-
PDF
- Split View
-
Views
-
Cite
Cite
Isbelia Reyes, Louis Bernier, Régis R. Simard, Phillipe Tanguay, Hani Antoun, Characteristics of phosphate solubilization by an isolate of a tropical Penicillium rugulosum and two UV-induced mutants, FEMS Microbiology Ecology, Volume 28, Issue 3, March 1999, Pages 291–295, https://doi.org/10.1111/j.1574-6941.1999.tb00584.x
- Share Icon Share
Abstract
After screening several fungal isolates obtained from Venezuelan soils, the isolate IR-94MF1 of Penicillium rugulosum was selected for its high mineral phosphate solubilizing activity (Mps+) with hydroxyapatite. Mutants with altered (Mps−) or amplified activity (Mps++) were obtained by UV irradiation of conidia. When glucose was used as the sole C source by the fungus, gluconic acid was associated with the hydroxyapatite activity. In solid media, in comparison to glucose and maltose, sucrose appeared to be the best C source for the solubilization of hydroxy-apatite and FePO4.
Soil microorganisms that solubilize mineral phosphates can significantly affect P cycling in both natural and agricultural ecosystems. Phosphate absorption by plants can be increased by the presence of symbiotic organisms such as mycorrhizal fungi [1] or by inoculation with the soil mineral phosphate solubilizing fungus, P. bilaii [2–4]. Other Penicillium species such as P. simplicissimum and P. aurantiogriseum[5], and P. variabile P16 [6] are also able to solubilize poorly soluble inorganic phosphates in vitro. The mineral phosphate solubilizing phenotype was designated Mps+ by Goldstein [7], and found to be the result of gluconic acid-mediated dissolution of hydroxyapatite (HA) in Gram-negative bacteria [7,8]. In this work the isolate IR-94MF1 of Penicillium rugulosum was selected among fungi isolated from Venezuelan acidic soils, because of its high calcium phosphate solubilizing activity. We report here for the first time in fungi the development of two unique mutants for the Mps+ phenotype. One mutant of P. rugulosum isolate IR-94MF1 exhibited a very high solubilization activity (Mps++) and the other a negative phenotype for HA solubilization (Mps−). Isolate IR-94MF1 was identified by the National Identification Service of Agriculture and Agri-food Canada (Ottawa, Ontario) as P. rugulosum Thom [9]. The isolation medium used was a modified minimal salts medium (MM) [10] containing per liter of distilled water: NH4Cl 0.4 g; KNO3 0.78 g; NaCl 0.1 g; MgSO4·7H2O 0.5 g; CaCl2·2H2O 0.1 g; FeSO4·7H2O 0.5 mg; MnSO4·H2O 1.56 mg; ZnSO4·7H2O 1.40 mg; glucose, 10 g and agar, 20 g. Phosphorus was added to the medium in the form of Ca(PO4)2 at 4 g l−1 and the pH was adjusted to 6.5. Pure cultures were maintained at room temperature (25°C) in Petri plates containing a complete agar medium (CM) made with 1 liter modified MM supplemented with: KH2PO4 0.45 g; vitamin B12 (Sigma) 2 μg; malt extract 5.0 g and yeast extract 1.0 g. Chloramphenicol (30 μg ml−1) and streptomycin sulfate (100 μg ml−1) were added to all media to inhibit any potential bacterial growth.
The solubilization rate of HA (Ca5HO13P3, Sigma) by IR-94MF1 was measured by the determination of the amount of soluble P present in the culture filtrate and by its pH variation. Flasks containing 150 ml MM medium with washed HA at 30 mM P were autoclaved at 121°C for 20 min and inoculated with 1 ml containing 5×105 mycelium pieces prepared by grinding mycelia in sterile water. Uninoculated flasks were used as controls. Triplicate flasks were incubated in the dark at 28°C (optimal growth temperature) on a rotary shaker (150 rpm). Collections of subsamples (1, 3, 5, 7, 12 and 20 days), pH and P determinations were done as described by Reyes et al. [11]. The anionic and cationic exchange resins, Dowex 1-X8 in Cl− form (Bio-Rad) and Dowex-50W in H+ form (Sigma) respectively, were used to isolate solubilizing substances from filtrates of cultures grown on KH2PO4-MM (3 mM P). A rapid qualitative test was developed in order to check for the presence of solubilizing substances before and after the passage of the filtrate through the resins. Petri plates with a 2 mm thick agar layer containing HA-MM (7.5 mM P) were prepared and 25 μl filtrate was applied on the plates. After a few minutes a solubilization halo was observed when a solubilizing substance was present. The presence of organic acids was determined in culture filtrates by a modification of the method of Baziramakenga et al. [12] on a Dionex 4000i ion chromatograph (Dionex Corp.). The presence of gluconic acid in the culture filtrates of HA-MM was confirmed with enzymatic methods [11,13].
