-
PDF
- Split View
-
Views
-
Cite
Cite
Daniel Guntli, Stéphane Burgos, Yvan Moënne-Loccoz, Geneviève Défago, Calystegine degradation capacities of microbial rhizosphere communities of Zea mays (calystegine-negative) and Calystegia sepium (calystegine-positive), FEMS Microbiology Ecology, Volume 28, Issue 1, January 1999, Pages 75–84, https://doi.org/10.1111/j.1574-6941.1999.tb00562.x
- Share Icon Share
Abstract
Calystegines are tropane alkaloids produced by the roots of a few plant species. A bioassay was developed to identify roots with a microbial rhizosphere community capable of calystegine degradation (i.e. MCD roots). In a field survey, the proportion of MCD roots of Zea mays (calystegine-negative) varied from 20 to 80%. In field experiments, the proportions of MCD roots of Z. mays and Calystegia sepium (calystegine-positive) grown in a particular plot were similar to each other but varied with time and, overall, were higher than those of Z. mays roots from adjacent plots free of C. sepium. In autoclaved soil, no root of C. sepium or Z. mays plants propagated as seeds was MCD, indicating that calystegine-degrading microorganisms were not seed-borne. However, MCD roots were found as early as 1 day after planting of rhizomes of C. sepium in autoclaved soil or planting of axenic seedlings of either plant in natural soil microcosms. In total, microorganisms capable of degrading calystegines were harboured not only in the rhizosphere of the calystegine-producing plant but also in that of the calystegine-negative plant and probably in bulk soil.
1 Introduction
Calystegines are plant secondary metabolites and were first found in the roots of Calystegia sepium (L.) R.Br. (i.e. hedge bindweed, morning glory) [1]. Other plant species have also been reported to produce these tropane alkaloids, e.g. Atropa belladonna L. and Convolvulus arvensis L. [1], Hyoscyamus niger L. [2], Morus alba L. [3], Physalis alkengi var. francheti L. [4], Solanum tuberosum L. and Solanum melongena L. [5]. Calystegines can be poisonous to arthropods [5] and mammals [6], presumably as a result of glycosidase inhibition [2,7]. Calystegines may also act as allelochemicals in interactions between plants [8].
Sinorhizobium meliloti strain Rm41 [1] and bacteria from a few other genera, including Pseudomonas[8,9], have been found to degrade calystegines. In strain Rm41, the genes responsible for calystegine catabolism (cac) are harboured on the 225-kb self-transmissible, non-symbiotic plasmid pRme41a [1]. Subcloning revealed that the cac locus is spread over a region 30–40 kb in length [10]. Since S. meliloti Rm41 can use calystegines as a source of carbon and nitrogen for growth [9], it is thought that the genes encoding the catabolism of these alkaloids can contribute to strain survival during its saprophytic life, i.e. in the absence of the host plant alfalfa [10]. In accordance with this concept, Tepfer et al. [9] found calystegine-degrading bacteria in the rhizosphere of calystegine-producing plants but not in that of calystegine-negative plants, suggesting a role for calystegines as nutritional mediators in plant-microbe interactions.
Our understanding of the role of calystegines in the rhizosphere is based exclusively on the results of calystegine degradation tests performed with a limited number of isolates of rhizosphere bacteria only [8,9]. The microbial community of the rhizosphere is highly diverse and it is unlikely that determinations carried out with individual microorganisms could provide a full account of the role of calystegines in plant-microbe interactions. Therefore, the objective of this study was to reevaluate, this time at the microbial community level, the relationship between the ability of plants to produce calystegines and that of rhizosphere microorganisms to degrade calystegines. For this purpose, a new assay was developed to score the capacity of rhizosphere microbial communities to degrade calystegines purified from C. sepium. The assay requires the presence of calystegine-degrading microorganisms at significant population levels for a positive response.
2 Materials and methods
2.1 Plants
Seeds of C. sepium were collected in parks located in the city of Zürich (Switzerland), and those of Z. mays cv. Silex 170 were obtained from the Seeds Department of the Swiss Federal Research Station for Agroecology and Agriculture (FAL) at Zürich-Reckenholz. A culture of C. sepium roots transformed by Agrobacterium rhizogenes[11] was used for the production of calystegines because the introduced T-DNA resulted in increased root biomass and alkaloid content. The transformed roots were cultivated in 65 ml of Gamborg B5 medium (Sigma, Buchs, Switzerland) in 300-ml Erlenmeyer flasks with continuous shaking (120 rpm) at 24°C in the dark for 3 weeks.
