-
PDF
- Split View
-
Views
-
Cite
Cite
Carola Gianni, Javier E Sanchez, Sanghamitra Mohanty, Chintan Trivedi, Domenico G Della Rocca, Amin Al-Ahmad, J David Burkhardt, G Joseph Gallinghouse, Patrick M Hranitzky, Rodney P Horton, Luigi Di Biase, Andrea Natale, Isolation of the superior vena cava from the right atrial posterior wall: a novel ablation approach, EP Europace, Volume 20, Issue 9, September 2018, Pages e124–e132, https://doi.org/10.1093/europace/eux262
- Share Icon Share
Abstract
Superior vena cava (SVC) isolation might be difficult to achieve because of the vicinity of the phrenic nerve (PN) and sinus node. Based on its embryogenesis, we hypothesized the presence of preferential conduction from the right atrial (RA) posterior wall, making it possible to isolate the SVC antrally, sparing its anterior and lateral aspect.
This is a descriptive cohort study of 105 consecutive patients in which SVC isolation was obtained with radiofrequency ablation, starting in the septal aspect of the SVC–RA junction and continued posteriorly and inferiorly targeting sites of early activation until electrical isolation was obtained. Acute SVC isolation was achieved in 103 (98%) patients; the mean distance between the site of SVC isolation and the SVC–RA junction was 19.9 ± 5.3 (range 9.7–33.7) mm. During follow-up, 2 (2%) patients developed symptomatic diaphragmatic paralysis due to transient right PN injury; 13 patients underwent a repeat ablation: SVC reconnection was observed in 5 patients, and re-isolation was easily achieved by targeting the corresponding sites of early activation.
Superior vena cava isolation can be completed by targeting its septal segment and sites of early activation in the posterior SVC–RA junction and RA posterior wall; this is a feasible alternative ablation strategy in patients in which SVC isolation cannot be completed with the standard approach. The risk of sinus node injury or SVC stenosis are eliminated; PN injury is still possible but can easily be prevented with high-output pacing to exclude a true posterior course of the PN.
What’s new?
Isolation of the superior vena cava (SVC) can be completed by targeting its septal segment and sites of early activation in the posterior SVC–right atrial (RA) junction and RA posterior wall.
By sparing the lateral and anterior aspect, the risk of sinus node injury or SVC stenosis are eliminated.
This is a feasible alternative ablation strategy in patients whose SVC isolation cannot be completed with the standard approach, most commonly for the presence of phrenic nerve capture in the lateral segments.
Introduction
Ablation of non-pulmonary vein (PV) triggers is an important step of atrial fibrillation (AF) ablation. The superior vena cava (SVC) is one of the most common non-PV sites: its isolation is valuable when triggers arise from it, and it can be done empirically as a reasonable addition to PV isolation in patients with paroxysmal AF.1–6 SVC isolation can be achieved with a segmental ablation approach at the level of the SVC–right atrial (RA) junction, targeting areas of earliest activation. However, given the vicinity of the sinus node and phrenic nerve (PN) in its lateral and anterior segments, complete isolation is not possible in up to 14% of patients.5 The SVC originates from the fusion of the right anterior and right common cardinal veins and drains into the primitive RA via the right horn of the sinus venosus; this is later incorporated into the developed RA becoming its smooth posterior part.7 Due to its embryogenesis, we hypothesized the presence of preferential conduction from the RA posterior wall to the SVC, making it possible to isolate the SVC more antrally, sparing its anterolateral aspect.
Methods
This is a prospective descriptive cohort study of consecutive patients who underwent AF ablation between March 2015 and January 2017 and in whom SVC isolation was performed ablating in the RA posterior wall, sparing its anterolateral aspect. Patients were included in the study when SVC isolation could not be obtained with a standard segmental ablation approach targeting the lateral segments for the presence of PN capture; patients were not included in the study if the SVC was silent or its isolation was obtained without targeting the RA posterior wall.
