-
PDF
- Split View
-
Views
-
Cite
Cite
Alessio Gasperetti, Fabrizio Assis, Hemantkumar Tripathi, Masahito Suzuki, Akhilesh Gonuguntla, Rushil Shah, James Sampognaro, Marco Schiavone, Parag Karmarkar, Harikrishna Tandri, Determinants of acute irreversible electroporation lesion characteristics after pulsed field ablation: the role of voltage, contact, and adipose interference, EP Europace, Volume 25, Issue 9, September 2023, euad257, https://doi.org/10.1093/europace/euad257
- Share Icon Share
Abstract
Pulsed field ablation (PFA) is a non-thermal ablative approach in which cardiomyocyte death is obtained through irreversible electroporation (IRE). Data correlating the biophysical characteristics of IRE and lesion characteristics are limited. The aim of this study was to assess the effect of different procedural parameters [voltage, number of cycles (NoCs), and contact] on lesion characteristics in a vegetal and animal model for IRE.
Two hundred and four Russet potatoes were used. Pulsed field ablation lesions were delivered on 3 cm cored potato specimens using a multi-electrode circular catheter with its dedicated IRE generator. Different voltage (from 300 to 1200 V) and NoC (from 1 to 5×) protocols were used. The impact of 0.5 and 1 mm catheter-to-specimen distances was tested. A swine animal model was then used to validate the results observed in the vegetable model. The association between voltage, the NoCs, distance, and lesion depth was assessed through linear regression. An almost perfect linear association between lesion depth and voltage was observed (R2 = 0.95; P < 0.001). A similarly linear relationship was observed between the NoCs and the lesion depth (R2 = 0.73; P < 0.001). Compared with controls at full contact, a significant dampening on lesion depth was observed at 0.5 mm distance (1000 V 2×: 2.11 ± 0.12 vs. 0.36 ± 0.04, P < 0.001; 2.63 ± 0.10 vs. 0.43 ± 0.08, P < 0.001). No lesions were observed at 1.0 mm distance.
In a vegetal and animal model for IRE assessment, PFA lesion characteristics were found to be strongly dependent on voltage settings and the NoCs, with a quasi-linear relationship. The lack of catheter contact was associated with a dampening in lesion depth.
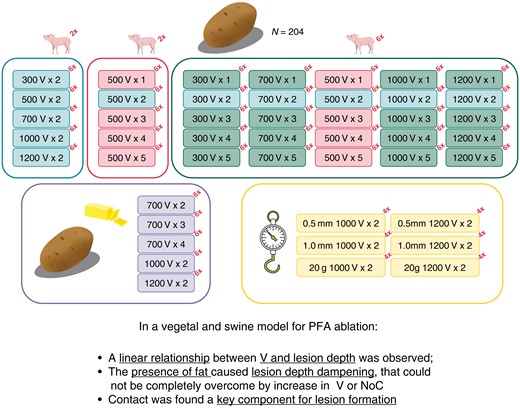
Changes in electroporation protocol parameters (voltage and number of cycles) are associated with significant changes in lesion depth; the data derived from a pre-clinical model in this study can help guide ablation protocols in humans treated with pulsed field ablation (PFA).
Lack of contact was associated with significant dampening and even complete disappearance of the lesion; these pre-clinical data call for a systematic assessment of contact in humans during PFA procedures.
Presence of an adipose layer is associated with lesion depth dampening; this finding suggests caution before the extensive use of PFA in an epicardial setting.
