-
PDF
- Split View
-
Views
-
Cite
Cite
Shajil Chalil, Paul W.X. Foley, Sarkaw A. Muyhaldeen, Kiran C.R. Patel, Zaheer R. Yousef, Russell E.A. Smith, Michael P. Frenneaux, Francisco Leyva, Late gadolinium enhancement-cardiovascular magnetic resonance as a predictor of response to cardiac resynchronization therapy in patients with ischaemic cardiomyopathy, EP Europace, Volume 9, Issue 11, November 2007, Pages 1031–1037, https://doi.org/10.1093/europace/eum133
- Share Icon Share
Abstract
To determine whether myocardial scarring, quantified using late gadolinium enhancement-cardiovascular magnetic resonance (LGE-CMR), predicts response to cardiac resynchronization therapy (CRT).
A total of 45 patients with ischaemic cardiomyopathy [age 67.1 ± 10.4 years (mean ± SD)] underwent assessment of 6 min walking distance (6MWD) and quality of life (QoL) before and after CRT. Scar size (percentage of left ventricular mass), location, and transmurality were assessed prior to CRT using LGE-CMR. Responders (survived for 1year with no heart failure hospitalizations, and improvement by ≥1 NYHA classes or ≥25% 6MWD) had a higher left ventricular ejection fraction (P = 0.048), smaller scars (<33%) (P = 0.009), and fewer scars with ≥51% transmurality (P = 0.002). Scar size correlated negatively with change in 6MWD (r = −0.54, P < 0.001) and positively with changes in QoL scores (r = 0.35, P = 0.028). Responder rates in patients with <33% scar were higher than in those with ≥33% scar (82 vs. 35%, P < 0.01). Responder rates in patients with scar transmurality <51% were higher than in those with ≥51% (89 vs. 46%, P < 0.01). Among the patients with posterolateral scars, a transmurality value of ≥51% was associated with a particularly poor response rate (23%), compared with scars with <51% transmurality (88%, P < 0.001). In multivariate analyses, both scar size (P = 0.022) and transmurality (P = 0.004) emerged as predictors of response. In patients with posterolateral scars, pacing outside the scar was associated with a better responder rate than pacing over the scar (86 vs. 33%, P = 0.004).
In patients with ischaemic cardiomyopathy, a scar size ≥33%, a transmurality ≥51%, and pacing over a posterolateral scar are associated with a suboptimal response to CRT.
Introduction
The Cardiac Resynchronisation Heart Failure (CARE-HF) study has recently shown that, in patients with heart failure, cardiac resynchronization therapy (CRT) leads to a 36% reduction in all-cause mortality.1 Other benefits include an improvement in symptoms and a reduction in hospitalizations.1–3 It is well recognized, however, that the clinical response to CRT is difficult to predict, with as many as 20–30% of patients failing to respond.4 Because CRT appears to work by reducing mechanical dyssynchrony, increasing attention has been focused on the latter as a possible predictor of clinical response to CRT. QRS duration was considered a surrogate measure of mechanical dyssynchrony, but recent studies refute this assumption.5,6 Numerous methods of assessing mechanical dyssynchrony directly using echocardiography have also emerged.5–9 Using such techniques, some reports have demonstrated that clinical benefit is predicted by the magnitude of mechanical dyssynchrony.4 Importantly, however, not all patients with dyssynchrony improve following CRT.
In the field of revascularization, it is well established that functional myocardial recovery is inversely related to the extent of myocardial scarring.10 In a study using late gadolinium-enhancement-cardiovascular magnetic resonance (LGE-CMR), Bleeker et al.11 have recently shown that in patients with a transmural posterolateral left ventricular (LV) scar, CRT did not lead to a reduction in LV dyssynchrony or to clinical improvement. In this study, we have assessed the impact of scar size as well as location and transmurality on the response to CRT. The effect of lead position in relation to scar tissue and to clinical benefit was also assessed.
Methods
Patients
The study was carried out at Good Hope Hospital. Subjects were recruited during the period from July 2002 to March 2006, during which 161 patients underwent CRT, 118 of whom had ischaemic cardiomyopathy and 43 of whom had non-ischaemic cardiomyopathy. Patients who underwent successful biventricular pacemaker implantation and LGE-CMR were included in this study. The following inclusion criteria were adopted: (1) heart failure because of coronary heart disease; (2) New York Heart Association (NYHA) class III or IV; (3) attendance to a dedicated heart failure clinic to achieve maximum tolerated treatment with diuretics, angiotensin-converting enzyme inhibitors or angiotensin-II receptor blockers, beta-blockers, spironolactone, and digoxin; (4) a left bundle branch block, with a QRS duration ≥120ms; and (5) left ventricular ejection fraction (LVEF) ≤35%. Exclusion criteria included (1) contraindications to cardiac pacing, (2) myocardial infarction or acute coronary syndrome within the previous 3 months, (3) presence of or indications for an implantable cardioverter-defibrillator, (4) severe structural valvular heart disease, (5) presence of co-morbidities likely to threaten survival for 12months, (6) pulmonary oedema requiring iv diuretics in the previous week, and (7) intermittent or chronic atrial fibrillation or atrial flutter. All participants had undergone coronary angiography. The clinical diagnosis of heart failure (HF) was made on the basis of documented evidence of LV systolic dysfunction on echocardiography. The diagnosis of ischaemic cardiomyopathy was made if LV systolic dysfunction was associated with a history of myocardial infarction12 and if there was angiographically documented coronary heart disease (>50% stenosis in ≥1 coronary arteries). The findings of LGE-CMR were also used to distinguish between ischaemic from non-ischaemic cardiomyopathy, as suggested by Assomull et al.13 Patients with LV dysfunction in combination with the finding of transmural or subendocardial late gadolinium uptake were classified as having ischaemic cardiomyopathy, whereas patients with LV dysfunction and no gadolinium uptake, patchy uptake, or mid-wall hyperenhancement were classified as having non-ischaemic cardiomyopathy. The latter were excluded from the study. The study conforms with the Declaration of Helsinki. All patients gave written informed consent and the study was approved by the North Birmingham Ethics Committee.