2.2 Purification of calystegines
The transformed roots were removed from liquid cultures, blotted dry and stored at −20°C prior to use. For extraction of calystegines, portions of about 5 g of roots were frozen in liquid N2 and homogenised using a mortar and pestle. The samples were transferred into 1.5-ml Eppendorf tubes and centrifuged at 13 000×g for 40 min. To remove tannins and phenols, the supernatants (which contained about one third of the initial amount of plant material) were treated with 45% (w/v) neutral aluminium oxide 90 (E. Merck AG, Dietikon, Switzerland). The samples were mixed with acetonitrile in a 1:9 (v/v) ratio and passed through a LC-NH2 solid phase extraction column (Supelco, Buchs, Switzerland) conditioned with 6 ml of acetonitrile solution (i.e. water:acetonitrile in a 1:9 ratio), to remove salts and ions. The column was washed with 3 ml of acetonitrile solution and elution was performed with 3 ml of 0.001 M HCl. The eluates were then passed through an ion exchange LC-SCX column (Supelco) conditioned with 6 ml of 0.001 M HCl to separate sugars. After washing the column with 3 ml of 0.001 M HCl, the column was rinsed with 2 M NH3. The eluates were evaporated to dryness in vacuo and redissolved each in 250 μl of sterile distilled water.
2.3 Analysis of calystegines
The method of Dräger [12] for calystegine analysis was slightly modified to facilitate identification of the alkaloids. Samples (2 μl) were applied to a thin-layer chromatography plate (Kieselgel 60, Merck), which was developed in a solvent system consisting of CH3OH, 0.6 M NH4Cl and CH3Cl in a 6:2:1 ratio, until the solvent front was 8 cm from the base line. The chromatogram was dried and immersed in a solution prepared with 0.1 ml saturated aqueous AgNO3 and 20 ml acetone for 60 s, before being transferred to a solution of ethanol containing 0.5 M NaOH until brown spots (calystegines) appeared on the white chromatogram (detection limit of about 0.5 μg of calystegines). Finally, the chromatogram was fixed in a bath of photographic fixative G 345 (Agfa-Gevaert AG, Dübendorf, Switzerland). After each bath, the chromatogram was dried using a hair dryer. The purity of each extract was checked by comparison with a calystegine standard obtained from D. Tepfer (INRA Versailles, France) according to published protocols [1]. Calystegine concentration in the extracts was adjusted to 2 μg μl−1 prior to use in degradation assays.
Biological analysis of extracted calystegines was carried out using S. meliloti strain Rm41 and its derivative Rm41-Ca1, which had been cured of the cac plasmid pRme41a and was unable to catabolise calystegines [1]. The strains were grown in liquid tryptone-yeast extract (TY [13]) at 27°C with shaking (120 rpm) until cultures reached an optical density of 1 at 600 nm (equivalent to approximately 7×109 colony-forming units (CFU) ml−1; late exponential phase). The cells were washed and resuspended in fresh M9 medium without carbon source [14]. Calystegine degradation was assayed in reaction mixtures (final volume 208 μl) consisting of 200 μl of M9 medium without carbon source, 8 μl of calystegine extract or calystegine standard (final concentration, 77 μg ml−1) and the test bacterium (cell density, 7×107 CFU ml−1). After incubation for 24 h at 27°C with shaking (120 rpm), the samples were centrifuged (10 min, 13 000×g) and the supernatant evaporated to dryness in vacuo. The residues were taken up in 8 μl sterile distilled water. A 2-μl aliquot was tested for the presence of calystegines by thin-layer chromatography, as described above. For each strain, seven assays were performed with extracted calystegines and seven assays with calystegine standard.
2.4 Bioassay for calystegine degradation by rhizosphere microbiota
To extract rhizosphere microorganisms, samples of about 0.25 g of root (and closely adhering soil) were transferred each into a 50-ml Erlenmeyer flask containing sterile distilled water (sample:water ratio of 1:100 w/v) and the flasks were shaken for 15 min at 350 rpm. Purified calystegines (8-μl aliquots) were added to 200 μl of rhizosphere extract (final concentration, 77 μg ml−1) and the samples (containing microorganisms extracted from 2 mg of root material) were incubated at 27°C with shaking (120 rpm) for 24 h. They were then centrifuged at 13 000×g for 10 min and the supernatant was evaporated to dryness in vacuo. The residue was taken up in 8 μl of sterile distilled water and 2 μl analysed for the presence of calystegines by thin-layer chromatography. The absence of calystegines indicated that the rhizosphere sample studied harboured microorganisms with the capacity to degrade these alkaloids and such roots were scored as being positive for microbial calystegine degradation (i.e. MCD roots). Since the detection limit of the chromatography was about 0.5 μg of calystegines and 2 μl of the 8 μl was tested, it means that the detection limit in the bioassay was about 2 μg of calystegines and that at least 14 μg of the 16 μg of calystegines used in the bioassay (i.e. 88%) had to be degraded before a root was rated MCD.
To demonstrate that the absence of calystegines in samples could not have resulted from physico-chemical interactions (adsorption) between the alkaloid and soil particles, the three soils used in microcosm experiments in this study (Table 1) were each suspended in sterile distilled water (1, 3, 30 and 300 mg soil ml−1). Purified calystegines (8-μl aliquots) were added to 200 μl of each suspension. The samples were vigorously shaken at 1000 rpm for 1.5 h and checked for the presence of calystegines, as described above. Three replicates were studied per treatment. Calystegines were clearly detected in all samples from each non-sterile soil, even when soil was used at a rate as high as 300 mg ml−1 (data not shown). Therefore, the presence of soil particles in rhizosphere extracts could not have resulted in false-positive results in the bioassay.
