-
PDF
- Split View
-
Views
-
Cite
Cite
Ida Gregersen, Morgan Elizabeth Scarth, Rang Abdullah, Per Medbøe Thorsby, Lisa E Hauger, Kristina H Haugaa, Ellen Lund Sagen, Annika E Michelsen, Thor Ueland, Thor Edvardsen, Pål Aukrust, Vibeke Marie Almaas, Astrid Kristine Bjørnebekk, Bente Halvorsen, Elevated interleukin 8 and matrix metalloproteinase 9 levels are associated with myocardial pathology in users of anabolic-androgenic steroids, European Journal of Preventive Cardiology, Volume 31, Issue 12, September 2024, Pages 1469–1476, https://doi.org/10.1093/eurjpc/zwae126
- Share Icon Share
Abstract
In the current paper, we aim to explore the effect of both current and former long-term anabolic-androgenic steroid (AAS) use on regulation of systemic inflammatory markers and mediators of extracellular matrix (ECM) remodelling and their association with hormones and echocardiographic myocardial pathology in weightlifters.
In a cross-sectional study, 93 weightlifting AAS users, of whom 62 were current and 31 were past users, with at least 1-year cumulative AAS use (mean 11 ± 7 accumulated years of AAS use), were compared with 54 non-using weightlifting controls (WLCs) using clinical interview, blood pressure measurements, and echocardiography. Serum levels of interleukin (IL)-6, IL-8, tumour necrosis factor (TNF), interferon (IFN)-γ, growth differentiation factor (GDF)-15, and matrix metalloproteinase (MMP)-9, sex hormones, and lipids were analysed. It was found that serum levels of IL-8, GDF-15, and MMP-9 were significantly increased in current AAS users compared with former users and WLCs. Matrix metalloproteinase 9, but not IL-8, correlated consistently with sex hormone levels, and sex hormone levels correlated consistently with mean wall thickness, in current users. Moreover, HDL cholesterol was significantly lower in current vs. former AAS users and significantly inversely correlated with MMP-9 in current users. Further, in current users, MMP-9 and IL-8 correlated with markers of myocardial strain, and MMP-9 also correlated with indices of cardiac mass, which was not seen in former users. Mediation analyses suggested that MMP-9 could partly explain hormone-induced alterations in markers of myocardial damage in current users.
Long-term AAS is associated with increased levels of markers of inflammation and ECM remodelling, which seems to have a hormone-dependent (MMP-9) and a hormone-independent (IL-8) association with markers of myocardial dysfunction.
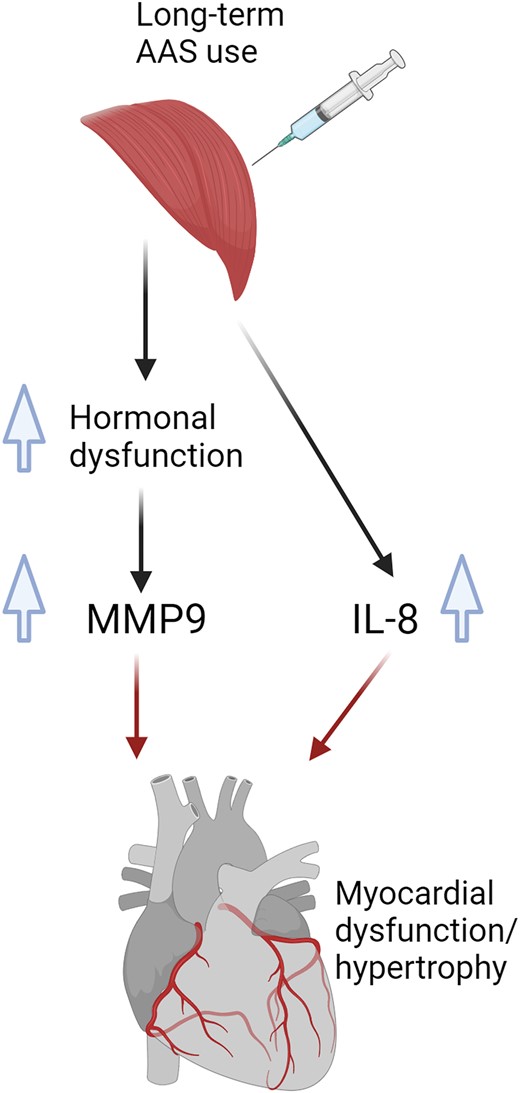
Lay Summary
Long-term use of anabolic-androgenic steroids (AASs) can increase inflammation and mediators of extracellular matrix (ECM) remodeling, which potentially could be involved in myocardial pathology seen in individuals using such steroids.
Anabolic-androgenic steroid use increased levels of inflammatory marker IL-8 and marker of ECM remodelling matrix metalloproteinase-9 (MMP-9).
Interleukin-8 and MMP-9 were both associated with myocardial pathology in current, but not former, users, suggesting that these markers are associated with the risk of myocardial damage during AAS use.
See the editorial comment for this article ‘Anabolic steroids in athletes: the interplay of hormones and inflammation leading to the heart's vulnerability’, by S. Marwaha and M. Papadakis, https://doi.org/10.1093/eurjpc/zwae253.
Introduction
The use of androgens, known as anabolic-androgenic steroids (AASs), is a worldwide substance use problem. Before the 1980s, AASs were primarily used by professional athletes, but today, most users are non-athlete weightlifters, especially young- to middle-aged men who enhance the anabolic effect for personal appearance.1 A meta-analysis from 2014 estimated that the global prevalence of AAS use was 6.4% among men and 1.6% among women, illustrating that AAS use is a serious and widespread public health problem.2 Anabolic-androgenic steroid use is associated with severe health problems, including hypogonadism,3 gynaecomastia, and infertility,4 as well as increased mortality.4 Further, AAS users are shown to have reduced levels of HDL and cholesterol efflux capacity, increased platelet counts, and endothelial dysfunction, suggesting increased cardiovascular (CV) risk in these individuals.5,6
Anabolic-androgenic steroid use causes drastic changes in hormonal levels. Users often practice ‘cycling’, taking high doses of AAS in a pattern of heavy use and periods of abstinence. The ingested doses are typically 5–100 times greater than endogenous testosterone production,7 leading to high systemic androgen levels while ‘on cycle’, followed by suppression of the hypothalamic-pituitary-gonadal axis when ‘off cycle’, due to negative feedback mechanisms.3 This suppression results in low or absent endogenous testosterone and symptoms of hypogonadism (depression, fatigue, anxiety, and sexual dysfunction), with implications for mood and behaviour.