Mutagenesis was performed according to the protocol of Bernier and Hubbes [14] modified as follows. Conidia were harvested from CM plates flooded with 5 ml of a 0.05% (v/v) Tween 20 sterile solution. Triplicate Petri plates containing 10 ml of conidial suspension (1×107 ml−1) were irradiated with a Philips 30 W germicidal UV lamp (254 nm). Plates were exposed to UV for 0, 10, 30, 60, 90, 120, 180, or 240 s. An irradiated suspension subsample was used for survival counts, whereas 5 ml were serially diluted and spread on CM plates supplemented with 0.01% (w/v) sodium deoxycholate to induce colony growth. Plates were manipulated and incubated in the dark at room temperature. Survival counts were obtained after 5 days incubation. To isolate Mps+ mutants, colonies obtained after 90 s UV exposure were used (74% conidial survival). A piece from the margin of each colony was transferred to a HA-MM plate supplemented with sodium deoxycholate. Colonies with a strong variation of the HA solubilization halo but morphologically similar to the wild-type were selected. Monosporic cultures of the isolates were made and cultured at least five times to assure their mitotic stability.
The MPS activity of the wild-type and its mutants was measured using MM plates containing glucose (10 g l−1), maltose (20 g l−1) or sucrose (30 g l−1) as the C source and HA or FePO4 (30 mM P) as the phosphate source. The plates were inoculated by placing in their centers a 3 mm diameter disk of a 3-day-old synchronous vegetative mycelium produced on MM agar plates (without any phosphate addition to avoid early sporulation), by the addition of 1 ml of a suspension containing 1–5×107 conidia. The plates were incubated in the dark at 28°C. Statistical analysis and data transformations were performed as described by Reyes et al. [11].
Filtrates obtained from the wild-type isolate IR-94MF1 cultured on KH2PO4 medium showed HA solubilization, indicating that the solubilizing agent is not induced by the presence of poorly soluble inorganic phosphates. The activity was retained by the anionic exchange resin which suggests that the solubilizing substance is an organic acid. Gluconic acid was then identified and determined by ion chromatography of filtrates from HA cultures (Fig. 1). During the HA solubilization, soluble P in solution reached its peak values (13–15 mM) between days 5 and 7. This was associated with the production of the highest gluconic acid concentrations (41–46 mM) and with a significant pH decrease (3.4–3.6). At 20 days, solubilized phosphate and gluconic acid production decreased to very low values while the pH increased to near neutrality (Fig. 1).
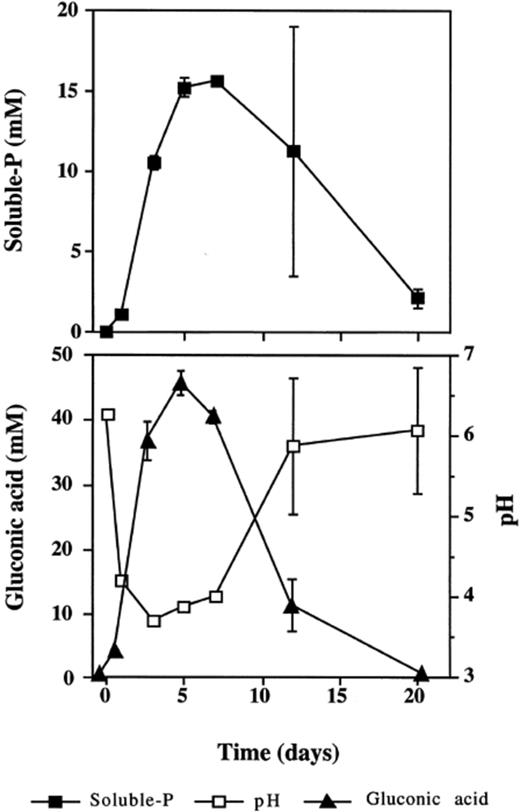
Rate of P solubilization, pH and gluconic acid production by the wild-type isolate P. rugulosum IR-94MF1 cultivated in a liquid medium with glucose as the sole C source and in the presence of 30 mM P provided as HA. Values are means of three replicates. Error bars (±S.D.) are shown when larger than the symbol.
