-
PDF
- Split View
-
Views
-
Cite
Cite
Gian Luca Ragazzoni, Luna Cavigli, Elena Cavarretta, Silvia Maffei, Giulia Elena Mandoli, Maria Concetta Pastore, Serafina Valente, Marta Focardi, Matteo Cameli, Giovanni Di Salvo, Guido Pieles, Flavio D’Ascenzi, How to evaluate resting ECG and imaging in children practising sport: a critical review and proposal of an algorithm for ECG interpretation, European Journal of Preventive Cardiology, Volume 30, Issue 5, April 2023, Pages 375–383, https://doi.org/10.1093/eurjpc/zwac218
- Share Icon Share
Abstract
The athlete’s heart is a well-known phenomenon in adults practising competitive sports. Unfortunately, to date, most of the studies on training-induced cardiac remodelling have been conducted in adults and the current recommendations refer mainly to adult individuals. However, an appropriate interpretation of resting ECG and imaging in children practising sports is crucial, given the possibility of early detect life-threatening conditions and managing therapy and eligibility to sports competitions in the rapidly growing paediatric athlete population. While several articles have been published on this topic in adult athletes, a practical guide for the clinical evaluation of paediatric athletes is still missing. In this critical review, we provided a comprehensive description of the current evidence on training-induced remodelling in paediatric athletes with a practical approach for clinicians on how to interpret the resting 12-lead ECG and cardiac imaging in the paediatric athlete. Indeed, given that training may mimic potential cardiovascular disorders, clinicians evaluating children practising sports should pay attention to the risk of missing a diagnosis of a life-threatening condition. However, this risk should be balanced with the risk of overdiagnosis and unwarranted disqualification from sports practice, when interpreting an ECG as pathological while, on the contrary, it may represent a physiological expression of athlete’s heart. Accordingly, we proposed an algorithm for the evaluation of normal, borderline, and abnormal ECG findings that can be useful for the readers for their daily clinical practice.
Causes of SCD in children and the role of pre-participation evaluation
In the general population, sudden cardiac death (SCD) is the most common cause of death in adults (>35 years), with ischaemic heart disease being the most frequent cause.1 Although rare, SCD can also occur in children and adolescents. In this population, it accounts for 10% of mortality after the first year of age.2
The leading cause of SCD is highly dependent on age.3 In young adults, the most common cause of SCD is structural cardiac abnormalities,4 with hypertrophic cardiomyopathy (HCM) being the most common cause (36% relative incidence) in the United States5–7 and arrhythmogenic cardiomyopathy (ACM) in Europe.8,9 Conversely, in children and adolescents, the most common causes are the anomalous origin of the coronary artery, channelopathies, in particular long QT syndrome (LQTS), and congenital pre-excitation.4,10–13 Other causes of SCD include myocarditis, Marfan syndrome5 and, more rarely and mainly in athletes, commotio cordis, a phenomenon in which a sudden blunt impact to the chest causes sudden death in the absence of cardiac damage.10
The risk of SCD increases consistently in young individuals involved in competitive sports,14 and SCDs more frequently occur during exercise.15 The increased adrenergic stimulation during intensive training and competition may potentially trigger ventricular arrhythmias.16 Furthermore, exercise can accelerate the progression of certain cardiac diseases, such as ACM.17,18 However, SCD may also occur at rest, particularly in channelopathies, such as Brugada syndrome and LQTS3.11
The increased risk during sport has called for a pre-participation evaluation (PPE), focusing on the identification of underlying, concealed cardiovascular disorders. However, the best modalities to conduct the PPE of competitive athletes are still debated. Indeed, the current US recommendations include a questionnaire on the personal and family history of the athlete, focusing on cardiac symptoms (e.g. chest pain, palpitation, or syncope) and a family history of SCD. The physical examination includes blood pressure measurement, evaluation of cardiac murmurs, and the search for connective tissue disorders stigma (e.g. longer limbs, excessive joint elasticity19). Other tests are performed to exclude the potential life-threatening cardiovascular disorders if any abnormalities are found. In this protocol, 12-lead resting ECG is not included in the routine screening.
Conversely, in Europe, the PPE of young adults practising sports includes a 12-lead resting ECG.20 The resting ECG has shown its value in detecting HCM, with a specificity of 90% and a 77% greater power of detecting HCM than history and physical examination alone.6,8,21 Furthermore, resting ECG increased the sensitivity of the PPE not only for the identification of HCM but also for other structural heart diseases accompanied by ECG abnormalities, such as ACM.8 Furthermore, the use of ECG can increase the likelihood of detecting LQTS, Brugada syndrome, Wolf-Parkinson-White (WPW), and other cardiomyopathies such as, left ventricular non-compaction, and dilated cardiomyopathy, thus helping to prevent SCD.11
To date, the vast majority of the studies on the importance of resting ECG in the PPE of competitive athletes have been conducted in adults, with limited data collected in children practising competitive sports. However, an appropriate interpretation of resting ECG in paediatric athletes is crucial, given the possibility of early detect life-threatening conditions and managing therapy and eligibility to sports competitions in the rapidly growing paediatric athlete population.