Study design
This is a single-centred, uncontrolled, observational study. Patients referred for CRT underwent pacemaker implantation during an elective admission. Patients underwent a clinical assessment, including a 6 min walk test,14 (the primary endpoint) and a quality of life (QoL) assessment using Minnesota Living with Heart Failure questionnaire15 (the secondary endpoint) (S.C., P.F., and S.A.M.) on the day prior to implantation and at 1.48 years after implantation. Echocardiography and LGE-CMR studies were undertaken within a month prior to implantation. Patients were classified as responders if they satisfied all the following criteria: (1) survival for 1year following implantation; (2) no hospitalizations for HF for 1year following implantation, and (3) improvement by ≥1 NYHA classes or by ≥25% in 6 min walking distance (6MWD). In patients who died, the clinical and echocardiographic data at follow-up pertains to the latest available follow-up.
Device therapy
None of the patients had previously been paced. Transvenous biventricular pacemaker implantation was undertaken using standard techniques under local anaesthesia. Patients underwent a pacemaker check and transmitral Doppler-directed optimization of atrioventricular delay16 prior to discharge and at the scheduled visit thereafter. Generators used included the InSync III, model 8042 (Medtronic Inc), Frontier II (St Jude Medical Inc), Stratos LV (Biotronik) and Contak Renewal TR2 (Boston Scientific).
The LV lead was placed in the venous tributary preferred by the implanter, who was blinded to the CMR study. Lead placement following implantation was determined retrospectively using Mortensen's o'clock method,17 which employs bi-plane fluoroscopy. In the left anterior oblique view, the coronary sinus encircles the mitral ring with its tributaries radiating out like the hands of a clock. In the right anterior oblique view, the LV is divided into basal, mid-ventricular, and apical segments. The distance from the mitral ring to the lead tip was also taken, using the lead tip coil as the distance reference in that plane. The exact localization of the lead tip in relation to myocardial scarring was determined using the o'clock position and the mitral ring-to-lead tip distance. The implanter was blinded to the results of the CMR scan.
Echocardiography
Two-dimensional echocardiography was performed using System 5 scanner (Vingmed-General Electric). Standard left parasternal long-axis and short-axis, and apical, four-, five-, and two-chamber views were obtained. Digital images were stored for off-line analysis (EchoPAC, Vingmed-General Electric). Left ventricular volumes were estimated through Simpson's equation by planimetry of apical four-chamber views. The frame at the beginning of the QRS complex was used to estimate end-diastolic volume (LVEDV), whereas the frame with the smallest ventricular area just prior to mitral valve opening was used to estimate end-systolic volume (LVESV). LVEF was derived according to the formula (LVEDV − LVSEV/LVEDV) × 100.
Cardiovascular magnetic resonance
Images were acquired on a 1.5 Tesla scanner (Signa, General Electric Healthcare Worldwide, Slough, UK) using a phased array cardiac coil during repeated 8-second breathholds. A short-axis stack of LV images was acquired using a steady state in free precession sequence (repetition time, 3.0–3.8 ms; excitation time, 1.0 ms; image matrix, 224 × 224; field of view, 36–42 cm; flip angle, 45°) in sequential 8 mm slices (2 mm inter-slice gap) from the atrioventricular ring to apex. Left ventricular volumes, ejection fraction, and mass (myocardial density = 1.05 g/cm3) were quantified using manual planimetry of all short-axis SSFP cine images with MASS analysis software (Medis, Leiden, The Netherlands).
For the LGE-CMR study, gadolinium-diethylenetriamine pentaacetic acid (0.1mmol/kg) was administered intravenously, and images were acquired after 10 min using a segmented inversion-recovery technique in identical short-axis slices. Inversion times were adjusted to null normal myocardium (260–400 ms). Quantification of myocardial scarring was carried out by planimetry of hyperenhanced tissue on LGE-CMR images, assumed to represent scar tissue. Because of heart size and shape, the number of slices per short-axis stack varied from six to eight. Infarct volume was calculated in cubic centimetre by multiplying the planimetred area in each segment by the slice thickness. Scar volume was expressed as percentage of LV myocardial volume in the diastolic phase. Examples of LGE-CMR images are shown in Figure 1. The observer was blinded to clinical data.

Late gadolinium enhancement-cardiovascular magnetic resonance in the basal short-axis view demonstrating hyper-enhancement of scar tissue (arrow) in patients with (A) a septal infarct and (B) a posterolateral infarct. Both infarcts, which appear white in colour, exceed 51% transmurality.
Transmurality was assessed on LGE images using a 17-segment model.18 A scar was regarded as having ≥51% transmurality if its transmurality was ≥51% in any of these 17 segments.