Introduction
Irreversible electroporation (IRE) is a technique that induces cell death through the application of electrical fields to the tissue.1,2 Pulsed field ablation (PFA) is a non-thermal ablative approach in which cardiomyocyte death is obtained through IRE, by applying high-voltage, short-duration pulses to induce pore formation in cellular membranes.2,3 In recent times, PFA has been proposed as a novel alternative to radiofrequency (RF) and cryo-energy-based ablation to achieve pulmonary vein isolation (PVI) for the management of atrial fibrillation (AF).4–6 Several in-human studies addressing the effectiveness of PFA have been reported recently.7,8 Pulsed field ablation-based PVI has been shown to be as effective as the other forms of single-shot PVI techniques, with a 100% first-pass PVI, a short-left atrium in-dwelling time, and mid-term arrhythmic outcomes in comparable ranges.9–11
Irreversible electroporation currently represents an appealing option for AF ablation procedures due to the minimal thermal energy imparted to the target tissue and the higher-than-surrounding-tissue susceptibility of cardiomyocytes to electrical fields.12 These characteristics allow PFA-based lesions to have high cardiac tissue selectivity, potentially minimizing oesophageal lesions compared with other forms of thermal energy.13–16 To date, data assessing the impact of changing biophysical characteristics of IRE delivery during PFA are limited. In fact, many published studies report data only from fixed PFA protocols, and IRE generators are often ‘black boxes’ that do not allow making parameter changes. Although presently, Watt and delivery time settings for RF can be changed, it is expected that in the future, IRE generators will allow operators to change procedural settings as well, to achieve personalized lesion delivery regimens. The aim of this study is therefore to assess the effect of different procedural parameters of the PFA procedure [voltage, number of cycles (NoCs), and contact] on lesion characteristics (surface and depth) in a pre-clinical validated vegetal model.
Methods
Experimental models
The effect of IRE biophysical parameters on PFA lesion characteristics was assessed using a bench vegetal model. The potato model has been well described as a reliable model to assess electroporation, and methods for IRE in this vegetal model have been previously published.17–20 Findings from the vegetal model were then confirmed in an animal model. No Institutional Review Board (IRB) approval for the vegetal model was required. This study complied with the Declaration of Helsinki for the use of animals in medical research.
Vegetal model
Russet potatoes are an established model for pre-clinical assessment of IRE.17–20 Two hundred and four freshly harvested Russet potatoes were used in the experiment. All potatoes were sliced horizontally to achieve a round core slice of 3 cm thickness. This slice was then placed in a clear glass box filled with a bath of 0.9% saline at controlled room temperature. The PFA procedure was then performed by delivering microsecond-scale bipolar biphasic energy through a multi-electrode circular catheter (Abbott Laboratories, St Paul, MN, USA), varying voltage output and the NoCs, as reported below. Saline was replaced every sixth specimen or if a change >1.5°C was observed at the thermometer level to maintain a constant environment. Catheter stability was ensured using a fixed lock system, employing a telescopic arm and a 9 Fr vascular sheath. During the contact force assessment setting, a custom-made linear catheter was used, with IRE energy being delivered from the same generator. Supplementary material online, Figure S1 displays the experimental setting. In all experimental settings using the circular catheter, complete electrode contact of all 12 electrodes was visually confirmed before energy application. Complete contact was visually assessed by two independent investigators (A.G. and F.A.) by inspecting the catheter/potato interface through the clear glass setting from all four projections, with the evaluation of a third expert investigator (H.T.) serving as a tiebreaker in case of disagreement. A high-sensitivity scale was used for contact force measurement (in grams) when the linear catheter was used.
Animal model validation
Ten adult female Yorkshire pigs (∼70 pounds of weight) were used to confirm findings from the vegetal model. After obtaining ultrasound-guided vascular access at a femoral level, lesions were performed under fluoroscopic guidance in the anterior, lateral, and posterior walls of the right atrium, as well as in the right atrial appendage, by using a multi-electrode circular catheter (Abbott Laboratories), varying voltage output and the NoCs, as reported in the section below.
Catheter used and study protocol settings
A multi-electrode circular catheter (Abbott Laboratories) with its dedicated IRE generator was used throughout the experiment. The catheter used for the experiment (14 electrodes on the catheter, 2 on the spline, 3 mm electrode spacing, exposed electrode length 1.5 mm; 7 Fr of diameter) delivered 40 bursts of 25 biphasic pulses each (quadrangular wave, number of pulses per generator cycle = 1000). While modifications to IRE generator parameters were allowed, as per manufacturer indications, voltage (V) ranges were restricted to the 50–1200 V window.
