-
PDF
- Split View
-
Views
-
Cite
Cite
Yongyi Tong, Bruce Lighthart, Effect of simulated solar radiation on mixed outdoor atmospheric bacterial populations, FEMS Microbiology Ecology, Volume 26, Issue 4, August 1998, Pages 311–316, https://doi.org/10.1111/j.1574-6941.1998.tb00515.x
- Share Icon Share
Abstract
Slit and Andersen cascade impact samplers were used to collect ambient atmospheric bacteria under clear conditions at noon and night onto Luria-Bertani agar surfaces. The bacteria were then directly exposed to simulated solar radiation (SSR) for increasing periods to evaluate the lethal effect of SSR. The results showed that SSR had marked differential lethal effects depending on the accumulated total solar irradiance, solar spectral property, bacterial species composition, and bacterial laden particle size. The simulated extraterrestrial solar spectrum had a greater lethality than the simulated terrestrial solar spectrum on the atmospheric bacteria collected at night (P<0.05); no such difference was found for the bacteria collected at noon. Statistical differences found only for the bacteria collected at night indicates that daytime solar radiation exposure could eliminate some sensitive bacterial populations from the ambient atmosphere. Sunlight exposure survival of the bacteria collected at noon linearly increased with respect to particle size. For each micrometer increase in particle aerodynamic diameter, there is an almost 11% increase in survival up to at least 7 µm aerodynamic diameter particles. The higher sunlight sensitivity of airborne bacteria in small particles could provide a partial explanation for the preponderance of large-sized culturable bacterial particles in the atmosphere during clear days.
1 Introduction
Although visible and infrared electromagnetic radiation may be damaging and/or lethal, the ultraviolet radiation (UV, 250–400 nm) waveband is more harmful to microorganisms [1–3]. Pure cultures of airborne bacteria are damaged by UV and solar radiation (SR; [4–8]), but may recover from UV damage while in an airborne state [9]. Because of their high sensitivity to UV, it has been suggested that pure cultures of certain bacteria would make good for solar UV radiation dosimeters [10–12].
Living bacteria have been found as free single cells, but are more commonly clumped or ‘rafted’ on pieces of plant or soil debris in the atmosphere from ground to at least 77 km [13–19]. The relatively small particle size on which airborne bacteria are ‘rafted’ (1–20 μm) and the strong solar irradiance along their trajectory, especially when transported to a higher altitude [20], may contribute to the damaging and lethal consequence of SR exposure. Therefore, we hypothesize that SR is an important environmental factor affecting the survival and dynamics of atmospheric bacteria. With the stratospheric ozone depletion and increased UV penetration [20–22], one might expect natural mixed populations of airborne bacteria to be vulnerable and therefore a potentially sensitive component, or indicator of environmental alteration.
This report describes experiments in which particle size differentiated or undifferentiated natural populations of outdoor atmospheric bacteria are exposed to two simulated SR (SSR) spectra: (a) the extraterrestrial SR spectrum, and (b) the terrestrial SR spectrum. The major difference between the two SSR spectra is their UV composition, which reflects the modification effect of the earth's atmosphere on the SR spectrum (Table 1). This study is aimed at investigating the influence of solar spectral property and bacteria laden particle size on SR lethality of outdoor atmospheric bacteria.
Output spectral distribution of simulated extraterrestrial and terrestrial solar radiation
Light | Wave range (nm) | Extraterrestrial SSR (%) | Terrestrial SSR (%) | Extraterrestrial/terrestrial |
UVC | <280 | 0.11 | 0.01 | 11.00 |
UVB | 280–320 | 0.60 | 0.18 | 3.33 |
UVA | 320–400 | 4.90 | 3.32 | 1.48 |
Visible | 400–700 | 38.20 | 37.50 | 1.02 |
Infrared | 700–2500 | 56.40 | 59.50 | 0.95 |
Light | Wave range (nm) | Extraterrestrial SSR (%) | Terrestrial SSR (%) | Extraterrestrial/terrestrial |
UVC | <280 | 0.11 | 0.01 | 11.00 |
UVB | 280–320 | 0.60 | 0.18 | 3.33 |
UVA | 320–400 | 4.90 | 3.32 | 1.48 |
Visible | 400–700 | 38.20 | 37.50 | 1.02 |
Infrared | 700–2500 | 56.40 | 59.50 | 0.95 |
Data are from the catalog of Oriel Corporation (1993).
