-
PDF
- Split View
-
Views
-
Cite
Cite
Georg Nölker, Klaus-Jürgen Gutleben, Stefan Asbach, Guido Ritscher, Harald Marschang, Anil M. Sinha, Peter Boileau, Michael E. Benser, Benoit Hallier, Alexander Hümmer, Johannes Brachmann, Dynamic ventricular overdrive stimulation in atrial fibrillation: effects on ventricular rate irregularity, ventricular pacing, and fusion beats, EP Europace, Volume 13, Issue 12, December 2011, Pages 1688–1694, https://doi.org/10.1093/europace/eur243
- Share Icon Share
Abstract
In pacemaker patients with preserved atrio-ventricular (AV) conduction, atrial fibrillation (AF) can lead to symptomatic ventricular rate irregularity and loss of ventricular stimulation. We tested if dynamic ventricular overdrive (DVO) as a potentially pacemaker-integrated algorithm could improve both aspects.
Different settings of DVO and ventricular–ventricular-inhibited-pacing (VVI) with different base rates were tested in two consecutive phases during electrophysiological studies for standard indications. Mean heart rate (HR), HR irregularity and percentage of ventricular pacing were evaluated. A fusion index (FI) indicative of the proportion of fusion beats was calculated for each stimulation protocol. Dynamic ventricular overdrive from the right ventricular apex was acutely applied in 38 patients (11 females, mean age 62.1 ± 11.5 years) with sustained AF and preserved AV conduction. Dynamic ventricular overdrive at LOW/MEDIUM setting increased the amount of ventricular pacing compared with VVI pacing at 60, 70, and 80 beats per minute (bpm; to 81/85% from 11, 25, and 47%, respectively; P< 0.05). It also resulted in a maximum decrease in interval differences (to 48 ± 18 ms from 149 ± 28, 117 ± 38, and 95 ± 46 ms, respectively; P< 0.05) and fusion (to 0.13 from 0.41, 0.42, and 0.36, respectively; P< 0.05) compared with VVI pacing at 60, 70, and 80 bpm. However, the application of DVO resulted in a significant increase in HR compared with intrinsic rhythm and VVI pacing at 80 bpm (to 97 bpm from 89 and 94 bpm, respectively; P< 0.05).
Dynamic ventricular overdrive decreases HR irregularity and increases ventricular pacing rate compared with VVI pacing at fixed elevated base rates and spontaneous rhythm. Fusion index might help to refine information on pacing percentages provided by device counters.
Introduction
Atrial fibrillation (AF) is the most common arrhythmia in Europe and the USA, and increases with age. It is associated with significant morbidity and mortality, including a four- to fivefold increased risk of stroke, a tripling of risk of heart failure and a 40–90% increased risk of overall mortality.1–3
Atrial fibrillation is a frequent and challenging condition to manage in patients with implanted pacemakers, implantable cardioverter-defibrillators (ICDs) and devices for cardiac resynchronization therapy (CRT). Atrial fibrillation has been documented as a frequent comorbidity in paced patients. The annual incidences of AF and chronic AF after pacemaker implantation are at least 5 and 3%. Mean lifetime cumulative incidences of AF and chronic AF are estimated ∼30–40 and 20%, respectively.4 In the DAVID and DAVID II trials, ICD patients showed a high incidence of AF and atrial flutter in the baseline characteristics.5,6 Cardiac resynchronization therapy patients show a prevalence of AF of 19% at implant and a first-year new onset of 8%.7,8
Converging evidence suggests that the ventricular rhythm irregularity during AF adversely affects cardiac haemodynamics independently of the fast ventricular rate.9–12
In this study, we tested the capability of an investigational device-based dynamic ventricular overdrive (DVO) algorithm to provide ventricular rate regularization and effective ventricular pacing during AF in the acute clinical setting of patients with AF and preserved atrio-ventricular (AV) nodal conduction.
Methods
Study population
We tested DVO in patients with sustained AF and preserved AV nodal conduction undergoing electrophysiology study for standard indications. The study was conducted in two consecutives phases which were approved by a registered ethics committee. All patients gave their written informed consent.
Dynamic ventricular overdrive algorithm
Dynamic ventricular overdrive is a modified version of the AF Suppression™ (St Jude Medical, Sylmar, CA, USA) pacing algorithm applied to the right ventricle.