One mutant (Mps− m1) with a significant decrease in HA solubilization halo was obtained after the first irradiation. After a second UV irradiation the survival curves of the conidia of both the wild-type and Mps− m1 were very similar. Thus, the same selection procedure was repeated and two mutants were obtained: Mps− was able to grow on HA-MM but did not show a measurable solubilization halo and Mps++ exhibited a solubilization halo larger than that of the wild-type.
The radial growth of the wild-type and Mps++ mutant was similar on the three C sources tested. However, the HA and FePO4 solubilization halos produced by the Mps++ mutant were significantly (P<0.001) larger than those of the wild-type with all C sources (Table 1). Also, the halos were always larger for HA than for FePO4. Solubilization halos produced in the presence of glucose or maltose were comparable in size and significantly (P<0.001) smaller than the halos observed in the presence of sucrose, indicating that this last C source was the best for P solubilization with both the wild-type and the Mps++ mutant.
Effect of C source on growth and phosphate solubilization by the wild-type Penicillium rugulosum IR-94MF1 and its mutants after 7 days incubation at 28°C
Isolate | C source | |||||
Glucose | Maltose | Sucrose | ||||
Colony | Halo | Colony | Halo | Colony | Halo | |
Hydroxyapatite | ||||||
Wild-type | 11.0 aA | 24.7 bB | 11.0 aA | 25.0 bB | 11.0 bA | 27.3 bA |
Mps− | 11.0 aB | 0.0 cB | 11.0 aB | 0.0 cB | 11.7 aA | 6.3 cA |
Mps++ | 11.0 aA | 36.3 aB | 11.0 aA | 36.7 aB | 11.0 bA | 43.2 aA |
FePO4 | ||||||
Wild-type | 11.3 aB | 5.3 bB | 12.2 aA | 2.8 bC | 11.3 aB | 9.7 bA |
Mps− | 10.0 bA | 0.0 cA | 11.0 bA | 0.0 cA | 10.3 bB | 0.0 cA |
Mps++ | 11.0 aA | 17.7 aB | 11.0 bA | 17.3 aB | 11.0 aA | 23.0 aA |
Isolate | C source | |||||
Glucose | Maltose | Sucrose | ||||
Colony | Halo | Colony | Halo | Colony | Halo | |
Hydroxyapatite | ||||||
Wild-type | 11.0 aA | 24.7 bB | 11.0 aA | 25.0 bB | 11.0 bA | 27.3 bA |
Mps− | 11.0 aB | 0.0 cB | 11.0 aB | 0.0 cB | 11.7 aA | 6.3 cA |
Mps++ | 11.0 aA | 36.3 aB | 11.0 aA | 36.7 aB | 11.0 bA | 43.2 aA |
FePO4 | ||||||
Wild-type | 11.3 aB | 5.3 bB | 12.2 aA | 2.8 bC | 11.3 aB | 9.7 bA |
Mps− | 10.0 bA | 0.0 cA | 11.0 bA | 0.0 cA | 10.3 bB | 0.0 cA |
Mps++ | 11.0 aA | 17.7 aB | 11.0 bA | 17.3 aB | 11.0 aA | 23.0 aA |
Values are mean diameters (mm) of three replicates. Different letters show values that are significantly different (P<0.001) according to the LSD test used with the rank procedure: between isolates (a–c) and between C sources (A–C).