Experiments were performed in five different settings:
Voltage assessment: PFA was performed in five groups of six red Russet potatoes each. Five different increasing voltage levels (300, 500, 700, 1000, and 1200 V) were used during the ablation, one for each potato group. All potato groups received a two-cycle protocol.
Number of cycles assessment: Five groups of six red Russet potatoes each were ablated. Five different cycle protocols (1×, 2×, 3×, 4× and 5×) were used during PFA. The voltage for all four groups was set at 500 V.
Interaction between voltage and the NoCs: 16 additional groups of 6 potatoes each were ablated with the following protocols (300 V 1×, 300 V 3×, 300 V 4×, 300 V 5×, 700 V 1×, 700 V 3×, 700 V 4×, 700 V 5×, 1000 V 1×, 1000 V 3×, 1000 V 4×, 1000 V 5×, 1200 V 1×, 1200 V 3×, 1200 V 4×, 1200 V 5×), in order to assess the effect on the lesion depth of the NoCs at all available voltages and from 1× to 5× NoC protocols.
Adipose interference assessment: Four groups of six red Russet potatoes each were used for this assessment. On the inferior half of every potato slice, a thin layer of fixed thickness of unsalted butter, obtained through a surgical slicer from a cold frozen butter block, was placed on the specimen. Excess butter at the borders was removed to facilitate specimen handling. Half of the catheter electrodes were placed on the butter-coated side of the potato slice, while the other half were placed on the non-butter-coated side. The conductivity characteristics of unsalted melted butter were meant to replicate the presence of interposed adipose tissue between the delivering catheter and the target tissue. The first group had energy delivery set at 700 V, the second at 1000 V, and the third at 1200 V. In the first three groups, all received a two-cycle protocol. The fourth group was composed of three potatoes receiving a 700 V 3× cycle and three potatoes receiving a 700 V 4× cycle protocol. For this setting, replication in the animal model was not pursued.
Impact of contact: Six groups of four specimens each were used for this assessment. Potatoes were placed in the saline bath, and then sub-millimetre (mm) tin-foil spacers (0.125 mm each) were layered over the study specimen. The first two groups had a layering of four spacers (0.5 mm total), while the second two groups had eight spacers (1.0 mm total). The linear catheter was then put in direct contact with the spacers by manoeuvring the telescoping arm and by using a contact force of 20 g as a mark of complete contact. Once adequate contact was achieved, the spacers were carefully removed by a lateral slide, leaving the linear catheter at a 0.5 or 1.0 mm distance from the specimen surface. A two-cycle protocol with 1000 or 1200 V was used, generating four groups: 0.5 mm 2× 1000 V; 0.5 mm 2× 1200 V; 1.0 mm 2× 1000 V; and 1.0 mm 2× 1200 V. Two additional groups of four specimens undergoing lesions with the linear catheter, 20 g of contact force, two cycles, no spacers, and either a 1000 or a 1200 V protocol were used as a control.
Figure 1 and Supplementary material online, Table S1 summarize the breakdown of the different specimen groups used for this experiment.

Experimental study workflow. A total of 204 potatoes and 10 piglets have been used in the study, grouped as represented. Each box represents a group of specimens. The presence of a piglet image over a box indicates the validation of those findings in an animal model. The number reported in the top right corner as a superscript represents the number of specimens in the group. The larger, rounded rectangles show for what analysis specimen groups were used. Grouping (assessments as per the Methods section)—top section, first rectangle: voltage assessment; top section, second rectangle: NoC assessment; top section, third rectangle: interaction between voltage and the NoCs; bottom section, first rectangle: adipose interference assessment; bottom section, second rectangle: contact assessment; NoCs, number of cycles.