This algorithm continuously and automatically adapts its ventricular pacing rate according to intrinsic ventricular activity. Dynamic ventricular overdrive continuously monitors for R-waves. On detection of the second intrinsic beat among the prior 16, DVO increases its pacing rate. After a programmable number of cycles without rate increase, DVO initiates a gradual decrease in its pacing rate until the base rate is reached or until intrinsic activity triggers another rate increase. Dynamic ventricular overdrive-triggered pacing rate depends on the pacing rate at the time of the increase according to one of several functions or ‘slopes’ (Figure 1).
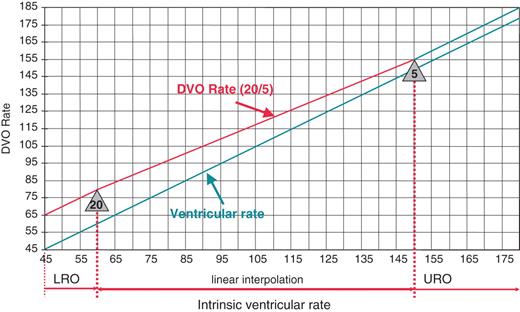
Example of the dynamic ventricular overdrive (DVO) rate behaviour for the slope HIGH. ‘Lower Rate Overdrive’ (LRO) is 20 bpm and ‘Upper Rate Overdrive’ (URO) is 5 bpm. In this example overdrive pacing is more aggressive when ventricular rate is lower and is continuously reduced until Upper Rate Overdrive is reached.
A slope is defined by a programmable lower and a programmable upper rate overdrive value. ‘Lower Rate Overdrive’ (LRO) is the programmed amount of rate increase in beats per minute (bpm) triggered by two intrinsic beats when the ventricular rate is 60 bpm or below. ‘Upper Rate Overdrive’ (URO) is the programmed amount of rate increase in bpm when the ventricular rate is ≥150 bpm. For rates in between 60 and 150 bpm, the rate increase is a function of rate which approximates a linear interpolation between LRO and URO. To prevent pacing at a high rate, a maximum DVO-triggered pacing rate is programmable.
Four slopes (LRO/URO) were evaluated in this study and will be referred to as LOW (5/5), MEDIUM (10/5), HIGH (20/5), and VERY HIGH (25/10) (Figure 1). The number of overdrive pacing cycles was set to 15 and the maximum DVO-triggered rate was programmed to 110 bpm.
Study conduct
Sensing and pacing were performed in ventricular–ventricular-inhibited (VVI) pacing mode via a conventional transvenous electrophysiology catheter placed at the apex of the right ventricle and connected to a pacing system analyser (Model 3150, St Jude Medical, Sylmar, CA, USA) capable of delivering all pacing regimens evaluated in this study. Pacing and sensing properties were programmed with appropriate safety margins to promote consistent ventricular sensing and pacing throughout the tests. A standard programmer (Model 3510, St Jude Medical, Sylmar, CA, USA) was configured to record surface electrocardiogram (ECG; lead I) and ventricular paced/sensed event markers during testing. Surface ECG and device marker data were downloaded in real-time from the programmer via ethernet to a battery-powered laptop computer and analysed offline with MATLAB® (The MathWorks Inc., Natick, MA, USA).
Study phase I
The first phase aimed at evaluating the effect of three DVO slopes (LOW, HIGH, and VERY HIGH) as compared with intrinsic rhythm and VVI pacing at 40 bpm. Each setting was applied in randomized order for 5min and separated by a period without pacing of at least 1min to allow recovery and re-initialization of the algorithm to base rate pacing. The last 4min of each recording were analysed.
Study phase II
In order to minimize the impact of DVO on ventricular rate increase and to compare it with alternative elevated base rate pacing, the protocol was amended and the second phase was conducted. The purpose of this phase was to compare the effects of DVO slopes (LOW and MEDIUM) to intrinsic rhythm and VVI base rate pacing at 60, 70, and 80 bpm. Each configuration was applied in randomized order and separated by a rest period without pacing of at least one minute. As results remained constant throughout the last 4 min of recordings in the first phase and to prevent any extension of the test procedure, the recording duration was decreased to 3min and the last 2min were analysed.
