-
PDF
- Split View
-
Views
-
Cite
Cite
Caijie Shen, Zhenyu Jia, Yibo Yu, Mingjun Feng, Xianfeng Du, Guohua Fu, Lipu Yu, Tao Wu, Yongxing Jiang, He Jin, Weidong Zhuo, Fang Gao, Binhao Wang, Si Chen, Jiating Dai, Renyuan Fang, Huimin Chu, Efficacy and safety of pulsed field ablation for accessory pathways: a pilot study, EP Europace, Volume 26, Issue 7, July 2024, euae139, https://doi.org/10.1093/europace/euae139
- Share Icon Share
Abstract
Radiofrequency ablation is used as a first-line therapy for accessory pathways (APs). However, data regarding the effects of pulsed field ablation (PFA) on APs are limited. We sought to evaluate the acute procedural and 6-month success and safety of PFA in a cohort of patients with APs.
A focal contact force–sensing PFA catheter was used for patients with APs. Pulsed field ablation generator generated a bipolar and biphasic waveform (±1000 V) with a duration of 100 ms from the tip of the PFA catheter. A 100% acute procedural success was achieved in 10 conscious patients with APs (7 left anterolateral, 2 left inferolateral, and 1 right posteroseptal APs) including 6 (60%) patients after an initial application. The average total ablation time was 6.3 ± 4.9 s for 4.7 ± 1.8 ablation sites (ASs), including 3.1 ± 2.4 s at targets and 3.2 ± 2.9 s at 3.2 ± 2 bolus ASs. The mean skin-to-skin time was 59.3 ± 15.5 min, and PFA catheter dwell time was 29.4 ± 7.8 min. One patient encountered transient sinus arrest during PFA due to parasympathetic overexcitation. Sinus rhythm was restored in all patients without any significant adverse events during the short-term follow-up.
Pulsed field ablation of APs was feasible, effective, and safe. Its efficiency was remarkable for its ultrarapid termination of AP conduction. Further studies are warranted to prove whether utilization of PFA with current parameters can extend to manifold AP ablation.
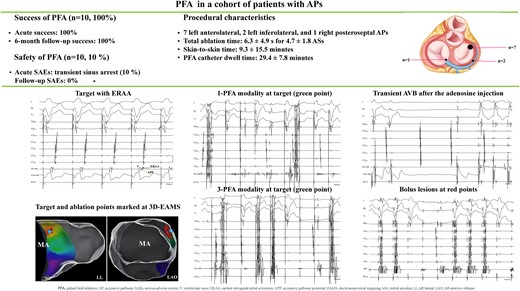
This study represents the largest sample of patients with accessory pathways (APs) to date receiving pulsed field ablation (PFA).
A 100% acute procedural and 6-month success was achieved, including seven left anterolateral APs, two left inferolateral Aps, and one right posteroseptal AP. One patient encountered transient sinus arrest due to pain-induced vagal overreaction during PFA.
No other serious adverse events were detected during the procedure and 6-month follow-up.
Introduction
Accessory pathways (APs), as strands of myocardial cells that bypass the physiological atrioventricular (AV) conduction system, are the anatomic substrates of atrioventricular reentrant tachycardia (AVRT).1 Radiofrequency ablation (RFA) is highly safe and effective for patients with symptomatic APs causing AVRT or in situations where APs pose a risk of sudden cardiac death.2 Radiofrequency ablation–induced lesion for AP termination is associated with several critical variables, generally including power, duration of application, catheter–tissue contact force (CF), and stability.3,4 For instance, catheter stability and moderate CF at the superior tricuspid annulus (TA) or lateral mitral annulus (MA) might be difficult to achieve.5 Consequently, more power should be applied to generate excessive tissue heating and lesion growth by thermal conduction.5 Besides being time consuming, RFA with high power can be associated with major complications, including AV conduction disturbances, coronary artery injuries, and cardiac perforation followed by steam pops.6,7 Therefore, the optimal strategy is still required to balance the effectiveness and safety of ablation for APs.
Pulsed field ablation (PFA) addresses some shortcomings of RFA for cardiac arrhythmias. PFA is a non-thermal ablation technique that causes ultrarapid apoptosis of targeted myocardial cells through irreversible electroporation (IRE).8 Also, PFA is attractive for its less dependence on electrode–tissue CF to create transmural and contiguous lesions compared with RFA.9 Prior studies have shown favourable safety and effectiveness when PFA is utilized for atrial fibrillation.10,11 Recently, two single-arm clinical studies demonstrated that PFA also showed favourable feasibility and safety in patients with atrioventricular nodal reentrant tachycardia (AVNRT).12,13 In our first-in-human (FIM) study, we reported that three patients with APs [two at left posterior septum (LSP) and one at right posterior septum (RSP)] received successful and safe PFA.12 Given the chief benefits of IRE, we hypothesize that PFA should be applicable to patients with APs. However, the effects of PFA on various APs have not been validated. Therefore, we performed this pilot study aiming to assess the acute procedural and short-term safety and efficacy of PFA for patients with APs with a focal CF-sensing catheter.
Methods
Trial design
A prospective, single-arm, multicentre, observational trial ‘A Multicenter Study of Pulsed Field Ablation for Paroxysmal Supraventricular Tachycardia’ (NCT05820035) has been launched. As an interim analysis of data, this pilot study was carried out in our centre aiming to evaluate the safety and effectiveness of PFA for APS which is instructive to implement the ongoing multicentre trial. The study protocol was approved by the ethics committee of the First Affiliated Hospital of Ningbo University and the corresponding regulatory board (approval code: 2023-1020-02).
