-
PDF
- Split View
-
Views
-
Cite
Cite
Alex Bittner, Gerold Mönnig, Ann Janine Vagt, Stephan Zellerhoff, Kristina Wasmer, Julia Köbe, Christian Pott, Peter Milberg, Cristina Sauerland, Johannes Wessling, Lars Eckardt, Pulmonary vein variants predispose to atrial fibrillation: a case-control study using multislice contrast-enhanced computed tomography, EP Europace, Volume 13, Issue 10, October 2011, Pages 1394–1400, https://doi.org/10.1093/europace/eur145
- Share Icon Share
Abstract
Pulmonary veins (PV) play a pivotal role in atrial fibrillation (AF). Anatomical variants of PV have been described and related to a higher arrhythmogenic potential. The aim of this study was to compare the prevalence of PV variants and diameters of PV ostia in AF patients and controls.
Variants of PV were defined as right or left common ostia (RCO, LCO), a right middle or right top PV . A long common trunk (LCT) was defined as an LCO with a distance to the first branching ≥10 mm. Multislice contrast-enhanced thoracic computed tomography was performed prior to AF ablation in 166 consecutive patients, 47.6% with paroxysmal, 52.4% with persistent AF, as well as in a sex- and age-matched control group without AF, for non-cardiological indications. Images were evaluated by two independent observers. The mean age was 59 ± 10 years, 108 were men (65.1%). A higher prevalence of LCO was found in the AF group: 33.7 vs. 19.9% (P= 0.004), odds ratio (OR) 2.1; 15.4% in patients vs. 10.2% in controls had an LCT (P= 0.14). No differences in other PV variants were found. The ostial diameters were greater in AF-patients (P< 0.001).
To the best of our knowledge, the present study shows for the first time a higher prevalence of an LCO in patients with AF as compared with controls, with an OR of 2.1. This suggests a pre-disposing role of LCO in the development of AF.
Introduction
Pulmonary veins (PV) play a pivotal role as triggers of atrial fibrillation (AF).1 Together with the antral portions of the atria, they are also important for maintenance of AF.2 Current catheter ablation strategies include PV isolation as the unique or starting point for treatment of paroxysmal, as well as persistent AF.3 Theoretically, anatomy of PV ostia could be relevant as a pre-disposing factor for the initiation or maintenance of AF, since myocardial sleeve distribution could condition conduction properties. Numerous imaging studies with computed tomography and magnetic resonance tomography (MRT) have described anatomical variants in PV anatomy among patients with AF in 18–45%,4–7 including common ostia and additional veins. Populations without AF have also been reported to show a proportion of PV variants of ∼30%.8,9 The great variability in the published incidence of such anatomical variations can at least in part be explained by variability in definitions of normality, and methodological differences. Few studies have directly compared anatomical characteristics in patients with and without AF, and have reported higher volumes of the left atria and greater PV ostial diameters in patients with AF. These studies, however, have not been designed to compare prevalence of PV variants and are therefore underpowered to answer that question.10,11 In lack of definite evidence, we decided to perform an imaging study with thorax contrast-enhanced multislice computed tomography (TCT), comparing the presence of PV variants and its anatomical characteristics in patients with and without AF.
Patients and methods
We performed a case-control study between two patient populations, with and without AF. We included a study group of 166 consecutive patients with paroxysmal, persistent, or longstanding persistent AF, in whom a TCT was performed prior to a first procedure of AF ablation. Exclusion criteria were advanced structural heart disease, defined as moderate-to-severe left ventricular dysfunction, with a left ventricular ejection fraction <40%, moderate, or severe mitral valve dysfunction, surgically corrected valvular heart disease, severe aortic valve stenosis or regurgitation, congenital heart disease, and hypertrophic or restrictive cardiomyopathy. The control group was a gender- and age-matched population assigned in a 1:1 fashion, with non-cardiovascular pathology, in which a TCT was performed for other reasons (e.g. staging for neoplasia, chest pain evaluation, and non-confirmed diagnosis of pulmonary embolism). Inclusion criteria were absence of AF, excluded at least by medical history and a resting surface-electrocardiogram, absence of mediastinal deformation due to extracardiac pathology, and a good visualization of the left atrium (LA) and PV in the TCT. Patients with evidence of advanced structural heart disease, as defined for the study group, were also excluded as controls. Controls were matched from a database with no information regarding PV anatomy.
Thoracic computed tomography protocol
All patients gave written informed consent. Multidetector computed tomography (MDCT) was performed on a 64 multidetector-row CT system (Somatom Definition, Siemens healthcare, Forchheim, Germany). In the control group thoracic images were captured during a single breath hold after full inspiration using the following parameters: detector configuration 32 × 0.6 mm, 120 kV, care dose with reference mAs of 80. All patients received a non-ionic contrast material of 80 mL (Ultravist-370, Bayer Schering Pharma, Germany) at a flow rate of 2.5 mL/s followed by a 50 mL saline chaser bolus after a scan delay of 45 s. Patients in the study group received a MDCT restricted to the contours of the heart. Images were acquired with the following parameters: detector configuration 32 × 0.6 mm, 100 kV, care dose with reference mAs of 150, and patient-adapted scan delay using care bolus with a peak enhancement threshold of 140 HU in theLA.