Androgens are known to induce myocardial pathology, and both our research group and others have demonstrated that AAS use is associated with myocardial hypertrophy and impaired myocardial function. Systolic dysfunction is also seen in former users, years after AAS discontinuation.8–11 Although elevated levels of C-reactive protein have been observed in AAS users,5 the regulation of other inflammatory mediators and their association with CV risk and pathology in these individuals remain unclear. During myocardial remodelling, the interaction between inflammation and mediators of extracellular matrix (ECM) remodelling seems to play an important role,12 and androgens can also affect ECM remodelling in various tissues.13 The interaction between endocrine disturbances, inflammation and ECM remodelling, and cardiac pathology in AAS users is still unclear. To further explore these issues, in the present study, we first explore the regulation of upstream central inflammatory markers [i.e. interleukin (IL)-6, IL-8, tumour necrosis factor (TNF), and interferon (IFN)-γ], as well as markers of ECM remodelling [matrix metalloproteinase (MMP)-9 and growth differentiation factor (GDF)-15], relevant for CV pathology, in both current and former AAS users. Next, we assess the associations among these markers, and hormone levels, lipid parameters, and myocardial pathology, hypothesizing that these processes are inter-connected in AAS users.
Methods
Participants
The participants in this study were recruited as part of a longitudinal investigation focusing on the long-term consequences of AAS use in individuals, conducted at Oslo University Hospital.14,15 Weightlifters with no prior history of AAS or equivalent doping substance use, or who reported previous or current AAS use corresponding to at least 1 year of cumulative AAS use (summarizing on-cycle periods), were recruited. In total, samples were available from 147 adult male weightlifters, of whom 93 had a current (n = 62), defined as having used AAS within the past 12 months, or past use (n = 31) corresponding to at least 1 year of cumulative AAS exposure, more than 1 year ago, and 54 were weightlifting controls (WLCs) with no history of AAS use or other image- and performance-enhancing drugs (Table 1). Participants were recruited through social media, forums addressing AAS use, and online forums targeting people interested in heavy-weight training and bodybuilding. In addition, posters and flyers were distributed in select gyms in Oslo and surrounding areas in Norway. Relevant background and health information was collected from questionnaires filled in by the participants, as previously described.16 We obtained blood pressure measurements using an automatic sphygmomanometer, with a standard bladder cuff for most patients and larger cuffs for bigger arm circumferences.
. | Control (n = 54)a . | Current (n = 62)a . | Former (n = 31)a . | P . |
---|---|---|---|---|
Age (years) | 37 (9) | 39 (11) | 37 (10) | 0.70 |
Height (cm) | 182 (6) | 181 (7) | 182 (8) | 0.90 |
Weight (kg) | 92 (9) | 101 (15) | 96 (16) | 0.01* |
Alcohol (units/week) | 3.5 (3) | 4 (3) | 3 (3) | 0.60 |
Squatb | 175.25 (30.12) | 224.36 (55.45) | 216.75 (45.89) | <0.001*,** |
Benchb | 141.46 (19.49) | 181.47 (35.64) | 160.42 (31.76) | <0.001*,**,*** |
Deadliftb | 198.81 (39.73) | 244.89 (54.79) | 233.57 (72.70) | <0.001*,** |
Strength training (min/week) | 331.67 (167.55) | 394.44 (223.79) | 245.00 (168.32) | 0.02*** |
Endurance training (min/week) | 111.96 (148.87) | 116.73 (199.95) | 83.33 (110.87) | 0.40 |
Age at AAS initiation (years) | NA (NA) | 22.47 (7.60) | 21.74 (5.37) | 0.90 |
Accumulated years of AAS use | NA (NA) | 12.21 (7.54) | 8.65 (6.12) | 0.02*** |
Smoker status (%) | 0.21 | |||
Never | 22/35 (63%) | 33/56 (59%) | 16/24 (67%) | |
Former | 13/35 (37%) | 16/56 (29%) | 5/24 (21%) | |
Current | 0/35 (0%) | 7/56 (12%) | 3/24 (12%) | |
Sleeping medication | 5/48 (10%) | 24/52 (46%) | 13/25 (52%) | <0.001*,** |
Anxiety medication | 4/48 (8%) | 13/56 (23%) | 7/25 (28%) | 0.06 |
Antidepressants | 4/48 (8%) | 13/56 (23%) | 8/25 (32%) | 0.03** |
Antipsychotics | 1/48 (2%) | 4/56 (7%) | 0/25 (0%) | 0.22 |
ADHD medication | 0/48 (0%) | 5/56 (9%) | 1/24 (4.2%) | 0.10 |
CV medicationc | 2/48 (4.2%) | 14/56 (25%) | 5/25 (20%) | 0.01* |
Current CV medication | 1/2 (50%) | 8/14 (57%) | 2/5 (40%) | 0.8 |
History of cocaine use | 6/48 (13%) | 32/54 (59%) | 13/24 (54%) | <0.001*,** |
History of amphetamine use | 5/48 (10%) | 32/54 (59%) | 12/24 (50%) | <0.001*,** |
. | Control (n = 54)a . | Current (n = 62)a . | Former (n = 31)a . | P . |
---|---|---|---|---|
Age (years) | 37 (9) | 39 (11) | 37 (10) | 0.70 |
Height (cm) | 182 (6) | 181 (7) | 182 (8) | 0.90 |
Weight (kg) | 92 (9) | 101 (15) | 96 (16) | 0.01* |
Alcohol (units/week) | 3.5 (3) | 4 (3) | 3 (3) | 0.60 |
Squatb | 175.25 (30.12) | 224.36 (55.45) | 216.75 (45.89) | <0.001*,** |
Benchb | 141.46 (19.49) | 181.47 (35.64) | 160.42 (31.76) | <0.001*,**,*** |
Deadliftb | 198.81 (39.73) | 244.89 (54.79) | 233.57 (72.70) | <0.001*,** |
Strength training (min/week) | 331.67 (167.55) | 394.44 (223.79) | 245.00 (168.32) | 0.02*** |
Endurance training (min/week) | 111.96 (148.87) | 116.73 (199.95) | 83.33 (110.87) | 0.40 |
Age at AAS initiation (years) | NA (NA) | 22.47 (7.60) | 21.74 (5.37) | 0.90 |
Accumulated years of AAS use | NA (NA) | 12.21 (7.54) | 8.65 (6.12) | 0.02*** |
Smoker status (%) | 0.21 | |||
Never | 22/35 (63%) | 33/56 (59%) | 16/24 (67%) | |
Former | 13/35 (37%) | 16/56 (29%) | 5/24 (21%) | |
Current | 0/35 (0%) | 7/56 (12%) | 3/24 (12%) | |
Sleeping medication | 5/48 (10%) | 24/52 (46%) | 13/25 (52%) | <0.001*,** |
Anxiety medication | 4/48 (8%) | 13/56 (23%) | 7/25 (28%) | 0.06 |
Antidepressants | 4/48 (8%) | 13/56 (23%) | 8/25 (32%) | 0.03** |
Antipsychotics | 1/48 (2%) | 4/56 (7%) | 0/25 (0%) | 0.22 |
ADHD medication | 0/48 (0%) | 5/56 (9%) | 1/24 (4.2%) | 0.10 |
CV medicationc | 2/48 (4.2%) | 14/56 (25%) | 5/25 (20%) | 0.01* |
Current CV medication | 1/2 (50%) | 8/14 (57%) | 2/5 (40%) | 0.8 |
History of cocaine use | 6/48 (13%) | 32/54 (59%) | 13/24 (54%) | <0.001*,** |
History of amphetamine use | 5/48 (10%) | 32/54 (59%) | 12/24 (50%) | <0.001*,** |
P-values based on either ANOVA with Tukey HSD or χ2 tests.