Study population
From March 2023 to May 2023, 40 consecutive patients with paroxysmal supraventricular tachycardia (PSVT) or ventricular pre-excitation admitted to our centre for PFA were preliminarily enrolled with a range of ages from 18 to 80 years. Paroxysmal supraventricular tachycardia was recorded by electrocardiogram (ECG) or oesophageal electrophysiologic study (EES). We finally enrolled the patients with symptomatic AVRT or the presence of an asymptomatic overt AP who have high-risk occupations or hobbies in this study. A diagnosis and reproducibility of AVRT or an overt AP was confirmed by an electrophysiological study (EPS) after all antiarrhythmic medications were discontinued for ≥5 drug half-lives.1 Supplementary material online, Table S1, contains the full details of exclusion criteria. All the patients agreed to provide informed consent.
Pulsed field ablation system
The PFA system has been previously described.12 The key components of the PFA system include an 8-F CF-sensing focal PFA catheter (PulseMagic™ TrueForce™, Shanghai MicroPort EP MedTech Co., Ltd., China) and a PFA generator (SYE-PFA-1A, Shanghai Shangyang MedTech Co., Ltd., China). The PFA generator output was set to 2000 V (±1000 V). Square waveforms were delivered as a train of biphasic pulses with a duration of 100 ms. The pulsed field energy (PFE) delivery was synchronized to the absolute ventricular non-response monitored by a cardiac R-wave monitor (Model 7200-3, AccuSync, Inc., USA).
Procedural workflow
We continued to perform PFA with local anaesthesia as in our FIM study, and electrophysiology protocol was followed. Via femoral veins, two or three quadripolar catheters were advanced to the Hisian bundle (HB), right ventricular apex (RVA) and/or high right atrial atrium, and a decapolar catheter was fed into the coronary sinus (CS) under fluoroscopy navigation approach. Then, elicitation of clinical tachycardia was attempted in all subjects using standard programmed electrical stimulation by a cardiac electrophysiological stimulator (EP WorkMate, Abbott Co., USA) with or without isoproterenol. The diagnosis of an AP-mediated AVRT and the conduction property of an AP were clarified by established criteria.
A R0 Swartz long sheath (Abbott Co., USA) was used to improve the stability of PFA catheter manipulation. A saline infusion pump was used to keep an irrigation rate of 2 mL/min during the procedure (EPE-IGP-1A, Shanghai MicroPort EP MedTech Co., Ltd., China). The image of TA or MA, HB, and CS was labelled when the PFA catheter moved along the cavity surface guided by a three-dimensional electroanatomical mapping system (3D-EAMS, Columbus™ 3D EP Navigation system, Shanghai MicroPort EP MedTech Co., Ltd., China). A transseptal puncture was conducted if an AP was located on the left atrium, and a retrograde approach was used as an alternative. The initial delivery of PFA with one pulse train delivery (1-PFA modality) was intentionally targeted to the site with the earliest retrograde atrial activation (ERAA) and/or the earliest antegrade ventricle activation (EAVA). When loss of ERAA and/or EAVA was detected, a second application with three pulse train deliveries (3-PFA modality) was followed at the same site. If the AP was not interpreted after both 1-PFA and 3-PFA modalities, careful activation mapping was reconducted to reach the desired origin of AP. The endpoints of the procedure were termination of loss of antegrade and/or retrograde AP conduction and non-inducibility of AVRT.1,2 Bonus applications were performed in 3-PFA modality adjacent to the target. The voltage of ERAA pre- and post-PFA was recorded. Contact force was controlled from 5 to 10 g.
Before discharge
A physical exam, 12-lead surface ECG, and routine laboratory testing including N-terminal pro-brain type natriuretic (NT-proBNP), serum creatinine (SCr), alanine transaminase (ALT), and haemoglobin were routinely performed upon hospital discharge. All patients were discharged within 24 h post-procedure if no serious adverse events (SAEs) occurred. Serious adverse events are described in Supplementary material online, Table S2. All patients would take aspirin (100 mg daily) or clopidogrel (75 mg daily) for 30 days.
Follow-up
Patients were followed for 6 months with outpatient visits at 1, 3, and 6 months after discharge. For patients with AP in the left atrium, a cerebral magnetic resonance imaging scan was scheduled within the 14-day follow-up. All patients received a 12-lead surface ECG and 24 h Holter monitoring at each follow-up visit. Any potentially indicative symptoms of recurrence were promptly evaluated using a 12-lead surface ECG and/or 24 h Holter monitoring. If no evidence of recurrence was found through these methods, an electrophysiology study (EES) was conducted. Transthoracic echocardiography (TTE) and blood testing were included in the assessment in the first month of follow-up. The EES was planned at the end of follow-up.
Study outcomes
The efficacy objective was to evaluate the acute procedural and 6-month (short-term) success rate after PFA. The safety objective was to evaluate the incidences of SAEs during the procedure and within 6-month follow-up.
Statistical analysis
Continuous data are presented as mean with standard deviation or median with interquartile range. Categorical variables are expressed as numbers with percentages. For comparison, the paired t-test was used for continuous variables Statistical analyses were performed using the software SPSS Statistics 27.0 (IBM Corp Inc., USA). A P value of <0.05 was considered significant.
Results
Patient characteristics
The detailed baseline characteristics of patients are given in Supplementary material online, Table S3. A total of 10 patients with APs were enrolled. There were five female and five male patients with an average age of 40 ± 22.5 years. Nine patients with AVRT and one overt AP were diagnosed by 12-lead surface ECG and 24-h Holter monitoring, and eight patients were also confirmed by EES before the procedure. The mean duration of AVNRT was 36.8 ± 41.2 months, and the ventricular rate was 169.2 ± 9.4 beats/min.