Multidetector computed tomography data analysis
Reconstructed images with a slice thickness of 1.0 mm and a reconstruction interval of 0.6 mm were transferred to a PACS workstation with 3D capabilities (Centricity, AW Suite, GE Healthcare, Muenchen, Germany) to create multiplanar reformations in the axial, coronal, and sagittal orientation. Furthermore, 3D volume-rendered images were included for depiction of pulmonary vein anatomical variants. The ostia of the PV were identified visually on 2D images. Ostial diameter measurements were performed strictly perpendicular to the defined PV ostium using a reformatted adaptive plane (Figure 1).
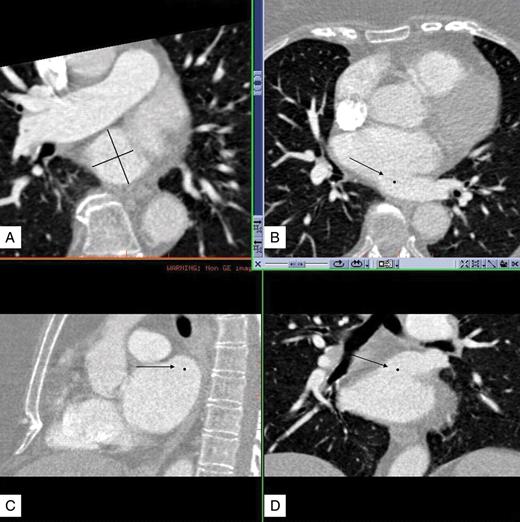
Reconstructed images of the multidetector computed tomography were transferred to a PACS workstation with 3D capabilities (Centricity, AW Suite, General Electric) to create multiplanar reformations in the axial (B), sagittal (C), and coronal (D) orientations. Arrows show the ostium of an LCO with LCT. Dots represent the plane of the ostium on each orientation. On a reformatted adaptive plane (A) we determined the strictly perpendicular plane to the ostium and measured the minimal and maximal ostial diameter. This procedure was performed for each pulmonary vein ostium.
All patients and controls were evaluated by two independent observers (A.B. and A.J.V.). In case of discrepancy between observers in terms of the presence of a PV anatomical variant, the TCT was evaluated again by both observers and a consensus anatomical diagnosis was made. The following parameters were determined: Quantitative measurements were performed using digital callipers. Mean values of both independent performed measurements represent final distances that are presented in the Results.
1. Description of anatomic PV variants:
Normal anatomy: presence of two septal or right and two lateral or left separate PV ostia.
Left common ostium (LCO): coalescence of the left superior (LSPV) and left inferior (LIPV) pulmonary veins proximal to the atrial body, irrespective of the length of the main trunk.
Long common trunk (LCT): ≥10 mm of common trunk from the LCO to the bifurcation.
Right common ostium (RCO): coalescence of the right superior (RSPV) and right inferior (RIPV) pulmonary veins proximal to the atrial body, irrespective of the length of the common trunk.
Right middle pulmonary vein (RMPV): accessory, separate ostium for the middle-lobe vein.
Right top pulmonary vein (RTPV): separate ostium above the RSPV.12 Other anatomical variants were not observed in our patients.
2. Measurement of PV maximal and minimal ostial diameter.
3. Measurement of the distance from the ostia to the first branch for every PV. Early Branching was defined as a distance to the first branch ≤5 mm.13
Two-dimensional echocardiographic images of the LA were obtained in the parasternal long-axis and apical two-chamber and four-chamber view. Measurement of left atrial (LA) diameter from M-mode was guided by parasternal short axis at the level of the aortic valve.14
Statistical analysis
Statistical analyses were performed using SAS 9.2 (SAS Institute Inc., Cary, NC, USA). χ2 test or Fisher's exact test were used for comparisons of categorical variables between the AF group and the control group. For continuous variables the comparisons were calculated with independent sample t-test. Analysis of variance with Tukey-test as post-hoc analysis was used for comparisons of PV dimensions for each group.
The result was considered significant in case of the two-sided P< 0.05.
For the significant PV anatomical variants odds ratio (OR) with 95% confidence interval (CI) were calculated.
Results
The 166 AF patients and the 166 control group patients had by study design the same age and gender distribution: mean age 59 ± 10 years, 65.1% were men. In the AF-group, 79 (47.6%) had paroxysmal, 87 (52.4%) persistent, or longstanding persistent AF. Table 1 shows the presence of PV anatomical variants in the AF- and control group. The initial independent evaluation of both observers showed a good concordance of pulmonary vein anatomy, with a ĸ-value = 0.81. After revision of discrepancies, a consensus anatomic description was possible in all cases. Anormal PV pattern was observed in 92 (55.4%) of AF patients vs. 117 (70.5%) in controls (P= 0,006), OR = 1.8, 95% CI (1.1–3.0). Analysing each PV variant separately, AF patients had significantly more LCO than controls (33.7 vs. 19.9%, P= 0.004), OR = 2.1, 95% CI (1.2–3.5). There was a trend towards more frequent LCT in AF patients (15.6 vs. 10.2%, P= 0.14). The frequency of other PV variants was not significantly different between both groups. The presence of an LCO in AF patients was neither related to gender nor to age: 34.2% of men, 35.7% of women, mean age with LCO: 59 ± 9.5 years, without LCO 59 ± 10.5 years; in controls: 20.4% of men, 15.1% of women, mean age with LCO: 58.7 ± 10.3 years, without LCO: 59.2 ± 9.9 years. LCO prevalence was not different among patients with paroxysmal AF (38.0%) vs. persistent or longstanding persistent AF (31.0%). We found no differences in LA diameter between patients with and without LCO (4.5 ± 0.9 vs. 4.6 ± 0.6 cm, P= 0.36). Figure 2 shows examples of 3D-reconstructions of the left atria with normal anatomy and different PV anatomical variants that were found in the present study.