Data processing and statistical analysis
Ventricular activation events were identified offline on the surface ECG recordings using a dynamic thresholding algorithm after narrow bandpass filtering and were manually adjudicated by a clinical engineer using the morphology of non-filtered associated complexes and the marker data. Intervals were measured between adjudicated detections (Figure 2).
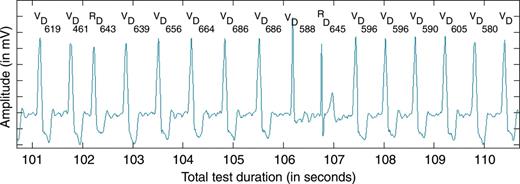
Typical 10 s segment of electrocardiogram and markers during the application of dynamic ventricular overdrive. The paced rate decreases gradually until the second R-wave triggers a new rate increase. V, ventricular pace marker; R, ventricular sense marker; D, event detected in post-processing; each number represents the intervals in milliseconds between two events detected in post-processing.
Based on the resulting R–R interval series and marker data, the mean heart rate (HR), the coefficient of variation of intervals (COV), the mean difference of consecutive intervals (ID), and percentage of ventricular pacing were calculated for each test configuration.
In order to evaluate the proportion of fusion beats, a new fusion index (FI) was developed and calculated for each setting. It was defined as the ratio between the number of paced beats with an intrinsic-like morphology on the lead I surface ECG and the total number of paced beats. Calculation of the fusion index is detailed and illustrated in Figure 3.
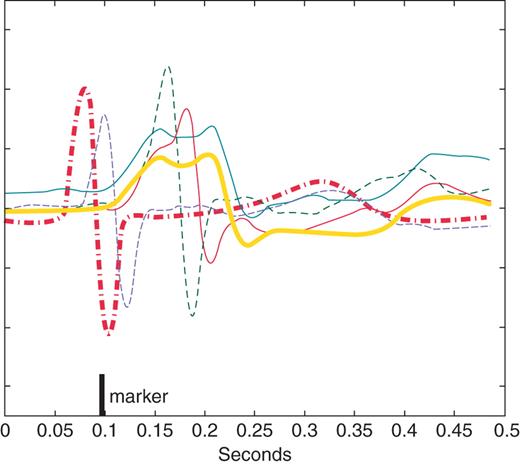
Example of calculation of the fusion index on four paced complexes (thin lines). To identify beats with intrinsic-like morphology, the average of all intrinsic complexes (thick red dashed line) and the average of all paced complexes (thick black solid line) were constructed for each pacing intervention. An amplitude-normalized cross-correlation with the intrinsic average and with the paced average was then calculated for each paced complex. Each complex having a larger peak correlation with the intrinsic average than with the paced average was deemed to have intrinsic-like morphology and scored as a fusion beat (thin dashed lines). The fusion index represents the proportion of fusion beats to the total number of paced beats.
Results are expressed as mean ± standard deviation in a per protocol analysis. Patient characteristics were compared using the χ2 test with Yates' correction except for age, which was compared using the Wilcoxon rank-sum test. A one-sided analysis of variance test for repeated measures was performed for each measure calculated in each phase. For each measure exhibiting a significant difference across the test settings to a level of P< 0.05, further comparisons were made according to the Dunnett or Tukey method. In phase I, significance tests according to the Dunnett method compared the three DVO settings to intrinsic rhythm, which served as a control. The Dunnett method also compared the three DVO settings to pacing at 40 bpm which served as an alternate control. The Tukey method compared the three DVO settings against each other. In phase II, the DVO settings served as controls to which the Dunnett method compared the three fixed rate pacing interventions. The Tukey method compared the three fixed rate pacing interventions to each other. Comparisons for which zero lay outside of the 95% confidence interval were considered statistically significant (P< 0.05).
Results
Patient characteristics
There were no significant differences between the patient populations of both phases. The patient characteristics are detailed in Table 1.