Statistical analysis
Continuous variables are expressed as mean ± SD. Comparisons between normally distributed continuous variables were made using ANOVA. Differences between categorical variables were assessed using the χ2 test test. The ability of percentage of scar volume to predict the changes in 6MWD and QoL was assessed using logistic regression analyses. Receiver-operating characteristic (ROC) curves were used to derive optimal cut-off points for scar size. The value of percentage of scar size with the optimum sensitivity and specificity for the differentiation between responders and non-responders was 33%. Follow-up time failed to emerge as a significant predictor when it entered as a covariate in all analyses. Statistical analyses were performed using Statview (Cary, NC, USA). A two-tailed P-value of <0.05 was considered statistically significant.
Results
As shown in Table 1, 64% of the study sample responded to CRT. Responders and non-responders were well matched for age, sex, NYHA class, QRS duration (range, 120–233ms), co-morbidities, and medication. With regard to CMR variables, the non-responder group had a lower LVEF (P = 0.048), a higher scar size (P = 0.009), and a greater number of scars with ≥51% transmurality (P = 0.002). After a mean follow-up of 540 days, there was neither improvement in LVEF, nor evidence of negative LV remodelling in either responders or non-responders (Table 2).
Clinical characteristics of all patients, categorised as responders and non-responders to cardiac resynchronisation therapy
. | All . | Responders . | Non-responders . | P-valuea . |
---|---|---|---|---|
n, (%) | 45 | 29 (64) | 16 (36) | |
Age years | 67.1 ± 10.4 | 65.5 ± 10.6 | 69.9 ± 9.7 | NS |
Sex, male (%) | 38 (84) | 24 (83) | 14 (88) | NS |
NYHA class at implant, n (%) | ||||
III | 35 (78) | 23 (79) | 12 (75) | NS |
IV | 10 (22) | 6 (21) | 4 (25) | NS |
QRS duration, ms | 151.0 ± 26.8 | 152.5 ± 25.2 | 148.3 ± 30.2 | NS |
Co-morbidity | ||||
Diabetes | 9 (20) | 5 (17) | 4 (25) | NS |
Hypertension | 12 (27) | 10 (34) | 2 (13) | NS |
CABG | 17 (38) | 11 (38) | 6 (38) | NS |
Medication, n (%) | ||||
Loop diuretics | 39 (87) | 24 (83) | 15 (94) | NS |
ACE-I or ARB | 41 (91) | 27 (93) | 14 (88) | NS |
Beta-blockers | 23 (51) | 16 (55) | 7 (44) | NS |
Spironolactone | 22 (49) | 15 (52) | 7 (44) | NS |
CMR variables | ||||
LVESV (cm3) | 190.4 ± 77.4 | 174.6 ± 77.0 | 219.1 ± 71.8 | 0.065 |
LVEDV (cm3) | 234.6 ± 79.5 | 222.6 ± 78.4 | 256.2 ± 79.4 | NS |
LVEF (%) | 22.1 ± 11.1 | 24.5 ± 12.3 | 17.7 ± 7.01 | 0.048 |
Scar sizeb (%) | 31.3 ± 18.0 | 26.2 ± 16.7 | 40.5 ± 17.1 | 0.009 |
Posterolateral scar location, n (%) | 29 (64) | 17 (59) | 12 (75) | NS |
Scar transmurality ≥51%, n (%) | 26 (59) | 12 (41) | 14 (87) | 0.002 |
. | All . | Responders . | Non-responders . | P-valuea . |
---|---|---|---|---|
n, (%) | 45 | 29 (64) | 16 (36) | |
Age years | 67.1 ± 10.4 | 65.5 ± 10.6 | 69.9 ± 9.7 | NS |
Sex, male (%) | 38 (84) | 24 (83) | 14 (88) | NS |
NYHA class at implant, n (%) | ||||
III | 35 (78) | 23 (79) | 12 (75) | NS |
IV | 10 (22) | 6 (21) | 4 (25) | NS |
QRS duration, ms | 151.0 ± 26.8 | 152.5 ± 25.2 | 148.3 ± 30.2 | NS |
Co-morbidity | ||||
Diabetes | 9 (20) | 5 (17) | 4 (25) | NS |
Hypertension | 12 (27) | 10 (34) | 2 (13) | NS |
CABG | 17 (38) | 11 (38) | 6 (38) | NS |
Medication, n (%) | ||||
Loop diuretics | 39 (87) | 24 (83) | 15 (94) | NS |
ACE-I or ARB | 41 (91) | 27 (93) | 14 (88) | NS |
Beta-blockers | 23 (51) | 16 (55) | 7 (44) | NS |
Spironolactone | 22 (49) | 15 (52) | 7 (44) | NS |
CMR variables | ||||
LVESV (cm3) | 190.4 ± 77.4 | 174.6 ± 77.0 | 219.1 ± 71.8 | 0.065 |
LVEDV (cm3) | 234.6 ± 79.5 | 222.6 ± 78.4 | 256.2 ± 79.4 | NS |
LVEF (%) | 22.1 ± 11.1 | 24.5 ± 12.3 | 17.7 ± 7.01 | 0.048 |
Scar sizeb (%) | 31.3 ± 18.0 | 26.2 ± 16.7 | 40.5 ± 17.1 | 0.009 |
Posterolateral scar location, n (%) | 29 (64) | 17 (59) | 12 (75) | NS |
Scar transmurality ≥51%, n (%) | 26 (59) | 12 (41) | 14 (87) | 0.002 |
aP-values refer to differences between responders and non-responders.
bScar size is expressed as a percentage of left ventricular myocardial volume. Continuous variables are expressed as mean (SD) and categorical variables as n (%).