Eschikon | Reckenholz | Mürren | |
Crop at sampling | maize | maize | potato |
Texture | loam | loam | sandy clay loam |
Clay (%) | 15 | 21 | 27 |
Silt (%) | 42 | 32 | 23 |
Sand (%) | 43 | 47 | 50 |
pHH2O | 7.0 | 7.3 | 6.9 |
Cation-exchange capacity (cmol kg−1) | 32.7 | 18.8 | 49.0 |
CaCO3 (%) | 0.8 | 0 | 0 |
Organic matter (%) | 3.5 | 3.0 | 12.8 |
N (%) | 0.16 | 0.27 | 0.90 |
Altitude (m) above sea level | 530 | 442 | 1590 |
Eschikon | Reckenholz | Mürren | |
Crop at sampling | maize | maize | potato |
Texture | loam | loam | sandy clay loam |
Clay (%) | 15 | 21 | 27 |
Silt (%) | 42 | 32 | 23 |
Sand (%) | 43 | 47 | 50 |
pHH2O | 7.0 | 7.3 | 6.9 |
Cation-exchange capacity (cmol kg−1) | 32.7 | 18.8 | 49.0 |
CaCO3 (%) | 0.8 | 0 | 0 |
Organic matter (%) | 3.5 | 3.0 | 12.8 |
N (%) | 0.16 | 0.27 | 0.90 |
Altitude (m) above sea level | 530 | 442 | 1590 |
All soils were sampled from the surface horizon of cropped cambisols.
Eschikon | Reckenholz | Mürren | |
Crop at sampling | maize | maize | potato |
Texture | loam | loam | sandy clay loam |
Clay (%) | 15 | 21 | 27 |
Silt (%) | 42 | 32 | 23 |
Sand (%) | 43 | 47 | 50 |
pHH2O | 7.0 | 7.3 | 6.9 |
Cation-exchange capacity (cmol kg−1) | 32.7 | 18.8 | 49.0 |
CaCO3 (%) | 0.8 | 0 | 0 |
Organic matter (%) | 3.5 | 3.0 | 12.8 |
N (%) | 0.16 | 0.27 | 0.90 |
Altitude (m) above sea level | 530 | 442 | 1590 |
Eschikon | Reckenholz | Mürren | |
Crop at sampling | maize | maize | potato |
Texture | loam | loam | sandy clay loam |
Clay (%) | 15 | 21 | 27 |
Silt (%) | 42 | 32 | 23 |
Sand (%) | 43 | 47 | 50 |
pHH2O | 7.0 | 7.3 | 6.9 |
Cation-exchange capacity (cmol kg−1) | 32.7 | 18.8 | 49.0 |
CaCO3 (%) | 0.8 | 0 | 0 |
Organic matter (%) | 3.5 | 3.0 | 12.8 |
N (%) | 0.16 | 0.27 | 0.90 |
Altitude (m) above sea level | 530 | 442 | 1590 |
All soils were sampled from the surface horizon of cropped cambisols.
For validation of the bioassay, S. meliloti strain Rm41 and its derivative Rm41-Ca1 were prepared as described above, except that cells were washed and resuspended in sterile distilled water. The cell suspensions were diluted in sterile distilled water, as needed. Samples (5 ml each) of rhizosphere extracts obtained from C. sepium plants sampled at Eschikon (described in Section 2.6) were sterilised by autoclaving. The bioassay was as described above, except that 190 μl of extract was used. Inoculation was performed with 10 μl of cell suspension of strains Rm41 or Rm41-Ca1, and final cell densities were 5×102 to 5×107 CFU ml−1 for strain Rm41 and 5×106 or 5×107 CFU ml−1 for strain Rm41-Ca1. The assay was then carried out as described above. Calystegine degradation was studied for non-sterile rhizosphere extract, as well as sterilised rhizosphere extract inoculated with strains Rm41 or Rm41-Ca1, and seven replicates were used per treatment.
An experiment was done to determine changes in bacterial populations during the 24-h calystegine degradation bioassay. First, the bioassay was performed using non-sterile rhizosphere extracts obtained from Z. mays (15 samples) or C. sepium (15 samples) grown in Eschikon soil. Total numbers of culturable aerobic bacteria were determined by colony counts on 10% tryptic soy agar. Second, the bioassay was carried out with sterilised rhizosphere extract of Z. mays (five samples) or C. sepium (five samples) inoculated with S. meliloti Rm41 at 7.5×106 CFU ml−1. Culturable cells of the strain were enumerated by colony counts on TY agar. All plates were incubated at 27°C for 3 days and the colonies counted.
2.5 Survey of the proportion of MCD Z. mays roots in the field
Five Z. mays fields were chosen at random in a 6×8-km region east of Zürich in July 1996. In each field, a 5-m2 area free of C. sepium was selected and 10 Z. mays plants were sampled. Root segments were collected from the surface horizon, at a depth of approximately 10 cm below the soil surface (one sample of about 0.25 g of root plus closely adhering soil per plant). Each sample was assayed for calystegine degradation, as described above. For each field, the percentage of MCD roots was calculated based on results obtained with the 10 samples.