. | Phase I (n= 18) . | Phase II (n= 20) . |
---|---|---|
Age (years) | 62.4 ± 10 | 61.9 ± 13 |
Gender (male/female) (%) | 76/24 | 65/35 |
Drugs (%) | ||
Beta-blockers | 59 | 65 |
Cardiac glycosides | 35 | 25 |
Other antiarrhythmic drugs | 12 | 25 |
Type of atrial fibrillation (%) | ||
Paroxysmal | 6 | 15 |
Persistent | 35 | 40 |
Long-standing persistent | 59 | 45 |
. | Phase I (n= 18) . | Phase II (n= 20) . |
---|---|---|
Age (years) | 62.4 ± 10 | 61.9 ± 13 |
Gender (male/female) (%) | 76/24 | 65/35 |
Drugs (%) | ||
Beta-blockers | 59 | 65 |
Cardiac glycosides | 35 | 25 |
Other antiarrhythmic drugs | 12 | 25 |
Type of atrial fibrillation (%) | ||
Paroxysmal | 6 | 15 |
Persistent | 35 | 40 |
Long-standing persistent | 59 | 45 |
. | Phase I (n= 18) . | Phase II (n= 20) . |
---|---|---|
Age (years) | 62.4 ± 10 | 61.9 ± 13 |
Gender (male/female) (%) | 76/24 | 65/35 |
Drugs (%) | ||
Beta-blockers | 59 | 65 |
Cardiac glycosides | 35 | 25 |
Other antiarrhythmic drugs | 12 | 25 |
Type of atrial fibrillation (%) | ||
Paroxysmal | 6 | 15 |
Persistent | 35 | 40 |
Long-standing persistent | 59 | 45 |
. | Phase I (n= 18) . | Phase II (n= 20) . |
---|---|---|
Age (years) | 62.4 ± 10 | 61.9 ± 13 |
Gender (male/female) (%) | 76/24 | 65/35 |
Drugs (%) | ||
Beta-blockers | 59 | 65 |
Cardiac glycosides | 35 | 25 |
Other antiarrhythmic drugs | 12 | 25 |
Type of atrial fibrillation (%) | ||
Paroxysmal | 6 | 15 |
Persistent | 35 | 40 |
Long-standing persistent | 59 | 45 |
Phase I
Eighteen patients were enrolled in this phase. All five recordings from one patient with an unusually high intrinsic ventricular rate (120 bpm) were excluded from analysis because the pacemaker's refractory period was not appropriately programmed to accommodate this high rate and competitive pacing resulted. Nine recordings from four of the remaining 17 patients were excluded from the analysis due to t-wave double-counting (3), loss of capture (4), and data acquisition malfunction (2). Table 2 presents the results for each test condition in phase I. Ventricular rate irregularity was significantly reduced when DVO (LOW, HIGH, or VERY HIGH) was applied compared with each control setting (P< 0.05). Dynamic ventricular overdrive also significantly increased the mean HR compared with each control setting (P< 0.05). Comparing the different DVO settings, all results except for those for COV were significantly different in the LOW setting compared with the two other settings (P< 0.05). Coefficient of variation only varied significantly for the VERY HIGH vs. the LOW setting. No result was significantly different for the HIGH vs. VERY HIGH settings.
. | No pacing . | 40 bpm . | LOW . | HIGH . | VERY HIGH . |
---|---|---|---|---|---|
HR (bpm) | 75 ± 19* | 76 ± 18* | 84 ± 18** | 90 ± 16 | 90 ± 15 |
COV | 0.23 ± 0.06* | 0.24 ± 0.06* | 0.13 ± 0.05*** | 0.14 ± 0.04 | 0.15 ± 0.06 |
ID (ms) | 225 ± 103* | 218 ± 91* | 73 ± 27** | 52 ± 20 | 57 ± 32 |
%Vp | – | 6 ± 13* | 83 ± 5** | 91 ± 5 | 91 ± 7 |
Fusion index | – | a | 0.14 ± 0.11** | 0.09 ± 0.10 | 0.07 ± 0.11 |
. | No pacing . | 40 bpm . | LOW . | HIGH . | VERY HIGH . |
---|---|---|---|---|---|
HR (bpm) | 75 ± 19* | 76 ± 18* | 84 ± 18** | 90 ± 16 | 90 ± 15 |
COV | 0.23 ± 0.06* | 0.24 ± 0.06* | 0.13 ± 0.05*** | 0.14 ± 0.04 | 0.15 ± 0.06 |
ID (ms) | 225 ± 103* | 218 ± 91* | 73 ± 27** | 52 ± 20 | 57 ± 32 |
%Vp | – | 6 ± 13* | 83 ± 5** | 91 ± 5 | 91 ± 7 |
Fusion index | – | a | 0.14 ± 0.11** | 0.09 ± 0.10 | 0.07 ± 0.11 |
HR, mean heart rate; COV, coefficient of variation; ID, mean interval difference of consecutive beats; %Vp, percentage of ventricular pacing.