Output spectral distribution of simulated extraterrestrial and terrestrial solar radiation
Light | Wave range (nm) | Extraterrestrial SSR (%) | Terrestrial SSR (%) | Extraterrestrial/terrestrial |
UVC | <280 | 0.11 | 0.01 | 11.00 |
UVB | 280–320 | 0.60 | 0.18 | 3.33 |
UVA | 320–400 | 4.90 | 3.32 | 1.48 |
Visible | 400–700 | 38.20 | 37.50 | 1.02 |
Infrared | 700–2500 | 56.40 | 59.50 | 0.95 |
Light | Wave range (nm) | Extraterrestrial SSR (%) | Terrestrial SSR (%) | Extraterrestrial/terrestrial |
UVC | <280 | 0.11 | 0.01 | 11.00 |
UVB | 280–320 | 0.60 | 0.18 | 3.33 |
UVA | 320–400 | 4.90 | 3.32 | 1.48 |
Visible | 400–700 | 38.20 | 37.50 | 1.02 |
Infrared | 700–2500 | 56.40 | 59.50 | 0.95 |
Data are from the catalog of Oriel Corporation (1993).
2 Materials and methods
2.1 Sampling atmospheric bacteria
Natural atmospheric bacteria were collected on the top (5 m above ground level) of an isolated small building located ∼5 km south of Corvallis, OR. The location had a large grass field to the southwest and a deciduous (oak, maple and ash) and evergreen (Douglas fir) mixed forest nearby in all other directions. Samples from this location were taken either under clear conditions at noon or midnight during the autumn of 1995 (Table 2).
Simulated SR spectrum | Air mass filter of solar simulator | SR intensity (W m−2) | Agar surface temperature (°C) | Sampling time | Sampler |
Extraterrestrial SR spectrum | AM0 | 847.2 | 38–40 | Oct 1, 1995 (clear noontime) | slit |
Oct 4, 1995 (midnight) | slit | ||||
1180.8 | 42–43 | Oct 28, 1995 (clear noontime) | Andersen | ||
Terrestrial SR spectrum | AM0+ | 494.6 | 30–31 | Sept 10, 1995 (clear noontime) | slit |
AM1D | Sept 13, 1995 (midnight) | slit |
Simulated SR spectrum | Air mass filter of solar simulator | SR intensity (W m−2) | Agar surface temperature (°C) | Sampling time | Sampler |
Extraterrestrial SR spectrum | AM0 | 847.2 | 38–40 | Oct 1, 1995 (clear noontime) | slit |
Oct 4, 1995 (midnight) | slit | ||||
1180.8 | 42–43 | Oct 28, 1995 (clear noontime) | Andersen | ||
Terrestrial SR spectrum | AM0+ | 494.6 | 30–31 | Sept 10, 1995 (clear noontime) | slit |
AM1D | Sept 13, 1995 (midnight) | slit |
Simulated SR spectrum | Air mass filter of solar simulator | SR intensity (W m−2) | Agar surface temperature (°C) | Sampling time | Sampler |
Extraterrestrial SR spectrum | AM0 | 847.2 | 38–40 | Oct 1, 1995 (clear noontime) | slit |
Oct 4, 1995 (midnight) | slit | ||||
1180.8 | 42–43 | Oct 28, 1995 (clear noontime) | Andersen | ||
Terrestrial SR spectrum | AM0+ | 494.6 | 30–31 | Sept 10, 1995 (clear noontime) | slit |
AM1D | Sept 13, 1995 (midnight) | slit |
Simulated SR spectrum | Air mass filter of solar simulator | SR intensity (W m−2) | Agar surface temperature (°C) | Sampling time | Sampler |
Extraterrestrial SR spectrum | AM0 | 847.2 | 38–40 | Oct 1, 1995 (clear noontime) | slit |
Oct 4, 1995 (midnight) | slit | ||||
1180.8 | 42–43 | Oct 28, 1995 (clear noontime) | Andersen | ||
Terrestrial SR spectrum | AM0+ | 494.6 | 30–31 | Sept 10, 1995 (clear noontime) | slit |
AM1D | Sept 13, 1995 (midnight) | slit |