Pulmonary vein anatomical variants in the atrial fibrillation -group and the control group
Pulmonary vein anatomy . | AF-group . | Control group . | P . |
---|---|---|---|
. | (n= 166), n (%) . | (n= 166), n (%) . | . |
Normal pattern | 92 (55.4%) | 117 (70.5%) | P= 0.006 |
LCO | 56 (33.7%) | 33 (19.9%) | P= 0.004 |
LCT | 26 (15.6%) | 17 (10.2%) | P= 0.14 |
RCO | 6 (3.6%) | 4 (2.4%) | P= 0.7 |
RMPV | 19 (11.5%) | 16 (9.6%) | P= 0.7 |
Right top pulmonary vein | 0 | 1 (0.6%) | |
LCO + RMPV | 6 (3.6%) | 4 (2.4%) | P= 0.7 |
LCO + RCO | 1 (0.6%) | 1 (0.6%) | P= 1 |
Pulmonary vein anatomy . | AF-group . | Control group . | P . |
---|---|---|---|
. | (n= 166), n (%) . | (n= 166), n (%) . | . |
Normal pattern | 92 (55.4%) | 117 (70.5%) | P= 0.006 |
LCO | 56 (33.7%) | 33 (19.9%) | P= 0.004 |
LCT | 26 (15.6%) | 17 (10.2%) | P= 0.14 |
RCO | 6 (3.6%) | 4 (2.4%) | P= 0.7 |
RMPV | 19 (11.5%) | 16 (9.6%) | P= 0.7 |
Right top pulmonary vein | 0 | 1 (0.6%) | |
LCO + RMPV | 6 (3.6%) | 4 (2.4%) | P= 0.7 |
LCO + RCO | 1 (0.6%) | 1 (0.6%) | P= 1 |
Pulmonary vein anatomical variants in the atrial fibrillation -group and the control group
Pulmonary vein anatomy . | AF-group . | Control group . | P . |
---|---|---|---|
. | (n= 166), n (%) . | (n= 166), n (%) . | . |
Normal pattern | 92 (55.4%) | 117 (70.5%) | P= 0.006 |
LCO | 56 (33.7%) | 33 (19.9%) | P= 0.004 |
LCT | 26 (15.6%) | 17 (10.2%) | P= 0.14 |
RCO | 6 (3.6%) | 4 (2.4%) | P= 0.7 |
RMPV | 19 (11.5%) | 16 (9.6%) | P= 0.7 |
Right top pulmonary vein | 0 | 1 (0.6%) | |
LCO + RMPV | 6 (3.6%) | 4 (2.4%) | P= 0.7 |
LCO + RCO | 1 (0.6%) | 1 (0.6%) | P= 1 |
Pulmonary vein anatomy . | AF-group . | Control group . | P . |
---|---|---|---|
. | (n= 166), n (%) . | (n= 166), n (%) . | . |
Normal pattern | 92 (55.4%) | 117 (70.5%) | P= 0.006 |
LCO | 56 (33.7%) | 33 (19.9%) | P= 0.004 |
LCT | 26 (15.6%) | 17 (10.2%) | P= 0.14 |
RCO | 6 (3.6%) | 4 (2.4%) | P= 0.7 |
RMPV | 19 (11.5%) | 16 (9.6%) | P= 0.7 |
Right top pulmonary vein | 0 | 1 (0.6%) | |
LCO + RMPV | 6 (3.6%) | 4 (2.4%) | P= 0.7 |
LCO + RCO | 1 (0.6%) | 1 (0.6%) | P= 1 |
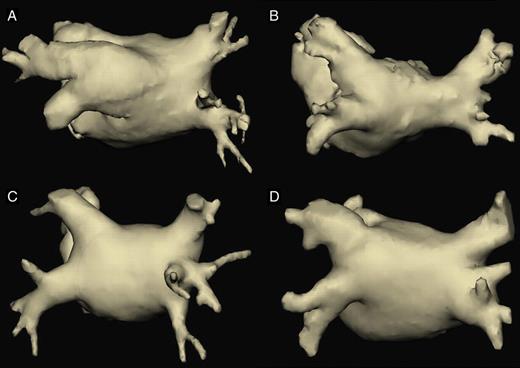
Three-dimensional reconstructions of the left atrium and pulmonary veins in a posterior view, showing different anatomical variants found in our study. (A) Left common ostium with a long common trunk. (B) Right common ostium. (C) Right middle pulmonary vein, with an early-branching pattern. (D) Example of a difficult classifiable anatomical pattern, finally classified as normal (two lateral and two septal ostia), although lateral veins are partially confluent. On the right side, what we described as an early branching of the right inferior pulmonary vein could by others have been classified as a right middle pulmonary vein.
The size of all PV ostia was significantly larger in AF patients than in controls for all veins. Table 2 summarizes ostial diameters in AF patients and controls. Right PV had a more circular morphology (a smaller maximal/minimal diameter ratio), and lateral veins a more elliptical form, a characteristic shared by AF patients and controls. Comparing the maximal diameter/minimal diameter ratio between AF patients and controls we found that the septal PV ostia were more round (smaller maximal/minimal diameter ratio) in AF-patients than in controls. Otherwise, the LIPV had a more elliptical form (a greater maximal/minimal diameter ratio) in AF patients than in controls. No significant differences were found for LSPV. Thus, AF patients had greater ostial diameters in the septal and lateral veins, but the form was more round for septal veins and more elliptical for the LIPV.