*P < 0.05 vs. LOW, HIGH, and VERY HIGH (Dunnett method).
**P< 0.05 vs. HIGH and VERY HIGH (Tukey method).
***P< 0.05 vs. VERY HIGH (Tukey method).
aDue to minimal pacing, fusion index is not presented.
. | No pacing . | 40 bpm . | LOW . | HIGH . | VERY HIGH . |
---|---|---|---|---|---|
HR (bpm) | 75 ± 19* | 76 ± 18* | 84 ± 18** | 90 ± 16 | 90 ± 15 |
COV | 0.23 ± 0.06* | 0.24 ± 0.06* | 0.13 ± 0.05*** | 0.14 ± 0.04 | 0.15 ± 0.06 |
ID (ms) | 225 ± 103* | 218 ± 91* | 73 ± 27** | 52 ± 20 | 57 ± 32 |
%Vp | – | 6 ± 13* | 83 ± 5** | 91 ± 5 | 91 ± 7 |
Fusion index | – | a | 0.14 ± 0.11** | 0.09 ± 0.10 | 0.07 ± 0.11 |
. | No pacing . | 40 bpm . | LOW . | HIGH . | VERY HIGH . |
---|---|---|---|---|---|
HR (bpm) | 75 ± 19* | 76 ± 18* | 84 ± 18** | 90 ± 16 | 90 ± 15 |
COV | 0.23 ± 0.06* | 0.24 ± 0.06* | 0.13 ± 0.05*** | 0.14 ± 0.04 | 0.15 ± 0.06 |
ID (ms) | 225 ± 103* | 218 ± 91* | 73 ± 27** | 52 ± 20 | 57 ± 32 |
%Vp | – | 6 ± 13* | 83 ± 5** | 91 ± 5 | 91 ± 7 |
Fusion index | – | a | 0.14 ± 0.11** | 0.09 ± 0.10 | 0.07 ± 0.11 |
HR, mean heart rate; COV, coefficient of variation; ID, mean interval difference of consecutive beats; %Vp, percentage of ventricular pacing.
*P < 0.05 vs. LOW, HIGH, and VERY HIGH (Dunnett method).
**P< 0.05 vs. HIGH and VERY HIGH (Tukey method).
***P< 0.05 vs. VERY HIGH (Tukey method).
aDue to minimal pacing, fusion index is not presented.
Phase II
Twenty patients were enrolled in phase II. Eight recordings from five patients were excluded from the analysis for undersensing and loss of capture (n= 4), and data acquisition malfunction (n= 4). Additionally, three records from two patients contributed no data to the fusion index analysis due to a lack of paced beats.
Table 3 presents the results for each test condition in phase II. Mean HR, percentage of ventricular pacing and fusion index were significantly affected when DVO (LOW or MEDIUM) was applied compared with intrinsic rhythm and each control setting in VVI pacing (P< 0.05). Heart rate irregularity was also significantly decreased with DVO settings except for the COV compared VVI pacing at 80 bpm. There were no significant differences when comparing LOW and MEDIUM settings. Comparing the control settings in VVI pacing, HR was significantly higher for 80 vs. 60 bpm but not for 80 vs. 70 bpm or for 70 vs. 60 bpm. Coefficient of variation decreased with each increase in VVI pacing rate. Interval difference was smaller for fixed-rate pacing at 70 and 80 vs. 60 bpm but not different between 70 vs. 80 bpm. Percentage of ventricular pacing increased with each increase in fixed rate VVI pacing. Fusion index was not changed between the fixed rate VVI pacing interventions.