Superior vena cava isolation was performed in sinus rhythm, after completing left atrial ablation, irrespective of the presence of spontaneous or high-dose isoproterenol-induced SVC-related arrhythmias.5 To perform SVC isolation, a 10- or 20-pole circular mapping catheter (CMC) was positioned at the junction between the RA and SVC: the correct position was confirmed by recording sharp vein potentials superimposed to blunt far-field atrial electrograms and visualizing the CMC between the right superior PV and the right pulmonary artery on intracardiac echocardiography (Figure 1). Radiofrequency (RF) ablation was performed with an irrigated-tip ablation catheter set at 35–40W with the endpoint of local potential abatement. In all patients, the septal aspect of the SVC–RA junction was ablated first, irrespective of the site of breakthrough recorded on the CMC. To follow, the posterior segment (12 o’clock when viewing the SVC from a left anterior oblique—LAO—superior projection) was ablated starting from the SVC–RA junction and continued inferiorly in the RA posterior wall, targeting the sites of early activation relative to the earliest local EGMs recorded in the SVC, until electrical isolation was obtained (Figure 2). A 3D electro-anatomical mapping (EAM) system (Carto 3 or Ensite NavX Velocity) was used to track RF lesions and PN capture sites. The 3D EAM system was used to measure the distance from the site of SVC isolation and the SVC–RA junction—approximated to the location of the CMC; this measure was confirmed in an anterior–posterior fluoroscopic view (Figure 3). Complete SVC isolation was defined as the absence of SVC potentials at each electrode pair of the CMC positioned at the SVC–RA junction.
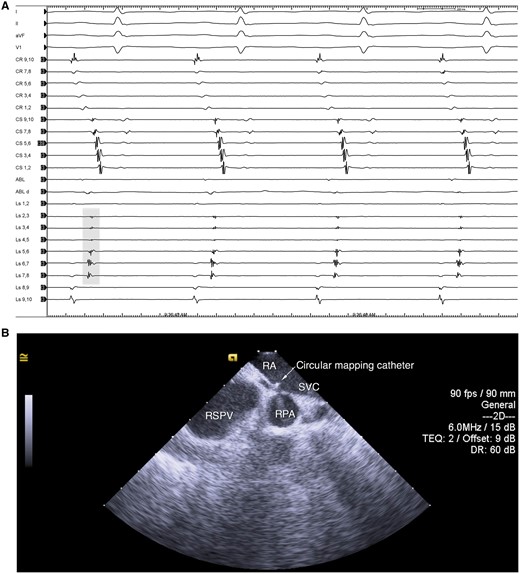
CMC at theSVC–RA junction. A: intracardiac electrograms showing sharp vein potentials (grey shade) and far-field RA electrograms. B: intracardiac echocardiography showing the CMC positioned between the RSPV and RPA. CMC, circular mapping catheter; RA, right atrium; RPA, right pulmonary artery; RSPV, right superior pulmonary vein; SVC, superior vena cava.
Example of SVC isolation from the RA posterior wall. A: 3D EAM in the RAO and LAO superior view, displaying ablation points (purple dots) with the site of SVC isolation (green dot), along with PN capture sites (white dots). B: electrograms recorded during the various stages of SVC isolation: left, baseline; middle, ablation at the level of septal SVC–RA junction (pink star in the EAM); right, ablation at the level of the posterior SVC-RA junction (yellow star). C: ablation in the RA posterior wall (green star) showing progressive delay and isolation. D: intracardiac echocardiography showing the site of SVC isolation. ABL, ablation; CR, crista terminalis; CS, coronary sinus; EAM, electroanatomical map; Ls, circular mapping catheter; LAO, left anterior oblique; PN, phrenic nerve; RA, right atrium; RAO, right anterior oblique; SVC, superior vena cava.
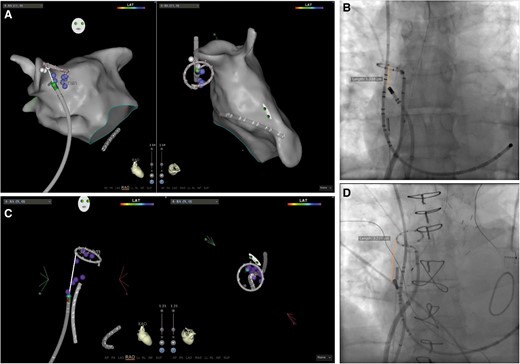
Example of measurements of the distance between the site of SVC isolation and the SVC–RA junction, which was approximated to the location of the CMC. The EAMs display a RAO, (left) and a LAO superior (right) view, whereas fluoroscopy shows an AP view. AP, anteroposterior; CMC, circular mapping catheter; EAM, electroanatomic map; LAO, left anterior oblieque; RA, right atrium; RAO, right anterior oblique; SVC, superior vena cava.