Pulmonary veins ostial dimensions in atrial fibrillation-patients and controls
. | AF-group (n= 166), . | Control group (n= 166), . | P . |
---|---|---|---|
. | mean ± SD . | mean ± SD . | . |
Right superior PV | n= 160 | n= 162 | |
Maximal diameter (mm) | 24.4 ± 3.0 | 20.5 ± 2.5 | P< 0.001 |
Minimal diameter (mm) | 19.6 ± 2.5 | 16.2 ± 2.6 | P< 0.001 |
Max/Min ratio | 1.25 ± 0.12 | 1.29 ± 0.17 | P= 0.03 |
Right inferior PV | n= 160 | n= 162 | |
Maximal diameter (mm) | 22.5 ± 3.0 | 20.0 ± 2.9 | P< 0.001 |
Minimal diameter (mm) | 19.7 ± 3.0 | 16.8 ± 2.7 | P< 0.001 |
Max/Min ratio | 1.15 ± 0.1 | 1.19 ± 0.11 | P< 0.001 |
Right middle PV | n= 19 | n= 16 | |
Maximal diameter (mm) | 11.5 ± 3.2 | 11.7 ± 3.9 | P= 0.87 |
Minimal diameter (mm) | 9.0 ± 2.8 | 9.0 ± 2.6 | P= 0.99 |
Max/Min ratio | 1.31 ± 0.25 | 1.30 ± 0.11 | P= 0.88 |
Right top PV | n= 0 | n= 1 | |
Maximal diameter (mm) | 14.4 | ||
Minimal diameter (mm) | 10.9 | ||
Max/Min ratio | 1.32 | ||
RCO | n= 6 | n= 4 | |
Maximal diameter (mm) | 37.1 ± 5.8 | 30.1 ± 4.6 | P= 0.08 |
Minimal diameter (mm) | 25.3 ± 4.3 | 21.7 ± 3.0 | P= 0.12 |
Max/Min ratio | 1.47 ± 0.14 | 1.39 ± 0.11 | P= 0.34 |
Left superior PV | n= 110 | n= 133 | |
Maximal diameter (mm) | 24.5 ± 2.8 | 20.0 ± 2.5 | P< 0.001 |
Minimal diameter (mm) | 17.6 ± 2.4 | 14.6 ± 2.0 | P< 0.001 |
Max/Min ratio | 1.41 ± 0.17 | 1.38 ± 0.18 | P= 0.25 |
Left inferior PV | n= 110 | n= 133 | |
Maximal diameter (mm) | 21.5 ± 3.2 | 18.1 ± 2.3 | P< 0.001 |
Minimal diameter (mm) | 15.5 ± 3.0 | 13.5 ± 2.0 | P< 0.001 |
Max/Min ratio | 1.41 ± 0.21 | 1.36 ± 0.16 | P= 0.03 |
LCO | n= 56 | n= 33 | |
Maximal diameter (mm) | 34.0 ± 4.2 | 28.9 ± 3.2 | P< 0.001 |
Minimal diameter (mm) | 21.0 ± 3.1 | 18.6 ± 2.6 | P< 0.001 |
Max/Min ratio | 1.65 ± 0.28 | 1.58 ± 0.26 | P= 0.26 |
. | AF-group (n= 166), . | Control group (n= 166), . | P . |
---|---|---|---|
. | mean ± SD . | mean ± SD . | . |
Right superior PV | n= 160 | n= 162 | |
Maximal diameter (mm) | 24.4 ± 3.0 | 20.5 ± 2.5 | P< 0.001 |
Minimal diameter (mm) | 19.6 ± 2.5 | 16.2 ± 2.6 | P< 0.001 |
Max/Min ratio | 1.25 ± 0.12 | 1.29 ± 0.17 | P= 0.03 |
Right inferior PV | n= 160 | n= 162 | |
Maximal diameter (mm) | 22.5 ± 3.0 | 20.0 ± 2.9 | P< 0.001 |
Minimal diameter (mm) | 19.7 ± 3.0 | 16.8 ± 2.7 | P< 0.001 |
Max/Min ratio | 1.15 ± 0.1 | 1.19 ± 0.11 | P< 0.001 |
Right middle PV | n= 19 | n= 16 | |
Maximal diameter (mm) | 11.5 ± 3.2 | 11.7 ± 3.9 | P= 0.87 |
Minimal diameter (mm) | 9.0 ± 2.8 | 9.0 ± 2.6 | P= 0.99 |
Max/Min ratio | 1.31 ± 0.25 | 1.30 ± 0.11 | P= 0.88 |
Right top PV | n= 0 | n= 1 | |
Maximal diameter (mm) | 14.4 | ||
Minimal diameter (mm) | 10.9 | ||
Max/Min ratio | 1.32 | ||
RCO | n= 6 | n= 4 | |
Maximal diameter (mm) | 37.1 ± 5.8 | 30.1 ± 4.6 | P= 0.08 |
Minimal diameter (mm) | 25.3 ± 4.3 | 21.7 ± 3.0 | P= 0.12 |
Max/Min ratio | 1.47 ± 0.14 | 1.39 ± 0.11 | P= 0.34 |
Left superior PV | n= 110 | n= 133 | |
Maximal diameter (mm) | 24.5 ± 2.8 | 20.0 ± 2.5 | P< 0.001 |
Minimal diameter (mm) | 17.6 ± 2.4 | 14.6 ± 2.0 | P< 0.001 |
Max/Min ratio | 1.41 ± 0.17 | 1.38 ± 0.18 | P= 0.25 |
Left inferior PV | n= 110 | n= 133 | |
Maximal diameter (mm) | 21.5 ± 3.2 | 18.1 ± 2.3 | P< 0.001 |
Minimal diameter (mm) | 15.5 ± 3.0 | 13.5 ± 2.0 | P< 0.001 |
Max/Min ratio | 1.41 ± 0.21 | 1.36 ± 0.16 | P= 0.03 |
LCO | n= 56 | n= 33 | |
Maximal diameter (mm) | 34.0 ± 4.2 | 28.9 ± 3.2 | P< 0.001 |
Minimal diameter (mm) | 21.0 ± 3.1 | 18.6 ± 2.6 | P< 0.001 |
Max/Min ratio | 1.65 ± 0.28 | 1.58 ± 0.26 | P= 0.26 |