ADHD, attention deficit/hyperactivity disorder; CV, cardiovascular.
aMean (SD) or n/N (%)
bMaximum weight for one repetition, kg.
cLifetime, previous or current.
*Control vs. current.
**Control vs. former.
***Current vs. former.
. | Control (n = 54)a . | Current (n = 62)a . | Former (n = 31)a . | P . |
---|---|---|---|---|
Age (years) | 37 (9) | 39 (11) | 37 (10) | 0.70 |
Height (cm) | 182 (6) | 181 (7) | 182 (8) | 0.90 |
Weight (kg) | 92 (9) | 101 (15) | 96 (16) | 0.01* |
Alcohol (units/week) | 3.5 (3) | 4 (3) | 3 (3) | 0.60 |
Squatb | 175.25 (30.12) | 224.36 (55.45) | 216.75 (45.89) | <0.001*,** |
Benchb | 141.46 (19.49) | 181.47 (35.64) | 160.42 (31.76) | <0.001*,**,*** |
Deadliftb | 198.81 (39.73) | 244.89 (54.79) | 233.57 (72.70) | <0.001*,** |
Strength training (min/week) | 331.67 (167.55) | 394.44 (223.79) | 245.00 (168.32) | 0.02*** |
Endurance training (min/week) | 111.96 (148.87) | 116.73 (199.95) | 83.33 (110.87) | 0.40 |
Age at AAS initiation (years) | NA (NA) | 22.47 (7.60) | 21.74 (5.37) | 0.90 |
Accumulated years of AAS use | NA (NA) | 12.21 (7.54) | 8.65 (6.12) | 0.02*** |
Smoker status (%) | 0.21 | |||
Never | 22/35 (63%) | 33/56 (59%) | 16/24 (67%) | |
Former | 13/35 (37%) | 16/56 (29%) | 5/24 (21%) | |
Current | 0/35 (0%) | 7/56 (12%) | 3/24 (12%) | |
Sleeping medication | 5/48 (10%) | 24/52 (46%) | 13/25 (52%) | <0.001*,** |
Anxiety medication | 4/48 (8%) | 13/56 (23%) | 7/25 (28%) | 0.06 |
Antidepressants | 4/48 (8%) | 13/56 (23%) | 8/25 (32%) | 0.03** |
Antipsychotics | 1/48 (2%) | 4/56 (7%) | 0/25 (0%) | 0.22 |
ADHD medication | 0/48 (0%) | 5/56 (9%) | 1/24 (4.2%) | 0.10 |
CV medicationc | 2/48 (4.2%) | 14/56 (25%) | 5/25 (20%) | 0.01* |
Current CV medication | 1/2 (50%) | 8/14 (57%) | 2/5 (40%) | 0.8 |
History of cocaine use | 6/48 (13%) | 32/54 (59%) | 13/24 (54%) | <0.001*,** |
History of amphetamine use | 5/48 (10%) | 32/54 (59%) | 12/24 (50%) | <0.001*,** |
. | Control (n = 54)a . | Current (n = 62)a . | Former (n = 31)a . | P . |
---|---|---|---|---|
Age (years) | 37 (9) | 39 (11) | 37 (10) | 0.70 |
Height (cm) | 182 (6) | 181 (7) | 182 (8) | 0.90 |
Weight (kg) | 92 (9) | 101 (15) | 96 (16) | 0.01* |
Alcohol (units/week) | 3.5 (3) | 4 (3) | 3 (3) | 0.60 |
Squatb | 175.25 (30.12) | 224.36 (55.45) | 216.75 (45.89) | <0.001*,** |
Benchb | 141.46 (19.49) | 181.47 (35.64) | 160.42 (31.76) | <0.001*,**,*** |
Deadliftb | 198.81 (39.73) | 244.89 (54.79) | 233.57 (72.70) | <0.001*,** |
Strength training (min/week) | 331.67 (167.55) | 394.44 (223.79) | 245.00 (168.32) | 0.02*** |
Endurance training (min/week) | 111.96 (148.87) | 116.73 (199.95) | 83.33 (110.87) | 0.40 |
Age at AAS initiation (years) | NA (NA) | 22.47 (7.60) | 21.74 (5.37) | 0.90 |
Accumulated years of AAS use | NA (NA) | 12.21 (7.54) | 8.65 (6.12) | 0.02*** |
Smoker status (%) | 0.21 | |||
Never | 22/35 (63%) | 33/56 (59%) | 16/24 (67%) | |
Former | 13/35 (37%) | 16/56 (29%) | 5/24 (21%) | |
Current | 0/35 (0%) | 7/56 (12%) | 3/24 (12%) | |
Sleeping medication | 5/48 (10%) | 24/52 (46%) | 13/25 (52%) | <0.001*,** |
Anxiety medication | 4/48 (8%) | 13/56 (23%) | 7/25 (28%) | 0.06 |
Antidepressants | 4/48 (8%) | 13/56 (23%) | 8/25 (32%) | 0.03** |
Antipsychotics | 1/48 (2%) | 4/56 (7%) | 0/25 (0%) | 0.22 |
ADHD medication | 0/48 (0%) | 5/56 (9%) | 1/24 (4.2%) | 0.10 |
CV medicationc | 2/48 (4.2%) | 14/56 (25%) | 5/25 (20%) | 0.01* |
Current CV medication | 1/2 (50%) | 8/14 (57%) | 2/5 (40%) | 0.8 |
History of cocaine use | 6/48 (13%) | 32/54 (59%) | 13/24 (54%) | <0.001*,** |
History of amphetamine use | 5/48 (10%) | 32/54 (59%) | 12/24 (50%) | <0.001*,** |
P-values based on either ANOVA with Tukey HSD or χ2 tests.
ADHD, attention deficit/hyperactivity disorder; CV, cardiovascular.
aMean (SD) or n/N (%)
bMaximum weight for one repetition, kg.
cLifetime, previous or current.
*Control vs. current.
**Control vs. former.
***Current vs. former.
Ethics, consent, and permissions
The study was approved by the Regional Committees for Medical and Health Research Ethics South East Norway (2013/601) and (2018/736) and conformed to the principles outlined in the Declaration of Helsinki. Written informed consent was obtained from all participants.
Blood sampling and routine analysis
Blood (5 mL) was sampled in anticoagulant-free tubes and kept at room temperature for 1 h before serum was isolated (centrifugation at 3500g for 15 min at 4°C). Serum was stored at −80°C until analysis. Routine measurements, including LDL, HDL, thyroid-stimulating hormone (TSH), and thyroxine (T4), were analysed at the Division of Laboratory Medicine, Oslo University Hospital, Oslo, Norway.