. | No pacing . | 60 bpm . | 70 bpm . | 80 bpm . | LOW . | MEDIUM . |
---|---|---|---|---|---|---|
HR (bpm) | 89 ± 17 | 90 ± 15*** | 92 ± 16 | 94 ± 14 | 97 ± 16* | 100 ± 16* |
COV | 0.23 ± 0.06 | 0.21 ± 0.04**** | 0.18 ± 0.06***** | 0.15 ± 0.07 | 0.12 ± 0.03** | 0.12 ± 0.04** |
ID (ms) | 171 ± 59 | 149 ± 28**** | 117 ± 38 | 95 ± 46 | 55 ± 20* | 48 ± 18* |
%Vp | 11 ± 15**** | 25 ± 29***** | 47 ± 34 | 81 ± 5* | 85 ± 6* | |
Fusion index | 0.41 ± 0.29 | 0.42 ± 0.27 | 0.36 ± 0.23 | 0.13 ± 0.05* | 0.13 ± 0.10* |
. | No pacing . | 60 bpm . | 70 bpm . | 80 bpm . | LOW . | MEDIUM . |
---|---|---|---|---|---|---|
HR (bpm) | 89 ± 17 | 90 ± 15*** | 92 ± 16 | 94 ± 14 | 97 ± 16* | 100 ± 16* |
COV | 0.23 ± 0.06 | 0.21 ± 0.04**** | 0.18 ± 0.06***** | 0.15 ± 0.07 | 0.12 ± 0.03** | 0.12 ± 0.04** |
ID (ms) | 171 ± 59 | 149 ± 28**** | 117 ± 38 | 95 ± 46 | 55 ± 20* | 48 ± 18* |
%Vp | 11 ± 15**** | 25 ± 29***** | 47 ± 34 | 81 ± 5* | 85 ± 6* | |
Fusion index | 0.41 ± 0.29 | 0.42 ± 0.27 | 0.36 ± 0.23 | 0.13 ± 0.05* | 0.13 ± 0.10* |
HR, mean heart rate; COV, coefficient of variation; ID, mean interval difference of consecutive beats; %Vp, percentage of ventricular pacing.
*P< 0.05 vs. no pacing, 60, 70 and 80 bpm. (Dunnett method). **P< 0.05 vs. no pacing, 60 and 70 bpm. (Dunnett method).
***P< 0.05 vs. 80 bpm (Tukey method).
****P< 0.05 vs. 70 and 80 bpm (Tukey method).
*****P< 0.05 vs. 60 and 80 bpm (Tukey method).
. | No pacing . | 60 bpm . | 70 bpm . | 80 bpm . | LOW . | MEDIUM . |
---|---|---|---|---|---|---|
HR (bpm) | 89 ± 17 | 90 ± 15*** | 92 ± 16 | 94 ± 14 | 97 ± 16* | 100 ± 16* |
COV | 0.23 ± 0.06 | 0.21 ± 0.04**** | 0.18 ± 0.06***** | 0.15 ± 0.07 | 0.12 ± 0.03** | 0.12 ± 0.04** |
ID (ms) | 171 ± 59 | 149 ± 28**** | 117 ± 38 | 95 ± 46 | 55 ± 20* | 48 ± 18* |
%Vp | 11 ± 15**** | 25 ± 29***** | 47 ± 34 | 81 ± 5* | 85 ± 6* | |
Fusion index | 0.41 ± 0.29 | 0.42 ± 0.27 | 0.36 ± 0.23 | 0.13 ± 0.05* | 0.13 ± 0.10* |
. | No pacing . | 60 bpm . | 70 bpm . | 80 bpm . | LOW . | MEDIUM . |
---|---|---|---|---|---|---|
HR (bpm) | 89 ± 17 | 90 ± 15*** | 92 ± 16 | 94 ± 14 | 97 ± 16* | 100 ± 16* |
COV | 0.23 ± 0.06 | 0.21 ± 0.04**** | 0.18 ± 0.06***** | 0.15 ± 0.07 | 0.12 ± 0.03** | 0.12 ± 0.04** |
ID (ms) | 171 ± 59 | 149 ± 28**** | 117 ± 38 | 95 ± 46 | 55 ± 20* | 48 ± 18* |
%Vp | 11 ± 15**** | 25 ± 29***** | 47 ± 34 | 81 ± 5* | 85 ± 6* | |
Fusion index | 0.41 ± 0.29 | 0.42 ± 0.27 | 0.36 ± 0.23 | 0.13 ± 0.05* | 0.13 ± 0.10* |
HR, mean heart rate; COV, coefficient of variation; ID, mean interval difference of consecutive beats; %Vp, percentage of ventricular pacing.