Pulmonary veins ostial dimensions in atrial fibrillation-patients and controls
. | AF-group (n= 166), . | Control group (n= 166), . | P . |
---|---|---|---|
. | mean ± SD . | mean ± SD . | . |
Right superior PV | n= 160 | n= 162 | |
Maximal diameter (mm) | 24.4 ± 3.0 | 20.5 ± 2.5 | P< 0.001 |
Minimal diameter (mm) | 19.6 ± 2.5 | 16.2 ± 2.6 | P< 0.001 |
Max/Min ratio | 1.25 ± 0.12 | 1.29 ± 0.17 | P= 0.03 |
Right inferior PV | n= 160 | n= 162 | |
Maximal diameter (mm) | 22.5 ± 3.0 | 20.0 ± 2.9 | P< 0.001 |
Minimal diameter (mm) | 19.7 ± 3.0 | 16.8 ± 2.7 | P< 0.001 |
Max/Min ratio | 1.15 ± 0.1 | 1.19 ± 0.11 | P< 0.001 |
Right middle PV | n= 19 | n= 16 | |
Maximal diameter (mm) | 11.5 ± 3.2 | 11.7 ± 3.9 | P= 0.87 |
Minimal diameter (mm) | 9.0 ± 2.8 | 9.0 ± 2.6 | P= 0.99 |
Max/Min ratio | 1.31 ± 0.25 | 1.30 ± 0.11 | P= 0.88 |
Right top PV | n= 0 | n= 1 | |
Maximal diameter (mm) | 14.4 | ||
Minimal diameter (mm) | 10.9 | ||
Max/Min ratio | 1.32 | ||
RCO | n= 6 | n= 4 | |
Maximal diameter (mm) | 37.1 ± 5.8 | 30.1 ± 4.6 | P= 0.08 |
Minimal diameter (mm) | 25.3 ± 4.3 | 21.7 ± 3.0 | P= 0.12 |
Max/Min ratio | 1.47 ± 0.14 | 1.39 ± 0.11 | P= 0.34 |
Left superior PV | n= 110 | n= 133 | |
Maximal diameter (mm) | 24.5 ± 2.8 | 20.0 ± 2.5 | P< 0.001 |
Minimal diameter (mm) | 17.6 ± 2.4 | 14.6 ± 2.0 | P< 0.001 |
Max/Min ratio | 1.41 ± 0.17 | 1.38 ± 0.18 | P= 0.25 |
Left inferior PV | n= 110 | n= 133 | |
Maximal diameter (mm) | 21.5 ± 3.2 | 18.1 ± 2.3 | P< 0.001 |
Minimal diameter (mm) | 15.5 ± 3.0 | 13.5 ± 2.0 | P< 0.001 |
Max/Min ratio | 1.41 ± 0.21 | 1.36 ± 0.16 | P= 0.03 |
LCO | n= 56 | n= 33 | |
Maximal diameter (mm) | 34.0 ± 4.2 | 28.9 ± 3.2 | P< 0.001 |
Minimal diameter (mm) | 21.0 ± 3.1 | 18.6 ± 2.6 | P< 0.001 |
Max/Min ratio | 1.65 ± 0.28 | 1.58 ± 0.26 | P= 0.26 |
. | AF-group (n= 166), . | Control group (n= 166), . | P . |
---|---|---|---|
. | mean ± SD . | mean ± SD . | . |
Right superior PV | n= 160 | n= 162 | |
Maximal diameter (mm) | 24.4 ± 3.0 | 20.5 ± 2.5 | P< 0.001 |
Minimal diameter (mm) | 19.6 ± 2.5 | 16.2 ± 2.6 | P< 0.001 |
Max/Min ratio | 1.25 ± 0.12 | 1.29 ± 0.17 | P= 0.03 |
Right inferior PV | n= 160 | n= 162 | |
Maximal diameter (mm) | 22.5 ± 3.0 | 20.0 ± 2.9 | P< 0.001 |
Minimal diameter (mm) | 19.7 ± 3.0 | 16.8 ± 2.7 | P< 0.001 |
Max/Min ratio | 1.15 ± 0.1 | 1.19 ± 0.11 | P< 0.001 |
Right middle PV | n= 19 | n= 16 | |
Maximal diameter (mm) | 11.5 ± 3.2 | 11.7 ± 3.9 | P= 0.87 |
Minimal diameter (mm) | 9.0 ± 2.8 | 9.0 ± 2.6 | P= 0.99 |
Max/Min ratio | 1.31 ± 0.25 | 1.30 ± 0.11 | P= 0.88 |
Right top PV | n= 0 | n= 1 | |
Maximal diameter (mm) | 14.4 | ||
Minimal diameter (mm) | 10.9 | ||
Max/Min ratio | 1.32 | ||
RCO | n= 6 | n= 4 | |
Maximal diameter (mm) | 37.1 ± 5.8 | 30.1 ± 4.6 | P= 0.08 |
Minimal diameter (mm) | 25.3 ± 4.3 | 21.7 ± 3.0 | P= 0.12 |
Max/Min ratio | 1.47 ± 0.14 | 1.39 ± 0.11 | P= 0.34 |
Left superior PV | n= 110 | n= 133 | |
Maximal diameter (mm) | 24.5 ± 2.8 | 20.0 ± 2.5 | P< 0.001 |
Minimal diameter (mm) | 17.6 ± 2.4 | 14.6 ± 2.0 | P< 0.001 |
Max/Min ratio | 1.41 ± 0.17 | 1.38 ± 0.18 | P= 0.25 |
Left inferior PV | n= 110 | n= 133 | |
Maximal diameter (mm) | 21.5 ± 3.2 | 18.1 ± 2.3 | P< 0.001 |
Minimal diameter (mm) | 15.5 ± 3.0 | 13.5 ± 2.0 | P< 0.001 |
Max/Min ratio | 1.41 ± 0.21 | 1.36 ± 0.16 | P= 0.03 |
LCO | n= 56 | n= 33 | |
Maximal diameter (mm) | 34.0 ± 4.2 | 28.9 ± 3.2 | P< 0.001 |
Minimal diameter (mm) | 21.0 ± 3.1 | 18.6 ± 2.6 | P< 0.001 |
Max/Min ratio | 1.65 ± 0.28 | 1.58 ± 0.26 | P= 0.26 |
