Marburg); 11, Bacillus stearothermophilus 12980T; 12, Bacillus sp. JG-A30; 13, Bacillus cereus 4415; 14, Bacillus thuringiensis (Berl.); M, 1-kb DNA ladder (Gibco-BRL). (B) RAPD-patterns with the primer AP19 of the strains: 1, Bacillus subtilis (Marburg); 2, Bacillus sp. JG-A22; 3, Bacillus megaterium B5385; 4, Paenibacillus macerans 8244T; 5, Paenibacillus polymyxa 3020; 6, Paenibacillus amylolyticus 9995T; 7, Bacillus stearothermophilus 12980T; 8, Brevibacillus brevis 8246T; 9, Bacillus circulans 2783; 10, Bacillus thuringiensis (Berl.); 11, Bacillus cereus 4415; 12, Bacillus sp. JG-A30; 13, Bacillus sphaericus 9602; 14, Bacillus sp. JG-A12; M, 1-kb DNA ladder (Gibco-BRL). (C) UPGMA dendrogram derived from the RAPD patterns presented in A and B.
As can be seen in the figure, strain JG-A30 has a pattern which is similar to that of strain B. cereus 4415, but not to the one of the B. thuringiensis reference strain. The similarity between strains JG-A30 and B. cereus 4415, however, is not very high. This is consistent with the results obtained by Daffonchio et al. [16] with other random primers. The authors of that work demonstrated that the RAPD analysis shows a remarkable diversity among the strains of B. cereus. They also demonstrated that one contribution to the diversity detected by RAPD analysis was determined by the presence of large extrachromosomal elements (a typical feature for this species) that were amplified during their RAPD analyses [16]. The RAPD pattern of strain JG-A22 had much higher similarity to those of the reference strain B. megaterium B5385 (see also Fig. 3B). The RAPD patterns of strain JG-A12 were completely different from those of the B. sphaericus NCTC 9602. This, however, is also not very surprising, bearing in mind that for some bacterial species, including bacilli too, it was found that even identical 16S rRNA structure may not be sufficient to guarantee species identity [17–20].
3.3 Selective accumulation of heavy metals by vegetative cells and spores of the isolates and reference strains
The next task of our work was to investigate the capability of the Bacillus waste isolates and the corresponding reference strains to accumulate heavy metals from the contaminated original waters of the pile. The interactions of the three phylogenetically related pairs, B. cereus/JG-A30, B. sphaericus/JG-A12, and B. megaterium/JG-A22 with 19 heavy metals (Al, Ba, Cd, Co, Cr, Cs, Cu, Fe, Ga, Mn, Ni, Rb, Pb, Si, Sn, Sr, Ti, U, and Zn) were investigated. The results of these analyses are presented in Fig. 4. As can be seen in the figure, U, Cu, Pb, Al and Cd were preferably accumulated by the vegetative cells of all strains studied. The spores of the strains followed the same tendency; however, those of the phylogenetically related pair B. cereus/JG-A30 possess much lower capability to bind Cd in comparison to the spores of the other two pairs as well as to their own vegetative cells. In addition, the spores of B. sphaericus/JG-A12 had higher ability to bind Ba than the vegetative cells of this pair.
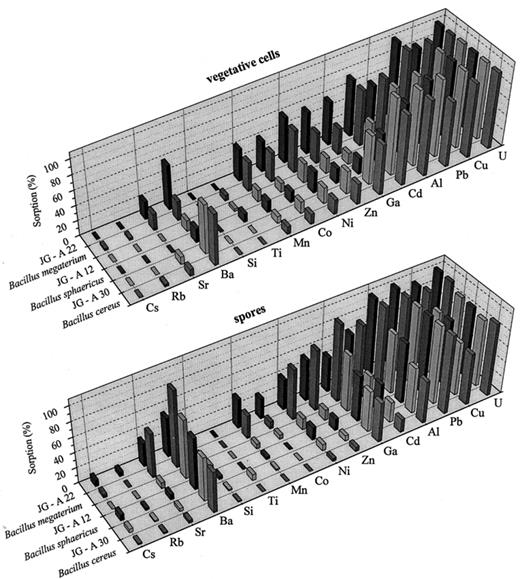
Selective accumulation of heavy metals by vegetative cells (upper panel) and spores (lower panel) of the strains studied.
The accumulation of some of the metal ions by the vegetative cells of the stains studied was species specific. For example the cells of the pair B. cereus/JG-A30 did accumulate higher amounts of Ba, Cd and Ga in comparison to the other two pairs, whereas the pair B. megaterium/JG-A22 did accumulate Co, Mn, Ni, and Zn to a higher extent. All these elements were accumulated very weakly by the pair B. sphaericus/JG-A12 (see Fig. 4, upper panel).