Measurement of inflammatory markers and mediators of extracellular matrix remodelling
The levels of IL-8, IL-6, TNF, and IFNγ were quantified by Meso Scale Diagnostic LLC (Rockville, MD, USA), and levels of GDF-15 and MMP-9 were assessed by DuoSet from R&D systems (Minneapolis, MN, USA). The CV% for IL-8, IL-6, TNF, and IFN was <10%, and the CV% for MMP-9 and GDF-15 was 5 and 11%, respectively.
Endocrine measurements
All hormone analyses were performed at the Hormone Laboratory, Oslo University Hospital, Oslo, Norway, and they were all accredited according to ISO 17025 as previously described.16 Briefly, follicle-stimulating hormone [FSH; limit of quantification (LOQ) 0.1 IU/L, CV% = 7], luteinizing hormone (LH; LOQ 0.1 IU/L, CV% = 3.8), and sex hormone–binding globulin (SHBG; LOQ 2 nmol/L, CV% = 7) were analysed by using non-competitive immunoluminometric assays (Siemens Healthineers), estradiol (E2; LOQ 0.06 nmol/L, CV% = 9) by competitive chemoluminescence (DiaSorin Inc.), and testosterone (LOQ 0.1 nmol/L, CV% = 8) by liquid chromatography–mass spectrometry (LCMS) (Hormone Laboratory, Oslo University Hospital, Oslo, Norway). The free testosterone index (FTI) was calculated by using the testosterone × 10/SHBG ratio.
Transthoracic echocardiography
As previously described,8 echocardiography was performed using Vivid E95 (GE Vingmed Ultrasound, Horten, Norway). All echocardiographic measurements were obtained by one investigator (V.M.A.) and analysed offline and blinded to AAS use status by another investigator (R.A.) using the software EchoPAC v203 (GE Vingmed Ultrasound). Two-dimensional (2D) and Doppler echocardiographic measurements were performed according to current standards.17 The left ventricle ejection fraction (LVEF) was calculated by the modified Simpson’s biplane method (LVEF Simpson). Left ventricle (LV) systolic function was further assessed by global longitudinal strain (LVGLS) using speckle tracking in the three apical views and defined as the average of peak longitudinal strains from a 16-LV segment model. If two or more segments in any given view were deemed inappropriate for strain assessments, LVGLS was excluded. Maximal wall thickness (MWT) was assessed from a parasternal short-axis view from all LV segments from the base to the apex of the LV. Left ventricular mass was estimated from parasternal views using the formula provided by Gottdiener et al.17 and indexed to body surface area (LVMI). Right ventricular (RV) systolic function was evaluated using RV global longitudinal strain (RVGLS). Right ventricular global longitudinal strain was obtained using speckle tracking.
Statistical analyses
All statistical analyses were done using R version 4.3.0.18 Demographic data were compared using analysis of variance (ANOVA) with post hoc Tukey’s honest significant difference (HSD) test for pairwise comparisons for continuous variables and χ2 tests for categorical variables. Group comparisons of hormones, inflammatory markers, and CV measures were computed using Kruskal–Wallis tests with post hoc Dunn tests for pairwise comparisons, and Spearman rank correlation coefficients were computed to identify associations among these measures. Also, since higher body weight and the use of stimulants may cause inflammatory or CV effects,19,20 sensitivity analyses of covariance were computed including body weight, age, and ordinal measures of amphetamine and cocaine use as additional covariates in the models. Mediation models were computed to investigate the potential role of inflammatory markers on the association between AAS use or hormone levels and CV pathology using structural equation modelling with the R package lavaan.21 In models with AAS use status as an exogenous variable, indicator variables (0/1) were created, where controls and former users make up the reference group. To account for skewed distributions, all variables were standardized prior to mediation analysis. Confidence intervals for the regression coefficients were computed based on 5000 bootstrap samples.
Within mediation models, the indirect effect is defined as the impact of the independent variable (hormones/AAS use status) on the dependent variable (CV measure) via the mediator (inflammatory marker), whereas the direct effect is the influence of the independent variable on the outcome without accounting for the mediating variable. The total effect is the sum of the effects from both direct and indirect pathways, and the proportion mediated is the ratio of the indirect effect to the total effect.
Results
Characteristics of study participants
The characteristics of the study population are presented in Table 1. The study participants displayed mostly similar characteristics, but current AAS users displayed a higher body weight and significantly greater strength in comparison with the WLC group, aligning with the anticipated anabolic properties of AAS. Further, the use of sleeping medication, antidepressants, and CV medications was significantly higher in current AAS users compared with WLCs. Information regarding missing data of all variables can be found in Supplementary material online, Table S1.
Anabolic-androgenic steroid users have increased levels of IL-8, GDF-15, and MMP-9
To explore the inflammatory status of AAS users, we measured a panel of markers of inflammation and ECM remodelling in serum from current and former AAS users and WLCs. As shown in Figure 1, serum levels of IL-8, GDF-15, and MMP-9 were significantly increased in current AAS users compared with former users and WLCs. No significant differences between the groups were observed for IL-6, TNF, or IFNγ. Adjusting for body weight and age or psychostimulant use did not affect the results (see Supplementary material online, Table S2).
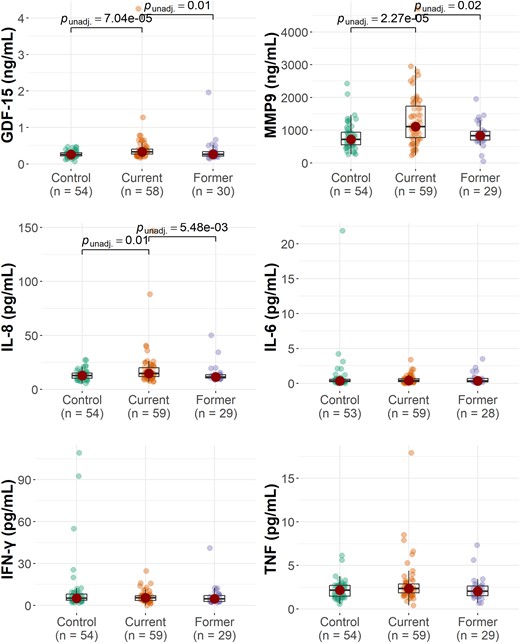
The group distribution of inflammatory markers. The P-values reflect the results of Kruskal–Wallis with post hoc Dunn tests.
Matrix metalloproteinase-9, but not interleukin-8, correlates with hormone levels in anabolic-androgenic steroid users
As expected, current users of AAS had a higher FTI, reflecting lower levels of SHBG and higher levels of testosterone, and lower levels of LH and FSH when compared with previous users and WLCs (Table 2). The differences compared with WLC were markedly attenuated in the former users’ group. Oestrogen was also increased in current users when compared with controls, but not when compared with former users (Table 2). In contrast, there were no differences in TSH and free T4 between current and previous AAS users, and all had values within the normal range. These parameters were therefore not included in further analyses.