Electrocardiographic evaluation
Resting ECG: normal vs. abnormal findings in children practising sport
The paediatric athlete’s ECG interpretation can be challenging due to the influence of growth and maturation, particularly because of the presence of T-wave inversion (TWI), that may be common and not necessarily associated with structural heart disease.22 Furthermore, although the resting ECG of paediatric athletes seems not to predominantly reflect exercise-induced morphological remodelling but maturation,23 incomplete right bundle branch block (RBBB), repolarization changes, and bradycardia can be observed more commonly in paediatric athletes rather than in sedentary children.23,24
The international criteria for ECG interpretation in competitive athletes have been validated in adults, and these recommendations cannot be directly transferred to paediatric athletes. Some authors applied the international criteria to paediatric athletes to evaluate their sensitivity and specificity in this population. High specificity was demonstrated in Black, Arabic, and Caucasian junior athletes (specificity 0.98 in Caucasian and 0.93 in Black and Arabic athletes, respectively), while the sensitivity was markedly lower than in adults (0.57 in Caucasian and 0.61 in Black and Arabic athletes, respectively): indeed, while 12-lead resting ECG was able to identify WPW and channelopathies, it failed to unmask cardiac anomalies that were revealed by echocardiography, such as structural congenital heart disease.13,24 Notably, in the study by McClean et al., the abnormal origin of the coronary artery and other structural abnormalities that could not be detected by resting ECG were included in the list of false-negative individuals, thus reducing the sensitivity of the international criteria.24 We, therefore, proposed (Figure 1) a revision of the international criteria for their application to children practising sports, based on the current evidence and clinical expertise. This proposal has been adapted to paediatric athletes and may differ from the classification of normal and abnormal ECG findings suggested for adult athletes.
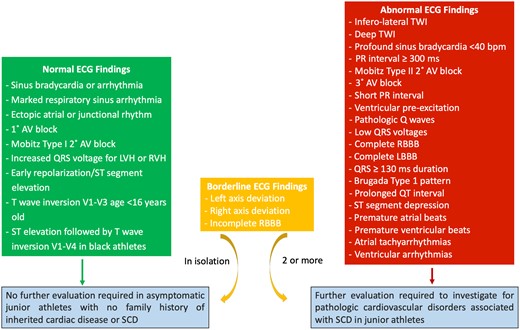
Proposal of revision of the international criteria for their application to paediatric athletes.
Compared with the international criteria proposed for adult athletes, the ECG findings deemed normal and thus not requiring further evaluation remain unchanged.
Among the borderline findings, we maintained the right and left axis deviation. Although incomplete RBBB is common in children who regularly practise sport (prevalence of incomplete RBBB = 25.8%, complete RBBB 0.5%25) and should not require further investigation, when associated with right axis deviation, it could be an indicator of right ventricular (RV) overload and should therefore require a careful interpretation.26 The ECG criteria for left atrial enlargement (LAE) had a poor diagnostic value in detecting LAE (AUC <0.60) in a population of 492 children27 and they were not deeply investigated in the population of paediatric athletes. Therefore, we removed ECG criteria for biatrial enlargement from the group of borderline abnormalities. Further studies are needed to investigate the sensitivity and specificity of these parameters in the population of paediatric athletes. The complete RBBB has been moved from normal to abnormal findings due to its correlation with right-sided lesions with volume loadings such as Epstein’s abnormality and atrial septal defects,26,28,29 Therefore, we suggest further evaluation (e.g. echocardiography) when a complete RBBB is found during the first clinical evaluation. Notably, a complete RBBB should be defined by the typical pattern and a QRS wider than 100 msec,30 contrary to the 120 msec recommended in adults.