*P< 0.05 vs. no pacing, 60, 70 and 80 bpm. (Dunnett method). **P< 0.05 vs. no pacing, 60 and 70 bpm. (Dunnett method).
***P< 0.05 vs. 80 bpm (Tukey method).
****P< 0.05 vs. 70 and 80 bpm (Tukey method).
*****P< 0.05 vs. 60 and 80 bpm (Tukey method).
Discussion
Main findings
DVO effectively increased the amount of ventricular pacing compared with VVI pacing at different levels and intrinsic rhythm. Moreover, it resulted in a significant decrease in rate irregularity and fusion compared with VVI pacing and to intrinsic rhythm. However, the application of DVO led to a significant increase in HR compared with intrinsic rhythm and VVI pacing at a fixed base rate. Fusion index was established as a novel tool to evaluate the proportion of fusion beats among stimulated QRS-complexes.
Effects of DVO on heart rate regularization
Rate irregularity due to AF may compromise haemodynamics and may be a source of discomfort for the patient due to palpitations.13 Rate regularization treats palpitations and may economize cardiac function. However, an increase in HRs may have detrimental effects on cardiac haemodynamics, especially if it is applied to patients with reduced ejection fractions.5 Furthermore, right ventricular pacing itself may negatively impact cardiac function.5 Therefore, rate regularizing at elevated pacing levels might have positive and negative consequences in right ventricular paced patients. This hypothesis is substantiated by a recent study of Tse et al.14 describing positive long-term effects of a different ventricular rate regularization algorithm on exercise time, metabolic equivalents, and peak oxygen capacity in patients with implanted VVI pacemakers suffering from permanent AF. Although long-term effects on rate regularization and peak ventricular rates were similar between their two groups, Tse et al. found improvements of cardiac function only in patients stimulated at septal pacing sites but not in patients stimulated at right ventricular apical sites. In our cohort a right ventricular apical pacing site was chosen to study an overdrive pacing algorithm primarily designed for an atrial application. It was proven that application of this algorithm at rest leads to a decrease in rate irregularity compared with both VVI pacing at fixed rates and to intrinsic rhythm. This is similar to what Tse et al. found under exercise conditions.
However, the impact of a decrease in rate irregularity on patients' symptoms was not tested in our study and may be low even in pacemaker patients as described by Sulke et al.15 Moreover, at least paroxysmal AF may be associated with symptoms in particular when it is preceded by higher frequencies as shown by Silberbauer et al.16 Therefore, DVO may have the potential to increase symptoms in patients suffering from paroxysmal AF.
Lian et al.17 quantitatively reported the effect of several ventricular rate stabilization algorithms using a computer model of the ventricular response to AF with ventricular pacing. The authors showed that elevated base rate pacing would not provide a reliable solution to regularize HR in patients with high intrinsic rates and find much better effectiveness on this parameter in ventricular rate smoothing algorithms. This is well in the line with our findings in a univentricular pacing protocol. In our study, pacing at elevated base rates lead to a decrease of rate irregularity represented by the COV and the ID comparable with DVO only when the highest tested base rate of 80 bpm was applied. Dynamic ventricular overdrive achieved significant HR regularization and a high percentage of ventricular pacing of 81–91% depending on the DVO level.
Effects of DVO on heart rates
Increased ventricular pacing, reduced fusion index, and improved rate regularity have been achieved by DVO at the expense of a significant and consistent increase in the mean HR. Although the optimization of the settings during the study allowed us to reduce this effect, DVO has significant impact on mean HR. However, whether this rate increase is tolerable or not is questionable. In particular, a recent study suggests that the benefit of strict HR control compared with lenient HR control in patients suffering from AF has been overestimated.18
The increase in HR compared with intrinsic rhythm and VVI pacing at rest is similar to what Tse et al.13 found under exercise conditions in their long-term study. Even though elevated pacing rates are likely to raise cardiac oxygen consumption they found no significant decreases in exercise time, peak oxygen consumption, and metabolic equivalents in a setting comparable with ours applying right ventricular apical pacing.