Group mean and standard deviations of cardiovascular measures, inflammatory markers, and hormone levels
. | Control, n = 54 . | Current, n = 62 . | Former, n = 31 . | η2 . | P . |
---|---|---|---|---|---|
LV mass index, g/m2 | 80 (16) | 110 (24) | 94 (26) | 0.29 | <0.001*,**,*** |
LV GLS, % | −18.3 (2.2) | −15.0 (2.3) | −17.7 (2.1) | 0.24 | <0.001*,*** |
RV GLS, % | −23.0 (1.7) | −16.9 (3.5) | −17.8 (3.8) | 0.32 | <0.001*,** |
Systolic BP, mmHg | 132 (9) | 141 (17) | 141 (17) | 0.05 | 0.01* |
Diastolic BP, mmHg | 74 (9) | 75 (10) | 78 (10) | 0.01 | 0.14 |
LVEF, % | 59 (5) | 49 (7) | 50 (7) | 0.39 | <0.001*,** |
MWT, mm | 10 (2) | 15 (4) | 13 (3) | 0.36 | <0.001*,**,*** |
GDF15, ng/mL | 0.27 (0.09) | 0.45 (0.54) | 0.35 (0.33) | 0.10 | <0.001*,*** |
IL-6, pg/mL | 0.57 (0.76) | 0.58 (0.59) | 0.65 (0.77) | 0.01 | 0.69 |
IL-8, pg/mL | 13.27 (4.55) | 19.71 (20.55) | 14.14 (8.49) | 0.06 | 0.01*,*** |
MMP-9, ng/mL | 821 (412) | 1327 (805) | 886 (368) | 0.12 | <0.001*,*** |
IFNγ, pg/mL | 10.50 (19.61) | 6.07 (4.09) | 6.72 (7.27) | 0.01 | 0.73 |
TNF, pg/mL | 2.20 (1.02) | 2.92 (2.51) | 2.32 (1.36) | 0.01 | 0.21 |
FSH, IU/L | 4.96 (3.02) | 1.13 (2.08) | 3.50 (2.65) | 0.39 | <0.001*,**,*** |
LH, IU/L | 4.56 (1.86) | 0.73 (1.25) | 3.27 (1.98) | 0.50 | <0.001*,**,*** |
Oestrogen, nmol/L | 0.08 (0.03) | 0.20 (0.22) | 0.09 (0.04) | 0.04 | 0.02* |
Testosterone, nmol/L | 19.65 (7.19) | 38.98 (37.73) | 15.21 (8.55) | 0.06 | 0.004*** |
SHBG, nmol/L | 42.60 (19.31) | 21.04 (16.95) | 32.43 (15.25) | 0.24 | <0.001*,**,*** |
FTI | 4.87 (1.29) | 50.06 (96.37) | 4.89 (2.68) | 0.10 | <0.001*,**,*** |
HDL, mmol/L | 0.8–2.1a | 0.90 (0.37) | 1.16 (0.27) | 0.06 | 0.00*** |
LDL, mmol/L | 1.9–4.8a | 2.90 (0.83) | 2.89 (0.84) | 0.00 | 1.00 |
TSH, mIU/L | 0.5–3.6a | 1.62 (0.80) | 1.77 (0.76) | 0.02 | 0.27 |
T4, pmol/L | 12–22a | 15.11 (3.37) | 15.04 (1.54) | 0.01 | 0.64 |
. | Control, n = 54 . | Current, n = 62 . | Former, n = 31 . | η2 . | P . |
---|---|---|---|---|---|
LV mass index, g/m2 | 80 (16) | 110 (24) | 94 (26) | 0.29 | <0.001*,**,*** |
LV GLS, % | −18.3 (2.2) | −15.0 (2.3) | −17.7 (2.1) | 0.24 | <0.001*,*** |
RV GLS, % | −23.0 (1.7) | −16.9 (3.5) | −17.8 (3.8) | 0.32 | <0.001*,** |
Systolic BP, mmHg | 132 (9) | 141 (17) | 141 (17) | 0.05 | 0.01* |
Diastolic BP, mmHg | 74 (9) | 75 (10) | 78 (10) | 0.01 | 0.14 |
LVEF, % | 59 (5) | 49 (7) | 50 (7) | 0.39 | <0.001*,** |
MWT, mm | 10 (2) | 15 (4) | 13 (3) | 0.36 | <0.001*,**,*** |
GDF15, ng/mL | 0.27 (0.09) | 0.45 (0.54) | 0.35 (0.33) | 0.10 | <0.001*,*** |
IL-6, pg/mL | 0.57 (0.76) | 0.58 (0.59) | 0.65 (0.77) | 0.01 | 0.69 |
IL-8, pg/mL | 13.27 (4.55) | 19.71 (20.55) | 14.14 (8.49) | 0.06 | 0.01*,*** |
MMP-9, ng/mL | 821 (412) | 1327 (805) | 886 (368) | 0.12 | <0.001*,*** |
IFNγ, pg/mL | 10.50 (19.61) | 6.07 (4.09) | 6.72 (7.27) | 0.01 | 0.73 |
TNF, pg/mL | 2.20 (1.02) | 2.92 (2.51) | 2.32 (1.36) | 0.01 | 0.21 |
FSH, IU/L | 4.96 (3.02) | 1.13 (2.08) | 3.50 (2.65) | 0.39 | <0.001*,**,*** |
LH, IU/L | 4.56 (1.86) | 0.73 (1.25) | 3.27 (1.98) | 0.50 | <0.001*,**,*** |
Oestrogen, nmol/L | 0.08 (0.03) | 0.20 (0.22) | 0.09 (0.04) | 0.04 | 0.02* |
Testosterone, nmol/L | 19.65 (7.19) | 38.98 (37.73) | 15.21 (8.55) | 0.06 | 0.004*** |
SHBG, nmol/L | 42.60 (19.31) | 21.04 (16.95) | 32.43 (15.25) | 0.24 | <0.001*,**,*** |
FTI | 4.87 (1.29) | 50.06 (96.37) | 4.89 (2.68) | 0.10 | <0.001*,**,*** |
HDL, mmol/L | 0.8–2.1a | 0.90 (0.37) | 1.16 (0.27) | 0.06 | 0.00*** |
LDL, mmol/L | 1.9–4.8a | 2.90 (0.83) | 2.89 (0.84) | 0.00 | 1.00 |
TSH, mIU/L | 0.5–3.6a | 1.62 (0.80) | 1.77 (0.76) | 0.02 | 0.27 |
T4, pmol/L | 12–22a | 15.11 (3.37) | 15.04 (1.54) | 0.01 | 0.64 |
Results of Kruskal-Wallis test with post hoc Dunn test.