2.6 Proportion of MCD Z. mays roots in plots with and without C. sepium at two field locations
One Z. mays field at Eschikon [15] and one at Reckenholz [16] were chosen, both soils corresponding to cambisols and both with patchy C. sepium infestation. On 9 July 1996, 10 Z. mays plants were chosen at random at each site from 5-m2 plots infested or not infested with C. sepium. Root segments (one root segment per plant) from these plants, and from C. sepium plants in both infested plots, assayed for calystegine degradation, as described above. The whole experiment was repeated on 24 July 1996 using the same plots.
2.7 Origin of calystegine-degrading rhizosphere microorganisms
The proportion of MCD roots of Z. mays and C. sepium grown in autoclaved soil microcosms was investigated to determine whether calystegine-degrading microorganisms are seed-borne. Surface soil (Table 1) from Eschikon plots infested with C. sepium (see Section 2.6) was passed through a 5-mm mesh screen and transferred into 300-ml Erlenmeyer flasks (65 g soil per flask). The flasks were autoclaved for 20 min at 121°C on two occasions that were 2 days apart. Seeds of C. sepium were collected aseptically from intact fruit capsules and scarified with a sterile scalpel in a sterile hood. Likewise, seeds of Z. mays were used without surface disinfection. All seeds were germinated on 0.85% water agar at 24°C in the dark for 3 days before planting (one seedling per microcosm). The experiment was also performed using C. sepium rhizome instead of seed, since the species can also propagate vegetatively. Rhizome pieces corresponding to two nodes (about 4 cm in length) were washed with sterile distilled water to remove soil particles prior to planting. The flasks were put in a growth chamber with 16 h of light (22°C) and 8 h of dark (18°C). The water content of the soil was approximately 20% (w/w) throughout the experiment. Destructive sampling was carried out at 1, 7 and 21 days after planting and the proportion of MCD root systems was determined. The experiment followed a fully randomised design and five replicates (i.e. five flasks) of each treatment were studied at each sampling time. The experiment was repeated on three independent occasions.
2.8 Proportion of MCD roots of Z. mays and C. sepium in microcosms prepared with soil from fields with calystegine-producing plants
To gain insight into the ability of calystegine-degrading microorganisms to colonise the rhizosphere of calystegine-negative (Z. mays) and calystegine-positive (C. sepium) plants, microcosm experiments were performed using soil (Table 1) from field sites infested with C. sepium (i.e. Eschikon) or free of C. sepium but grown with the calystegine-positive plant S. tuberosum (potato) (i.e. Mürren). The microcosms were prepared as described above, except that soil was not sterilised. The seeds were surface-disinfected to remove any possibility that calystegine-degrading microorganisms associated with the seeds could have colonized the rhizosphere. This was achieved by immersing seeds of C. sepium (after scarification in 95% H2SO4 for 60 min) and Z. mays successively in 5% NaOCl for 15 min and 10% H2O2 for 15 min. Seeds were germinated on 0.85% water agar at 24°C in the dark for 3 days prior to planting (one seedling per microcosm). The experiment was not performed with rhizomes of C. sepium because an effective method for surface disinfection of rhizome is not available. The water content of the soil was approximately 20% (w/w) throughout the experiment. Growth chamber conditions, sampling characteristics and determinations were as described above. A randomised design was used and each treatment was studied in five replicates at each sampling time. The experiment was repeated three times. Similar results were obtained in each of the three repeated experiments (as indicated by non-parametric Kruskal-Wallis statistics) and all data were therefore pooled for statistical analyses.
2.9 Data analysis
The field survey was not replicated and therefore the observations were not analysed statistically. In the field experiment, non-parametric Kruskal-Wallis statistics were used to test the effects of sampling time (9 July 1996 and 24 July 1996), field site (Eschikon and Reckenholz), and plants (C. sepium, Z. mays with and without C. sepium). Mann-Whitney U-tests were used for pairwise comparisons between the three plant treatments (i.e. C. sepium, Z. mays in plots with C. sepium, Z. mays in C. sepium-free plots). Data obtained in non-sterile soil microcosms were analysed by Kruskal-Wallis statistics to determine the effects of soil origin (Eschikon and Mürren) and plant species (Z. mays and C. sepium). Mann-Whitney U-tests were used for pairwise comparisons between the four treatments (Z. mays in Eschikon soil and in Mürren soil, and C. sepium in Eschikon soil and in Mürren soil). All analyses were performed at P= 0.05 and Systat 5.05 (SPSS Inc., Evanston, IL) was used.
3 Results and discussion
3.1 Calystegine extraction
Several methods have been proposed to extract calystegines from the roots of C. sepium or other plant species [1,2,6,12]. In the current work, solid-phase extraction columns were exploited to achieve rapid (1 h) extraction of calystegines from C. sepium roots. One extraction carried out using 5 g of plant material yielded 400–500 μg of purified calystegines. The efficacy of the extraction was similar to that achieved by Dräger [12], who obtained about 100 μg of calystegines per g of fresh root.
The purity of the calystegine extracts obtained here was similar to that of the calystegine standard produced as described by Tepfer et al. [1], as indicated by thin-layer chromatography (data not shown). S. meliloti Rm41 degraded the purified calystegines obtained in the current work as well as the calystegine standard, whereas its Cac− derivative Rm41-Ca1 did not catabolise purified calystegines or the calystegine standard.