Relatively rapid pacing may be advantageous in terms of increased cardiac output in RV-paced patients. Disadvantages of more rapid pacing may include decreased battery longevity and increased metabolic demand especially when it leads to ventricular desynchronization.
In patients undergoing CRT biventricular pacing is likely to compensate for desynchronization. Beyond that advantages and disadvantages of pacing at higher rates will be more or less the same as in RV-paced patients. Whereas optimal cardiac output has been found at rates between 70 and 90 bpm,19 it has not been finally answered whether long term programming of lower rates in this range is favourable in patients with congestive heart failure. However, Tse et al.20 found that the use of rate-adaptive pacing with CRT in patients suffering from chronotropic incompetence provides incremental benefit on exercise capacity in a DDDR pacing mode. Compared with their protocol using a lower rate limit of 50 bpm and an upper rate limit of 140 bpm, mean HRs of 90–100 bpm as found in our patients undergoing the most aggressive DVO-level seem to be acceptable.
Role of fusion in ventricular pacing
In addition to the percentage of ventricular pacing, the amount of fusion beats is also an important parameter to monitor during AF especially in CRT patients. Kamath et al.21 recently reported that pacing counters clearly overestimate the degree of effective biventricular pacing and that fusion beats are a major contributor to this overestimation. During conducted AF, pacing efficacy can be significantly compromised by transient or permanent loss of stimulation or fusion beats.22 An obvious prerequisite for effective CRT is biventricular stimulation being delivered a sufficient proportion of the time but the appropriate dose of biventricular pacing below which the CRT therapy effects are jeopardized is still under discussion.21,23 Atrial fibrillation in CRT patients with preserved AV nodal conduction can jeopardize optimal cardiac resynchronization through transient or permanent loss of stimulation and an increased proportion of fusion beats. A study by Gasparini et al.22 was the first to describe a therapeutic strategy using a cut-off value at 85%, as indicated by implantable device diagnostics. Kamath et al.21 reported an average of 86.4% effective pacing in responders to CRT vs. 66.8% in non-responders, using a 12 lead Holter monitoring system. Recently, Koplan et al.23 retrospectively evaluated the effect of the amount of biventricular pacing in a cohort of 1812 patients implanted with CRT devices followed for 1 year after implantation. The authors concluded that an overall percentage of biventricular-paced beats >92% resulted in a 44% reduction risk on a combined endpoint of heart failure hospitalization and all-cause mortality. Furthermore, this post-hoc analysis confirmed that atrial arrhythmias are a significant contributor to the decrease of biventricular pacing percentage. Our study shows that DVO can provide a high amount of effective biventricular pacing during AF and therefore could be a means to achieve a sufficient dose of biventricular pacing during atrial arrhythmias if integrated in a biventricular device. Integration of the algorithm into biventricular stimulation may also compensate for detrimental effects of right ventricular apical pacing on cardiac function.
In order to evaluate the proportion of purely paced beats, we developed a new fusion index based on ECG morphology correlation. Based on this index, DVO achieves a significant increase of effective biventricular pacing as compared with a fixed-rate pacing at elevated base rate. To our knowledge, this is the first study evaluating the fusion beats during the application of device-based algorithms to increase the percentage of ventricular pacing.
Study limitations
This study was conducted at rest and in a supine position as an acute evaluation. Therefore, performance at exercise and effects of autonomic or catecholaminergic influences were not evaluated. The number of patients was limited and further reduced due to some limitations with data collection. It tested only right ventricular pacing and the haemodynamic impact of this algorithm was not evaluated.
Conclusions
Dynamic ventricular overdrive is a helpful tool to regularize heart rhythm in patients suffering from AF and this could represent a potential benefit in dual-chamber pacemaker patients suffering from paroxysmal AF. Additionally, DVO allows for a high percentage of stimulation in patients with preserved AV conduction associated with a low incidence of fusion beats. Such therapy could also represent an option for assuring effective biventricular stimulation during AF in CRT patients. Therefore, DVO is a promising algorithm for biventricular stimulation and should be further tested in this setting.
Conflict of interest: P.B., M.E.B., B.H., and A.H. are employees of St Jude Medical.
Funding
This study was financially supported by St. Jude Medical.