-
PDF
- Split View
-
Views
-
Cite
Cite
Keping Chen, Xiaolin Zheng, Yan Dai, Hao Wang, Yue Tang, Tingyu Lan, Jinping Zhang, Yi Tian, Baojie Zhang, Xiaohong Zhou, Matthew Bonner, Shu Zhang, Multiple leadless pacemakers implanted in the right ventricle of swine, EP Europace, Volume 18, Issue 11, November 2016, Pages 1748–1752, https://doi.org/10.1093/europace/euv418
- Share Icon Share
The miniaturized leadless pacemaker has recently emerged as a bradycardia therapy in humans, and many patients may need at least two pacemakers in their lifetime. Thus, the present study assessed the effect of two leadless pacemakers in the right ventricle (RV) on cardiac function in a swine model.
Fourteen mini pigs were chronically studied. Each animal received two sequential leadless pacemakers in the RV with 1 month between two separate implantations. All animals were then followed for 6 months. Cardiac function was assessed with echocardiography at baseline, the second implantation, and the end of the 6-month follow-up. A necropsy at the end of the study was performed to measure the length of the fibrous tissue covering the devices and assess tricuspid valve integrity. Four animals died during the surgical procedure, and one animal died of infection during the follow-up period. In the remaining nine animals, there were no significant changes in left ventricular ejection fraction, aortic time integral, cardiac output, and left ventricular size from baseline to the end of the study. The mean length of fibrous tissue covering Micra devices was 14.3 ± 7.8 mm. No tricuspid valve injury was observed.
Multiple leadless pacemakers implanted in the RV do not appear to impact cardiac function. Therefore, it appears safe to implant multiple leadless pacemakers in the same heart.
Transcatheter leadless pacemaker.
Majority of pacemaker patients need at least two devices in their life time. The present study demonstrated the feasibility of implanting two leadless pacemakers in the right ventricle in an animal model.
The study raised several safety issues that should be considered during clinical practice.
Introduction
Conventional pacemakers with transvenous leads in the right ventricle (RV) have been used clinically for more than 50 years. While pacemakers have demonstrated effectiveness in treating bradycardia, complications associated with pacemaker implantation can cause patient injury and increased healthcare costs. The most common complications after pacemaker implantation include pacing lead dislodgement and fracture, pocket haematoma and infection, and tricuspid valve impairment.1–11 The investigation by Kirkfeldt et al.10 found that 9.5% of 5492 patients who received a pacemaker experienced at least one complication.
A transcatheter pacemaker with a significantly smaller size compared with conventional pacemaker has been developed for bradycardia therapy because of the advancement of technology in the areas of miniaturization, batteries, electronic packaging, biocompatibility, ultra-low power circuitry, and communication systems. Due to its small size, the transcatheter pacemaker has been designed to be implanted inside the RV. Leadless pacemakers such as Nanostim™ and Micra™ have recently been investigated clinically in patients with bradycardia worldwide.12–16
It has been estimated that the average patient age at implantation is 75 years, and the average pacemaker now lasts ∼11 years.17 Therefore, many pacemaker patients will need at least two devices in their life time. Concerns have been raised that multiple devices in the heart may compromise cardiac function. Thus, in the present study, it was investigated whether two transcatheter pacemakers (Micra) sequentially implanted in the RV impacted cardiac function in a swine model.
Methods
The study protocol was reviewed and approved by the Ethical Committee of Animal Experimental Center in Fuwai hospital and complied with the institutional and national guidelines for the care and use of laboratory animals.
General procedures
A total of 14 mini pigs (45–55 kg, either sex) were used. Animals were sedated with mixture of ketamine (35 mg/kg, Jiangsu Hengrui Medicine Co. Ltd, Jiangsu, China) and diazepam (1.5 mg/kg, Sanjing Pharmaceutical Co. Ltd, Heilongjiang, China) and then intubated for artificial respiration with a respirator (Siemens Servo 900C; Siemens Medical Solutions, Erlangen, Germany) and under continuous cardiac-respiratory monitoring. Anaesthesia was maintained by intravenous infusion of 3.5% pentobarbital (Sigma-Aldrich Co. LLC., MO, USA). Ringer's solution was supplied whenever necessary depending on blood gas–electrolyte analysis. Cardiac functional assessment was conducted with transthoracic echocardiography before implantation. After implanting the first Micra device, animals were allowed to recover for 1 month. Each pig was then implanted with a second device, and during the procedure, cardiac functional assessment with echocardiography was repeated. Following the second implantation, animals were followed with daily monitoring of temperature, activity, and dietary intake for additional 6 months. At the end of the study, animals were anaesthetized, the locations of the Micra pacemakers were documented using fluoroscopy, and cardiac function assessment was repeated. Then, animals were sacrificed with 3 M KCl (Zizhu Pharmaceutical Co. Ltd, Beijing, China), the chest was opened, the epicardial surface was examined, and the RV was carefully opened to examine the devices in the RV. Tissue near the electrode of each device was fixed, sliced, and stained for histological analysis.