Lesion maturation, processing, and assessment
After lesion delivery, all potato central slices were covered with the removed, non-ablated potato slices to prevent air oxidation and wrapped in paper towels. After 120 min from lesion delivery, the central slice was placed into a blue dye solution for 45 min for lesion enhancement (as per methods reported by Castellví et al.20). The overall timing of lesion maturation was chosen in accordance with what would be considered an ‘acute’ setting (<3 h), similar to what has been previously reported in both clinical and pre-clinical studies.20,21 Lesions on each slice were then individually assessed both longitudinally and transmurally. For transmural assessment, the circular lesion was cut at full thickness at the deepest lesion site, and maximal lesion depth was measured using an image-processing software [ImageJ software (nih.gov)] by two independent reviewers (R.S. and H.T.). The mean value between the reviewers was then considered. If a variance >10% was observed, a third assessment by an additional independent reviewer (F.A.) was introduced as a tiebreaker.
In the animal model, a 3 h waiting time after last atrial lesion delivery was observed, and animals were then euthanized as per our standard animal laboratory protocol. Triphenyl tetrazolium chloride was infused 15 min prior to euthanasia to facilitate the differentiation between metabolically active and inactive tissue, as reported in previous animal studies addressing this topic.22 Heart and lungs were then harvested, and lesion characteristics were assessed at a gross pathology level, with measurements of lesion depth and lesion surface area. Supplementary material online, Figures S2 and S3 display examples of post-lesion specimens.
Statistical analysis
The Shapiro–Wilk test was used to assess the normality of the distribution of continuous variables. Normally distributed continuous variables were expressed as mean ± standard deviation, and comparisons were performed using the Student’s t-test. Non-normally distributed variables were expressed as median (inter-quartile range), and comparisons were performed using the Mann–Whitney U test. The association between lesion depth and study variables was graphically assessed first and then analysed using a linear regression if the association was deemed linear. Beta-coefficient and R2 were reported. A two-sided P-value <0.05 was deemed statistically significant throughout the study.
Results
Impact of voltage on lesion surface area
A progressive increase in lesion surface area was observed for the 300–500–700 V (1.04 ± 0.24 vs. 1.88 ± 0.52 vs. 2.57 ± 0.27 cm2; P < 0.001) protocols. The lesion surface area then plateaued at the 1000 and 1200 V protocols (5.21 ± 0.18 vs. 5.15 ± 0.15; P = 0.796). Figure 2 shows the lesion surface area increasing with an increase in voltage. In the animal model, a similar dose response was seen, with a plateau at 1000 V protocol as well (5.01 ± 0.18 cm2 at 1000 V vs. 5.04 ± 0.11 cm2; P = 0.759).
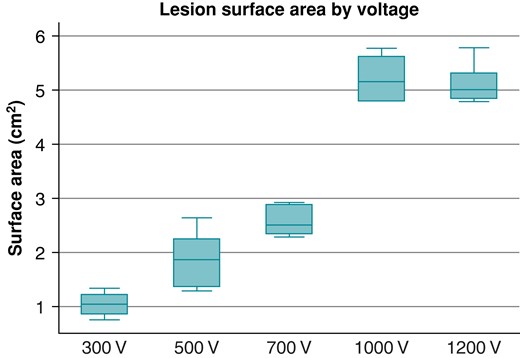
Impact of voltage and cycle-number variation on maximal lesion depth