Mapping and procedural characteristics
Detailed mapping and procedural characteristics are shown in Table 1. In two patients, slight skeletal muscle contraction occurred during PFA. Intravenous paralytic or conscious sedation was not performed, and no apparent electroanatomical map shift was recorded. Orthodromic tachycardia was induced in all patients. Electrophysiological study revealed a single AP in each patient, including one AP with bidirectional conduction and nine APs with retrograde conduction. Nine left free wall APs [seven APs at left anterolateral wall (LALW, Figure 1), two APs at left posterolateral wall (LPLW, Figure 2)] and one AP at RSP (Figure 3) were precisely identified. All APs were successfully eliminated by PFA including nine left free wall with transseptal approach, and six APs were abolished after an initial application. The average total ablation time was 6.3 ± 4.9 s at 4.7 ± 1.8 ablation sites (ASs). The mean ablation time at target was 3.1 ± 2.4 s and was 3.2 ± 2.9 s at 3.2 ± 2 bonus ASs. Pulsed field ablation at targets resulted in a significant decrease of atrial bipolar voltage after PFA at the targets with ERAA (0.3 ± 0.16 mV vs. 0.11 ± 0.06 mV, P = 0.001). Of note, #1 patient encountered sinus arrest due to pain-induced vagal reaction. Ventricle pacing was supported, and sinus arrest was relieved in 25 s after the abolishment of ablation. Coronary angiography excluded coronary artery injuries (Figure 2). Sinus arrest was no longer recorded after the administration of intravenous fentanyl during the PFA afterward.

Successful ablation of AP at LALW. (A) AVRT was induced after RVA pacing. From top to bottom, the following tracings are displayed: surface ECG, CS electrograms from proximal (CS9–10) to distal (CS1–2), distal (HB1–2), and proximal (HB3–4) His bundle electrograms, distal (Bi-Abl) bipolar and unipolar (Uni-Abl) recording from the PFA catheter, and RVA electrograms. Earliest retrograde atrial activation with bipolar recording was at LALW during RVA pacing navigated by 3D electroanatomical mapping (B, green point) and fluoroscopy (C), and the morphology of unipolar electrograms of ERAA was QS. Note the distinct APP inserting (orange arrow) between ERAA (black arrow) and retrograde ventricular activation (V). Successful ablation was achieved after one pulse train delivery (D, 1-PFA modality), and three pulse train deliveries (E, 3-PFA modality) at the same AS were applied. (F) Transient AV conduction block for a short period after the adenosine injection, then left ventricular pacing confirmed terminating conduction over AP. Bonus lesions (red points) were created near the target with 3-PFA modality. Sustained AP conduction termination was observed by RVA pacing. Abl, ablation catheter; AP, accessory pathway; APP, accessory pathway potential; AS, ablation site; AVRT, atrioventricular reentrant tachycardia; CS, coronary sinus; ERAA, earliest retrograde atrial activation; LALW, left anterolateral wall; LAO, left anterior oblique; LL, left lateral; MA, mitral annulus; PFA, pulsed field ablation; RAO, right anterior oblique; RVA, right ventricular apex.

Successful ablation of AP at LPLW. (A) As a bidirectional conduction AP, RVA pacing confirmed ERAA characterized by eccentric atrial activation and a fusion of atrial and ventricular deflections. (B) After reconstruction of the left atrium with transseptal approach, MA was outlined. Activation mapping indicated the AP was positioned at LPLW. Initial one pulse train delivery at the atrial insertion site abolished pre-excitation (C, white point in B) and terminated retrograde conduction through AP (D). (E) Retrograde AP block was recorded after 3-PFA modality during RVA pacing. (F) Unexpected spontaneous orthodromic reciprocating tachycardia was observed. The tachycardia circuit was immediately obliterated after PFA at the green point adjacent to the white point (B). The presence of VA disconnection during RVA (G) and left ventricular pacing (H) was detected, and consolidation ablation was conducted (red points in B) in the 3-PFA modality (I). (J) Sinus beat stop exhibited when AS located at the ventricular part of MA showing low amplitude of atrial signals and high amplitude of ventricular signals from the tip of PFA (red point 1 in B). Right ventricular apex pacing was performed, and coronary angiography excluded coronary spasm or occlusion. (K) The coronary angiogram showed a normal left main stem, LAD, LCX, and RCA. The left coronary artery was viewed in RAO 30° + caudal angle 30° (a, b) and the right coronary artery in LAO 45° (c, d). Abl, ablation catheter; AP, accessory pathway; AS, ablation site; CS, coronary sinus; ERAA, earliest retrograde atrial activation; LAD, left anterior descending artery; LAO, left anterior oblique; LCX, left circumflex artery; LL, left lateral; LPLW, left posterolateral wall; MA, mitral annulus; RAO, right anterior oblique; RCA, right coronary artery; RVA, right ventricular apex; VA, ventriculo-atrial.