Implantation of Micra device
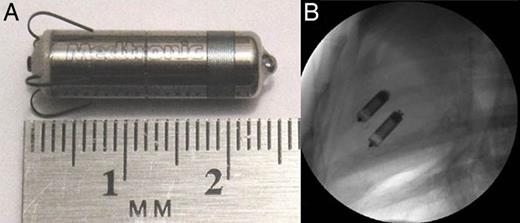
Micra device and fluoroscopic imaging with Micra devices: (A) an example of the Micra device and (B) fluoroscopic imaging of two Micra devices implanted in the RV.
Then, the delivery system was articulated to access the RV through the tricuspid valve. Once the system reached the RV apex, the Micra device was deployed from the delivery system allowing the fixation tines to embed into the cardiac tissue. A fixation test was performed under fluoroscopy to ensure that at least two of the four tines were embedded in the myocardium. Once the device was adequately fixed in the RV, the tether was gently pulled out of the delivery system to free the device. Then, the delivery system was removed from the introducer, and the introducer was withdrawn from the jugular vein. The jugular vein was repaired, and the subcutaneous tissue and skin were sutured. One month later, the second Micra device was implanted in an identical manner ∼1 cm from the previously implanted Micra as shown in Figure 1B.
Data collection and analyses
Procedure time and fluoroscopy time
The implantation procedure times were recorded from skin cut to closure. The procedure fluoroscopy times in minutes were also recorded.
Echocardiography
In all sedated animals, transthoracic echocardiography was conducted with a 3.5-MHz phased-array transducer of the echo machine (GE VividE9 Dimension, GE Health, Milwaukee, WI). Two-dimensional and Doppler examinations were performed to make the measurements of cardiac four-chamber left ventricular end-diastolic diameter (LVEDD), left ventricular end-diastolic volume (LVEDV), left ventricular end-systolic volume (LVESV), left ventricular ejection fraction (LVEF, 2-D Simpson's method), aortic velocity time integral (VTI), cardiac output (CO, = stroke volume × heart rate), the aortic pre-ejection interval (APEI), and pulmonic pre-ejection interval (PPEI). Aortic pre-ejection interval is the time from Q-wave onset on the electrocardiogram (ECG) to aortic valve opening as measured by echocardiography. Pulmonic pre-ejection interval is the time from Q-wave on the ECG to the pulmonic valve opening. All echocardiographic values were obtained from the average of measurements from six continuous normal cardiac beats.
Complications
All complications including implantation failure, device dislodgement, implantation-related infection, and death were documented.
Post-mortem
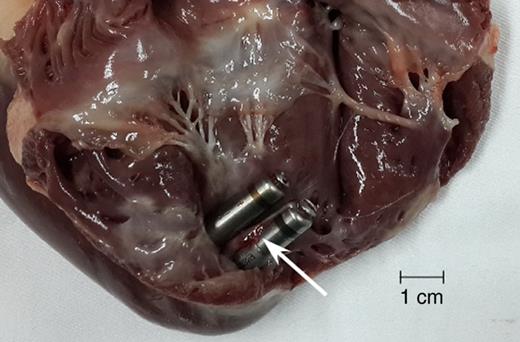
Fibrous tissue attaching around Micra devices observed at necropsy. Arrow indicates the fibrous tissue attachment.
Histology examinations of cardiac tissue
The Micra devices were removed from the heart, and then the implantation sites in the RV were excised transmurally. Samples of the myocardium in the right and left ventricles that were far away from the implantation sites were also collected as control in each heart. These samples were fixed in 10% neutral buffered formalin. Fixed tissue samples were sectioned transmurally (endocardium to epicardium) with steps of 200 µm intervals, and slides were stained with haematoxylin and eosin (HE) and Masson's trichrome stain for microscopic examination.