3.2 Effectiveness of the bioassay proposed to study calystegine degradation by rhizosphere microbiota
A majority of rhizosphere extracts from rhizomes of C. sepium sampled at Eschikon degraded calystegines in the bioassay proposed here. In contrast, calystegine degradation did not take place when the bioassay was performed with rhizosphere extracts sterilised by autoclaving, indicating microbial calystegine degradation in the original bioassay.
When sterilised rhizosphere extracts were inoculated with a cell suspension of S. meliloti Rm41 prior to conducting the bioassay, calystegine degradation was observed in treatments where the strain was introduced at 5×106 or 5×107 CFU ml−1, but not when at ≤5×105 CFU ml−1. However, full calystegine degradation took place in all treatments with strain Rm41 when assays were incubated for 4 days instead of 24 h, regardless of inoculation levels. Calystegine degradation did not take place when sterilised extracts were inoculated with the Cac− strain Rm41-Ca1, even after incubation for 4 days. These results suggest that complete calystegine degradation requires the presence of calystegine-degrading microorganisms at high cell numbers, and that these calystegine-degrading microorganisms could grow during the bioassay. Indeed, the population size of culturable cells of strain Rm41 increased by two orders of magnitude during the bioassay, regardless of whether rhizosphere extracts were obtained from C. sepium or Z. mays (Table 2). It is possible that the efficiencies of calystegine degradation by strain Rm41 and the resident soil microorganisms differ. However, the finding that full calystegine degradation by S. meliloti Rm41 in the 24-h bioassay took place only when the strain was added at levels of 5×106 CFU ml−1 (corresponding to 5×108 CFU (g root)−1) or higher suggests that the calystegine degradation bioassay may be useful to identify rhizospheres comprising a significant microbial community displaying this catabolic capacity.
Colony countsa of microorganisms in rhizosphere extracts from C. sepium or Z. mays grown in Eschikon soil at the beginning and the end of the 24-h calystegine degradation bioassay
Microorganisms present | Plant species | Colony counts (log CFU ml−1) | |
0 h | 24 h | ||
Resident microorganisms | C. sepium | 5.6±0.3 | 7.8±0.2 |
Z. mays | 6.0±0.5 | 7.5±0.1 | |
S. meliloti strain Rm41b | C. sepium | 5.8 | 8.6±0.2 |
Z. mays | 5.8 | 8.6±0.1 |
Microorganisms present | Plant species | Colony counts (log CFU ml−1) | |
0 h | 24 h | ||
Resident microorganisms | C. sepium | 5.6±0.3 | 7.8±0.2 |
Z. mays | 6.0±0.5 | 7.5±0.1 | |
S. meliloti strain Rm41b | C. sepium | 5.8 | 8.6±0.2 |
Z. mays | 5.8 | 8.6±0.1 |
Means±S.D. are shown. They were calculated after pooling all data from three independent experiments. The plant species had no influence on colony counts of the resident microorganisms (at each sampling), or on colony counts of strain Rm41 at 24 h. Colony counts of the resident microorganisms were not influenced by the MCD status of roots.
Strain Rm41 was added to sterilised rhizosphere extracts at the start of the experiment.
Colony countsa of microorganisms in rhizosphere extracts from C. sepium or Z. mays grown in Eschikon soil at the beginning and the end of the 24-h calystegine degradation bioassay
Microorganisms present | Plant species | Colony counts (log CFU ml−1) | |
0 h | 24 h | ||
Resident microorganisms | C. sepium | 5.6±0.3 | 7.8±0.2 |
Z. mays | 6.0±0.5 | 7.5±0.1 | |
S. meliloti strain Rm41b | C. sepium | 5.8 | 8.6±0.2 |
Z. mays | 5.8 | 8.6±0.1 |
Microorganisms present | Plant species | Colony counts (log CFU ml−1) | |
0 h | 24 h | ||
Resident microorganisms | C. sepium | 5.6±0.3 | 7.8±0.2 |
Z. mays | 6.0±0.5 | 7.5±0.1 | |
S. meliloti strain Rm41b | C. sepium | 5.8 | 8.6±0.2 |
Z. mays | 5.8 | 8.6±0.1 |
Means±S.D. are shown. They were calculated after pooling all data from three independent experiments. The plant species had no influence on colony counts of the resident microorganisms (at each sampling), or on colony counts of strain Rm41 at 24 h. Colony counts of the resident microorganisms were not influenced by the MCD status of roots.
Strain Rm41 was added to sterilised rhizosphere extracts at the start of the experiment.
Bacterial growth took place also when the bioassay was performed using non-sterile rhizosphere extracts of either plant, as indicated by the increase in colony counts of the resident aerobic bacteria during the bioassay (Table 2). Similar numbers of resident culturable aerobic bacteria were obtained whether the samples scored MCD or not (data not shown), suggesting that bacterial growth did not depend on calystegine degradation in this bioassay. Counts of resident culturable bacteria were lower than CFUs of strain Rm41 (Table 2), probably because nutrient availability was higher in autoclaved soil.