NYHA, New York Heart Association; LVESV, left ventricular end-systolic volume; LVEDV, left ventricular end-diastolic volume; LVEF, left ventricular ejection fraction; ACE-I, angiotensin-converting enzyme inhibitors; ARB, angiotensin receptor blockers.
Clinical characteristics of all patients, categorised as responders and non-responders to cardiac resynchronisation therapy
. | All . | Responders . | Non-responders . | P-valuea . |
---|---|---|---|---|
n, (%) | 45 | 29 (64) | 16 (36) | |
Age years | 67.1 ± 10.4 | 65.5 ± 10.6 | 69.9 ± 9.7 | NS |
Sex, male (%) | 38 (84) | 24 (83) | 14 (88) | NS |
NYHA class at implant, n (%) | ||||
III | 35 (78) | 23 (79) | 12 (75) | NS |
IV | 10 (22) | 6 (21) | 4 (25) | NS |
QRS duration, ms | 151.0 ± 26.8 | 152.5 ± 25.2 | 148.3 ± 30.2 | NS |
Co-morbidity | ||||
Diabetes | 9 (20) | 5 (17) | 4 (25) | NS |
Hypertension | 12 (27) | 10 (34) | 2 (13) | NS |
CABG | 17 (38) | 11 (38) | 6 (38) | NS |
Medication, n (%) | ||||
Loop diuretics | 39 (87) | 24 (83) | 15 (94) | NS |
ACE-I or ARB | 41 (91) | 27 (93) | 14 (88) | NS |
Beta-blockers | 23 (51) | 16 (55) | 7 (44) | NS |
Spironolactone | 22 (49) | 15 (52) | 7 (44) | NS |
CMR variables | ||||
LVESV (cm3) | 190.4 ± 77.4 | 174.6 ± 77.0 | 219.1 ± 71.8 | 0.065 |
LVEDV (cm3) | 234.6 ± 79.5 | 222.6 ± 78.4 | 256.2 ± 79.4 | NS |
LVEF (%) | 22.1 ± 11.1 | 24.5 ± 12.3 | 17.7 ± 7.01 | 0.048 |
Scar sizeb (%) | 31.3 ± 18.0 | 26.2 ± 16.7 | 40.5 ± 17.1 | 0.009 |
Posterolateral scar location, n (%) | 29 (64) | 17 (59) | 12 (75) | NS |
Scar transmurality ≥51%, n (%) | 26 (59) | 12 (41) | 14 (87) | 0.002 |
. | All . | Responders . | Non-responders . | P-valuea . |
---|---|---|---|---|
n, (%) | 45 | 29 (64) | 16 (36) | |
Age years | 67.1 ± 10.4 | 65.5 ± 10.6 | 69.9 ± 9.7 | NS |
Sex, male (%) | 38 (84) | 24 (83) | 14 (88) | NS |
NYHA class at implant, n (%) | ||||
III | 35 (78) | 23 (79) | 12 (75) | NS |
IV | 10 (22) | 6 (21) | 4 (25) | NS |
QRS duration, ms | 151.0 ± 26.8 | 152.5 ± 25.2 | 148.3 ± 30.2 | NS |
Co-morbidity | ||||
Diabetes | 9 (20) | 5 (17) | 4 (25) | NS |
Hypertension | 12 (27) | 10 (34) | 2 (13) | NS |
CABG | 17 (38) | 11 (38) | 6 (38) | NS |
Medication, n (%) | ||||
Loop diuretics | 39 (87) | 24 (83) | 15 (94) | NS |
ACE-I or ARB | 41 (91) | 27 (93) | 14 (88) | NS |
Beta-blockers | 23 (51) | 16 (55) | 7 (44) | NS |
Spironolactone | 22 (49) | 15 (52) | 7 (44) | NS |
CMR variables | ||||
LVESV (cm3) | 190.4 ± 77.4 | 174.6 ± 77.0 | 219.1 ± 71.8 | 0.065 |
LVEDV (cm3) | 234.6 ± 79.5 | 222.6 ± 78.4 | 256.2 ± 79.4 | NS |
LVEF (%) | 22.1 ± 11.1 | 24.5 ± 12.3 | 17.7 ± 7.01 | 0.048 |
Scar sizeb (%) | 31.3 ± 18.0 | 26.2 ± 16.7 | 40.5 ± 17.1 | 0.009 |
Posterolateral scar location, n (%) | 29 (64) | 17 (59) | 12 (75) | NS |
Scar transmurality ≥51%, n (%) | 26 (59) | 12 (41) | 14 (87) | 0.002 |
aP-values refer to differences between responders and non-responders.
bScar size is expressed as a percentage of left ventricular myocardial volume. Continuous variables are expressed as mean (SD) and categorical variables as n (%).
NYHA, New York Heart Association; LVESV, left ventricular end-systolic volume; LVEDV, left ventricular end-diastolic volume; LVEF, left ventricular ejection fraction; ACE-I, angiotensin-converting enzyme inhibitors; ARB, angiotensin receptor blockers.