Two types of airborne microbial samplers were used to collect atmospheric bacteria: slit samplers (S-T-A Biological Air Sampler, New Brunswick Scientific Co., Inc., Edison, NJ; 15 cm diameter petri dish) and Andersen cascade impact samplers (Flow sensor 15–500 model, Andersen Samplers, Inc., Atlanta, GA; 10 cm diameter petri dish). The slit samplers collected a temporal distribution while the Andersen samplers collected a particle size distribution of airborne bacterial particles [23]. To minimize any unwanted light effects of the slit samplers, the plexiglass bonnets were covered with aluminum foil and duct tape. The culture medium was Luria-Bertani agar (LB; Difco Laboratories, Inc., Detroit, MI) amended with cycloheximide (200 μg/ml; Sigma Chemical Co., St. Louis, MO). The agar concentration in the medium was 1.5% for slit samplers, but increased to 2.0% for Andersen samplers. It was thought that higher agar content might reduce imbedding of bacterial particles in the agar during the prolonged impaction process, which would protect them from later SR exposure effects. Before loading the petri dishes into the samplers, each stage of the Andersen samplers and the slit barrel, the bonnet and turntable of the slit samplers were sterilized with 70% ethanol.
Six samplers of each kind were arranged in a line perpendicular to the prevailing wind, and run concurrently. Depending on the expected bacterial particle concentration in the atmosphere, the slit samplers were run at 47.5 L/min for 20–60 min to impact 50–500 bacterial particles per petri dish, while the Andersen samplers were run at 28.3 L/min for 3 h to collect 20–200 bacterial particles on different stages.
To prevent any sunlight effects on the collected bacteria, the plates were removed from the sampler, and placed in light-tight boxes within a dark chamber. The bacteria collected on petri dishes were either immediately exposed to SSR, or stored at 4°C for less than 24 h, and then exposed.
2.2 Exposure to simulated solar radiation
A 300-W Xenon lamp solar simulator (Model 8160, Oriel Corporation, Stratford, CT) with airmass zero (AM0) and airmass one direct (AM1D) filters were used to simulate extraterrestrial and terrestrial SR spectra. A recently factory-calibrated radiometer (Model IL440, International Light Co., Inc., Newburyport, MA) was employed to measure SR intensity on the exposure area. The quality and intensity of simulated solar radiation on the exposure area are shown in Tables 1 and 2.
Agar temperature was measured in a separate experiment by placing a mercury thermometer on the exposed agar surface. To avoid photoreactivation of the SSR-exposed bacteria due to stray light during laboratory manipulations, a 15-W tungsten safe-light covered with a straw-colored filter (P39380, Edmund Scientific Co., Inc., Barrington, NJ) was used to illuminate the laboratory. The filter removes most of the photoreactivation stimulating light from the lamp [24].
An open-lid sample plate was positioned on a black plastic box 15 cm below the collimating lens of the solar simulator. The black box was used to absorb the incoming SR, thereby reducing the effect of reflected SR on the bacteria. The bacteria deposited on the entire agar surface of the Andersen sampler's plates (diameter: 10 cm) were exposed to SSR, while only those on the central region of the slit sampler's plates (diameter: 15 cm) were exposed and counted due to the limited output area of the solar simulator. Exposure times were 0, 5, 15, 35, 75, and 120 min for slit sampler plates, and 30 min for Andersen sampler plates. Although the laboratory air was filtered and clean throughout the experiments, a room-air fallout control petri dish was placed beside each of the exposed plates during radiation exposure.