The tissue fibrosis surrounding the implantation sites were measured by a pathologist who was blinded to study design. Sections were analysed using a Q-Imaging Micro Publisher 5.0 RTV microscope camera (Q-Imaging, BC, Canada) on a microscope (Olympus BX51, Olympus Corporation, Japan) with a ×10 magnification objective. Tissue fibrosis was assessed with (i) the distance between the tip of the pacing electrode and the edge of tissue fibrosis, (ii) fibrosis area around the Micra device per slide view, and (iii) the fibrosis thickness around the tines of Micra devices.
Statistical analysis
Data collected in animals that survived the second device implantation were included in the statistical analysis. Data are presented as mean ± SD or percentage wherever applicable. A paired or unpaired t-test was used to compare numeral values. Longitudinal data were analysed with repeated measures ANOVA. A statistically significant difference required a probability of P < 0.05. All statistical analyses were conducted using statistical software package SPSS 19.0 (SPSS, Chicago, IL, USA).
Results
Implantation outcomes
Fourteen pigs underwent implantation procedures, of which 5 animals died prior to the end of the 6-month follow-up. The deaths of 4 animals were caused by procedure-related reasons, and 1 died due to chronic infection 139 days after the second Micra device implantation. The procedural-related complications included one acute device dislodgement, two atrial ruptures by the introducer, and one massive air embolism at the time of the second implantation.
Surgical procedure time and fluoroscopy time
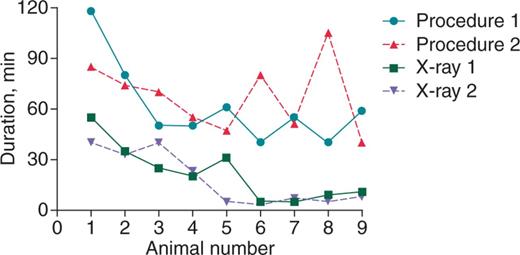
Procedure and fluoroscopy duration (minutes) of each animal. Four symbols and lines represent the time of procedure and fluoroscopy in the first and second implantations, respectively.
Assessment of cardiac function
Cardiac dimensions and functional measurements are summarized in Table 1. Comparing the 1- and 7-month measurements with baseline, there was no significant change in LVEF, VTI, and CO. Similarly, there was no significant change in left ventricular size in terms of LVESV, LVEDV, and LVEDD. The inter-ventricular mechanical delay was assessed by the difference between APEI and PPEI. The difference was 6.6 ± 3.7 ms at baseline, 7.3 ± 4.4 ms at the second implantation, and 9.6 ± 7.6 ms at the end of the study (P = 0.46 among three time points).
. | Implantation 1 . | Implantation 2 . | End . | P-value . |
---|---|---|---|---|
LVESV (mL) | 15.8 ± 8.3 | 13.4 ± 4.3 | 16.1 ± 5.0 | 0.14 |
LVEDV (mL) | 42.6 ± 10.2 | 43.8 ± 9.7 | 46.2 ± 7.8 | 0.73 |
LVEDD (mm) | 38.0 ± 2.9 | 38.0 ± 2.8 | 39.2 ± 4.9 | 0.70 |
LVEF (%) | 66.4 ± 5.1 | 69.7 ± 6.2 | 65.4 ± 7.4 | 0.39 |
VTI (cm) | 17.2 ± 3.2 | 16.9 ± 2.9 | 16.5 ± 2.8 | 0.89 |
CO (L) | 2.0 ± 0.4 | 2.1 ± 0.2 | 2.0 ± 0.2 | 0.91 |
. | Implantation 1 . | Implantation 2 . | End . | P-value . |
---|---|---|---|---|
LVESV (mL) | 15.8 ± 8.3 | 13.4 ± 4.3 | 16.1 ± 5.0 | 0.14 |
LVEDV (mL) | 42.6 ± 10.2 | 43.8 ± 9.7 | 46.2 ± 7.8 | 0.73 |
LVEDD (mm) | 38.0 ± 2.9 | 38.0 ± 2.8 | 39.2 ± 4.9 | 0.70 |
LVEF (%) | 66.4 ± 5.1 | 69.7 ± 6.2 | 65.4 ± 7.4 | 0.39 |
VTI (cm) | 17.2 ± 3.2 | 16.9 ± 2.9 | 16.5 ± 2.8 | 0.89 |
CO (L) | 2.0 ± 0.4 | 2.1 ± 0.2 | 2.0 ± 0.2 | 0.91 |
P-value: to test the time impact on the measurements at the first implant, the second implantation and the end of the study.
LVESV, left ventricular end-systolic volume; LVEDV, left ventricular end-diastolic volume; LVEDD, left ventricular end-diastolic diameter; LVEF, left ventricular ejection fraction; VTI, velocity time integral; CO, cardiac output.