Clinical and echocardiographic characteristics of responders and non-responders to cardiac resynchronisation therapy at baseline and follow-up
. | Responders . | Non-responders . | P-value . |
---|---|---|---|
n | 29 (64) | 16 (36) | |
NYHA class, I/II/III/IV | |||
Baseline | 0/0/23/6 | 0/0/12/4 | NS |
Follow-up | 11/17/1/0 *** | 1/4/10/1*** | <0.001 |
6 Min walk test (m) | |||
Baseline | 306.0 ± 95.0 | 218.7 ± 94.4 | 0.005 |
Follow-up | 389.2 ± 97.5 *** | 244.4 ± 103.2 | <0.001 |
Quality of life score | |||
Baseline | 49.5 ± 15.5 | 52.3 ± 25.4 | NS |
Follow-up | 24.8 ± 17.2 *** | 45.6 ± 29.5 | 0.004 |
LVEF (%) | |||
Baseline | 28.7 ± 10.0 | 20.0 ± 8.5 | 0.009 |
Follow-up | 28.4 ± 12.6 | 28.7 ± 9.0 | NS |
LVEDV (mL) | |||
Baseline | 203.7 ± 66.9 | 237.3 ± 53.6 | NS |
Follow-up | 191.2 ± 59.4 | 237.5 ± 72.9 | 0.029 |
LVESV (mL) | |||
Baseline | 148.9 ± 63.4 | 193.5 ± 54.0 | 0.022 |
Follow-up | 143.8 ± 60.1 | 176.4 ± 72.8 | NS |
. | Responders . | Non-responders . | P-value . |
---|---|---|---|
n | 29 (64) | 16 (36) | |
NYHA class, I/II/III/IV | |||
Baseline | 0/0/23/6 | 0/0/12/4 | NS |
Follow-up | 11/17/1/0 *** | 1/4/10/1*** | <0.001 |
6 Min walk test (m) | |||
Baseline | 306.0 ± 95.0 | 218.7 ± 94.4 | 0.005 |
Follow-up | 389.2 ± 97.5 *** | 244.4 ± 103.2 | <0.001 |
Quality of life score | |||
Baseline | 49.5 ± 15.5 | 52.3 ± 25.4 | NS |
Follow-up | 24.8 ± 17.2 *** | 45.6 ± 29.5 | 0.004 |
LVEF (%) | |||
Baseline | 28.7 ± 10.0 | 20.0 ± 8.5 | 0.009 |
Follow-up | 28.4 ± 12.6 | 28.7 ± 9.0 | NS |
LVEDV (mL) | |||
Baseline | 203.7 ± 66.9 | 237.3 ± 53.6 | NS |
Follow-up | 191.2 ± 59.4 | 237.5 ± 72.9 | 0.029 |
LVESV (mL) | |||
Baseline | 148.9 ± 63.4 | 193.5 ± 54.0 | 0.022 |
Follow-up | 143.8 ± 60.1 | 176.4 ± 72.8 | NS |
LVESV, left ventricular end-systolic volume; LVEDV, left ventricular end-diastolic volume; LVEF, left ventricular ejection fraction. Asterisks refer to P-values of differences from baseline values within the group. *P < 0.01, ** P < 0.001, ***P < 0.0001. P-values in the column refer to comparisons between the responder and non-responder groups.
Clinical and echocardiographic characteristics of responders and non-responders to cardiac resynchronisation therapy at baseline and follow-up
. | Responders . | Non-responders . | P-value . |
---|---|---|---|
n | 29 (64) | 16 (36) | |
NYHA class, I/II/III/IV | |||
Baseline | 0/0/23/6 | 0/0/12/4 | NS |
Follow-up | 11/17/1/0 *** | 1/4/10/1*** | <0.001 |
6 Min walk test (m) | |||
Baseline | 306.0 ± 95.0 | 218.7 ± 94.4 | 0.005 |
Follow-up | 389.2 ± 97.5 *** | 244.4 ± 103.2 | <0.001 |
Quality of life score | |||
Baseline | 49.5 ± 15.5 | 52.3 ± 25.4 | NS |
Follow-up | 24.8 ± 17.2 *** | 45.6 ± 29.5 | 0.004 |
LVEF (%) | |||
Baseline | 28.7 ± 10.0 | 20.0 ± 8.5 | 0.009 |
Follow-up | 28.4 ± 12.6 | 28.7 ± 9.0 | NS |
LVEDV (mL) | |||
Baseline | 203.7 ± 66.9 | 237.3 ± 53.6 | NS |
Follow-up | 191.2 ± 59.4 | 237.5 ± 72.9 | 0.029 |
LVESV (mL) | |||
Baseline | 148.9 ± 63.4 | 193.5 ± 54.0 | 0.022 |
Follow-up | 143.8 ± 60.1 | 176.4 ± 72.8 | NS |
. | Responders . | Non-responders . | P-value . |
---|---|---|---|
n | 29 (64) | 16 (36) | |
NYHA class, I/II/III/IV | |||
Baseline | 0/0/23/6 | 0/0/12/4 | NS |
Follow-up | 11/17/1/0 *** | 1/4/10/1*** | <0.001 |
6 Min walk test (m) | |||
Baseline | 306.0 ± 95.0 | 218.7 ± 94.4 | 0.005 |
Follow-up | 389.2 ± 97.5 *** | 244.4 ± 103.2 | <0.001 |
Quality of life score | |||
Baseline | 49.5 ± 15.5 | 52.3 ± 25.4 | NS |
Follow-up | 24.8 ± 17.2 *** | 45.6 ± 29.5 | 0.004 |
LVEF (%) | |||
Baseline | 28.7 ± 10.0 | 20.0 ± 8.5 | 0.009 |
Follow-up | 28.4 ± 12.6 | 28.7 ± 9.0 | NS |
LVEDV (mL) | |||
Baseline | 203.7 ± 66.9 | 237.3 ± 53.6 | NS |
Follow-up | 191.2 ± 59.4 | 237.5 ± 72.9 | 0.029 |
LVESV (mL) | |||
Baseline | 148.9 ± 63.4 | 193.5 ± 54.0 | 0.022 |
Follow-up | 143.8 ± 60.1 | 176.4 ± 72.8 | NS |
LVESV, left ventricular end-systolic volume; LVEDV, left ventricular end-diastolic volume; LVEF, left ventricular ejection fraction. Asterisks refer to P-values of differences from baseline values within the group. *P < 0.01, ** P < 0.001, ***P < 0.0001. P-values in the column refer to comparisons between the responder and non-responder groups.