Bacterial colony forming units (CFUs) were counted using a colony counter (Spiral System Inc., Cincinnati, OH) after a 7-day dark incubation period at 25°C.
2.3 Analysis
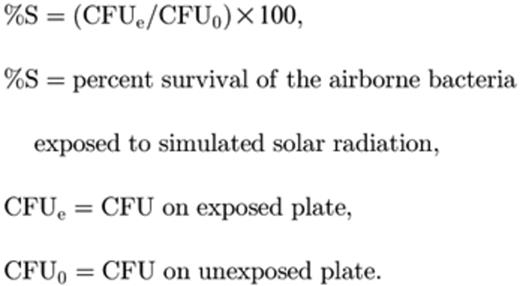
Exposure of airborne bacteria on plates in a 42–44°C water bath for 30 min or at 38–40°C for 120 min did not show any significant effect on bacterial survival (data not shown here), therefore temperature effect on bacteria is not considered in this equation.
3 Results
The lethal effects of SSR on the atmospheric bacteria collected at different times of day were shown in Fig. 1 and described in the equations in Table 3. A significant linear relationship was found between percent survival (%S) of the bacteria and accumulated solar irradiances (Fig. 1; Table 3). Statistical tests of bacterial decay rates (i.e. slope) showed that the simulated extraterrestrial solar spectrum has a significantly greater lethal effect than the simulated terrestrial solar spectrum for bacteria collected at night (P<0.05); no such difference was observed for the cloudless noontime collected bacteria (Fig. 1).
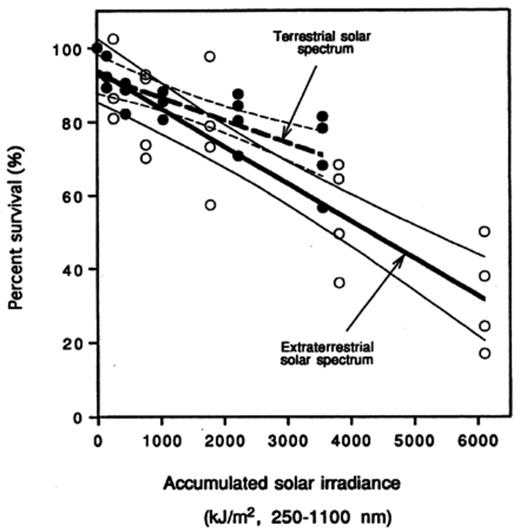
Effects of simulated solar radiation (SSR) on the survival of natural populations of ambient outdoor atmospheric bacteria collected at nighttime during the autumn of 1995 in Corvallis, OR. The two SSR conditions are: (a) the extraterrestrial SR spectrum, and (b) the terrestrial SR spectrum. The 95% confidence ranges were shown for each SSR.
Linear regression fits of noon- and nighttime collected atmospheric bacteria exposed to stimulated extraterrestrial and terrestrial solar irradiation
Simulated solar spectrum | Collection time | n | Linear regression equations (KiSR m−1) | r2 | P |
Extraterrestrial | noon | 16 | %survival=99.82±6.47−(0.51±0.04) | 0.93 | <0.01 |
Extraterrestrial | night | 21 | %survival=93.93±12.6−(0.52±0.06) | 0.77 | <0.01 |
Terrestrial | noon | 28 | %survival=96.03±7.43−(0.26±0.04) | 0.64 | <0.01 |
Terrestrial | night | 18 | %survival=92.87±6.78−(0.18±0.04) | 0.61 | <0.01 |
Simulated solar spectrum | Collection time | n | Linear regression equations (KiSR m−1) | r2 | P |
Extraterrestrial | noon | 16 | %survival=99.82±6.47−(0.51±0.04) | 0.93 | <0.01 |
Extraterrestrial | night | 21 | %survival=93.93±12.6−(0.52±0.06) | 0.77 | <0.01 |
Terrestrial | noon | 28 | %survival=96.03±7.43−(0.26±0.04) | 0.64 | <0.01 |
Terrestrial | night | 18 | %survival=92.87±6.78−(0.18±0.04) | 0.61 | <0.01 |
Linear regression fits of noon- and nighttime collected atmospheric bacteria exposed to stimulated extraterrestrial and terrestrial solar irradiation
Simulated solar spectrum | Collection time | n | Linear regression equations (KiSR m−1) | r2 | P |
Extraterrestrial | noon | 16 | %survival=99.82±6.47−(0.51±0.04) | 0.93 | <0.01 |
Extraterrestrial | night | 21 | %survival=93.93±12.6−(0.52±0.06) | 0.77 | <0.01 |
Terrestrial | noon | 28 | %survival=96.03±7.43−(0.26±0.04) | 0.64 | <0.01 |
Terrestrial | night | 18 | %survival=92.87±6.78−(0.18±0.04) | 0.61 | <0.01 |
Simulated solar spectrum | Collection time | n | Linear regression equations (KiSR m−1) | r2 | P |
Extraterrestrial | noon | 16 | %survival=99.82±6.47−(0.51±0.04) | 0.93 | <0.01 |
Extraterrestrial | night | 21 | %survival=93.93±12.6−(0.52±0.06) | 0.77 | <0.01 |