The course of the right PN was determined by performing high-output pacing (bipolar pacing >10 mA at 2 ms) with an adequate washout of paralytics, as confirmed by the presence of facial muscle contraction with peripheral nerve stimulation. PN mapping was initially only performed to exclude PN capture in the posterolateral aspect of the SVC; however, after two cases of transient PN injury (see Results), we began performing PN mapping also in the posterior aspect of the SVC–RA junction and inferiorly in the RA posterior wall. If PN capture was present, ablation was not performed at the site of pacing to avoid PN injury. At the end of the procedure, normal diaphragmatic motion was confirmed with fluoroscopy; at follow-up, patients were instructed to report symptoms compatible with PN injury (shortness of breath, cough), which prompted a chest X-ray evaluation to look for elevation of the right hemidiaphragm. For sinus node injury, any acceleration of the sinus rate observed during ablation was considered a sign of impending sinus node injury, prompting RF energy discontinuation. Additionally, at follow-up patients underwent periodical clinical evaluation along with 7-day Holter recordings, which would reveal sinus node dysfunction.
Demographics, medical history, procedural, and follow-up data were obtained from an Institutional Review Board-approved prospective database. The RF time was collected from the start of ablation of the SVC/RA junction to the end of ablation in the RA posterior wall following SVC isolation.
Statistical analysis was performed with GraphPad Prism 6.0 for Mac (GraphPad Software, La Jolla, CA, USA). Continuous variables are presented as mean ± standard deviation or median and interquartile range, as appropriate; categorical variables are expressed as percentages.
Results
Results are presented in Table 1. A total of 105 patients were included in the study: mean age was 67 ± 9 years, and 19 (18%) were females. Forty (38%) patients had paroxysmal AF, 32 (31%) patients had persistent AF, and 33 (31%) patients had long-standing persistent AF. The procedure was a repeat ablation in 59 (56%) cases, with a mean procedure time of 125 ± 37 min.
. | n = 105 . |
---|---|
Age (years) | 67 ± 9 |
Female gender | 19 (18%) |
AF type | |
Paroxysmal AF | 40 (38%) |
Persistent AF | 32 (31%) |
Longstanding persistent AF | 33 (31%) |
Acute SVC isolation | 103 (98%) |
RF time (s) | 134 (89–203) |
Site of first delay | |
Septal RA/SVC junction | 22 (22%) |
Posterior RA/SVC junction | 9 (8%) |
RA posterior wall | 72 (70%) |
Distance between site of SVC isolation and SVC/RA junction | |
Mean (mm) | 19.9 ± 5.3 |
Range (mm) | 9.7–33.7 |
Category | |
<5.0 mm | 0 (0%) |
5.0–9.9 mm | 2 (2%) |
10.0–14.9 mm | 11 (11%) |
15.0–19.9 mm | 43 (41%) |
20.0–24.9 mm | 31 (30%) |
25.0–29.9 mm | 13 (13%) |
≥30.0 mm | 3 (3%) |
. | n = 105 . |
---|---|
Age (years) | 67 ± 9 |
Female gender | 19 (18%) |
AF type | |
Paroxysmal AF | 40 (38%) |
Persistent AF | 32 (31%) |
Longstanding persistent AF | 33 (31%) |
Acute SVC isolation | 103 (98%) |
RF time (s) | 134 (89–203) |
Site of first delay | |
Septal RA/SVC junction | 22 (22%) |
Posterior RA/SVC junction | 9 (8%) |
RA posterior wall | 72 (70%) |
Distance between site of SVC isolation and SVC/RA junction | |
Mean (mm) | 19.9 ± 5.3 |
Range (mm) | 9.7–33.7 |
Category | |
<5.0 mm | 0 (0%) |
5.0–9.9 mm | 2 (2%) |
10.0–14.9 mm | 11 (11%) |
15.0–19.9 mm | 43 (41%) |
20.0–24.9 mm | 31 (30%) |
25.0–29.9 mm | 13 (13%) |
≥30.0 mm | 3 (3%) |
AF, atrial fibrillation; RA, right atrium; SVC, superior vena cava.