Notably, the cut-offs of certain values have been adapted to children: indeed, resting heart rate (HR) decreases with ageing and is commonly high in children; accordingly, profound sinus bradycardia, arbitrarily defined as a resting HR below 30 b.p.m. for adult athletes, has been set at 40 b.p.m. under the age of 14 years, taking also into account recent evidences.31 Although the presence of I degree AV block in athletes has been deemed normal, an excessive prolongation should prompt further investigation. The cut-off for defining an excessive prolongation has been arbitrarily set at 400 msec in adult athletes. The PR interval in the paediatric population is shorter than in adults:30,32 accordingly, the cut-off has been moved from 400 to 300 msec.32–34 Similarly, the QRS duration requiring further investigation has been moved from 140 to 130 msec, given that the QRS widens with the growth and, in the population of children between 4 and 16 years of age, a normal QRS should be around 100 msec.30,32–34 The definition of normal and abnormal ECG findings adapted to the paediatric population are reported in Table 1. In Table 1, the definition of QTc interval is reported. According to the current recommendations, the QT interval should be corrected by the Bazett formula. However, in borderline cases, we suggest correcting the QT interval using both Bazett and Fridericia correction formulae, given that they are not interchangeable; furthermore, the Fridericia correction should be preferred in preadolescents with a resting HR ≥82 b.p.m.35
Resting electrocardiogram in junior athletes: peculiarities of definition and interpretation
ECG . | Definition . |
---|---|
Normal ECG findings | |
Juvenile T-wave pattern | T-wave inversion V1–V3 in athletes <16 years |
I degree AV block | PR interval longer than 200 msec but shorter than 300 msec |
Increased QRS voltage | Isolated QRS voltage criteria for left (SV1 + RV5 or RV6 > 3 mVa) or right ventricular hypertrophy (RV1 + SV5 or SV6 > 0.8 mV) |
Borderline ECG findings | |
Incomplete RBBB | rSR′ pattern in lead V1 and a qRS pattern in lead V6 with QRS duration <100 ms |
Left axis deviation | −9° to −90° |
Right axis deviation | >110° |
Abnormal ECG findings | |
Short PR interval | PR interval ≤90 ms |
Complete RBBB | rSR′ pattern in lead V1 and an S wave wider than R wave in lead V6 with QRS duration ≥100 ms |
Complete LBBB | QRS ≥100 ms, predominantly negative QRS complex in lead V1 (QS or rS) and upright notched or slurred R wave in leads I and V6 |
Prolonged QT interval | QTc ≥470 msec in high-level junior athletes |
QTc ≥460 msec | |
Profound sinus bradycardia | <40 beats per minute or sinus pauses ≥3 s |
Ventricular pre-excitation | PR interval ≤90 msec with a delta wave (slurred upstroke in the QRS complex) and wide QRS (≥90 ms) |
Profound, non-specific intraventricular conduction delay | Any QRS duration ≥130 ms |
Profound 1° AV block | PR interval ≥300 ms |
T-wave inversion | ≥1 mm in depth in two or more contiguous leads, excluding leads aVR, III, and V1 |
– Anterior | V2–V4 |
– excludes: black athletes with J-point elevation and convex ST segment elevation followed by TWI in V2–V4; athletes < age 16 with TWI in V1–V3; and biphasic T waves in only V3 | |
– Lateral | I and aVL, V5 and/or V6 (only one lead of TWI required in V5 or V6) |
– Inferolateral | II and aVF, V5–V6, I and aVL |
– Inferior | II and aVF |
ECG . | Definition . |
---|---|
Normal ECG findings | |
Juvenile T-wave pattern | T-wave inversion V1–V3 in athletes <16 years |
I degree AV block | PR interval longer than 200 msec but shorter than 300 msec |
Increased QRS voltage | Isolated QRS voltage criteria for left (SV1 + RV5 or RV6 > 3 mVa) or right ventricular hypertrophy (RV1 + SV5 or SV6 > 0.8 mV) |
Borderline ECG findings | |
Incomplete RBBB | rSR′ pattern in lead V1 and a qRS pattern in lead V6 with QRS duration <100 ms |
Left axis deviation | −9° to −90° |
Right axis deviation | >110° |
Abnormal ECG findings | |
Short PR interval | PR interval ≤90 ms |
Complete RBBB | rSR′ pattern in lead V1 and an S wave wider than R wave in lead V6 with QRS duration ≥100 ms |
Complete LBBB | QRS ≥100 ms, predominantly negative QRS complex in lead V1 (QS or rS) and upright notched or slurred R wave in leads I and V6 |
Prolonged QT interval | QTc ≥470 msec in high-level junior athletes |
QTc ≥460 msec | |
Profound sinus bradycardia | <40 beats per minute or sinus pauses ≥3 s |
Ventricular pre-excitation | PR interval ≤90 msec with a delta wave (slurred upstroke in the QRS complex) and wide QRS (≥90 ms) |
Profound, non-specific intraventricular conduction delay | Any QRS duration ≥130 ms |
Profound 1° AV block | PR interval ≥300 ms |
T-wave inversion | ≥1 mm in depth in two or more contiguous leads, excluding leads aVR, III, and V1 |
– Anterior | V2–V4 |
– excludes: black athletes with J-point elevation and convex ST segment elevation followed by TWI in V2–V4; athletes < age 16 with TWI in V1–V3; and biphasic T waves in only V3 | |
– Lateral | I and aVL, V5 and/or V6 (only one lead of TWI required in V5 or V6) |
– Inferolateral | II and aVF, V5–V6, I and aVL |
– Inferior | II and aVF |
AV, atrioventricular; LBBB, left bundle branch block; RBBB, right bundle branch block; TWI, T-wave inversion.