Effect of C source on growth and phosphate solubilization by the wild-type Penicillium rugulosum IR-94MF1 and its mutants after 7 days incubation at 28°C
Isolate | C source | |||||
Glucose | Maltose | Sucrose | ||||
Colony | Halo | Colony | Halo | Colony | Halo | |
Hydroxyapatite | ||||||
Wild-type | 11.0 aA | 24.7 bB | 11.0 aA | 25.0 bB | 11.0 bA | 27.3 bA |
Mps− | 11.0 aB | 0.0 cB | 11.0 aB | 0.0 cB | 11.7 aA | 6.3 cA |
Mps++ | 11.0 aA | 36.3 aB | 11.0 aA | 36.7 aB | 11.0 bA | 43.2 aA |
FePO4 | ||||||
Wild-type | 11.3 aB | 5.3 bB | 12.2 aA | 2.8 bC | 11.3 aB | 9.7 bA |
Mps− | 10.0 bA | 0.0 cA | 11.0 bA | 0.0 cA | 10.3 bB | 0.0 cA |
Mps++ | 11.0 aA | 17.7 aB | 11.0 bA | 17.3 aB | 11.0 aA | 23.0 aA |
Isolate | C source | |||||
Glucose | Maltose | Sucrose | ||||
Colony | Halo | Colony | Halo | Colony | Halo | |
Hydroxyapatite | ||||||
Wild-type | 11.0 aA | 24.7 bB | 11.0 aA | 25.0 bB | 11.0 bA | 27.3 bA |
Mps− | 11.0 aB | 0.0 cB | 11.0 aB | 0.0 cB | 11.7 aA | 6.3 cA |
Mps++ | 11.0 aA | 36.3 aB | 11.0 aA | 36.7 aB | 11.0 bA | 43.2 aA |
FePO4 | ||||||
Wild-type | 11.3 aB | 5.3 bB | 12.2 aA | 2.8 bC | 11.3 aB | 9.7 bA |
Mps− | 10.0 bA | 0.0 cA | 11.0 bA | 0.0 cA | 10.3 bB | 0.0 cA |
Mps++ | 11.0 aA | 17.7 aB | 11.0 bA | 17.3 aB | 11.0 aA | 23.0 aA |
Values are mean diameters (mm) of three replicates. Different letters show values that are significantly different (P<0.001) according to the LSD test used with the rank procedure: between isolates (a–c) and between C sources (A–C).
Production of gluconic acid in fungi, such as P. variabile[8] and our isolate P. rugulosum IR-94MF1, is a trait that is related to the Mps+ phenotype as already found in Gram-negative bacteria [7,8]. This organic acid has also been found in several saprophytic fungi such as P. chrysogenum[15], P. notatum[16], Aspergillus niger[17] and the ectomycorrhizal species Tricholoma robustum[18]. Gluconic acid is produced extracellularly or in the perisplasmic space of the cells of some filamentous fungi by a direct oxidation of aldonic sugars. Subsequently, gluconate is oxidized by the enzyme gluconate kinase producing 6-phosphogluconate. The oxidation of this product continues through the pentose phosphate pathway and⧸or through a non-phosphorylative pathway analogous to the Entner-Doudoroff pathway in bacteria [7] where gluconate is converted to 2-keto-3-deoxygluconate and further converted to pyruvate and glyceraldehyde [17,19]. When glucose was used as the sole C source, the solubilization of HA and the production of gluconic acid by the wild-type isolate P. rugulosum IR-94MF1 were accompanied by a drop in the pH of the liquid medium. Iwase [18] showed that the acidification of media was caused by the accumulation of gluconic acid. As the concentration of gluconic acid in the culture medium starts to decrease after 5 days (Fig. 1), our results suggest that the wild-type isolate IR-94MF1 is probably able to catabolize this organic acid. In fact, it was previously reported that P. chrysogenum[15] can catabolize gluconic acid through the pentose phosphate pathway.
In order to understand the potential variability of the Mps+ phenotype of P. rugulosum IR-94MF1, the Mps++ and the Mps− mutants were developed. As the highest P solubilization activities were observed with the disaccharide sucrose, this C source was used in experiments performed to investigate the effects of different N sources on the solubilization of poorly soluble mineral phosphates by isolate IR-94MF1 [11]. In that study, the use of sucrose as C source in liquid media with HA and FePO4 allowed the detection of citric and gluconic acids. Thus, our results revealed that when using glucose as the sole C source only gluconic acid is produced, whereas in the presence of sucrose two organic acids, citric and gluconic, are implicated in the mineral phosphate solubilization [11].
Acknowledgements
The authors are grateful to Dr. R. Baziramakenga for his help in organic acid determinations. I. Reyes was the recipient of a doctoral fellowship from the Venezuelan Council for Science and Technology (CONICIT) and the Táchira National Experimental University (UNET) of Venezuela. P. Tanguay was the recipient of a scholarship provided by FCAR. This work was supported by grants from FCAR and NSERC.
References