Effect of scar size
As shown in Figure 2, percentage of scar correlated negatively with change in 6MWD (r = −0.54, P < 0.0001) and positively with changes in QoL scores (r = 0.35, P = 0.028). At a cut-off of <33%, scar size predicted a response to CRT, with a sensitivity of 79% and a specificity of 69% [area under ROC curve (AUC) = 0.74, P = 0.016)] (Figure 3). A scar size cut-off of 15% predicted a response to CRT with a sensitivity of 27% and a specificity of 87%, whereas a cut-off of 40% predicted a response to CRT with a sensitivity of 83%, but a specificity of 50%. As shown in Table 3, the responder rate in patients with <33% scar was 2.3 times greater than that in patients with ≥33% scar (P < 0.01). Increasing scar size to ≥33% in scars with ≥51% transmurality or in scars of any location was associated with a poorer response to CRT.
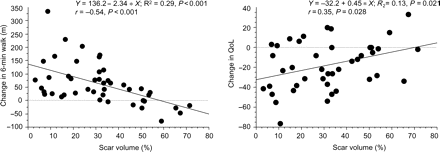
Scattergrams of changes in 6 min walking distance and quality of life scores in relation to percentage of scar. A negative change in quality of life represents an improvement in quality of life and a positive change represents deterioration. The graph therefore indicates that the greatest improvement in quality of life occurs in patients with least scar.
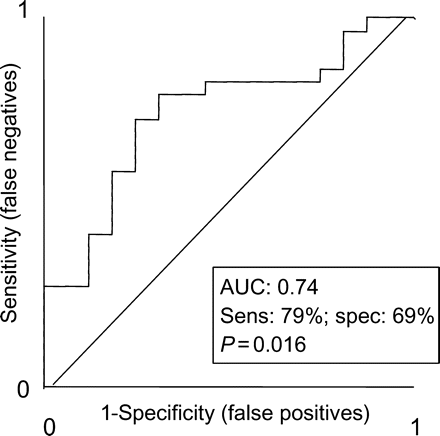
Receiver operator characteristic curves for ability of percentage of scar to predict a positive response to cardiac resynchronization therapy. AUC, area under curve; sens, sensitivity; spec, specificity; NYHA, New York Heart Association; 6MWD, 6 min walking distance.
. | Size . | Transmurality . | Location . | |||
---|---|---|---|---|---|---|
. | <33% . | ≥33% . | <51% . | ≥51% . | Posterolateral . | Non-posterolateral . |
23/28 (82) | 6/17 (35)** | 17/19 (89) | 12/26 (46)** | 17/29 (59) | 12/16 (75) | |
Size, (%) | ||||||
<33 | – | – | 13/14 (93) | 10/14 (71) | 12/16 (75) | 11/12 (92) |
≥33 | – | – | 4/5 (80) | 2/12 (17)* | 5/13 (38) | 1/4 (25) |
Transmurality | ||||||
<51% | 13/14 (93) | 4/5 (80) | – | – | 14/16 (88) | 3/3 (100) |
≥51% | 10/14 (71) | 2/12 (17)** | – | – | 3/13 (23) | 9/13 (69)* |
Location | ||||||
Posterolateral | 12/16 (75) | 5/13 (38)* | 14/16 (88) | 3/13 (23)*** | – | – |
Non-posterolateral | 11/12 (92) | 1/4 (25)** | 3/3 (100) | 9/13 (69) | – | – |
. | Size . | Transmurality . | Location . | |||
---|---|---|---|---|---|---|
. | <33% . | ≥33% . | <51% . | ≥51% . | Posterolateral . | Non-posterolateral . |
23/28 (82) | 6/17 (35)** | 17/19 (89) | 12/26 (46)** | 17/29 (59) | 12/16 (75) | |
Size, (%) | ||||||
<33 | – | – | 13/14 (93) | 10/14 (71) | 12/16 (75) | 11/12 (92) |
≥33 | – | – | 4/5 (80) | 2/12 (17)* | 5/13 (38) | 1/4 (25) |
Transmurality | ||||||
<51% | 13/14 (93) | 4/5 (80) | – | – | 14/16 (88) | 3/3 (100) |
≥51% | 10/14 (71) | 2/12 (17)** | – | – | 3/13 (23) | 9/13 (69)* |
Location | ||||||
Posterolateral | 12/16 (75) | 5/13 (38)* | 14/16 (88) | 3/13 (23)*** | – | – |
Non-posterolateral | 11/12 (92) | 1/4 (25)** | 3/3 (100) | 9/13 (69) | – | – |
*P < 0.05, **P < 0.01, ***P < 0.001.