Early branching was by far more common in the RIPV in AF patients and controls, but we found no difference between both groups (31.8% in AF patients vs. 27.8% in controls), followed by the RSPV. In the RSPV, an early branching pattern was more frequently found among AF patients (20 vs. 10.2%, P= 0.02). Early branching was very uncommon in the left PV (Table 3). As shown in the table, although distance to first branching was slightly shorter in the LSPV and LIPV in AF patients, we did not find differences in the pre-defined ‘early branching pattern’ prevalence between AF patients and controls.
Branching pattern of pulmonary veins in atrial fibrillation-patients and controls
. | AF-group . | Control group . | P . |
---|---|---|---|
. | (n= 166) . | (n= 166) . | . |
Right superior PV | n= 160 | n= 162 | |
Distance to the first branch (mm) | 9.6 ± 5.1 | 10.7 ± 5.2 | P= 0.048 |
Early-branching pattern (n) | 32 (20%) | 17 (10.5%) | P= 0.02 |
Right inferior PV | n= 160 | n= 162 | |
Distance to first branch (mm) | 6.9 ± 2.9 | 6.8 ± 2.6 | P= 0.65 |
Early-branching pattern (n,%) | 51 (31.9%) | 45 (27.8%) | P= 0.42 |
Right middle PV | n= 19 | n= 16 | |
Distance to the first branch (mm) | 8.8 ± 7.8 | 11.7 ± 7.5 | P= 0.26 |
Early-branching pattern (n,%) | 9 (47.4%) | 5 (31.3) | P= 0.49 |
Right common ostium | n= 6 | n= 4 | |
Distance to the first branch (mm) | 4.2 ± 0.8 | 5.4 ± 1.4 | P= 0.65 |
Left superior PV | n= 110 | n= 133 | |
Distance to the first branch (mm) | 21.4 ± 6.4 | 19.8 ± 6.6 | P= 0.11 |
Early-branching pattern (n,%) | 0 (0%) | 2 (1.5%) | P= 0.2 |
Left inferior PV | n= 110 | n= 133 | |
Distance to the first branch (mm) | 14.3 ± 4.9 | 16.0 ± 4.6 | P= 0.008 |
Early-branching pattern (n,%) | 0 (0%) | 2 (1.5%) | P= 0.2 |
LCO | n= 56 | n= 33 | |
Distance to the first branch (mm) | 9.9 ± 4.8 | 10.8 ± 6.6 | P= 0.48 |
. | AF-group . | Control group . | P . |
---|---|---|---|
. | (n= 166) . | (n= 166) . | . |
Right superior PV | n= 160 | n= 162 | |
Distance to the first branch (mm) | 9.6 ± 5.1 | 10.7 ± 5.2 | P= 0.048 |
Early-branching pattern (n) | 32 (20%) | 17 (10.5%) | P= 0.02 |
Right inferior PV | n= 160 | n= 162 | |
Distance to first branch (mm) | 6.9 ± 2.9 | 6.8 ± 2.6 | P= 0.65 |
Early-branching pattern (n,%) | 51 (31.9%) | 45 (27.8%) | P= 0.42 |
Right middle PV | n= 19 | n= 16 | |
Distance to the first branch (mm) | 8.8 ± 7.8 | 11.7 ± 7.5 | P= 0.26 |
Early-branching pattern (n,%) | 9 (47.4%) | 5 (31.3) | P= 0.49 |
Right common ostium | n= 6 | n= 4 | |
Distance to the first branch (mm) | 4.2 ± 0.8 | 5.4 ± 1.4 | P= 0.65 |
Left superior PV | n= 110 | n= 133 | |
Distance to the first branch (mm) | 21.4 ± 6.4 | 19.8 ± 6.6 | P= 0.11 |
Early-branching pattern (n,%) | 0 (0%) | 2 (1.5%) | P= 0.2 |
Left inferior PV | n= 110 | n= 133 | |
Distance to the first branch (mm) | 14.3 ± 4.9 | 16.0 ± 4.6 | P= 0.008 |
Early-branching pattern (n,%) | 0 (0%) | 2 (1.5%) | P= 0.2 |
LCO | n= 56 | n= 33 | |
Distance to the first branch (mm) | 9.9 ± 4.8 | 10.8 ± 6.6 | P= 0.48 |
Branching pattern of pulmonary veins in atrial fibrillation-patients and controls
. | AF-group . | Control group . | P . |
---|---|---|---|
. | (n= 166) . | (n= 166) . | . |
Right superior PV | n= 160 | n= 162 | |
Distance to the first branch (mm) | 9.6 ± 5.1 | 10.7 ± 5.2 | P= 0.048 |
Early-branching pattern (n) | 32 (20%) | 17 (10.5%) | P= 0.02 |
Right inferior PV | n= 160 | n= 162 | |
Distance to first branch (mm) | 6.9 ± 2.9 | 6.8 ± 2.6 | P= 0.65 |
Early-branching pattern (n,%) | 51 (31.9%) | 45 (27.8%) | P= 0.42 |
Right middle PV | n= 19 | n= 16 | |