. | Implantation 1 . | Implantation 2 . | End . | P-value . |
---|---|---|---|---|
LVESV (mL) | 15.8 ± 8.3 | 13.4 ± 4.3 | 16.1 ± 5.0 | 0.14 |
LVEDV (mL) | 42.6 ± 10.2 | 43.8 ± 9.7 | 46.2 ± 7.8 | 0.73 |
LVEDD (mm) | 38.0 ± 2.9 | 38.0 ± 2.8 | 39.2 ± 4.9 | 0.70 |
LVEF (%) | 66.4 ± 5.1 | 69.7 ± 6.2 | 65.4 ± 7.4 | 0.39 |
VTI (cm) | 17.2 ± 3.2 | 16.9 ± 2.9 | 16.5 ± 2.8 | 0.89 |
CO (L) | 2.0 ± 0.4 | 2.1 ± 0.2 | 2.0 ± 0.2 | 0.91 |
. | Implantation 1 . | Implantation 2 . | End . | P-value . |
---|---|---|---|---|
LVESV (mL) | 15.8 ± 8.3 | 13.4 ± 4.3 | 16.1 ± 5.0 | 0.14 |
LVEDV (mL) | 42.6 ± 10.2 | 43.8 ± 9.7 | 46.2 ± 7.8 | 0.73 |
LVEDD (mm) | 38.0 ± 2.9 | 38.0 ± 2.8 | 39.2 ± 4.9 | 0.70 |
LVEF (%) | 66.4 ± 5.1 | 69.7 ± 6.2 | 65.4 ± 7.4 | 0.39 |
VTI (cm) | 17.2 ± 3.2 | 16.9 ± 2.9 | 16.5 ± 2.8 | 0.89 |
CO (L) | 2.0 ± 0.4 | 2.1 ± 0.2 | 2.0 ± 0.2 | 0.91 |
P-value: to test the time impact on the measurements at the first implant, the second implantation and the end of the study.
LVESV, left ventricular end-systolic volume; LVEDV, left ventricular end-diastolic volume; LVEDD, left ventricular end-diastolic diameter; LVEF, left ventricular ejection fraction; VTI, velocity time integral; CO, cardiac output.
Necropsy
Nine animals reached the study endpoint with a mean follow-up time of 215 ± 7 days. The mean length of fibrous tissue covering the Micra devices was 14.3 ± 7.8 mm. There were no observations of tricuspid valve injury in any of the 9 animals (0.0%). Furthermore, the fixation tines of the Micra device could be seen from the epicardium for 6 implanted devices in 5 animals (33.3% of all implanted Micra devices).
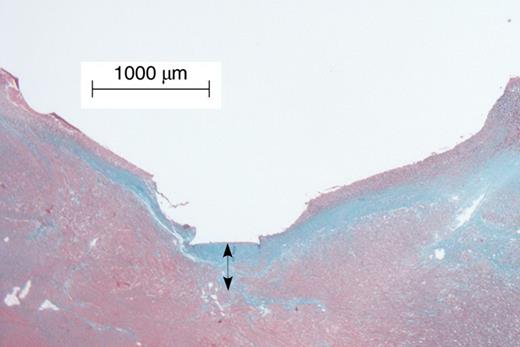
Tissue fibrosis beneath the Micra pacing electrode. Arrow indicates the thickness of the fibrosis measured from the endocardium where the device was placed.
Discussion
The present study with animals chronically implanted with two Micra leadless pacemakers showed no significant changes in cardiac dimension and function in terms of echocardiographic parameters LVEDV, LVESV, LVEDD, LVEF, VTI, CO, and RV-LV synchrony. There was no observation of injury to the tricuspid valve. Additionally, the fluoroscopy time was significantly shortened as the implanters gained experience with the procedure. Histological examination showed tissue fibrosis surrounding the fixation tines of the Micra device. Overall, the present study demonstrates the feasibility of multiple Micra devices implanted in the RV.