The maximal lesion depth measured with the 2× protocol was significantly different in the five different voltages tested (1.09 ± 0.11 vs. 1.50 ± 0.09 vs. 1.68 ± 0.05 vs. 2.07 ± 0.16 vs. 2.49 ± 0.09; P < 0.001). A progressive increase in the maximal lesion depth with an increase in the voltage used for lesion delivery was observed with an almost perfect linear association [beta-coefficient/100 V: 0.146 (0.132–0.159); R2 = 0.95; P < 0.001). Figure 3A graphically displays the association between voltage and the observed increase in maximal lesion depth. A similarly linear relationship was observed between the NoCs and the maximal lesion depth observed (1.35 ± 0.12 vs. 1.51 ± 0.10 vs. 1.54 ± 0.10 vs. 1.68 ± 0.13 vs. 1.84 ± 0.08; P < 0.001) (R2 = 0.73; P < 0.001). Figure 3B graphically illustrates this linear relationship. Both observations were confirmed in the lesions retrieved in the animal model [maximal lesion depth observed at 1200 V 2.21 ± 0.11; beta-coefficient/100 V: 0.112 (0.108–0.141); R2 = 0.87; P < 0.001; maximal lesion depth observed at 5× NoC 1.71 ± 0.09; beta-coefficient for the NoCs: 1.11 (1.08–1.19); R2 = 0.71; P = 0.001]. A multivariate model integrating the impact of voltage and the NoCs showed that both parameters were strongly associated with lesion depth [beta-coefficient/100 V: 0.151 (0.145–0.157), P < 0.001; beta-coefficient/one cycle: 0.116 (0.102–0.131), P < 0.001]. A margin plot showing lesion depth according to the integration of voltage and the NoCs is reported in Figure 4. While a linear relationship between voltage and lesion depth at all NoCs is preserved, the impact of adding extra cycles resulted in only an incremental gain after the 3× cycle protocol.
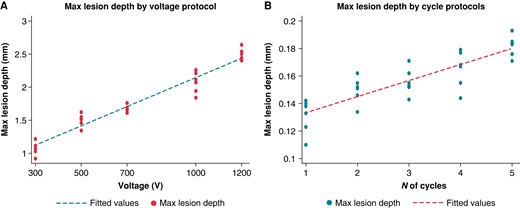
Relationship between voltage increases and maximal lesion depth (A) and between the NoCs and maximal lesion depth (B). NoCs, number of cycles.
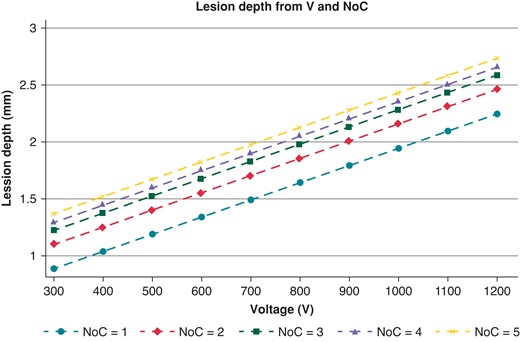
Modification of lesion depth (in mm) at the variation of voltage and the NoCs. NoCs, number of cycles; V, voltage.
Adipose interference and contact assessment
While the lesions observed in the half of the vegetal specimens without the thin layer of unsalted butter were identical to the ones observed in specimens treated with similar protocols, no lesion was visualized in the half of specimens in which adipose interference was tested, at 700 V 2×, 1000 V 2× or 1200 V 2× protocols. Although lesions beneath the butter were visualized when a protocol with increased NoCs was used (700 V 3× and 700 V 4×), both lesion surface area (0.75 ± 0.11 vs. 3.03 ± 0.21, P < 0.001; 0.98 ± 0.14 vs. 3.38 ± 0.21, P < 0.001) and lesion depth (0.95 ± 0.07 vs. 1.80 ± 0.09, P < 0.001; 1.00 ± 0.04 vs. 1.86 ± 0.10, P < 0.001) were significantly lower than what was observed in specimens without adipose interference treated with the same protocol IRE protocol. Figure 5 reports examples of surface lesions in specimens with adipose interference. Compared with the control group, a significant dampening effect on lesion depth was observed at 0.5 mm of distance at both tested voltage protocols (1000 V 2×: 2.11 ± 0.12 vs. 0.36 ± 0.04, P < 0.001; 1200 V 2×: 2.63 ± 0.10 vs. 0.43 ± 0.08, P < 0.001). No lesions were observed at 1.0 mm distance. Figure 6 reports examples of surface lesions in specimens at 0.5 and 1.0 mm distance.