The selective accumulation of several heavy metals (Mn, Co, Ni, Cu, Zn, Cd, Hg, Pb, and U) by bacteria was demonstrated earlier by Nakajima and Sakaguchi [21]. Two of the 32 different bacterial strains studied by these authors (B. cereus IAM 1656 and B. sphaericus IAM 1026) belonged to the genus Bacillus. Our results obtained with the pairs B. cereus/JG-A30 and B. sphaericus/JG-A12 are in general agreement to those published in [21] with one important exception: in contrast to our results, no accumulation of Cd by B. cereus IAM 1656 was observed in [21].
Interestingly, the corresponding members of the pairs B. sphaericus/JG-A12 and B. cereus/JG-A30 share almost the same binding capability to each particular metal ion involved in the experiment. This was not the case with the pair B. megaterium/JG-A22. As seen in Fig. 4 (upper panel), the vegetative cells of the uranium waste isolate JG-A22 did accumulate Ba, Ga, and Zn to a higher and Al and Cu to a lesser extent compared to the B. megaterium reference strain. This result may be connected to the higher phylogenetic distance between these two strains evaluated as shown above by 16S ARDRA (see Fig. 2). The spores of the two B. megaterium strains also interacted differently to the metal ions studied. The spores of the uranium waste pile isolate did accumulate lesser amounts of Sr, Ba, Ni, Zn, Ga, and Cd, but higher amounts of Cs, Rb, Mn, and Co than the reference strain. In general, the spores of the B. megaterium strains possess a higher ability to bind heavy metals in comparison to the spores of the other four strains investigated (see Fig. 4, lower panel).
In order to determine how strong the heavy metals were bound by both, vegetative cells and spores of the strains studied, desorption experiments were performed.
As shown in Fig. 5 (upper panels), for the vegetative cells, most of the heavy metals accumulated were easily extracted with the EDTA/Tris solution (see Section 2).
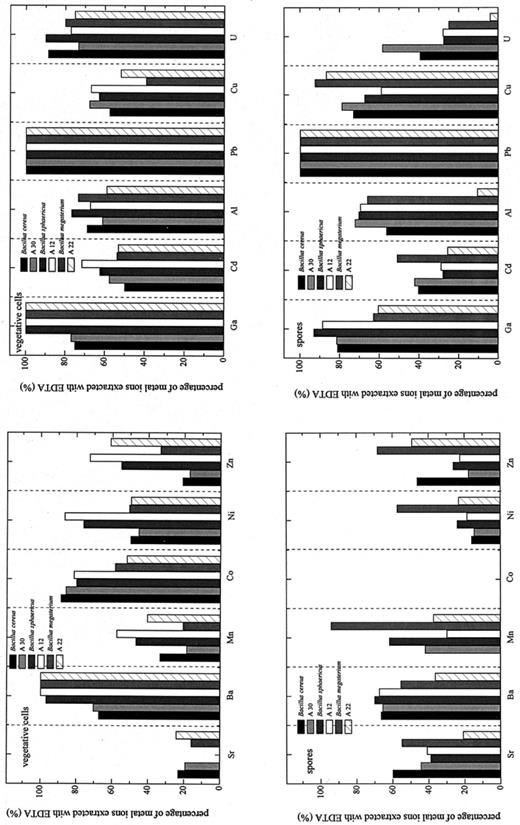
Some of the metals, however, were irreversibly bound by the spores. Fig. 5 (lower panels) shows that significant amounts of Ba, Ni, Cd, and U could not be extracted with EDTA from the spores. The binding of U, Al, and Cd by the spores of the uranium waste isolate JG-A22 was especially strong.
The nature of the complexes formed with the U(VI) on the surfaces of the vegetative cells and spores of the Bacillus strains studied is currently being analysed by extended X-ray absorption near edge (EXAFS) and X-ray absorption near edge spectroscopy (XANES) measurements.
In summary, our study demonstrates that Bacillus strains related to the species B. spaericus, B. cereus, and B. megaterium are present in uranium wastes. These strains are selectively accumulating different heavy metals from contaminated waters. Moreover, some of these strains, and especially their spores, possess the capability to irreversibly bind large amounts of metals such as Al, Cd, and U.
Studying bacterial communities in uranium waste piles gives promising prospectives to identify strains which are well adapted to these polluted environments and which are able to interact significantly with the dissolved toxic metals. Such strains may be useful for the development of bacteria-based techniques for the removal of toxic metals from polluted effluents and waste waters of uranium mines and mill-tailings.
Acknowledgements
This work was supported by Grant 7531.50-03-FZR/607 from the Sächsisches Staatsministerium für Wissenschaft und Kunst, Germany. The participation of I. Boudakov was financed by the Federal Ministry of Science and Technology, Germany.
References