3.3 MCD roots of a calystegine-negative plant (Z. mays) can be found in the field
The calystegine degradation bioassay was used to study Z. mays plants sampled at five field sites (Fig. 1Fig. 2). MCD Z. mays roots were found at each location, and their proportion comprised between 20% (Pfäffikon) and 80% (Uster, Seegräben and Auslikon). Overall, calystegine-degrading microorganisms at levels sufficient to achieve full degradation of the alkaloids in the bioassay were present in 32 of the 50 rhizosphere samples studied. C. sepium, the main calystegine-producing plant known in this agrosystem, was not found at any of the five sites.
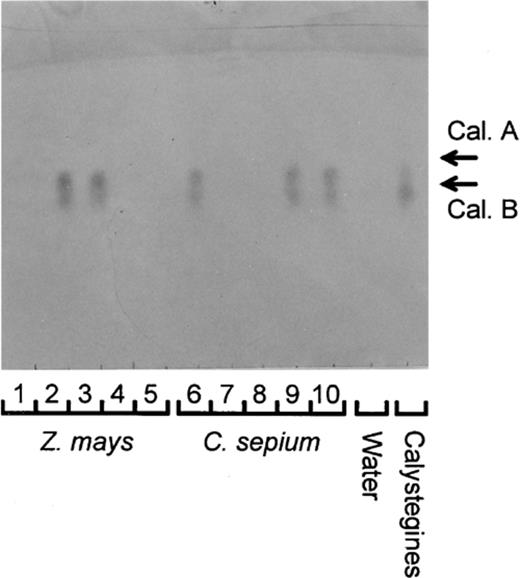
Thin-layer chromatogram for determination of calystegines in the calystegine degradation bioassay. Calystegines (Cal. A and Cal. B) are seen in the calystegine control and in the bioassay carried out with root samples 2, 3 (Z. mays) and 6, 9, 10 (C. sepium). As control, the bioassay was done also without the addition of calystegines (‘Water’). Root samples 1, 4, 5 (Z. mays) and 7, 8 (C. sepium) were scored as MCD as no calystegines were seen.
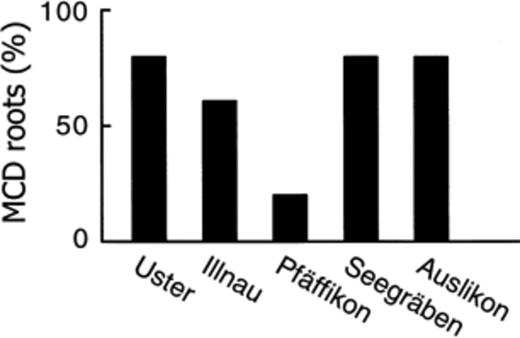
Proportion of MCD Z. mays roots sampled at various locations in the region of Zürich, Switzerland. A plot free of C. sepium was chosen at random at each location and one root sample was assayed for each of 10 plants collected at random in each plot. Overall, the proportion of MCD Z. mays roots in the survey was 64%.
These results were not expected as Z. mays does not produce calystegines. Tepfer et al. [9] did not find any bacterial isolate capable of degrading calystegines in the rhizosphere of plants other than calystegine producers. Similarly, Goldmann et al. [8] observed that 11 of 51 bacterial isolates obtained from the rhizosphere of C. sepium or C. arvensis could degrade calystegines, whereas no calystegine-degrading bacterium was obtained from the rhizosphere of calystegine-negative plants. However, the number of strains investigated in these two studies was limited, because each bacterial isolate had to be tested individually for calystegine degradation. Indeed, the model strain for calystegine degradation is S. meliloti Rm41 [1], which was isolated from a nodule of the calystegine-negative plant Medicago sativa L. and not from C. sepium[17]. The fact that the proportion of MCD Z. mays roots was high (Fig. 2) suggests that microorganisms capable of calystegine degradation are widespread in soil. Based on the results obtained with strain Rm41 in the current bioassay, it can be estimated that calystegine-degrading microorganisms may represent as much as a few percent of all culturable rhizosphere bacteria for roots scored as MCD. From this estimation, it is conceivable that the number of isolates tested in previous studies (less than 100) was not sufficient to detect calystegine-degrading bacteria in the rhizosphere of calystegine-negative plants.
3.4 Presence of C. sepium in the field causes a higher proportion of MCD roots of Z. mays
The effect of C. sepium infestation on the proportion of MCD Z. mays roots was assessed in field experiments where adjacent plots free of C. sepium or infested with C. sepium were available. Whereas data obtained at Eschikon were similar to those from Reckenholz, sampling time had a strong influence on the proportions of MCD roots (Fig. 3). Apparently, calystegine-degrading microorganisms became favoured for colonisation of the rhizosphere of Z. mays by the second sampling, perhaps as a consequence of specific climatic conditions or changes in exudation patterns of Z. mays roots linked to plant development between the two samplings. Since Z. mays is calystegine-negative, this effect appears to be independent of the presence of calystegines in the rhizosphere. Overall, the proportion of MCD Z. mays roots was statistically higher in plots infested with the bindweed than in those without C. sepium (Fig. 3), obviously a result contributed by data from the first sampling. In C. sepium-infested plots, C. sepium and Z. mays displayed a similar proportion of MCD roots.