Reference values may differ according to sex.
Resting electrocardiogram in junior athletes: peculiarities of definition and interpretation
ECG . | Definition . |
---|---|
Normal ECG findings | |
Juvenile T-wave pattern | T-wave inversion V1–V3 in athletes <16 years |
I degree AV block | PR interval longer than 200 msec but shorter than 300 msec |
Increased QRS voltage | Isolated QRS voltage criteria for left (SV1 + RV5 or RV6 > 3 mVa) or right ventricular hypertrophy (RV1 + SV5 or SV6 > 0.8 mV) |
Borderline ECG findings | |
Incomplete RBBB | rSR′ pattern in lead V1 and a qRS pattern in lead V6 with QRS duration <100 ms |
Left axis deviation | −9° to −90° |
Right axis deviation | >110° |
Abnormal ECG findings | |
Short PR interval | PR interval ≤90 ms |
Complete RBBB | rSR′ pattern in lead V1 and an S wave wider than R wave in lead V6 with QRS duration ≥100 ms |
Complete LBBB | QRS ≥100 ms, predominantly negative QRS complex in lead V1 (QS or rS) and upright notched or slurred R wave in leads I and V6 |
Prolonged QT interval | QTc ≥470 msec in high-level junior athletes |
QTc ≥460 msec | |
Profound sinus bradycardia | <40 beats per minute or sinus pauses ≥3 s |
Ventricular pre-excitation | PR interval ≤90 msec with a delta wave (slurred upstroke in the QRS complex) and wide QRS (≥90 ms) |
Profound, non-specific intraventricular conduction delay | Any QRS duration ≥130 ms |
Profound 1° AV block | PR interval ≥300 ms |
T-wave inversion | ≥1 mm in depth in two or more contiguous leads, excluding leads aVR, III, and V1 |
– Anterior | V2–V4 |
– excludes: black athletes with J-point elevation and convex ST segment elevation followed by TWI in V2–V4; athletes < age 16 with TWI in V1–V3; and biphasic T waves in only V3 | |
– Lateral | I and aVL, V5 and/or V6 (only one lead of TWI required in V5 or V6) |
– Inferolateral | II and aVF, V5–V6, I and aVL |
– Inferior | II and aVF |
ECG . | Definition . |
---|---|
Normal ECG findings | |
Juvenile T-wave pattern | T-wave inversion V1–V3 in athletes <16 years |
I degree AV block | PR interval longer than 200 msec but shorter than 300 msec |
Increased QRS voltage | Isolated QRS voltage criteria for left (SV1 + RV5 or RV6 > 3 mVa) or right ventricular hypertrophy (RV1 + SV5 or SV6 > 0.8 mV) |
Borderline ECG findings | |
Incomplete RBBB | rSR′ pattern in lead V1 and a qRS pattern in lead V6 with QRS duration <100 ms |
Left axis deviation | −9° to −90° |
Right axis deviation | >110° |
Abnormal ECG findings | |
Short PR interval | PR interval ≤90 ms |
Complete RBBB | rSR′ pattern in lead V1 and an S wave wider than R wave in lead V6 with QRS duration ≥100 ms |
Complete LBBB | QRS ≥100 ms, predominantly negative QRS complex in lead V1 (QS or rS) and upright notched or slurred R wave in leads I and V6 |
Prolonged QT interval | QTc ≥470 msec in high-level junior athletes |
QTc ≥460 msec | |
Profound sinus bradycardia | <40 beats per minute or sinus pauses ≥3 s |
Ventricular pre-excitation | PR interval ≤90 msec with a delta wave (slurred upstroke in the QRS complex) and wide QRS (≥90 ms) |
Profound, non-specific intraventricular conduction delay | Any QRS duration ≥130 ms |
Profound 1° AV block | PR interval ≥300 ms |
T-wave inversion | ≥1 mm in depth in two or more contiguous leads, excluding leads aVR, III, and V1 |
– Anterior | V2–V4 |
– excludes: black athletes with J-point elevation and convex ST segment elevation followed by TWI in V2–V4; athletes < age 16 with TWI in V1–V3; and biphasic T waves in only V3 | |
– Lateral | I and aVL, V5 and/or V6 (only one lead of TWI required in V5 or V6) |
– Inferolateral | II and aVF, V5–V6, I and aVL |
– Inferior | II and aVF |
AV, atrioventricular; LBBB, left bundle branch block; RBBB, right bundle branch block; TWI, T-wave inversion.