Distance to the first branch (mm) | 8.8 ± 7.8 | 11.7 ± 7.5 | P= 0.26 |
Early-branching pattern (n,%) | 9 (47.4%) | 5 (31.3) | P= 0.49 |
Right common ostium | n= 6 | n= 4 | |
Distance to the first branch (mm) | 4.2 ± 0.8 | 5.4 ± 1.4 | P= 0.65 |
Left superior PV | n= 110 | n= 133 | |
Distance to the first branch (mm) | 21.4 ± 6.4 | 19.8 ± 6.6 | P= 0.11 |
Early-branching pattern (n,%) | 0 (0%) | 2 (1.5%) | P= 0.2 |
Left inferior PV | n= 110 | n= 133 | |
Distance to the first branch (mm) | 14.3 ± 4.9 | 16.0 ± 4.6 | P= 0.008 |
Early-branching pattern (n,%) | 0 (0%) | 2 (1.5%) | P= 0.2 |
LCO | n= 56 | n= 33 | |
Distance to the first branch (mm) | 9.9 ± 4.8 | 10.8 ± 6.6 | P= 0.48 |
. | AF-group . | Control group . | P . |
---|---|---|---|
. | (n= 166) . | (n= 166) . | . |
Right superior PV | n= 160 | n= 162 | |
Distance to the first branch (mm) | 9.6 ± 5.1 | 10.7 ± 5.2 | P= 0.048 |
Early-branching pattern (n) | 32 (20%) | 17 (10.5%) | P= 0.02 |
Right inferior PV | n= 160 | n= 162 | |
Distance to first branch (mm) | 6.9 ± 2.9 | 6.8 ± 2.6 | P= 0.65 |
Early-branching pattern (n,%) | 51 (31.9%) | 45 (27.8%) | P= 0.42 |
Right middle PV | n= 19 | n= 16 | |
Distance to the first branch (mm) | 8.8 ± 7.8 | 11.7 ± 7.5 | P= 0.26 |
Early-branching pattern (n,%) | 9 (47.4%) | 5 (31.3) | P= 0.49 |
Right common ostium | n= 6 | n= 4 | |
Distance to the first branch (mm) | 4.2 ± 0.8 | 5.4 ± 1.4 | P= 0.65 |
Left superior PV | n= 110 | n= 133 | |
Distance to the first branch (mm) | 21.4 ± 6.4 | 19.8 ± 6.6 | P= 0.11 |
Early-branching pattern (n,%) | 0 (0%) | 2 (1.5%) | P= 0.2 |
Left inferior PV | n= 110 | n= 133 | |
Distance to the first branch (mm) | 14.3 ± 4.9 | 16.0 ± 4.6 | P= 0.008 |
Early-branching pattern (n,%) | 0 (0%) | 2 (1.5%) | P= 0.2 |
LCO | n= 56 | n= 33 | |
Distance to the first branch (mm) | 9.9 ± 4.8 | 10.8 ± 6.6 | P= 0.48 |
Discussion
To the best of our knowledge the present study is the first case-control study that proved that high proportion of AF patients present with PV anatomical variants as compared to a sex- and age-matched control group without AF. Specifically, the presence of a LCO, irrespective of the length of common trunk, was more common among AF patients, with an OR = 2.1.
Since PV have been recognized as important structures in AF pathophysiology and its isolation has become the cornerstone in AF ablation, several imaging studies have been performed in recent years to describe the PV anatomy. Different techniques, such as TCT, MRT, intracardiac echocardiography, and angiography have been used for such purposes.4,5,7,15 In general, all of these found a high proportion of PV variants. However, according to definition, the reported frequency of LCO had a wide dispersion and varied from a 3 to 75% prevalence.6,16 Studies that reported lower frequency of LCO included just common trunks ≥1.5 cm. According to our definition, a relative high proportion of LCO is therefore not surprising. Prevalence of LCT reported in our study agrees with most previous studies including AF patients. Compared with the largest reported series of PV anatomy in AF patients, recently published by Anselmino et al.,17 we found a similar prevalence of LCO and LCT, but a lower incidence of accessory pulmonary veins. That study, however, did not include a control group. Some previous publications included a small control group of patients without AF, but were not designed, and therefore underpowered to demonstrate differences in prevalence of PV anatomical variants among these two different populations.