Elimination of an AP at RSP after PFA. (A) Spontaneous AVRT was observed with a long VA interval and discernible His bundle potential. Mapping during RVA pacing identified the site of ERAA at RSP (B, orange arrow) and marked at 3D activation mapping (red point 1, C). (D) A simple one delivery at the target suppressed the retrograde AP conduction. (E) The 3-PFA modality was applied at the same AS. (F) The AP elimination was confirmed by RVA pacing. The rest two red points indicated bonus lesions (C). Fluoroscopic images of the PFA catheter at target (a, LAO 40° view; b, RAO 30° view). Abl, ablation catheter; AP, accessory pathway; AS, ablation site; AVRT, atrioventricular reentrant tachycardia; CS, coronary sinus; ERAA, earliest retrograde atrial activation; HB, His bundle; LAO, left anterior oblique; PFA, pulsed field ablation; RAO, right anterior oblique; RSP, right posteroseptum; RVA, right ventricular apex; VA, ventriculo-atrial.
. | Pt no. . | . | |||||||||
---|---|---|---|---|---|---|---|---|---|---|---|
. | 1 . | 2 . | 3 . | 4 . | 5 . | 6 . | 7 . | 8 . | 9 . | 10 . | Averagea, n = 10 . |
Mapping rhythm | Ventricle pacing | Ventricle pacing | Ventricle pacing | Ventricle pacing | ORT | Ventricle pacing | Ventricle pacing | Ventricle pacing | Ventricle pacing | Ventricle pacing | – |
Type of conduction | Bidirectional conduction | Retrograde conduction | Retrograde conduction | Retrograde conduction | Retrograde conduction | Retrograde conduction | Retrograde conduction | Retrograde conduction | Retrograde conduction | Retrograde conduction | – |
Location of APs | LPLW | LALW | LALW | LALW | LPLW | LPS | LALW | LPLW | LPLW | RPS | – |
Acute success, n (%) | Yes | Yes | Yes | Yes | Yes | Yes | Yes | Yes | Yes | Yes | 10 (100%) |
Acute l success at the initial site, n (%) | No | No | Yes | Yes | Yes | Yes | Yes | No | No | Yes | 6 (60%) |
Successful ablation with transseptal approach | Yes | Yes | Yes | Yes | Yes | Yes | Yes | Yes | Yes | NO | 9 (90%) |
No. of total ASs | 5 | 5 | 8 | 5 | 3 | 7 | 2 | 5 | 3 | 4 | 4.7 ± 1.8 |
No. of bolus Ass | 3 | 2 | 7 | 4 | 2 | 6 | 1 | 3 | 1 | 3 | 3.2 ± 2 |
Total ablation time (s) | 8.7 | 3 | 18 | 4.4 | 2.9 | 9.6 | 1.6 | 5.3 | 2.3 | 7.5 | 6.3 ± 4.9 |
Ablation time at target (s) | 5.3 | 2.1 | 8.5 | 1 | 1.7 | 2.6 | 1 | 2.3 | 1.6 | 5.3 | 3.1 ± 2.4 |
Ablation time at bolus ASs (s) | 3.4 | 0.9 | 9.5 | 3.3 | 1.2 | 7 | 0.6 | 3 | 0.7 | 2.2 | 3.2 ± 2.9 |
Maximal CF at target (g) | 8 | 9 | 8 | 9 | 6 | 7 | 7 | 8 | 8 | 10 | 8 ± 1.2 |
Minimal CF at target (g) | 5 | 5 | 5 | 5 | 4 | 4 | 5 | 6 | 6 | 5 | 5 ± 0.7 |
Voltage of ERAA pre-PFA (mV) | 0.13 | 0.16 | 0.57 | 0.39 | 0.42 | 0.18 | 0.18 | 0.18 | 0.27 | 0.51 | 0.3 ± 0.16 |
Voltage of ERAA post-PFA (mV) | 0.08 | 0.07 | 0.22 | 0.08 | 0.2 | 0.08 | 0.09 | 0.05 | 0.11 | 0.1 | 0.11 ± 0.06 |
Total procedure time (min) | 48 | 61 | 60 | 74 | 73 | 104 | 45 | 54 | 65 | 72 | 65.5 ± 16.8 |
Skin-to-skin procedure time (min)b | 44 | 52 | 52 | 70 | 68 | 92 | 40 | 48 | 61 | 66 | 59.3 ± 15.5 |
PFA catheter dwell time (min) | 22 | 27 | 23 | 42 | 34 | 39 | 23 | 19 | 32 | 33 | 29.4 ± 7.8 |
Transseptal puncture time (min) | 5 | 6 | 7 | 5 | 5 | 13 | 6 | 7 | 10 | 7 | 7.1 ± 2.6 |
Fluoroscopy time (min) | 6.1 | 12 | 2.6 | 6.7 | 6.3 | 7.1 | 7.2 | 6.3 | 9.2 | 8.5 | 7.2 ± 2.4 |
. | Pt no. . | . | |||||||||
---|---|---|---|---|---|---|---|---|---|---|---|
. | 1 . | 2 . | 3 . | 4 . | 5 . | 6 . | 7 . | 8 . | 9 . | 10 . | Averagea, n = 10 . |
Mapping rhythm | Ventricle pacing | Ventricle pacing | Ventricle pacing | Ventricle pacing | ORT | Ventricle pacing | Ventricle pacing | Ventricle pacing | Ventricle pacing | Ventricle pacing | – |