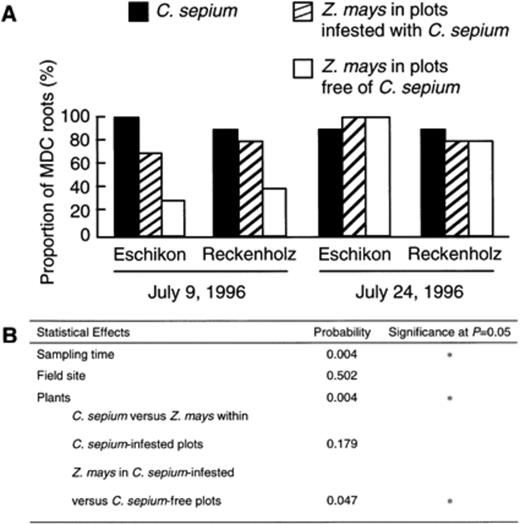
Proportion (%) of MCD roots of Z. mays and C. sepium in field experiments carried out at Eschikon and at Reckenholz (A). Z. mays was sampled from plots infested with C. sepium and from plots without bindweed. The statistical effect of the experimental factors were studied by Kruskal-Wallis and Mann-Whitney U statistics (B).
The presence of C. sepium plants in these plots implies that calystegines could be released into soil as part of root exudates and/or in decaying roots of C. sepium. The presence of calystegines in soil organic matter may sustain the presence of calystegine-degrading microorganisms at significant population levels in non-rhizosphere soil and contribute to their subsequent colonisation of the rhizosphere of a calystegine-negative plant like Z. mays. Unfortunately, no information is available on the persistence of calystegines released into soil after the death of roots. Other types of alkaloids have been shown to persist in soil and become adsorbed to soil particles [18]. The ability of bacteria to catabolise a specific compound present in root exudates, e.g. opine, rhizopine or flavonoids, is thought to improve their growth [19,20] or competitiveness in the rhizosphere [21–22,23]. Calystegines may have a wider significance by favouring calystegine-degrading microorganisms not only in the rhizosphere of calystegine-producing plants but perhaps also in bulk soil containing residual calystegines.
3.5 Calystegine-degrading rhizosphere microorganisms are not seed-propagated
No root of C. sepium or Z. mays was MCD when autoclaved Eschikon soil was sown with seeds. Since the majority of roots of either plant species were MCD at the Eschikon field site (Fig. 3), this result indicates that the rhizosphere microorganisms responsible for the ability of rhizosphere extracts to degrade calystegines do not belong to the microbiota naturally associated with the seed. Indeed, a significant proportion of C. sepium samples were MCD when autoclaved Eschikon soil was planted with washed rhizomes of the plant (i.e. 25±3%, 50±3% and 35±17% at 1, 7 and 21 days after planting, respectively; results pooled from three independent experiments). Furthermore, this indicates that calystegine-degrading microorganisms can be present in close association with the surface of the C. sepium rhizome.
3.6 High proportions of MCD roots of Z. mays and C. sepium grown in microcosms prepared with soil from fields where calystegine-producing plants are present
In soil microcosms, a significant proportion of seedlings were already MCD 1 day after planting of axenic seedlings in Eschikon or Mürren soil, regardless of whether C. sepium or Z. mays was studied (Fig. 1,Table 3). This suggests that calystegine-degrading microorganisms were present at significant numbers in soil before the introduction of the plants, as suggested earlier for soils from Eschikon and Reckenholz (see Section 3.4). The presence of microorganisms capable of calystegine degradation in C. sepium-free soil from Mürren (1600 m above sea level) cannot be explained by earlier C. sepium infestation since this plant species does not grow at this altitude in Switzerland. However, the Mürren soil has been cropped continuously with S. tuberosum, another calystegine-producing plant species [5].
Proportion (%)a of roots of C. sepium and Z. mays with a microbial rhizosphere community capable of calystegine degradation (i.e. MCD roots) in natural soil microcosms prepared with Eschikon soil (site infested with C. sepium) or Mürren soil (site cropped with S. tuberosum)
Soil origin | Plant speciesb | Sampling time | ||
Day 1 | Day 7 | Day 21 | ||
Eschikon | C. sepium | 33±12 ac | 47±12 ab | 58±8 a |
Z. mays | 40±0 a | 13±12 a | 18±17 b | |
Mürren | C. sepium | 53±12 a | 60±20 b | 58±22 a |
Z. mays | 53±42 a | 67±31 b | 43±12 ab | |
Statistical effects | Soil origin | NSd | * | NS |
Plant species | NS | NS | * |
Soil origin | Plant speciesb | Sampling time | ||
Day 1 | Day 7 | Day 21 | ||
Eschikon | C. sepium | 33±12 ac | 47±12 ab | 58±8 a |
Z. mays | 40±0 a | 13±12 a | 18±17 b | |
Mürren | C. sepium | 53±12 a | 60±20 b | 58±22 a |
Z. mays | 53±42 a | 67±31 b | 43±12 ab | |
Statistical effects | Soil origin | NSd | * | NS |
Plant species | NS | NS | * |
Means±S.D. are shown.