. | Size . | Transmurality . | Location . | |||
---|---|---|---|---|---|---|
. | <33% . | ≥33% . | <51% . | ≥51% . | Posterolateral . | Non-posterolateral . |
23/28 (82) | 6/17 (35)** | 17/19 (89) | 12/26 (46)** | 17/29 (59) | 12/16 (75) | |
Size, (%) | ||||||
<33 | – | – | 13/14 (93) | 10/14 (71) | 12/16 (75) | 11/12 (92) |
≥33 | – | – | 4/5 (80) | 2/12 (17)* | 5/13 (38) | 1/4 (25) |
Transmurality | ||||||
<51% | 13/14 (93) | 4/5 (80) | – | – | 14/16 (88) | 3/3 (100) |
≥51% | 10/14 (71) | 2/12 (17)** | – | – | 3/13 (23) | 9/13 (69)* |
Location | ||||||
Posterolateral | 12/16 (75) | 5/13 (38)* | 14/16 (88) | 3/13 (23)*** | – | – |
Non-posterolateral | 11/12 (92) | 1/4 (25)** | 3/3 (100) | 9/13 (69) | – | – |
. | Size . | Transmurality . | Location . | |||
---|---|---|---|---|---|---|
. | <33% . | ≥33% . | <51% . | ≥51% . | Posterolateral . | Non-posterolateral . |
23/28 (82) | 6/17 (35)** | 17/19 (89) | 12/26 (46)** | 17/29 (59) | 12/16 (75) | |
Size, (%) | ||||||
<33 | – | – | 13/14 (93) | 10/14 (71) | 12/16 (75) | 11/12 (92) |
≥33 | – | – | 4/5 (80) | 2/12 (17)* | 5/13 (38) | 1/4 (25) |
Transmurality | ||||||
<51% | 13/14 (93) | 4/5 (80) | – | – | 14/16 (88) | 3/3 (100) |
≥51% | 10/14 (71) | 2/12 (17)** | – | – | 3/13 (23) | 9/13 (69)* |
Location | ||||||
Posterolateral | 12/16 (75) | 5/13 (38)* | 14/16 (88) | 3/13 (23)*** | – | – |
Non-posterolateral | 11/12 (92) | 1/4 (25)** | 3/3 (100) | 9/13 (69) | – | – |
*P < 0.05, **P < 0.01, ***P < 0.001.
Effect of scar transmurality
As shown in Table 3, the responder rate in scars with <51% transmurality was 1.9 times the responder rate in the group with ≥51% transmurality. Increasing transmurality to ≥51% in scars ≥33% in size was associated with a poorer response to CRT (P < 0.05). In patients with posterolateral scars, a transmurality of ≥51% was associated with a poor response to CRT (P < 0.001). A tendency towards a poor response with increasing transmurality was observed in patients with non-posterolateral scars, but this did not reach the statistical significance.
Effect of scar location
As shown in Table 3, the responder rate is higher in patients with posterolateral scars compared with patients with non-posterolateral scars, but this difference did not reach statistical significance. Among scars with ≥51% transmurality, however, a posterolateral location was associated with a significantly worse responder rate than scars with <51% transmurality (23 vs. 69%, P < 0.05).
Scar size, transmurality, and location combined
The relative contribution of scar size, transmurality, and location to response was explored using logistic regression. As shown in Table 4, both scar size (P = 0.003) and transmurality (P = 0.007) emerged as significant predictors of response. In multivariate analyses, scar size (P = 0.022) and transmurality (P = 0.004) also emerged as predictors of response, whereas scar location failed to reach the statistical significance.
Univariate and multivariate logistic regression analyses of predictors of response to cardiac resynchronisation therapy*
. | Univariate . | Multivariate . | ||
---|---|---|---|---|
. | χ2 . | P-value . | χ2 . | P-value . |
Scar<33% | 9.1 | 0.003 | 5.21 | 0.022 |
Scar location (non-posterolateral) | 1.18 | NS | 3.55 | 0.059 |
Transmurality (<51%) | 7.4 | 0.007 | 8.43 | 0.004 |
. | Univariate . | Multivariate . | ||
---|---|---|---|---|
. | χ2 . | P-value . | χ2 . | P-value . |
Scar<33% | 9.1 | 0.003 | 5.21 | 0.022 |
Scar location (non-posterolateral) | 1.18 | NS | 3.55 | 0.059 |
Transmurality (<51%) | 7.4 | 0.007 | 8.43 | 0.004 |
*response is defined as an improvement in ≥1 NYHA classes or ≥25% in 6-min walking distance.
Univariate and multivariate logistic regression analyses of predictors of response to cardiac resynchronisation therapy*
. | Univariate . | Multivariate . | ||
---|---|---|---|---|
. | χ2 . | P-value . | χ2 . | P-value . |
Scar<33% | 9.1 | 0.003 | 5.21 | 0.022 |
Scar location (non-posterolateral) | 1.18 | NS | 3.55 | 0.059 |
Transmurality (<51%) | 7.4 | 0.007 | 8.43 | 0.004 |
. | Univariate . | Multivariate . | ||
---|---|---|---|---|
. | χ2 . | P-value . | χ2 . | P-value . |
Scar<33% | 9.1 | 0.003 | 5.21 | 0.022 |
Scar location (non-posterolateral) | 1.18 | NS | 3.55 | 0.059 |
Transmurality (<51%) | 7.4 | 0.007 | 8.43 | 0.004 |
*response is defined as an improvement in ≥1 NYHA classes or ≥25% in 6-min walking distance.
Effect of pacing over the scar
Of the 29 patients with a posterolateral scar, 14 underwent CRT with the LV lead deployed outside the scar and 15 with the lead over the scar. In patients with the LV lead deployed outside the scar, 6WMD increased from 300.0 ± 107.2 to 370.4 ± 110.1 m (P = 0.011) and QoL scores improved from 46.2 ± 20.3 to 19.2 ± 15.4 (P < 0.001). In contrast, neither 6MWD or QoL improved in patients receiving CRT with the LV lead deployed over the scar (Figure 4). Pacing outside the scar was associated with a better responder rate than pacing over the scar [12/14 (86%) vs. 5/15 (33%), P = 0.004].