LV, left ventricle; GLS, global longitudinal strain; RV, right ventricle; BP, blood pressure; LVEF, left ventricle ejection fraction; MWT, Maximal wall thickness; GDF-15, growth differentiation factor; IL, interleukin; FSH, follicle-stimulating hormone; IU/L, international units/litre; LH, luteinizing hormone; SHBG, sex hormone–binding globulin; FTI, free testosterone index.
aReference values from the Division of Laboratory Medicine, Oslo University Hospital.
Significant P-values (<0.05) based on post hoc Dunn test.
*control vs. current.
**control vs. former.
***current vs. former.
Group mean and standard deviations of cardiovascular measures, inflammatory markers, and hormone levels
. | Control, n = 54 . | Current, n = 62 . | Former, n = 31 . | η2 . | P . |
---|---|---|---|---|---|
LV mass index, g/m2 | 80 (16) | 110 (24) | 94 (26) | 0.29 | <0.001*,**,*** |
LV GLS, % | −18.3 (2.2) | −15.0 (2.3) | −17.7 (2.1) | 0.24 | <0.001*,*** |
RV GLS, % | −23.0 (1.7) | −16.9 (3.5) | −17.8 (3.8) | 0.32 | <0.001*,** |
Systolic BP, mmHg | 132 (9) | 141 (17) | 141 (17) | 0.05 | 0.01* |
Diastolic BP, mmHg | 74 (9) | 75 (10) | 78 (10) | 0.01 | 0.14 |
LVEF, % | 59 (5) | 49 (7) | 50 (7) | 0.39 | <0.001*,** |
MWT, mm | 10 (2) | 15 (4) | 13 (3) | 0.36 | <0.001*,**,*** |
GDF15, ng/mL | 0.27 (0.09) | 0.45 (0.54) | 0.35 (0.33) | 0.10 | <0.001*,*** |
IL-6, pg/mL | 0.57 (0.76) | 0.58 (0.59) | 0.65 (0.77) | 0.01 | 0.69 |
IL-8, pg/mL | 13.27 (4.55) | 19.71 (20.55) | 14.14 (8.49) | 0.06 | 0.01*,*** |
MMP-9, ng/mL | 821 (412) | 1327 (805) | 886 (368) | 0.12 | <0.001*,*** |
IFNγ, pg/mL | 10.50 (19.61) | 6.07 (4.09) | 6.72 (7.27) | 0.01 | 0.73 |
TNF, pg/mL | 2.20 (1.02) | 2.92 (2.51) | 2.32 (1.36) | 0.01 | 0.21 |
FSH, IU/L | 4.96 (3.02) | 1.13 (2.08) | 3.50 (2.65) | 0.39 | <0.001*,**,*** |
LH, IU/L | 4.56 (1.86) | 0.73 (1.25) | 3.27 (1.98) | 0.50 | <0.001*,**,*** |
Oestrogen, nmol/L | 0.08 (0.03) | 0.20 (0.22) | 0.09 (0.04) | 0.04 | 0.02* |
Testosterone, nmol/L | 19.65 (7.19) | 38.98 (37.73) | 15.21 (8.55) | 0.06 | 0.004*** |
SHBG, nmol/L | 42.60 (19.31) | 21.04 (16.95) | 32.43 (15.25) | 0.24 | <0.001*,**,*** |
FTI | 4.87 (1.29) | 50.06 (96.37) | 4.89 (2.68) | 0.10 | <0.001*,**,*** |
HDL, mmol/L | 0.8–2.1a | 0.90 (0.37) | 1.16 (0.27) | 0.06 | 0.00*** |
LDL, mmol/L | 1.9–4.8a | 2.90 (0.83) | 2.89 (0.84) | 0.00 | 1.00 |
TSH, mIU/L | 0.5–3.6a | 1.62 (0.80) | 1.77 (0.76) | 0.02 | 0.27 |
T4, pmol/L | 12–22a | 15.11 (3.37) | 15.04 (1.54) | 0.01 | 0.64 |
. | Control, n = 54 . | Current, n = 62 . | Former, n = 31 . | η2 . | P . |
---|---|---|---|---|---|
LV mass index, g/m2 | 80 (16) | 110 (24) | 94 (26) | 0.29 | <0.001*,**,*** |
LV GLS, % | −18.3 (2.2) | −15.0 (2.3) | −17.7 (2.1) | 0.24 | <0.001*,*** |
RV GLS, % | −23.0 (1.7) | −16.9 (3.5) | −17.8 (3.8) | 0.32 | <0.001*,** |
Systolic BP, mmHg | 132 (9) | 141 (17) | 141 (17) | 0.05 | 0.01* |
Diastolic BP, mmHg | 74 (9) | 75 (10) | 78 (10) | 0.01 | 0.14 |
LVEF, % | 59 (5) | 49 (7) | 50 (7) | 0.39 | <0.001*,** |
MWT, mm | 10 (2) | 15 (4) | 13 (3) | 0.36 | <0.001*,**,*** |
GDF15, ng/mL | 0.27 (0.09) | 0.45 (0.54) | 0.35 (0.33) | 0.10 | <0.001*,*** |
IL-6, pg/mL | 0.57 (0.76) | 0.58 (0.59) | 0.65 (0.77) | 0.01 | 0.69 |
IL-8, pg/mL | 13.27 (4.55) | 19.71 (20.55) | 14.14 (8.49) | 0.06 | 0.01*,*** |
MMP-9, ng/mL | 821 (412) | 1327 (805) | 886 (368) | 0.12 | <0.001*,*** |
IFNγ, pg/mL | 10.50 (19.61) | 6.07 (4.09) | 6.72 (7.27) | 0.01 | 0.73 |
TNF, pg/mL | 2.20 (1.02) | 2.92 (2.51) | 2.32 (1.36) | 0.01 | 0.21 |
FSH, IU/L | 4.96 (3.02) | 1.13 (2.08) | 3.50 (2.65) | 0.39 | <0.001*,**,*** |
LH, IU/L | 4.56 (1.86) | 0.73 (1.25) | 3.27 (1.98) | 0.50 | <0.001*,**,*** |
Oestrogen, nmol/L | 0.08 (0.03) | 0.20 (0.22) | 0.09 (0.04) | 0.04 | 0.02* |
Testosterone, nmol/L | 19.65 (7.19) | 38.98 (37.73) | 15.21 (8.55) | 0.06 | 0.004*** |
SHBG, nmol/L | 42.60 (19.31) | 21.04 (16.95) | 32.43 (15.25) | 0.24 | <0.001*,**,*** |
FTI | 4.87 (1.29) | 50.06 (96.37) | 4.89 (2.68) | 0.10 | <0.001*,**,*** |
HDL, mmol/L | 0.8–2.1a | 0.90 (0.37) | 1.16 (0.27) | 0.06 | 0.00*** |
LDL, mmol/L | 1.9–4.8a | 2.90 (0.83) | 2.89 (0.84) | 0.00 | 1.00 |
TSH, mIU/L | 0.5–3.6a | 1.62 (0.80) | 1.77 (0.76) | 0.02 | 0.27 |
T4, pmol/L | 12–22a | 15.11 (3.37) | 15.04 (1.54) | 0.01 | 0.64 |
Results of Kruskal-Wallis test with post hoc Dunn test.