Type of conduction | Bidirectional conduction | Retrograde conduction | Retrograde conduction | Retrograde conduction | Retrograde conduction | Retrograde conduction | Retrograde conduction | Retrograde conduction | Retrograde conduction | Retrograde conduction | – |
Location of APs | LPLW | LALW | LALW | LALW | LPLW | LPS | LALW | LPLW | LPLW | RPS | – |
Acute success, n (%) | Yes | Yes | Yes | Yes | Yes | Yes | Yes | Yes | Yes | Yes | 10 (100%) |
Acute l success at the initial site, n (%) | No | No | Yes | Yes | Yes | Yes | Yes | No | No | Yes | 6 (60%) |
Successful ablation with transseptal approach | Yes | Yes | Yes | Yes | Yes | Yes | Yes | Yes | Yes | NO | 9 (90%) |
No. of total ASs | 5 | 5 | 8 | 5 | 3 | 7 | 2 | 5 | 3 | 4 | 4.7 ± 1.8 |
No. of bolus Ass | 3 | 2 | 7 | 4 | 2 | 6 | 1 | 3 | 1 | 3 | 3.2 ± 2 |
Total ablation time (s) | 8.7 | 3 | 18 | 4.4 | 2.9 | 9.6 | 1.6 | 5.3 | 2.3 | 7.5 | 6.3 ± 4.9 |
Ablation time at target (s) | 5.3 | 2.1 | 8.5 | 1 | 1.7 | 2.6 | 1 | 2.3 | 1.6 | 5.3 | 3.1 ± 2.4 |
Ablation time at bolus ASs (s) | 3.4 | 0.9 | 9.5 | 3.3 | 1.2 | 7 | 0.6 | 3 | 0.7 | 2.2 | 3.2 ± 2.9 |
Maximal CF at target (g) | 8 | 9 | 8 | 9 | 6 | 7 | 7 | 8 | 8 | 10 | 8 ± 1.2 |
Minimal CF at target (g) | 5 | 5 | 5 | 5 | 4 | 4 | 5 | 6 | 6 | 5 | 5 ± 0.7 |
Voltage of ERAA pre-PFA (mV) | 0.13 | 0.16 | 0.57 | 0.39 | 0.42 | 0.18 | 0.18 | 0.18 | 0.27 | 0.51 | 0.3 ± 0.16 |
Voltage of ERAA post-PFA (mV) | 0.08 | 0.07 | 0.22 | 0.08 | 0.2 | 0.08 | 0.09 | 0.05 | 0.11 | 0.1 | 0.11 ± 0.06 |
Total procedure time (min) | 48 | 61 | 60 | 74 | 73 | 104 | 45 | 54 | 65 | 72 | 65.5 ± 16.8 |
Skin-to-skin procedure time (min)b | 44 | 52 | 52 | 70 | 68 | 92 | 40 | 48 | 61 | 66 | 59.3 ± 15.5 |
PFA catheter dwell time (min) | 22 | 27 | 23 | 42 | 34 | 39 | 23 | 19 | 32 | 33 | 29.4 ± 7.8 |
Transseptal puncture time (min) | 5 | 6 | 7 | 5 | 5 | 13 | 6 | 7 | 10 | 7 | 7.1 ± 2.6 |
Fluoroscopy time (min) | 6.1 | 12 | 2.6 | 6.7 | 6.3 | 7.1 | 7.2 | 6.3 | 9.2 | 8.5 | 7.2 ± 2.4 |
AP, accessory pathway; AS, ablation site; CF, contact force; ERAA, earliest retrograde atrial activation; LALW, left anterolateral wall; LPLW, left posterolateral wall; LSP, left posteroseptum; ORT, orthodromic reciprocating tachycardia; PFA pulsed field ablation; RSP, right posteroseptum.
aReported as mean with SD or percentage.
bDefined as the time from first femoral sheath inserted to last sheath removed.
. | Pt no. . | . | |||||||||
---|---|---|---|---|---|---|---|---|---|---|---|
. | 1 . | 2 . | 3 . | 4 . | 5 . | 6 . | 7 . | 8 . | 9 . | 10 . | Averagea, n = 10 . |
Mapping rhythm | Ventricle pacing | Ventricle pacing | Ventricle pacing | Ventricle pacing | ORT | Ventricle pacing | Ventricle pacing | Ventricle pacing | Ventricle pacing | Ventricle pacing | – |
Type of conduction | Bidirectional conduction | Retrograde conduction | Retrograde conduction | Retrograde conduction | Retrograde conduction | Retrograde conduction | Retrograde conduction | Retrograde conduction | Retrograde conduction | Retrograde conduction | – |
Location of APs | LPLW | LALW | LALW | LALW | LPLW | LPS | LALW | LPLW | LPLW | RPS | – |
Acute success, n (%) | Yes | Yes | Yes | Yes | Yes | Yes | Yes | Yes | Yes | Yes | 10 (100%) |
Acute l success at the initial site, n (%) | No | No | Yes | Yes | Yes | Yes | Yes | No | No | Yes | 6 (60%) |
Successful ablation with transseptal approach | Yes | Yes | Yes | Yes | Yes | Yes | Yes | Yes | Yes | NO | 9 (90%) |
No. of total ASs | 5 | 5 | 8 | 5 | 3 | 7 | 2 | 5 | 3 | 4 | 4.7 ± 1.8 |
No. of bolus Ass | 3 | 2 | 7 | 4 | 2 | 6 | 1 | 3 | 1 | 3 | 3.2 ± 2 |
Total ablation time (s) | 8.7 | 3 | 18 | 4.4 | 2.9 | 9.6 | 1.6 | 5.3 | 2.3 | 7.5 | 6.3 ± 4.9 |
Ablation time at target (s) | 5.3 | 2.1 | 8.5 | 1 | 1.7 | 2.6 | 1 | 2.3 | 1.6 | 5.3 | 3.1 ± 2.4 |