Terrestrial | noon | 28 | %survival=96.03±7.43−(0.26±0.04) | 0.64 | <0.01 |
Terrestrial | night | 18 | %survival=92.87±6.78−(0.18±0.04) | 0.61 | <0.01 |
Evaluation of particle size effect on the survival of bacteria collected at noon showed a positive linear regression (r2=0.92, n= 19) with particle size where, for a micrometer increase in aerodynamic particle diameter, there is an approximately 11% increase in survival up to at least 7 μm aerodynamic diameter (Fig. 2).

Lethal effect of a 30-min exposure of the outdoor atmospheric bacteria ‘rafted’ on different-sized aerodynamic particles to simulated extraterrestrial solar radiation. Dotted lines are 95% confidence ranges.
4 Discussion
This study showed that SSR had marked differential lethal effects on the mixed populations of outdoor airborne bacteria depending on the accumulated total solar irradiance, solar spectral properties, and bacterial laden particle size. This result could be explained by the high UV waveband proportion, especially UVC and UVB in the simulated extraterrestrial SR spectrum (Table 1). The statistical difference found only for the bacteria collected at night suggests that some SR sensitive bacterial populations might have been reduced during daytime by SR exposure. Therefore collected bacterial populations at night appear more sensitive to UV increases than those collected at noon.
UVC, UVB, and UVA in the simulated extraterrestrial SR spectrum was greater by about 11.0, 3.3 and 1.5 times, respectively, than in the simulated terrestrial SR spectrum (Table 1). Although we could not determine which UV wavelength(s) were primarily responsible for the bacterial lethality increase in this experiment, the results suggest that the increased UV due to stratospheric ozone loss could have some influence on the microbiota of the earth's atmosphere. In the future, more specific experiments, e.g. only increasing UVB to different ozone depletion levels in SR [20–22], need to be done to explore this problem.
As found in the Andersen collected samples, survival of airborne bacteria is directly associated with particle size (Fig. 2). The increased survival of bacteria in larger particles may be explained in two ways: (1) by a light-shielding effect of surface situated particle components for the inside viable cells, and/or (2) a greater probability of SR resistant bacterial species contained in the larger particles that contain more bacteria. Differential SR sensitivity of small as compared to large particle containing bacteria might be a selective survival mechanism that could be a partial explanation for the preponderance of larger-sized viable bacteria-associated particles in the atmosphere during clear days [25,26].
Acknowledgements
We would like to thank Ms. Brenda T. Shaffer and Mrs. Lynn Rogers of Dynamac Corporation, and Mr. Richard Kretz of NAPCA for their technical assistance. We also want to thank Dr. Charles Wick of the US Army's Aberdeen Proving Ground, MD for loaning us the solar simulator.
References
Author notes
Research performed during a National Research Council/US Environmental Protection Agency Associateship.
Present address: Microbial Aerosol Research Lab, Monmouth, OR 97361, USA.