LV, left ventricle; GLS, global longitudinal strain; RV, right ventricle; BP, blood pressure; LVEF, left ventricle ejection fraction; MWT, Maximal wall thickness; GDF-15, growth differentiation factor; IL, interleukin; FSH, follicle-stimulating hormone; IU/L, international units/litre; LH, luteinizing hormone; SHBG, sex hormone–binding globulin; FTI, free testosterone index.
aReference values from the Division of Laboratory Medicine, Oslo University Hospital.
Significant P-values (<0.05) based on post hoc Dunn test.
*control vs. current.
**control vs. former.
***current vs. former.
Among the inflammatory markers up-regulated in AAS users, IL-8 showed no correlation with any of the hormones in current or former users. Growth differentiation factor-15 displayed a negative correlation with FSH in current users (r = −0.30, P < 0.05). In contrast, MMP-9 demonstrated significant correlations with all hormones, showing positive associations with testosterone levels, FTI, and oestrogen (r = 0.52 and r = 0.62, P < 0.001 and r = 0.36, respectively, P < 0.01), as well as negative correlations with FSH, LH, and SHBG levels (r = −0.55, r = −0.55, r = −0.72, respectively, P < 0.001). These findings were observed exclusively in current users of AAS, and not in former users, as illustrated in Supplementary material online, Figure S1.
Lipid levels in anabolic-androgenic steroid users: HDL levels are inversely correlated with matrix metalloproteinase-9
As shown in Table 2, both current and previous AAS users had LDL cholesterol levels within normal limits, with no differences between the AAS groups. In contrast, although both AAS groups had HDL cholesterol levels within the normal range, HDL levels were significantly reduced in current users, compared with former users, and the mean levels in current users were close to the lower limit for the normal range (Table 2). Moreover, HDL showed a negative correlation with MMP-9, in current but not in former users (r = −0.6, P < 0.001, see Supplementary material online, Figure S3). Also, only in current users, HDL showed a negative correlation with MWT (r = −0.38, P < 0.001), but not with any of the other cardiac markers (see Supplementary material online, Figure S2).
Hormone levels correlate with markers of myocardial dysfunction in anabolic-androgenic steroid users
As previously mentioned, the long-term AAS use is associated with biventricular cardiomyopathy, and we found significant group differences across all selected cardiac measures except diastolic blood pressure, as shown in Table 2. Among the current users of AAS, several associations between various cardiac measures and hormone profiles were observed. First, FTI, testosterone, and oestrogen showed a positive association with increased LV wall thickness and MWT (r = 0.54, r = 0.50, and r = 0.51, respectively, P < 0.001), and FTI showed a positive correlation with myocardial hypertrophy and LVMI (r = 0.29, P < 0.05). Second, as expected, an opposite pattern was seen for FSH, LH, and SHBG with a negative correlation with LVMI (r = −0.29, r = −0.33, and r = −0.33, respectively, P < 0.05 for all) and MWT (r = −0.46, r = −0.44, and r = −0.44, respectively, P < 0.001 for all). Further, SHBG showed a negative correlation (r = −0.33, P < 0.05) and FTI showed a positive correlation with LVGLS (r = 0.34, P < 0.05) reflecting a more complex effect on myocardial function (see Supplementary material online, Figure S2). Whereas the association with LVMI and MWT were attenuated in former users, FSH was negatively correlated with RVGLS (r = −0.47, P < 0.05) and oestrogen was positively correlated with LVGLS (r = 0.59, P < 0.01), indicating a high oestrogen and a low FSH associated with impaired global longitudinal strain (GLS) in former users (see Supplementary material online, Figure S2).
Markers of inflammation and extracellular matrix remodelling are associated with myocardial dysfunction in anabolic-androgenic steroid users
We further explored the relationship between markers of inflammation and ECM remodelling and cardiac measures in AAS users. In current users, IL-8 correlated positively with RVGLS and LVGLS (r = 0.38, P < 0.01 and r = 0.33, P < 0.05, respectively), implying that high IL-8 levels are associated with impaired LV and RV functions, as well diastolic blood pressure (r = 0.31, P < 0.05, see Supplementary material online, Figure S3). In contrast, we found no correlation between IL-8 and cardiac measures in former users. Furthermore, in current users, MMP-9 exhibited positive correlations with measures indicative of impaired LV and RV functions and hypertrophy, including RVGLS and LVGLS (r = 0.35 and r = 0.33, respectively, P < 0.05), as well as LVMI and MWT (r = 0.52 and r = 0.47, respectively, P < 0.001). These relationships were not observed among former users. Growth differentiation factor-15, a known marker and a mediator of myocardial hypertrophy,22 correlated with MWT in current users (r = 0.29, P < 0.05) and with systolic blood pressure in former users (r = 0.37, P < 0.05, see Supplementary material online, Figure S3).
Matrix metalloproteinase-9 partially mediates the association between hormone levels and markers of myocardial hypertrophy in anabolic-androgenic steroid users
Whereas MMP-9 correlated with several hormone parameters in the AAS group, GDF-15 showed a less pronounced association and IL-8 did not correlate with any of the hormones. To further explore the relationship between MMP-9 and hormones, which was associated with myocardial pathology in AAS users, we performed mediation analyses combining these three elements (i.e. MMP-9, hormones, and myocardial parameters). Of the total effect of AAS use on LVMI and MWT (β = 0.97 and β = 1.00, respectively), the proportion mediated by MMP-9 was 19 and 24%, respectively (see Supplementary material online, Figure S4). Further, testosterone and MWT were highly correlated (r = 0.50, P < 0.001) in current users, and MMP-9 correlated with both testosterone and MWT. Of the total effect testosterone had on MWT (β = 0.41), mediation analysis showed that MMP-9 accounted for 24% (see Supplementary material online, Figure S4). Further, MMP-9 was significantly correlated with SHBG and with MWT and LVMI, and mediation analysis showed that MMP-9 could explain 37 and 41% of the direct effect that SHBG had on MWT and LVMI, respectively (β = −0.38, β = −0.29, respectively, see Supplementary material online, Figure S5).
Discussion
There are limited data on the effect of AAS use on inflammation, especially concerning long-term use. As immune cells express androgen receptors, it is likely that AASs affect immune activation and inflammation; however, this will likely be dosage- and substance-dependent.
Our previous research has recently shown that AAS users commonly exhibit severe biventricular cardiomyopathy and hypertension.8 Herein, we demonstrate that this phenotype could be related to increased levels of GDF-15 and in particular to IL-8 and MMP-9. Our findings underscore that AASs have effects on markers of inflammation and ECM remodelling, potentially playing a role in mediating CV side effects in AAS users. Further, we have shown that levels of all three mediators are reduced after cessation of AAS use, even when strength training is sustained, supporting a direct but partly reversible effect of the AAS.