. | n = 105 . |
---|---|
Age (years) | 67 ± 9 |
Female gender | 19 (18%) |
AF type | |
Paroxysmal AF | 40 (38%) |
Persistent AF | 32 (31%) |
Longstanding persistent AF | 33 (31%) |
Acute SVC isolation | 103 (98%) |
RF time (s) | 134 (89–203) |
Site of first delay | |
Septal RA/SVC junction | 22 (22%) |
Posterior RA/SVC junction | 9 (8%) |
RA posterior wall | 72 (70%) |
Distance between site of SVC isolation and SVC/RA junction | |
Mean (mm) | 19.9 ± 5.3 |
Range (mm) | 9.7–33.7 |
Category | |
<5.0 mm | 0 (0%) |
5.0–9.9 mm | 2 (2%) |
10.0–14.9 mm | 11 (11%) |
15.0–19.9 mm | 43 (41%) |
20.0–24.9 mm | 31 (30%) |
25.0–29.9 mm | 13 (13%) |
≥30.0 mm | 3 (3%) |
. | n = 105 . |
---|---|
Age (years) | 67 ± 9 |
Female gender | 19 (18%) |
AF type | |
Paroxysmal AF | 40 (38%) |
Persistent AF | 32 (31%) |
Longstanding persistent AF | 33 (31%) |
Acute SVC isolation | 103 (98%) |
RF time (s) | 134 (89–203) |
Site of first delay | |
Septal RA/SVC junction | 22 (22%) |
Posterior RA/SVC junction | 9 (8%) |
RA posterior wall | 72 (70%) |
Distance between site of SVC isolation and SVC/RA junction | |
Mean (mm) | 19.9 ± 5.3 |
Range (mm) | 9.7–33.7 |
Category | |
<5.0 mm | 0 (0%) |
5.0–9.9 mm | 2 (2%) |
10.0–14.9 mm | 11 (11%) |
15.0–19.9 mm | 43 (41%) |
20.0–24.9 mm | 31 (30%) |
25.0–29.9 mm | 13 (13%) |
≥30.0 mm | 3 (3%) |
AF, atrial fibrillation; RA, right atrium; SVC, superior vena cava.
Acute SVC isolation was achieved in 103 (98%) patients, with a median RF time of 134 (89–203) s; in 2 (2%) patients, it was not possible to achieve acute SVC isolation for the presence of PN capture in the RA posterior wall. While performing SVC isolation, initial conduction delay from the RA to the SVC was observed during ablation in RA posterior wall in 72 (70%) patients, followed by ablation in the septal and posterior aspect of the SVC/RA junction, i.e. 22 (22%) and 9 (8%) patients, respectively. The mean distance between the site of SVC isolation and the SVC–RA junction was 19.9 ± 5.3 (range 9.7–33.7) mm; in the majority (74, or 71%) of patients, the site of SVC isolation was located between 15 mm and 24.9 mm from the SVC–RA junction.
There were no periprocedural complications. More specifically, in no patient we observed sinus node acceleration during RF nor reduction/absence of right diaphragm movement at the end of the procedure.
During follow-up, 2 (2%) patients developed symptomatic (dyspnoea on exertion) diaphragmatic paralysis due to delayed right PN injury: in both cases, this was not present at the end of the procedure and was transient, resolving within 6 months’ post-ablation. Following this, we began to routinely perform PN mapping in the posterior segment of the SVC–RA junction and inferiorly in the RA posterior wall: this precluded complete SVC isolation in the aforementioned two cases (Figure 4). No sinus node dysfunction was observed at follow-up in any patient.