Two theories may explain the observed anatomical differences in the present study. First, the presence of an LCO could be a congenital variant and thus constitute a risk factor for developing AF. The other possibility is that in patients with AF the remodelling of LA geometry ‘pushes’ the carina between left PVs into the vein, creating an ‘acquired'LCO. Several points may support the first as the most probable explanation: (i) we identified LCO in controls, who have no known cardiac disease, and therefore, no pathological remodelling process could have taken place in their left atria; (ii) in patients with AF, we found no association between the presence of an LCO and patient age or type of AF (paroxysmal or persistent). A more advanced age and a persistent form of AF may argue for a more advanced step in evolution of atrial remodelling, and in case of an ‘acquired’ LCO one would expect a higher prevalence of this PV variant in an older population with persistent AF; (iii) there was no difference in LA diameter between patients with and without an LCO; (iv) It appears very unlikely that a geometrical remodelling may ‘create’ a long LCT. The length of the common trunk was equal in AF patients and controls, and there was a tendency towards a higher prevalence of a LCT in AF patients, although it did not reach statistical significance. Thus, the length of the left common trunk may just represent a continuum of a congenitally present anatomic characteristic.
The arrhythmogenic potential of an LCO has been demonstrated in a few reports, representing a frequent source of ectopies triggering AF.18 A recent published study compared as the primary endpoint the anatomical pulmonary vein variants and dimensions between an AF population and a control group. Wozniak-Skowerska et al.19 compared CT images of 51 AF patients with 31 non-paired controls. They found a higher proportion of additional PV (12 vs. 0%, P< 0.05), and a trend towards more frequent common ostia (37.2 vs. 19.3%, P= 0.08), predominantly LCO (27.4 vs. 12.9%, P= 0.12) among AF patients. Interestingly, our results are similar in terms of numerical difference in LCO prevalence in patients with and without AF, but due to the present much larger sample size our results were highly statistically significant. In contrast to that report, we did not find a statistically significant different prevalence of additional right middle pulmonary veins.
We were able to identify larger PV diameters of all pulmonary veins, including common ostia, in patients with AF. This information is in accordance with previous published studies.10,16,20,21 We cannot establish whether larger vein ostia are a cause, a consequence, or just a bystander in AF. Although Knackstedt et al.22 did not find any correlation between LA enlargement and pulmonary vein ostial diameters by transoesophageal echocardiography, the most probable explanation is that larger PV ostia are a manifestation of ostial enlargement as part of the atrial structural remodelling process in AF. Of note, although all PV ostia were larger than in controls, the right PV were more circular, and the LIPV more elliptical in AF-patients than in controls, probably reflecting a different ostial remodelling for right and left pulmonary veins.
We believe that the results of our case-control study strongly support the hypothesis that an LCO represents a risk factor for developing AF. For determining the impact of an LCO as a risk factor for AF in the general population a cohort study will be necessary. Nevertheless, our study may have the following potential implications: First, anatomical PV information may be integrated in predictive algorithms of AF risk. This information could be especially important for patients in whom the anticoagulation is discussed, for example, in patients presenting with a cryptogenic ischaemic stroke. It could also be taken into account in symptomatic patients without documented arrhythmia. Second, knowing that an anatomical PV variant pre-disposed to AF may lead to further investigation that helps us to better understand the mechanisms involved in this complex arrhythmia. Third, in terms of catheter ablation strategy, one could hypothesize that isolating just the LCO could be sufficient for this patient population. Maybe in some patients the LCO represents the unique font of atrial AF triggers. Faced with our results, we believe that the LCO represents a very important target during ablation, but we have observed in multiple opportunities spontaneous ectopy and atrial tachycardias emerging additionally from septal pulmonary veins. This prevents us from recommending the sole isolation of the LCO as a general strategy.
Limitations
We included AF patients with an indication for catheter ablation had been decided. There could be selection bias in this group of symptomatic patients not responding to at least one antiarrhythmic drug and not necessarily representing the majority of the AF population. To precisely define the ostium of the PV, irrespective of the technique used, is partially subjective, so that defining universally accepted and reproducible measurement methods for PV remains difficult. Especially two descriptions result challenging: (i) differentiation between an accessory right middle PV ostium and a very early branching of the RSPV or RIPV; and (ii) defining partial confluent veins as a short common ostium or as two separate ostia on the left side. This limitation could be the main factor explaining differences between various publications. Another limitation of our study is the non-blinded design during the TCT evaluation. However, the double evaluation by two independent observers, with uniform applied criteria and high accuracy between both, validate our results.
Conclusions
Our case-control study for the first time shows solid evidence in favour of a higher prevalence of pulmonary vein variants, especially an LCO, among patients with AF as compared with gender and age-matched controls. Therefore, LCO might pre-dispose to the development of AF. The exact value of an LCO as a risk factor for developing AF in the general population requires prospective long-term evaluation.
Conflict of interest: none declared.
Funding
L.E. holds the Peter Osypka Professorship for experimental and clinical electrophysiology.
References
Author notes
These authors contributed equally to this work.