Herein, we demonstrate that markers of myocardial hypertrophy, i.e. LVMI and MWT, were associated with hormone levels (FTI, testosterone, and oestrogen) in AAS users. Whether these associations with the hormone profile reflect direct effects of the actual hormones is not yet clear. Anabolic-androgenic steroid use is shown to affect the CV system in multiple ways, by directly harming the myocardium and through indirect mechanisms such as increased blood pressure or by increasing atherosclerosis.23 A disturbed hormone profile is a marker of AAS use and may also directly contribute to myocardial hypertrophy. Thus, testosterone through its more potent metabolite 5α-dihydrotestosterone has been shown to induce cardiac hypertrophy in animal models,24,25 but it is not yet clear whether similar mechanisms are operating in humans.
Although within the normal range for both groups, HDL was significantly reduced in current vs. former users, and the mean level in current users was close to the lower limit for the normal range. This effect of AAS on HDL is well known and could have implications on CV risk.26,27 Further, and of interest, HDL showed a negative correlation with MMP-9 in current users, which was not found in former users. HDL is previously shown to abolish oxLDL-induced MMP-9 levels in macrophages in vitro.28 Moreover, MMP-9 has been shown to bind to HDL, attenuating its function including its potential anti-inflammatory properties.29 Whether these potential harmful interactions between HDL and MMP-9 are operating in vivo in AAS users is however to be determined.
Anabolic-androgenic steroid has been shown to modulate remodelling of ECM,13 and our study suggests that this interplay could involve MMP-9, known to be involved in myocardial remodelling.30 Matrix metalloproteinase-9 was strongly associated with markers of both myocardial dysfunction and myocardial hypertrophy and with hormone disturbances following AAS use. This association was strengthened by our analyses showing a partial mediation of MMP-9 on the effect of hormone disturbances in AAS users on markers of myocardial hypertrophy. There are some in vitro data on the interaction between testosterone and MMPs in endothelial cells (i.e. MMP-2),31 but an interaction among AAS-induced hormone disturbances, MMP-9, and cardiac hypertrophy has not been previously shown.
A major finding in the present study was the association of IL-8 with impaired right and left myocardial function in AAS users. Of interest, in contrast to MMP-9, IL-8 was not associated with hormone levels but correlated with impairment of both LVGLS and RVGLS, suggesting a hormone-independent route for inflammation-driven CV risk after AAS use for IL-8. Studies have shown that supra-physiological doses of AAS can have both immunostimulatory or immunosuppressive effects; however, there is a lack of human studies on long-term AAS use and its effect on immune function,32 and how and whether androgen can directly induce increased IL-8 levels is at present not clear. Further, how IL-8 can affect myocardial remodelling in AAS users is not known, but IL-8 has a known role in CV disease,33 and importantly, is previously shown to have a possible role in reperfusion-related injury and LV remodelling after ST- elevation myocardical infarction (STEMI).34,35 Thus, increased levels of IL-8 after AAS use could be involved in the development of myocardial damage. Together, our findings suggest that whereas MMP-9 interacts with hormone disturbances in mediating cardiac hypertrophy, IL-8 primarily affects myocardial dysfunction independently of the disturbed hormone levels.
We have recently shown the presence of persistent myocardial pathology, even years after discontinuation of AAS use.8 Notably, levels of GDF-15, IL-8, and MMP-9 were normalized in former users. There is however a possibility that these mediators have contributed to either irreversible damage or damage that heals slower than the normalization of the mediators themselves, resulting in prolonged myocardial pathophysiology, even after normalization of serum levels. Also, other mechanisms are likely in play. For instance, GDF-15 can be released upon mechanical stress and injury and thus be a marker more than a mediator in this case.36 Further, our present data suggest that the sustained damage to some degree could be mediated through endocrine mechanisms. While most of the hormones were normalized in former users, some were still altered in former users, and association with myocardial damage remained, including a negative correlation between RVGLS and FSH and a positive correlation between oestrogen and LVGLS. This is in accordance with previous findings that structural parameters (LVMI) seem to normalize more after cessation of AAS use than functional measures (i.e. ejection fraction and GLS).10
This study has some limitations. Due to the cross-sectional nature of the study, we cannot provide causality, and further, associations do not necessarily mean any causal relationship. Second, while the WLC is chosen to match the AAS group on several aspects, we cannot rule out that AAS use correlates with lifestyle factors influencing inflammation and CV health, such as underlying medical conditions, diet, and drug use. Albeit, our findings were not impacted by adjusting for weight, age, and use of psychostimulants, and other potential confounding factors were not accounted for in our analyses, suggesting that our results may encompass broader influences beyond the pharmacological effects of AAS. Third, as the history and nature of exercise and AAS use were self-reported, it can be a subject to recall bias. Further, there is a possibility of selection bias, in which participants volunteering are more health conscious than the general AAS users; however, this would most likely under- rather than over-estimate our results. Moreover, the ability to generalize the data is somewhat limited by the homogeneity of the population, being almost exclusively Norwegians and only male participants. Finally, a higher number of study participants would strengthen the findings and would also make it possible to adjust for more possible confounders.
Conclusions
Our study shows evidence that long-term AAS usage is associated with elevated levels of markers of inflammation and ECM remodelling, which seems to have a hormone-dependent (MMP-9) and hormone-independent (IL-8) association with markers of myocardial pathology in individuals using AAS. The causal relationship between these mediators is, however, still not known and should be addressed in future studies.
Supplementary material
Supplementary material is available at European Journal of Preventive Cardiology.
Author contribution
R.A., A.K.B., T.E., K.H.H., V.M.A., B.H., and I.G., contributed to the conception or design of the work and project administration. R.A., M.E.S., P.M.T., E.L.S., A.E.M., T.U., A.K.B., L.E.H., T.E., K.H.H., I.G., B.H., P.A., and V.M.A. contributed to the acquisition, analysis, or interpretation of data for the work. I.G., B.H., and P.A. drafted the manuscript. I.G., B.H., A.K.B., M.E.S., V.M.A, and P.A. critically revised the manuscript. All authors reviewed and approved the manuscript.
Funding
South-Eastern Norway Regional Health Authority (nos. 2019067 and 2021071 to B.H.; no. 2020047 to I.G.; and nos. 2016049, 2017025, 2018075, and 2020088 to A.K.B.), Norwegian Research Council (no. 309762), and Precision Health Center for Optimized Cardiac Care (ProCardio).
Data availability
The datasets used and/or analysed during the current study are available from the corresponding author on reasonable request.
References
Author notes
Conflict of interest: R.A. and L.E.H. have received speaker’s honoraria from Eisai; L.E.H. has received travel grants from ERN EpiCARE; P.M.T. has received consulting fees from Anti-doping Norge; K.H.H. has received a research grant from the Norwegian Research Council; B.H., A.K.B., and I.G. have received a research grant from the South-Eastern Norway Regional Health Authority. The rest of the authors report no conflict of interest.
Comments