Transcatheter leadless pacemakers like the Micra device have emerged as a result of technology advancement in the fields of electronic circuit, battery, power management, materials, miniature packaging, communication, and biocompatibility. There are several clinical advantages in implanting a small pacing device inside the ventricle for the treatment of bradycardia when compared with conventional pacemakers with transvenous leads. The transcatheter pacemaker without a transvenous lead and subcutaneous device pocket can avoid the complications such as lead dislodgement and fracture, and lead/device-related infection that have been frequently observed clinically.1–11
The longevity of conventional pacemakers in today's clinical practice varies from 8 to 10 years depending on different manufacturers and pattern of device service (programming). The average age of patients implanted with a pacemaker is 75 years and will survive on average 12 years.17,18 Therefore, many patients who receive their pacemaker will need a second pacemaker. Transcatheter pacemakers also have an estimated longevity of 10 years. Once the end of the device life approaches, there are two possibilities for the replacement of Micra device. The first is to implant another transcatheter pacemaker adjacent to the first one leaving the first one in place. To allow this approach, the Micra pacemaker has an off mode in which it cannot turn itself on under any circumstances. As shown in this study, placing two transcatheter pacemakers in the ventricle does not impact cardiac function. The second possibility is to extract the first transcatheter pacemaker and then implant a second one. It is expected that after 10 years, the device will be completely encapsulated making extraction more difficult. While it is possible to retrieve a transcatheter pacemaker before it is encapsulated,19 it is likely attempting to extract a completely encapsulated device would create additional risk to the patient. Because of this uncertainty around transcatheter pacemaker extraction, it seems prudent to leave the first transcatheter pacemaker and implant a second device next to it.
The size of these pig hearts was significantly smaller than the typical human heart. The LVEDV in adult humans ranges from 58 to 120 mL20 compared with the LVEDV between 40 and 50 mL for the pigs in this study. Therefore, the impact of the devices in the hearts of these pigs is likely stronger than what would be seen in humans. The fact that no difference in cardiac function was found in these smaller animals indicates that there is even less chance of an impact of multiple devices in the larger human hearts.
There were a number of safety findings in this study. Firstly, there was no case of tricuspid valve injury observed in the present study. The tricuspid valve injury incidence has been reported for pacing and defibrillation leads, which may lead to the development of right ventricular heart failure clinically.21 Secondly, no large fibrous tissues were found around any of the Micra devices, which have been reported before around pacing/defibrillation leads. Therefore, the impact of fibrous attachment around the Micra device on cardiac contraction may be minimal. Additionally, the fluoroscope time in the present study significantly decreased below 10 min once implanters mastered the implantation techniques after few cases of implantation.
In the present study, Micra tines were visible from the epicardial surface in some of the animals. This was probably due in part to the fact that the pig hearts have no trabeculae, so the devices were implanted deep in the apex. Penetration into the pericardial space by helixes is a known phenomenon with helix electrodes, and rarely requires any further intervention clinically.22 Thus, it is expected that the tine penetrations will have similarly few consequences.
The present study demonstrates that (i) it is technically feasible to implant two sequential Micra devices in the RV, and (ii) there are no significant changes in cardiac function in terms of LVEF, VTI, CO, and RV-LV synchrony assessed by echocardiography. Thus, the results of the present study demonstrate that multiple transcatheter pacemakers can be implanted in the RV with no negative impact on cardiac function.
Limitations
While the present study was a chronic observation in cardiac function, the follow-up period of 6 months is limited when compared with the device longevity of 10 years at which the second implantation is required. Thus, the present study cannot provide the long-term information regarding cardiac function and adverse events over 6 months. Furthermore, the implantation procedure of Micra devices used in the present study is different from that used in humans, especially the route of vein access. The present study used the jugular venous access, while femoral venous access is used clinically. This delivery route created some procedure-related complications in the study, which will not likely be seen using the femoral route. Moreover, the main objective of cardiac functional assessment following multiple Micra device implantations in the present study would not be affected by the measurement of Micra device electrical parameters because no pacing therapy was provided in the present study.
Conclusions
The present study demonstrates the feasibility of implanting multiple Micra devices in the RV without a negative impact on cardiac function in a swine model. Considering the uncertainty of the tool for extraction of Micra devices in the future, it is possible to implant two or more than two Micra devices in the same ventricle once the end of life of the first implanted Micra device approaches in clinical practice.
Funding
The study was sponsored by a research grant from CRHF Division, Medtronic, plc.
Conflict of interest: X.Z. and M.B. are employees of Medtronic that makes pacemaker products.
Acknowledgements
The authors would like to thank Lepeng Zeng and Tiger Wang of Medtronic China (Shanghai) for their help in coordinating and monitoring the study.
References
Author notes
†The first two authors contributed equally to this study.
- aorta
- artificial cardiac pacemaker
- left ventricular ejection fraction
- echocardiography
- bradycardia
- cardiac output
- tricuspid valve
- left ventricle
- right ventricle
- autopsy
- follow-up
- surgical procedures, operative
- suidae
- infections
- heart
- heart valve injury
- cardiac function
- medical devices
- implantation procedure
- implants