Reference values may differ according to sex.
Finally, in young athletes, low QRS voltages tend to be infrequent: a study on more than 2200 young athletes, with a mean age of 18 years (16–25 years), showed a prevalence of low QRS voltages of 1.1%,36 while another study conducted in a population of older Olympic athletes (mean age: 25 years) demonstrated a prevalence of 4%.37 The presence of a low QRS voltage was associated with increasing age, elite status, body surface area (BSA), and body mass index. Given the possibility that they are even more infrequent in paediatric athletes and may reflect underlying cardiomyopathy, they were included for the first time among the abnormal findings.36,38
This proposal should be validated by further studies aimed at investigating the specificity and sensitivity of these criteria when applied to the population of paediatric athletes.
Common clinical scenarios when interpreting ECG in paediatric athletes
T-wave inversion
TWI can be the first and only manifestation of early cardiomyopathy. For this reason, it is of paramount importance to distinguish between a benign juvenile pattern and a possible sign of cardiomyopathy that requires further evaluation.
Contrary to adults, TWI is common in the paediatric population and is especially found in adolescent black and Arabic athletes.25
Anterior TWI (from V1 to V3) is relatively common in children (up to 35% of children between 5 and 11 years,39 with the percentage decreasing in older children22,40) and correlates with age and pubertal development.22,40 Therefore, anterior TWI in asymptomatic children without a family history of SCD or cardiomyopathies does not usually represent a sign of underlying structural heart disease and should not prompt further investigations in children <16 years41 or <15 years, according to other authors.22,40,42,43 The presence of extensive TWI (from V1 to V4) can also be considered normal, especially in black athletes, if associated with a J-point elevation of at least 0.1 mV. Therefore, these ECG findings should be interpreted as a normal variant in young adults and paediatric athletes,25 in the absence of symptoms and positive family history, defined as the presence of one or more first degree relatives with cardiomyopathy or SCD aged below 40 years. Conversely, the presence of TWI in older adolescents or the demonstration of TWI in the inferolateral leads should be carefully investigated to exclude underlying cardiomyopathies or a mitral valve prolapse.22,43 In some cases, inferolateral TWI seen in adolescents may be related to maturation and may disappear; therefore, beyond the first clinical evaluation, a strict follow-up should be performed in these cases.
Beyond the localization of TWI, clinicians should also pay attention to the presence of deep TWI, particularly with isoelectric ST segments and in the Caucasian population, because it infrequently occurs in adolescent athletes (0.8%) and may prompt further evaluation.44
Ventricular arrhythmias
Premature ventricular beats (PVBs) are common in the general population and competitive athletes,45 particularly paediatric athletes. Their prevalence varies with age; nearly 20–30% of otherwise normal adolescents have uncomplicated ventricular ectopy, usually decreasing or disappearing with growth.46–48
Nevertheless, ventricular arrhythmias may also represent a sign of underlying cardiomyopathy. For this reason, according to the current recommendations, the presence of PVBs should prompt further investigations.49 The first line tests should include an echocardiogram, exercise testing and a 12-lead ambulatory ECG monitoring. During the 24 h, it is essential to include the recording of a training session,45,50 aimed at evaluating the response of arrhythmias to exercise in a real setting, rather than only during the monitoring of an incremental ECG testing that may not completely reflect the exercise performed by the athlete. Although only 6% of normal adolescents have more than 500 PVBs per 24 h,48 the general number of ectopy alone is not sufficient to determine whether the arrhythmias are associated or not with a pathological substrate.51