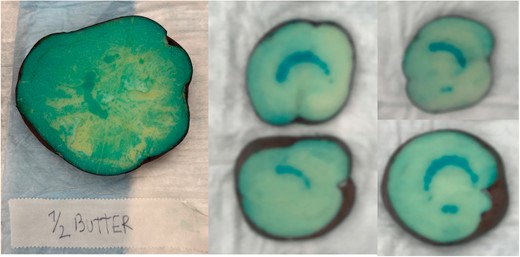
Significant lesion dampening observed in the presence of butter (mimicking intramyocardial fat characteristics).
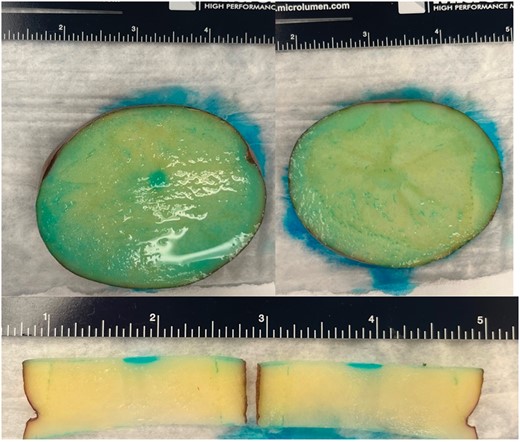
Lesion obtained at 0.5 mm (left panel and lower panel) and at 1 mm (right panel) catheter-specimen distance.
Discussion
This study reports a serial and sequential assessment of the relationship between IRE parameters (voltage, the NoCs, and contact force) and lesion characteristics in a vegetal and swine model of PFA. The main results may be summarized as follows:
A strong linear correlation between voltage and both lesion surface area and lesion depth was observed.
An increase in the NoCs from 1× to 3× was associated with a progressive increase in lesion surface area. At 4×, however, this observed effect plateaued, and further increases in the NoCs did not result in larger lesions. A strong linear correlation between the NoCs and lesion depth was observed as well.
When accounting for both voltage and NoC changes, both parameters were strongly associated with lesion depth, with voltage maintaining a perfectly linear relationship with lesion depth and the NoCs instead showing only an incremental gain after a 3× protocol (Graphical Abstract).
The presence of a thin layer of butter interfered with lesion formation, regardless of the voltage of the lesion protocol. Increasing the NoCs allowed lesion formation, but with smaller and shallower lesions compared with specimens without a layer of butter.
Adequate contact was a strong determinant of lesion characteristics, with a catheter-specimen distance of 0.5 mm significantly dampening lesion depth. No lesions were visualized with a catheter-specimen distance of 1.0 mm.
Irreversible electroporation and potatoes
In accordance with the concept of 3Rs (Replacement, Reduction, and Refinement) in animal research, the use of potato models for IRE has been strongly endorsed in the last decade. While their use in cardiac electrophysiology has been limited, raw potato tubers have been shown to be a reliable model for IRE experiments due to their homogenous electrical properties and isotropic tissue structure, as well as the distinctly visible pattern of lesions that can be observed after IRE delivery by numerous experiments.18–20 Changes in viscoelastic and electrical properties in potatoes following IRE delivery have been extensively characterized,23,24 and potatoes have been successfully used to study the effects of IRE delivery on electric field distribution, metabolic changes, and tissue damage.18,24–27 This study bridges an extensively validated vegetal model into the field of cardiac electrophysiology research, using it to test the impact of changes in procedural parameters and lesion characteristics in a reliable and reproducible setting. The subsequent validation with an animal model assures adequate data replicability in a model extensively used for AF ablation lesion assessment.
Irreversible electroporation determinants and pulsed field ablation
Among IRE determinants, the main two, as well as the easiest to tweak, are voltage and the NoCs.28 Currently available data, both in pre-clinical models and in humans, have been retrieved from settings in which a fixed PFA protocol was used.9,10,29 Hence, data addressing the individual impact of these determinants on lesion surface area and depth in a serial and sequential, controlled fashion are limited. The aim of this study was to partially address this knowledge gap, testing the correlation between voltage, the NoCs, and lesion characteristics in vegetal and animal models. By presenting this data, our hope is to pave a data-driven way to the possibility of operator-customized PFA procedures.