Seeds of C. sepium were scarified with H2SO4 before surface disinfection. Seeds of Z. mays and C. sepium were surface-disinfected with 5% NaOCl and 10% H2O2.
At each sampling time, the statistical relationships between the four treatments is shown with the letters a and b (P= 0.05).
NS, not significant; *, significant at P= 0.05.
Proportion (%)a of roots of C. sepium and Z. mays with a microbial rhizosphere community capable of calystegine degradation (i.e. MCD roots) in natural soil microcosms prepared with Eschikon soil (site infested with C. sepium) or Mürren soil (site cropped with S. tuberosum)
Soil origin | Plant speciesb | Sampling time | ||
Day 1 | Day 7 | Day 21 | ||
Eschikon | C. sepium | 33±12 ac | 47±12 ab | 58±8 a |
Z. mays | 40±0 a | 13±12 a | 18±17 b | |
Mürren | C. sepium | 53±12 a | 60±20 b | 58±22 a |
Z. mays | 53±42 a | 67±31 b | 43±12 ab | |
Statistical effects | Soil origin | NSd | * | NS |
Plant species | NS | NS | * |
Soil origin | Plant speciesb | Sampling time | ||
Day 1 | Day 7 | Day 21 | ||
Eschikon | C. sepium | 33±12 ac | 47±12 ab | 58±8 a |
Z. mays | 40±0 a | 13±12 a | 18±17 b | |
Mürren | C. sepium | 53±12 a | 60±20 b | 58±22 a |
Z. mays | 53±42 a | 67±31 b | 43±12 ab | |
Statistical effects | Soil origin | NSd | * | NS |
Plant species | NS | NS | * |
Means±S.D. are shown.
Seeds of C. sepium were scarified with H2SO4 before surface disinfection. Seeds of Z. mays and C. sepium were surface-disinfected with 5% NaOCl and 10% H2O2.
At each sampling time, the statistical relationships between the four treatments is shown with the letters a and b (P= 0.05).
NS, not significant; *, significant at P= 0.05.
In Mürren soil, the proportion of MCD roots remained similar for C. sepium and Z. mays at the other two samplings carried out (Table 3). In contrast, the proportion of MCD Z. mays roots in Eschikon soil decreased significantly by day 7 and did not change from days 7 to 21, whereas the proportion of MCD roots of C. sepium increased somewhat with time. Consequently, MCD roots were three times more frequent for C. sepium than for Z. mays at 21 days after sowing. Obviously, calystegine-degrading microorganisms were more competitive for colonisation of C. sepium than Z. mays in this soil. The reason why this did not take place in Mürren soil remains to be determined. Perhaps the amount of calystegines in a soil under continuous, intensive S. tuberosum cultivation (Mürren) was higher than that resulting from natural C. sepium infestation (Eschikon), because of the amount of calystegine-containing crop residues produced in the case of potato cultivation. Consequently, in the rhizosphere, the calystegine status of the plants was more influential in Eschikon soil than in Mürren soil.
3.7 Significance of calystegines and calystegine degradation capacity for soil microorganisms
Microorganisms with the capacity to degrade organic substrates specific for certain plant roots are considered to be favoured for colonisation of the rhizosphere of these plants [19–23], including C. sepium[8–9,10]. However, MCD roots of Z. mays, a calystegine-negative plant, were commonly found. Apparently, calystegine-degrading microorganisms were neither restricted to the rhizosphere of calystegine-producing plants nor impaired for colonisation of the rhizosphere of calystegine-negative plants. So far, only rhizobia and pseudomonads have been identified among rhizosphere isolates capable of calystegine degradation [8,9]. Calystegine degradation by bacteria other than S. meliloti Rm41 does not involve the cac genes, as in the latter strain [8,9], raising the possibility that the rhizosphere microbiota involved in calystegine degradation is highly diverse in terms of both microbial taxonomy and catabolic pathways.
The occurrence of MCD roots of Z. mays and C. sepium shortly (1 day) after introduction of axenic seedlings into natural soil suggests that calystegine-degrading microorganisms were present at significant population levels in soil, perhaps because of the presence of calystegines in soil organic matter following the death of roots containing these alkaloids. If this were the case, it could provide an explanation for the higher proportion of MCD Z. mays roots in field plots infested with C. sepium compared with plots not infested. In this context, the ability of symbiotic bacteria such as S. meliloti Rm41 to catabolise calystegines would constitute not only an adaptation to alternative, non-symbiotic host plants but probably also a selective advantage for survival in soil in the absence of roots (i.e. in bulk soil).
Acknowledgements
We are indebted to D. Tepfer (INRA Versailles, France) for kindly providing calystegine standard, root culture of transformed C. sepium and strains Rm41 and Rm41-Ca1. We thank J. von Allmen for providing soil from Mürren, H.P. Pfirter for discussions and technical assistance, and U. Schenk and M. Hildman for technical assistance. This work was supported in part by the COST Action 816 (European cooperation in the field of scientific and technical research).
References
Author notes
Present address: UMR CNRS d'Ecologie Microbienne du Sol, Université Claude Bernard (Lyon 1), 69622 Villeurbanne Cedex, France.