Conversely, a qualitative analysis of the PVBs, particularly their morphology and response to exercise, should be conducted to unmask life-threatening diseases. Indeed, the morphology of the PVBs can provide critical information for the diagnosis and prognosis: in young athletes, the morphology and complexity of PVBs, but not their number, predicted the probability of an underlying disease.51 Furthermore, the response of ventricular arrhythmias to exercise is relevant in this population, considering that, in paediatric athletes, benign and common PVBs tend to decrease or disappear with exercise, particularly in the case of infundibular PVBs. In Table 2, we propose to adopt for paediatric athletes the classification of PVBs into common and uncommon, according to their characteristics, recommended for young athletes.49,50 Particularly in children, the common morphology of PVBs often observed, in the absence of structural heart disease, are the infundibular pattern, with a left bundle branch block (LBBB) morphology and inferior axis, and the fascicular pattern, with an RBBB morphology and a narrow QRS (<130 ms).45,50 These arrhythmias tend to disappear with growth and are associated with a good prognosis.45,48,52 Therefore, in the case of normal first-line evaluation and no family history for SCD, the asymptomatic junior athlete should not undergo further evaluation, be eligible for competitive sports,45 and do not usually require treatment. A strict follow-up, including 12-lead ambulatory ECG monitoring, is recommended to evaluate whether PVBs disappear with the growth and complete pubertal development. On the contrary, paediatric athletes showing PVBs with uncommon characteristics need to be further evaluated, even if the first-line tests are normal.45,49,50
. | Common . | Uncommon . |
---|---|---|
PVBs characteristics | ||
Morphology | LBBB/inferior axis | RBBB and wide QRS |
RBBB and narrow QRS | LBBB/int. or superior axis | |
Response to exercise | Decrease/suppression | Persistence/increase |
Complexity | Isolated, monomorphic | Repetitive, polymorphic |
Short coupling interval (R on T) | No | Yes |
Clinical findings | ||
Symptoms | No | Yes |
Family history of premature SCDa or cardiomyopathy | No | Yes |
ECG abnormalities | No | Yes |
Imaging abnormalities | No | Yes |
. | Common . | Uncommon . |
---|---|---|
PVBs characteristics | ||
Morphology | LBBB/inferior axis | RBBB and wide QRS |
RBBB and narrow QRS | LBBB/int. or superior axis | |
Response to exercise | Decrease/suppression | Persistence/increase |
Complexity | Isolated, monomorphic | Repetitive, polymorphic |
Short coupling interval (R on T) | No | Yes |
Clinical findings | ||
Symptoms | No | Yes |
Family history of premature SCDa or cardiomyopathy | No | Yes |
ECG abnormalities | No | Yes |
Imaging abnormalities | No | Yes |
LBBB, left bundle branch block; PVBs, premature ventricular beats; RBBB, right bundle branch block; SCD, sudden cardiac death.
Premature sudden cardiac death (SCD) is defined as that occurring before 40 years old in males and before 50 years old in females.
. | Common . | Uncommon . |
---|---|---|
PVBs characteristics | ||
Morphology | LBBB/inferior axis | RBBB and wide QRS |
RBBB and narrow QRS | LBBB/int. or superior axis | |
Response to exercise | Decrease/suppression | Persistence/increase |
Complexity | Isolated, monomorphic | Repetitive, polymorphic |
Short coupling interval (R on T) | No | Yes |
Clinical findings | ||
Symptoms | No | Yes |
Family history of premature SCDa or cardiomyopathy | No | Yes |
ECG abnormalities | No | Yes |
Imaging abnormalities | No | Yes |
. | Common . | Uncommon . |
---|---|---|
PVBs characteristics | ||
Morphology | LBBB/inferior axis | RBBB and wide QRS |
RBBB and narrow QRS | LBBB/int. or superior axis | |
Response to exercise | Decrease/suppression | Persistence/increase |
Complexity | Isolated, monomorphic | Repetitive, polymorphic |
Short coupling interval (R on T) | No | Yes |
Clinical findings | ||
Symptoms | No | Yes |
Family history of premature SCDa or cardiomyopathy | No | Yes |
ECG abnormalities | No | Yes |
Imaging abnormalities | No | Yes |
LBBB, left bundle branch block; PVBs, premature ventricular beats; RBBB, right bundle branch block; SCD, sudden cardiac death.
Premature sudden cardiac death (SCD) is defined as that occurring before 40 years old in males and before 50 years old in females.