Ablation time at bolus ASs (s) | 3.4 | 0.9 | 9.5 | 3.3 | 1.2 | 7 | 0.6 | 3 | 0.7 | 2.2 | 3.2 ± 2.9 |
Maximal CF at target (g) | 8 | 9 | 8 | 9 | 6 | 7 | 7 | 8 | 8 | 10 | 8 ± 1.2 |
Minimal CF at target (g) | 5 | 5 | 5 | 5 | 4 | 4 | 5 | 6 | 6 | 5 | 5 ± 0.7 |
Voltage of ERAA pre-PFA (mV) | 0.13 | 0.16 | 0.57 | 0.39 | 0.42 | 0.18 | 0.18 | 0.18 | 0.27 | 0.51 | 0.3 ± 0.16 |
Voltage of ERAA post-PFA (mV) | 0.08 | 0.07 | 0.22 | 0.08 | 0.2 | 0.08 | 0.09 | 0.05 | 0.11 | 0.1 | 0.11 ± 0.06 |
Total procedure time (min) | 48 | 61 | 60 | 74 | 73 | 104 | 45 | 54 | 65 | 72 | 65.5 ± 16.8 |
Skin-to-skin procedure time (min)b | 44 | 52 | 52 | 70 | 68 | 92 | 40 | 48 | 61 | 66 | 59.3 ± 15.5 |
PFA catheter dwell time (min) | 22 | 27 | 23 | 42 | 34 | 39 | 23 | 19 | 32 | 33 | 29.4 ± 7.8 |
Transseptal puncture time (min) | 5 | 6 | 7 | 5 | 5 | 13 | 6 | 7 | 10 | 7 | 7.1 ± 2.6 |
Fluoroscopy time (min) | 6.1 | 12 | 2.6 | 6.7 | 6.3 | 7.1 | 7.2 | 6.3 | 9.2 | 8.5 | 7.2 ± 2.4 |
. | Pt no. . | . | |||||||||
---|---|---|---|---|---|---|---|---|---|---|---|
. | 1 . | 2 . | 3 . | 4 . | 5 . | 6 . | 7 . | 8 . | 9 . | 10 . | Averagea, n = 10 . |
Mapping rhythm | Ventricle pacing | Ventricle pacing | Ventricle pacing | Ventricle pacing | ORT | Ventricle pacing | Ventricle pacing | Ventricle pacing | Ventricle pacing | Ventricle pacing | – |
Type of conduction | Bidirectional conduction | Retrograde conduction | Retrograde conduction | Retrograde conduction | Retrograde conduction | Retrograde conduction | Retrograde conduction | Retrograde conduction | Retrograde conduction | Retrograde conduction | – |
Location of APs | LPLW | LALW | LALW | LALW | LPLW | LPS | LALW | LPLW | LPLW | RPS | – |
Acute success, n (%) | Yes | Yes | Yes | Yes | Yes | Yes | Yes | Yes | Yes | Yes | 10 (100%) |
Acute l success at the initial site, n (%) | No | No | Yes | Yes | Yes | Yes | Yes | No | No | Yes | 6 (60%) |
Successful ablation with transseptal approach | Yes | Yes | Yes | Yes | Yes | Yes | Yes | Yes | Yes | NO | 9 (90%) |
No. of total ASs | 5 | 5 | 8 | 5 | 3 | 7 | 2 | 5 | 3 | 4 | 4.7 ± 1.8 |
No. of bolus Ass | 3 | 2 | 7 | 4 | 2 | 6 | 1 | 3 | 1 | 3 | 3.2 ± 2 |
Total ablation time (s) | 8.7 | 3 | 18 | 4.4 | 2.9 | 9.6 | 1.6 | 5.3 | 2.3 | 7.5 | 6.3 ± 4.9 |
Ablation time at target (s) | 5.3 | 2.1 | 8.5 | 1 | 1.7 | 2.6 | 1 | 2.3 | 1.6 | 5.3 | 3.1 ± 2.4 |
Ablation time at bolus ASs (s) | 3.4 | 0.9 | 9.5 | 3.3 | 1.2 | 7 | 0.6 | 3 | 0.7 | 2.2 | 3.2 ± 2.9 |
Maximal CF at target (g) | 8 | 9 | 8 | 9 | 6 | 7 | 7 | 8 | 8 | 10 | 8 ± 1.2 |
Minimal CF at target (g) | 5 | 5 | 5 | 5 | 4 | 4 | 5 | 6 | 6 | 5 | 5 ± 0.7 |
Voltage of ERAA pre-PFA (mV) | 0.13 | 0.16 | 0.57 | 0.39 | 0.42 | 0.18 | 0.18 | 0.18 | 0.27 | 0.51 | 0.3 ± 0.16 |
Voltage of ERAA post-PFA (mV) | 0.08 | 0.07 | 0.22 | 0.08 | 0.2 | 0.08 | 0.09 | 0.05 | 0.11 | 0.1 | 0.11 ± 0.06 |
Total procedure time (min) | 48 | 61 | 60 | 74 | 73 | 104 | 45 | 54 | 65 | 72 | 65.5 ± 16.8 |
Skin-to-skin procedure time (min)b | 44 | 52 | 52 | 70 | 68 | 92 | 40 | 48 | 61 | 66 | 59.3 ± 15.5 |
PFA catheter dwell time (min) | 22 | 27 | 23 | 42 | 34 | 39 | 23 | 19 | 32 | 33 | 29.4 ± 7.8 |
Transseptal puncture time (min) | 5 | 6 | 7 | 5 | 5 | 13 | 6 | 7 | 10 | 7 | 7.1 ± 2.6 |
Fluoroscopy time (min) | 6.1 | 12 | 2.6 | 6.7 | 6.3 | 7.1 | 7.2 | 6.3 | 9.2 | 8.5 | 7.2 ± 2.4 |
AP, accessory pathway; AS, ablation site; CF, contact force; ERAA, earliest retrograde atrial activation; LALW, left anterolateral wall; LPLW, left posterolateral wall; LSP, left posteroseptum; ORT, orthodromic reciprocating tachycardia; PFA pulsed field ablation; RSP, right posteroseptum.
aReported as mean with SD or percentage.
bDefined as the time from first femoral sheath inserted to last sheath removed.