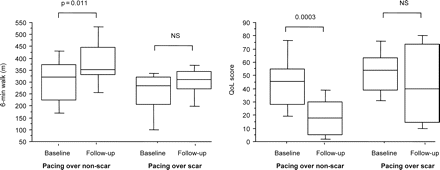
Box and whisker plots of changes in 6 min walking distance and quality of life in patients with posterolateral scars, grouped according to pacing site in relation to scar. The five horizontal lines represent the 10th, 25th, 50th, 75th, and 90th percentiles of each variable, from bottom to top.
Discussion
In this study, we have explored the clinical response to CRT in relation to the extent of myocardial scarring, assessed using LGE-CMR. We have shown that, in patients with ischaemic cardiomyopathy and conventional indications for CRT, improvements in exercise capacity and QoL following CRT are inversely related to the extent of myocardial scarring. A scar transmurality of ≥51% and pacing over the scar were also associated with a suboptimal response to CRT.
The clinical response to treatments such as revascularization in patients with LV dysfunction is critically dependent on the presence of viable myocardium.19–22 Similar findings have been shown in relation to beta-blockade in patients with HF.23 Although CRT improves cardiac performance by different mechanisms to revascularization, the relationship between myocardial scarring and subsequent clinical improvement still holds. In revascularization studies, clinical benefit decreases markedly when the area of viable myocardium reaches a critical size.24,25 In the present study, a scar volume of 33% appears to be the best cut-off for the prediction of favourable response to CRT. White et al.26 have identified a lower cut off of 15% for scar size as the best predictor of response. In that study, however, only 12 of 23 patients studied had a history of myocardial infarction and 5 of 23 had no scar at all. The inclusion in our study of patients only with a history of myocardial infarction and with myocardial scar on LGE-CMR is perhaps responsible for the higher threshold for percentage of scar size identified. Notwithstanding, White et al.26 found that septal scars of ≤40% provided a 100% sensitivity and specificity for a response to CRT. Taken together, both studies support the hypothesis that there is a limit of scar size beyond which resynchronization becomes ineffective.
We have also found an association between increasing transmurality and poor response to CRT. Across the study group, the response to CRT in patients with a transmurality of ≥51% was half the response observed in patients with a transmurality of <51%. In posterolateral scars, a transmurality of ≥51% was associated with almost a four-fold reduction in response. These findings are consistent with revascularization studies showing that an increase in the transmurality value is associated with a suboptimal response to revascularization, i.e. with reduced myocardial viability.21
With regard to scar location, a trend towards a lower response in patients with a posterolateral scar. Statistical significance for this difference, however, was only reached after taking into account transmurality. Accordingly, the symptomatic responder rate in patients with a posterolateral scar of transmurality value of ≥51% was 23%. These findings are consistent with those of Bleeker et al.11, who demonstrated that in patients with a transmural posterolateral scar, CRT does not lead to improvements in 6MWD or QoL. A further finding from this study is that pacing over the scar was associated with a lack of response to CRT, both in terms of 6MWD and QoL. Several factors might contribute to this. First, scar tissue does not contract. Secondly, pacing over a scar is likely to lead to suboptimal resynchronization of the neighbouring myocardium, as electrical conduction is slower over scar tissue.27,28
The observed mortality rate of 33% over 1.48 years is admittedly high, as is the absence of reverse remodelling. This may be because of the inclusion of patients with, exclusively, ischaemic cardiomyopathy. This is in contrast to the CARE-HF study, in which 40% of patients in the paced group had ischaemic cardiomyopathy. Moreover, our study involves a greater proportion of patients in NYHA class IV (22%) than the CRT arms of the Multicenter InSync Randomized Clinical Evaluation (MIRACLE) (9.9%)2 and the Multisite Stimulation in Cardiomyopathies (MUSTIC) (0%)3 studies. Patients in this study also had lower 6MWD (275 m) than in the MIRACLE (307.2 m) and the MUSTIC (399 m) studies.
Limitations of the study
Our measure of myocardial scarring does not take into account the effects of myocardial thinning. If thinning has occurred, the planimetred area of myocardial scar is likely to be an underestimation of the original scar size. Our finding of 33% as a cut-off for clinical response following CRT is the result of a retrospective analysis. Clearly, this threshold would be different if patients were not selected on the basis of QRS duration. These finding therefore requires confirmation in a validation series.
Conclusions
We conclude that the extent of myocardial scarring, assessed using LGE-CMR, is a strong negative predictor of clinical benefit following CRT in patients with ischemic cardiomyopathy. Scar transmurality and a posterolateral scar location were also found to influence the response to CRT negatively. In patients with posterolateral scars, pacing over the scar was associated with a suboptimal response. These findings illustrate how LGE-CMR undertaken prior to implantation can predict the likely benefit of CRT in individual patients. Moreover, this imaging modality also provides useful information for guiding LV lead deployment.
Acknowledgements
We are grateful to Lisa Ball, Janet Brashaw-Smith, and Nick Irwin for their invaluable contribution to the follow-up of patients included in this study. We are also grateful to Medtronic Inc. for their continued support.
Conflict of interest: S.C. and S.A.M. held research fellowships sponsored by Medtronic Inc. F.L. has also received sponsorship from St Jude Medical Inc.