Echocardiographic evaluation
LV remodelling
The physiological determinants of the exercise-induced cardiac remodelling in the athlete’s heart include age, ethnicity, sex, sports discipline, training volume and intensity, BSA, lean body mass, and years of training.25,53 The four cardiac chambers in the fully developed athlete’s heart may be homogeneously dilated due to training-induced adaptation associated with an increased stroke volume, coupled with a symmetric increase in the wall thickness and left ventricular (LV) mass. In the paediatric athlete’s heart, the adaptive changes of the LV are still present when compared with sedentary controls (LV end-diastolic diameter +8.2%, LV end-systolic diameter +14.2%, interventricular septum thickness +12.9%, posterior wall thickness +12.2%, LV mass +27.6%, and left atrial diameter +12.3%), although they are less evident as compared with adult athletes.25 Contrary to previous beliefs, exercise-induced adaptations occur early, even in preadolescent athletes and in a relatively short-time period.25,54 In 13 naive male adolescents, all with baseline LV findings falling within normal ranges (within two standard deviation of population mean), a 10-week endurance training programme (8–10 supervised hours of rowing training weekly) was sufficient to cause exercise-induced LV remodelling with a significant increase in wall thickness (8.0 ± 0.7 vs. 9.2 ± 0.5 mm, P < 0.001), LV end-diastolic volume (114 ± 20 vs. 127 ± 23 mL, P < 0.001), and LV mass (122 ± 21 vs. 140 ± 22 g, P < 0.001).55 In this small population, only 1 subject showed post-training general population Z-scores for posterior wall thickness and LV mass 2.6 and 2.3, respectively, but 85% of the study population has increased in at least 1 parameter of Z-score >1 and 38% of the population demonstrated Z-score increase of two standard deviation on the general population nomogram. When using reference values specific for the athletic population, the maximum individual post-training Z-score was 1.8.56
In preadolescent athletes, the initial training-induced remodelling is predominantly characterized by a small increase in the LV wall thickness, mass and relative wall thickness (RWT), with a predominantly form of concentric LV remodelling.57 A cardiac magnetic resonance (CMR) study in preadolescent soccer players confirmed the initial training-induced LV concentric remodelling without significantly increasing LV volumes.58 In the 3-year follow-up of the same population of endurance skiers, continued endurance training determined an increase in LV end-diastolic volume without significant changes in LV wall thickness and mass.59 In terms of LV geometry, the progressive LV chamber dilatation, without an additional increase in LV wall thickening at the age of 15 years, resulted in a normalization of the RWT59 and then in a subsequent eccentric remodelling.60 The adolescent female athletes showed a less pronounced degree of LV remodelling and hypertrophy than males, as shown by echocardiographic61 and CMR studies.62
Both the systolic and diastolic LV function remain normal at rest and during maximal efforts, although a greater stroke volume index, cardiac output, and a higher e′ velocity have been described at maximal effort in relation to a greater allometrically scaled LV end-diastolic volume in preadolescent soccer players in comparison with sedentary controls.54 Interestingly, the training-induced changes in the cardiac mechanics observed in greater circumferential strains, twist and faster diastolic lengthening occur without significant changes in the LV wall thickness but only in the LV end-diastolic volume index.63 A 3-year longitudinal study by the same authors demonstrated that the training-induced increase in LV function during submaximal exercise is independent of growth and sexual maturation.64 Notably, the increase in cardiac output due to endurance training in peri-pubertal athletes is also mediated by a progressive decrease in the HR.33,65
In terms of ethnicity, black junior athletes revealed the greatest LV wall thickness than white and mixed-ethnicity athletes. Nevertheless, LV thickness >12 mm of hypertrophy was present only in 7.1% of black athletes, 5.9% of mixed-race, and 1.3% of white athletes.66 Mixed-race athletes demonstrated LV wall thickness and dimensions that fell between the black and the white athlete groups.66 Therefore, it is crucial for adolescent athletes to correctly scale the LV dimensions and wall thickness, particularly when follow-up data are needed due to the rapid growth of stature. In paediatric athletes, reference values and the corresponding Z-score equations have been proposed to correctly interpret exercise-induced cardiac remodelling.56,67 Currently, it seems reasonable in athletes to define a mild increase in LV dimensional parameters on the basis of the Z-score for the general population, being between 2 and 2.5.
Right ventricular remodelling
RV remodelling has been extensively described in highly trained adult athletes;7,68–74 however, data collected in preadolescent and adolescent athletes are few.59,60,75,76 Unfortunately, evidence obtained in adults cannot be transferable directly to paediatric athletes, as cardiac adaptation in young individuals is a dynamically changing process with a progressing remodelling of the young athlete’s heart into a complete structural adaptation.62 However, to date, a morphological RV remodelling, as a consequence of intensive exercise, has also been demonstrated in preadolescent and adolescent athletes.59,62,75–78 Indeed, in a longitudinal study, male competitive swimmers (aged 10.8 ± 0.2 years) were evaluated with echocardiography before (baseline) and after 5 months of the training (peak-training) and compared to age- and sex-matched non-athlete children evaluated at baseline and after 5 months of natural growth.75 Both trained and sedentary groups significantly increased their height, weight, and BSA after 5 months, but RV dimensional remodelling was more evident in endurance-trained children, suggesting that intensive training has an additive effect on the growth of RV size.75 Similarly, in a study with a longer follow-up, in junior endurance athletes (baseline at 12 years and follow-up at 15 years and 18 years), there was a trend toward greater indexed RV end-diastolic and end-systolic areas in the active endurance athletes at follow-up than in the former athletes, with comparable values at baseline.59,60 There were moderate, positive correlations between weekly hours of endurance training and changes in RV areas.59
CMR studies confirmed these findings, demonstrating a correlation between training volume and RV dilatation in healthy adolescent athletes.62 Comparing preadolescent soccer players (mean age 10.1 ± 1.4 years) with age-matched controls, the formers had significantly higher RV indexed volumes at CMR, suggesting that RV may be prone to remodelling not only in the lifetime of athletes but also at the very beginning of the sporting life.