No char or thrombus was observed upon PFA catheter removal. The mean total procedure time was 65.5 ± 16.8 min, skin-to-skin procedure time 59.3 ± 15.5 min, PFA dwell time 29.4 ± 7.8 min, transseptal puncture time 7.1 ± 2.6 min, and fluoroscopy time 7.2 ± 4.4 min, respectively. The maximal and minimal CF at targets was 8 ± 1.2 and 5 ± 0.7 g, respectively.
Primary endpoints during short-term follow-up
All patients completed follow-up visits at 1, 3, and 6 months. No recurrence of arrhythmia or SAEs was detected during the 6-month follow-up (see Supplementary material online, Table S4). Beyond 12-lead surface and 24-h Holter monitoring, in five (50%) patients receiving EES at the conclusion of follow-up, clinical tachycardias were no longer elicited by the same stimulation protocol as at baseline. No patients complained of any symptoms of suspicious arrhythmia, chest pain, paralysis, and loss of consciousness during the whole follow-up. There was no evidence of embolic lesion with the brain magnetic resonance imaging in nine patients with left APs after PFA. The blood tests, TEE, 12-lead surface ECG, and 24-h Holter monitoring were similar and within normal range during the follow-up (see Supplementary material online, Table S5).
Discussion
This study represents the largest sample of patients with APs to date receiving PFA. Under the current PFA modality and parameters, 100% acute procedural and 6-month success was achieved, including seven left anterolateral APs, two left inferolateral APs, and one right posteroseptal AP. One patient encountered transient sinus arrest because of pain-induced vagal overreaction during PFE delivery. No other SAEs were detected during the procedure and 6-month follow-up.
Success of pulsed field ablation
Radiofrequency ablation aimed at scarring arrhythmogenic myocardium or abnormal AV myocardial connections was successful in the vast majority of patients suffering from a symptomatic AVRT.1,2 Corcia et al.5 found that the recurrence of left and right lateral APs related mainly to poor CF after RFA. Recurrence of para-Hisian and anteroseptal APs can be caused by low RF energy delivery. In posteroseptal substrates, the main reason accounting for recurrence was failure to damage the deep or epicardial location of the APs. Therefore, activation mapping to precisely locate the position of the APs using a 3D-EAMS beyond fluoroscopy, the utilization of irrigated-tip catheters, CF monitoring, and consolidation ablation were introduced to increase the efficiency of RFA.13 In theory, PFE acts on a volume, irrespective of whether it includes blood or contacted tissue. So, lesion formation should not depend on CF during PFE delivery. However, recent studies indicated that PFE delivery falls off as the square of the distance. There will be less current density travelling through adjacent tissue where the electrodes are not in tight contact. Using a catheter in bipolar electrodes, CF-dependent effectiveness is mounting because relatively less PFE density travels through the target tissue than monopolar electrodes.14 Thus, we ensured moderate CF for deeper lesion formation during PFA after learning lessons from our previous study.12,15 Additionally, a large-curve catheter will improve catheter contact and steerability along the full length of the MA where nine APs were located at the left free wall with the help of a long sheath with a transseptal approach. An accurate formula incorporating CF and PFE intensity is desired for accurate prediction of lesion size in the future.
In our pre-clinical experiments, continuous and transmural lesions were produced by the same PFA catheter and parameters.15 The current study demonstrated a notably high efficiency of PFA, as supported by shorter ablation and procedure times compared to reported data with RFA.16 We applied repetitive PFE delivery near the target in order to create an expanded range of lesions.15,17 In this study, we suggest that the 3-PFA modality for bonus lesions is rational for the elimination of APs. Although the cumulative damage mainly determined the effects of PFA protocol, the effects of a given population of cardiomyocytes and its neighbouring environment should not be neglected.9 For instance, PFA can hardly damage HB under physiological doses of PFE for its deep distribution and thick fibrous tissue coverage. Accessory pathways are composed almost entirely of highly abnormal myocytes characterized by aberrant myofibril organization. An irrigated-tip catheter was recommended for deep myocardial or epicardial substrates for posteroseptal APs,18 rarely requiring combined right and left atrial RF applications or intracoronary applications. However, a deep myocardial band wrapped in rich fibroblasts might be the risk factor for the conduction recovery of posteroseptal AP after the delivery of non-thermal PFE. When AP connections are located in the subepicardial region, it may be extremely difficult to eliminate the AP in the CS using PFA due to its selective damage to cardiomyocytes. We hypothesize that successful abolition of posteroseptal AP with current power (one AP at RPS in this study; two APs at left posteroseptum and one AP at RSP in our previous study) should contribute to the shallow endocardial distribution of AP band or vulnerable insertion in atria and ventricle conduction junction to cumulative IRE.