Voltage, the number of cycles, and lesion characteristics
A clear linear correlation between voltage increases and both lesion surface area and lesion depth has been observed for all voltages between 100 and 1200 V. This finding seems fairly intuitive, with voltage serving in PFA procedures a very similar role to Watt in RF procedures. In line with previous pre-clinical findings reported by Suárez et al.,26 a similarly linear relationship between the NoCs and lesion depth was observed in all tested settings, with the NoCs approximating the role of ablation time in RF procedures. When accounting for both voltage and the NoCs at the same time, both parameters were clearly associated with lesion depth and surface, although with a different relative weight. The relationship between lesion depth and voltage remained linear, regardless of the NoCs, while the gain in lesion depth achieved with an increase in the NoCs seemed to become only fractionally incremental, at all voltages, after a 3× protocol was established. It should, however, be noted that all these lesions were delivered in settings maximizing catheter contact with target tissue. The relative weight of the IRE parameters may be different if contacts were to change. Finally, it should also be noted that previous computational studies on IRE ablation showed the importance of accounting for anisotropy in the lesion formation protocol.30 Our study did not address this topic, as our models were not adequate for such analyses. Anisotropy, however, clearly represents an important new frontier in IRE and catheter ablation studies. Although not implemented in recently published studies targeting regions with anisotropic tissue,31,32 we believe that future studies should take anisotropy into consideration, when assessing the effects of IRE on lesion formation.
Adipose interference and contact assessment
Our data clearly showed how even the presence of a small amount of non-conductive tissue can really reduce the effectiveness of this non-thermal ablation strategy. As the clinical indications for the use of PFA expand, these data acquire further importance.33,34 The presence of this adipose interference is in fact of particular importance when ventricular or epicardial lesions are delivered, due to the potential presence of intramyocardial or peri-myocardial fat. While increasing voltages were generally associated with improved lesion formation, in this setting, increasing the NoCs seemed more important than voltages in achieving lesions. A reason for this finding may be a potentially non-linear additive effect obtained through multiple NoCs, with the first cycles overcoming the difference in conductivity and the subsequent ones directly generating a lesion. Regardless of the reason, these findings seem to suggest that a single, uniform ablation protocol for both the atria and the ventricles may not be so easy to achieve. Allowing operators to have full access to and control over the IRE procedural parameters during PFA procedures may further augment the versatility of this new form of ablation, enabling procedure-specific and operator-tailored protocols, similar to what is currently available for RF and cryo-energy procedures.
Finally, our study presents concrete evidence that adequate contact between catheter and target tissue is required to achieve viable lesions even during PFA procedures. A gap of 0.5 mm between the tip of the linear catheter and the specimen resulted in a dampening of >80% in lesion depth, and no lesions were visualized when IRE was delivered with the catheter at 1.0 mm distance. Only a very modest gain in lesion depth was achieved at 0.5 mm with the increase in voltage output, and, although our study settings were capped at 1200 V, it does not seem that higher voltage protocols can completely overcome lack of contact with target tissue. In the only other available study on this topic, the impact of catheter-to-tissue distance on epicardial lesion formation in a porcine model was similar, with a linear dampening shown at the increase of catheter-to-tissue distance; although when using a different generator with a more aggressive protocol (bipolar energy 1500 V 4× NoC), some shallow lesions were observed at even >2 mm distances.35 Such findings seem to strongly support the need for the introduction of a contact-force feedback loop in the design of future IRE-delivering catheters. Given the sometimes-higher range of voltage used in clinical patient setting, the possibility of overcoming distance with an increase in voltage cannot be completely ruled out. One additional potential question that arises from this combined data is whether the real driver of this lesion dampening is the lack of contact or the catheter-to-tissue distance, as most fields dissipate following a non-linear (often exponential) relationship with distance from the source (the catheter electrode). While contact and catheter-to-tissue distance are often collinear and can be used as a proxy measure of one another, there are situations in which they are formally distinct. It would be interesting to test whether, once all electrodes are in complete contact, an additional increase in contact force brings any further advantages in terms of lesion characteristics. In a recent, preliminary porcine analysis, additional contact force did not seem to yield significant incremental value once complete contact was achieved.36 With currently available technologies, however, complete contact feedback is not yet routinely available. These findings therefore have multiple implications at an intraprocedural and manufacturing level, showing the need for feedback systems addressing contact during catheter-based PFA procedures. Further studies will be needed to address this issue in humans and the impact of tissue contact on lesion durability.