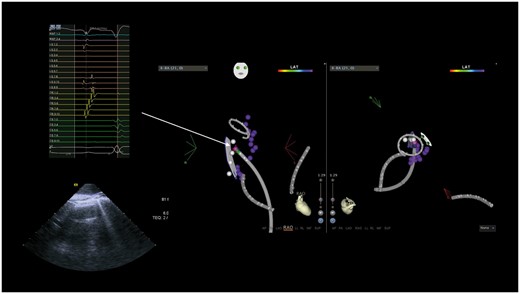
Phrenic nerve mapping (white dots) showing capture in the RA posterior wall, in a site of early activation (pink dot, MAP 1–2) relative to the earliest activation recorded on the CMC; in this patient, SVC isolation was not completed. CMC, circular mapping catheter; CR, crista terminalis; CS, coronary sinus; LS, circular mapping catheter; MAP, ablation catheter; RA, right atrium; SVC, superior vena cava.
At a mean follow-up of 9 ± 6 months, 13 patients underwent a repeat ablation because of an atrial arrhythmia recurrence (11 patients for atypical atrial flutter, 1 patient for focal atrial tachycardia, and 1 patient for atrial fibrillation). When the atypical atrial flutter was present at the time of the repeat ablation procedure (2 of the 11 patients), it was left sided, with the isthmus located in the anterior left atrial septum in one patient and the anterior wall at the base of the left atrial appendage in the other. Reconnection was observed in 5 of 13 patients: the site was the septal segment in three patients and the RA posterior wall in the other two patients. In all, re-isolation was easily achieved obtained by targeting the corresponding sites of early activation.
Discussion
This is the first study that shows the feasibility of completing SVC isolation by targeting early activation sites in the RA posterior wall, thus sparing its anterior and lateral aspects. With this approach, the rate of SVC isolation is high, with no occurrence of sinus node injury. PN injury is still possible but can be easily prevented with high-output pacing to exclude a true posterior course of the PN.
The SVC is a well-appreciated trigger for AF: similar to the PVs, there are myocardial sleeves that extend from the atrium into the vein and contain cells that display enhanced automaticity and triggered activity.8 For this reason, the SVC is an important target during AF ablation procedures: its isolation is valuable in patients with triggers arising for it, and its empirical isolation has been shown to be beneficial in patients with paroxysmal AF.3–6 The myocardial sleeves that extend from the RA to the SVC are highly asymmetric.9 Therefore, contrary to the PVs, circumferential ablation is not necessary and segmental isolation is the strategy of choice.10 However, when the earliest activation is found to be in the anterior and lateral segments, it might not be possible to complete SVC isolation, given the vicinity of the sinus node and right PN. Indeed, in a previous study we have shown that, for this reason, complete SVC isolation is not possible in up to 14% (21 of 155) of patients.5 To overcome this limitation, an alternative ablation approach is needed.
In this study, we have shown that—following ablation of the septal aspect of the SVC–RA junction—targeting early potentials lower in the RA posterior wall leads to complete SVC isolation, obviating the need to ablate laterally and anteriorly. This can be explained by the presence of preferential conduction from the RA posterior wall to the SVC. During embryogenesis, the SVC originates from the fusion of the right anterior and right common cardinal veins and drains into the primordial RA via right horn of the sinus venosus (Figure 5). The latter is then incorporated into the developed RA to form its smooth posterior wall, called sinus venarum, which is separated from the anterolateral trabeculated wall (derived from the primordial RA) by the crista terminalis.7 The crista terminalis is a fibromuscular ridge with marked anisotropic conduction that extends from the high interatrial septum, passing anterior to the ostium of the SVC, descending along the posterolateral wall of the RA up to the isthmus.12 Given the shared embryological origin and the presence of the potential barrier of the crista terminalis, it is possible to hypothesize the existence of preferential conduction from the RA posterior wall to the SVC. Indeed, in our population, SVC isolation was obtained following septal ablation, by targeting early potentials in the posterior segment, ablating an average of 2 cm below the SVC–RA junction in the smooth-walled portion of the RA. This allows to avoid ablation inside the SVC and in the lateral/anterior aspect of the SVC–RA junction, preventing SVC stenosis and sinus node injury.