Safety of pulsed field ablation
Besides efficacy, the safety advantages of PFA over RFA have aroused tremendous enthusiasm and prompted clinical studies in treating various arrhythmias. Delivery of PFE to myocardial tissue can preferentially cause cardiomyocyte-specific necrosis.6,19 One severe complication was persistent AV block after RFA at APs close to the HB.20,21 Effects of PFE on electrophysiological properties of different components of AV conduction system depend on cell morphological difference, such as myocyte size, myofibril ratio, and adjacent tissue.19 Unlike HB, the AV node is not insulated with a thick fibrous sheath, which should expose this delicate structure to a greater risk of damage from PFA. Interestingly, the AV conduction system has been proven to be less susceptible than cardiomyocytes to PFE.21 Our recent study demonstrated that PFA-induced transient AV block was associated with the close distance between ASs and HB during slow pathway modification. In the setting of thermal safety profile, one study indicated that the maximal temperature rises were slight (7.6°C) for PFE and substantial for RF energy (39.8°C) at the surface of perfused thigh muscle of swine.22 Overall, we propose that PFA might be particularly applicable to para-Hisian APs. The strategy of 1-PFA modality as a test ahead 3-PFA modality for APs is like RFA using lower energy with titration method. This strategy is based on the results from our animal experiments showing that the damaged degree of tissue was positively correlated with PFE intensity.15 Further, we can utilize the distinct reaction to PFE in the AV conduction system and AP to identify the critical substrate of tachycardia circuits near the AV node.23 Another fatal complication of RFA was coronary injuries as a result of anatomic proximity to the AS.24,25 Some reports revealed that the posterolateral branch was the most frequently injured vessel after posteroseptal AP ablations, followed by the distal right coronary artery and left circumflex artery.24 Among some cases of left lateral AP ablation, the left coronary system injury could be involved.25 Of note, one patient suffered from brief sinus arrest during PFA at the anterolateral AP. The underlying causes can contribute to coronary artery spams or air embolism, modulation of vagal ganglia, and sympathetic overexcitation.26–28 Opposite to RFA, a high prevalence of coronary artery spasms was reported during PFA compared to RFA, but the spasm resolution was rapidly achieved following the administration of nitrates.26 Moreover, PEF compared with RF energy induced significantly weaker and less durable suppression of cardiac autonomic regulations.29 After excluding coronary artery injury or air embolism by coronary angiography, we consider that parasympathetic overexcitation due to pain or vagal ganglia stimuli during ablation may be the rational explanation for the occurrence of brief sinus arrest. In this study, no ST-T segment changes were detected during PFA for all APs; thus, coronary angiography was not routinely conducted for each patient.
Another safety concern of PFA was silent cerebral lesions after PFA in the left atrium.30,31 The mechanism of silent cerebral lesions is associated with air or thrombus entry during catheter exchange, over-delivered ASs, microbubble formation, left atrial dwell time, modality of ablation, and multielectrode catheter.31 From the outcome of IMPULSE and PERCAT I + II studies, few patients who suffered a clinical stroke or transient ischaemic attack were attributed to sheath management and catheter exchange, rather than PFA itself, although this is impossible to prove.32 We have observed microbubble formation using intracardiac echocardiography in our FIM trial as well. In the current study, there was no evidence to support cerebral ischaemic events according to signs of stroke or cerebral magnetic resonance imaging post left atrial PFA. Compared to previous PFA studies showing evidence of stroke, we considered fewer catheter electrodes, lower power, and less left atrial dwell time used with the current PFA catheter as the important factors in avoiding the generation of mass gaseous emboli.33–35 Further trials to prove the relationship between silent cerebral lesions and PFA are necessary.
In addition to those safety advantages, there are other potential practical merits in terms of reducing procedure time, which can translate into improved patient comfort and safety. The easy-to-uptake RF-like focal PFA catheter, CF monitoring, 3D-EAMS technology, ultrarapid lesion formation, and uncommon slight muscle contractions have further reduced the left atrial dwell time and total procedure time.12,36,37 The low incidence of muscle contractions decreased perforation for catheter misplacement and remapping time. The PAF parameters employed in our study, such as biphasic waveforms, low power, and short pulse duration, permit the generation of clinically relevant lesions without treatment-prohibitive muscle contraction.38
Limitations
This present analysis is limited by the small sample size and single-arm study from one centre. A randomized multicentre trial with a larger population and long-term follow-up is warranted. Furthermore, the recurrence was roughly diagnosed by external surface ECG recorders and EES. While it is difficult to conduct invasive remapping in patients without any sign of persistent clinical tachycardia. Ultimately, the ablation parameters used were confined to left APs and may not be applicable to other APs.
Conclusions
Accessory pathways receiving PFA showed remarkable efficacy and safety. The positive results from the pilot study definitely will boost confidence for the ongoing multicentre trial.
Supplementary material
Supplementary material is available at Europace online.
Acknowledgements
We thank Mr Zhifang Zhang from MicroPort EP, for his efforts pertaining to intracardiac electrogram data entry. We also express our thanks to Mrs Wei Luo from Jinshan Primary School and her son Yizhe Shen for their contribution to data entry and verification. Clinical trial registration numbers: NCT05770921 (ClinicalTrials.gov) and NCT05820035 (ClinicalTrials.gov).
Funding
This work was supported by the Key Technology R&D Program of Ningbo (2022Z149, 2023Z188) and the Basic Public Welfare Research Project of Zhejiang Province (LGJ20H20001).
Data availability
The data underlying this article are available in the article and in its online Supplementary material.
References
Author notes
Caijie Shen, Zhenyu Jia and Yibo Yu contributed equally to the study.
Conflict of interest: This study is granted by the manufacturer of the PFA system (Shanghai MicroPort EP MedTech Co., Ltd., China) to provide free use of the equipment. H.C. has received research grant support from the company. The authors and MicroPort EP company have no conflicts of interest to disclose.