Limitations and future directions
The data have been collected from well-established vegetal and animal models of PFA. While we hope that in the future a direct lesion assessment may be performed in-human non-invasively, to date no direct data on lesion assessment can be found in an in-human model. Although we fully recognize the limitations of our non-human model, we made an attempt to get to the closest that we could to an in vivo model. In this regard, a limitation of our study is represented by the use of 0.9% saline as the medium for the potato lesion, which has a different conductivity from human blood. Because the electrical conductivity of saline is higher than that of blood flow, we acknowledge that this might affect the impedance of the electric field. On the other hand, the saline temperature is lower than the body temperature, thereby potentially affecting the generation of the thermal lesion in the heart tissue due to convective heat transfer from RF ablation. This difference might be hampered when considering non-thermal lesions obtained with PFA. Moreover, the use of butter to emulate epicardial fat was justified by the physical characteristics of the unsalted dairy product. Nevertheless, we acknowledge that this model could not account for the complex cell-to-cell interactions that happen between epicardial adipocytes and myocardiocytes, as well as potentially presenting some inequalities in terms of overall width on the surfaces of potatoes. Further studies and the development of additional models are required to fully explore this topic. Moreover, the staining of potatoes represents a relative marker of field distribution and not a direct assessment of lesion size in a non-beating heart model, a factor that should be taken into account when considering the actual biological effect of IRE on myocardiocytes. This study, however, presents proof-of-concept data and at least partially fills a gap in current IRE knowledge. Another potential limitation of this manuscript pertains to the range of voltages used in the lesion protocols: due to the manufacturer’s indications, we were not able to test voltage settings higher than 1200 V, a voltage setting slightly lower than the current voltage being used in clinical practice. Furthermore, while it is true that higher voltages may be used in the left atrial setting to achieve a completely transmural set of lesions for PVI, there are multiple situations in which a less-than-transmural lesion may be of interest for the operator (epicardial lesion, area in proximity to the sinus or atrio-ventricular node). Knowing the shape of voltage/depth or NoC/depth dose–response curves, even at low-to-mid voltages, may be of great importance for the future application of this ablation technique. Finally, it should be noted that our assessment has been performed in an acute setting. However, even if a longer lesion maturation time were to result in a deeper lesion, the shape of the relationship between IRE parameters and lesion characteristics is unlikely to change, at least in a vegetal model. Still, further studies testing the observed relationship at longer lesion maturation times and with catheters from different manufacturers are needed to complement and complete our findings.
Conclusions
In validated vegetal and animal models for IRE assessment, PFA lesion characteristics were found to be strongly dependent on voltage settings and the NoCs, with a clear linear association between lesion depth and voltage. The presence of a small adipose layer, as well as lack of catheter contact, was shown to be significantly associated with a dampening in lesion surface area and depth in the vegetal model. This dampening could only be partially overcome through increases in voltage or the NoCs in the ablation protocol.
Supplementary material
Supplementary material is available at Europace online.
Funding
No external funding was received for the performance of this study.
Data availability
The data supporting this manuscript are available upon reasonable request with the corresponding author.
References
Author notes
Conflict of interest: none declared.