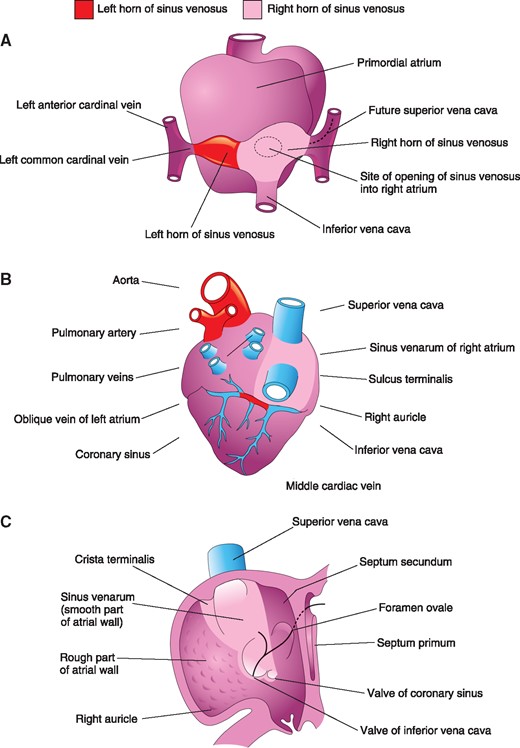
Illustrations of the primordial (A) and developed (B, C) right atrium and sinus venosus, showing SVC embryogenesis. With permission from Moore et al.11
With this approach, SVC isolation can be obtained in most of the patients (98%). Moreover, in case of a repeat procedure, the majority of patients had persistent SVC isolation, whereas in the remaining reconnection occurred in only one segment (two in the posterior RA wall, three in the septal aspect of the SVC–RA junction), and it can be easily addressed with limited ablation at this site. Moreover, no patient developed sustained atrial flutter involving the posterior wall of the RA, at the level of ablation set required for SVC isolation. Of note, although the risk of sinus node injury and SVC stenosis are eliminated, PN injury is still possible: overall, at least 4% of the patients displayed a true posterior course of the PN, as shown by the occurrence of transient diaphragmatic paralysis in two patients and incomplete SVC isolation following high-output PN mapping in another two patients. This is explained by the highly variable course of the right PN: although anterolateral when it runs along the SVC in the mediastinum, its course can shift posteriorly when it crosses the SVC–RA junction, approaching the right pulmonary veins.13 Indeed, in a study by Schmidt et al.14, pace mapping showed a true posterior course of the PN (12 o’clock in a LAO superior projection) in 4 of 15 patients. Therefore, PN mapping is still recommended to delineate the course of the PN prior to ablation in the RA posterior wall. However, the high acute success rate along with the ease of performing high-output pacing makes this a feasible alternative approach to perform SVC isolation.
Limitations
This is a descriptive cohort study, therefore the main limitation is the absence of a control group. Nonetheless, this is a large cohort and the acute success rate is higher than what we previously reported for segmental SVC isolation obtained with a traditional ablation approach.5 Another limitation is the lack of screening for diaphragmatic paralysis. Although we confirm normal diaphragm motion with fluoroscopy at the end of the procedure, during follow-up we do not perform routine chest radiography, and PN injury is suspected only in case of symptoms. This might have led to underestimation of the true risk of PN injury; however, the significance of asymptomatic PN injury is unknown, and, in most patients, recovery occurs within 1 year.15 Finally, only a small number of patients underwent a repeat procedure; therefore, the true prevalence of SVC isolation durability with this approach is unknown.
Conclusions
SVC isolation can be obtained with ablation of its septal segment and completed by targeting sites of early activation in the posterior SVC–RA junction and RA posterior wall, thus sparing its anterior and lateral aspect. With this approach, the rate of SVC isolation is high, with no occurrence of sinus node injury or SVC stenosis. PN injury is still possible, but can easily be prevented with high-output pacing to exclude a true posterior course of the PN. Therefore, this is a feasible alternative ablation strategy in patients whose SVC isolation cannot be completed with the standard approach.
Conflict of interest: none declared.