Unfortunately, RV dilatation is also a phenotypic expression of ACM.80 Therefore, reference ranges have been proposed for athletes to help differentiating physiological conditioning from ACM but mainly for adults,72,81–83 while robust data in the paediatric population are missing80 and current adult imaging criteria for echocardiography and CMR are not sensitive enough in the paediatric population.84,85 A previous longitudinal study in adult athletes demonstrated that, while global RV size increased after training, right ventricle out-flow tract (RVOT) diameters did not change, supporting the usefulness of RVOT diameters in differentiating between ACM and athlete’s heart.69 Conversely, in another study on young athletes, while the percentage of changes in right ventricular end-diastolic diameters was similar between preadolescent and adult athletes, RVOT diameters increased to a greater extent in the former.75 Therefore, although none of the children exhibited RV wall motion abnormalities, exercise-induced RV remodelling in highly trained preadolescent athletes may hamper the interpretation of exercise-induced physiological adaptation of the right heart in athletes, with a potential risk of ACM overdiagnosis.75,80
The studies conducted on paediatric athletes demonstrated normal RV functional parameters, including E/A ratio, tricuspid annular plane systolic excursion (TAPSE), s′ velocity, global RV strain, RV fractional area change, and RV ejection fraction.59,62,75–77 In a study conducted in long-term endurance-trained adolescents, RV s′ and TAPSE were higher, whereas RV longitudinal strain and fractional area change did not differ between athletes and age- and sex-matched sedentary controls.76 As described for the basal and RV mid-segments in adult elite athletes,86 lower RV mid-segment strain values were also found in young athletes as compared with controls; however, these values were within normal limits, indicating that functional differences may exist in various segments of the RV, in the absence of structural heart disease.76
Overall, these findings support the hypothesis that RV function is not impaired by exercise training also in the early phase of the sports career of an athlete and RV dilatation is a physiological adaptation of the heart in asymptomatic individuals with an otherwise normal echocardiographic examination, normal ECG findings, and negative family history for SCD.75,76
Wall motion abnormalities are not a common training-related finding and should prompt further investigations to unmask an underlying structural heart disease. Indeed, regional wall motion abnormalities may represent an early sign of cardiomyopathy affecting the left and/or the right ventricle and should not be interpreted as a physiological adaptation to exercise.
Biatrial remodelling
In athletes, training-induced biatrial remodelling has been questioned because of its potential role as a substrate for supraventricular tachyarrhythmias.87 However, in healthy athletes, exercise-induced atrial enlargement is accompanied by normal reservoir function and phasic volumes,88,89 suggesting that atrial dysfunction is uncommon in athletes and should be regarded as an early sign of cardiovascular disorders. Unfortunately, few data are currently available on paediatric athletes. A longitudinal study on endurance junior athletes found that indexed biatrial volumes increased after 5 months of training while maintaining a normal atrial function, as demonstrated by speckle-tracking and three-dimensional echocardiography. Similar data were also observed when CMR was applied to investigate cardiac remodelling in preadolescent athletes. This preliminary evidence suggests that training may influence the growing heart of paediatric athletes, with an additive increase in biatrial size, suggesting that morphological adaptations can also occur in the early phases of the sports career. Training-induced remodelling was associated with a preserved biatrial function, supporting the hypothesis of a physiological remodelling. Further studies are needed to confirm this evidence and further investigate the influence on the biatrial remodelling of factors such as type of sport, sex, and ethnicity.
During the echocardiographic evaluation of children practising sports, in addition to the classic echocardiographic measurements, it is essential to evaluate, among other characteristics, the atrioventricular connection, the venous return connection, and the presence of coronary artery abnormalities. The most important structures to visualize during an echocardiographic evaluation in paediatric athletes are summarized in Table 3.
What to check in a paediatric echocardiogram . | |
---|---|
Situs of the heart |
|
Atria |
|
Ventricles |
|
Aortic valve |
|
Coronary arteries |
|
Aorta |
|
Pulmonary arteries |
|
What to check in a paediatric echocardiogram . | |
---|---|
Situs of the heart |
|
Atria |
|
Ventricles |
|
Aortic valve |
|
Coronary arteries |
|
Aorta |
|
Pulmonary arteries |
|
What to check in a paediatric echocardiogram . | |
---|---|
Situs of the heart |
|
Atria |
|
Ventricles |
|
Aortic valve |
|
Coronary arteries |
|
Aorta |
|
Pulmonary arteries |
|
What to check in a paediatric echocardiogram . | |
---|---|
Situs of the heart |
|
Atria |
|
Ventricles |
|
Aortic valve |
|
Coronary arteries |
|
Aorta |
|
Pulmonary arteries |
|
Future perspectives
The population of paediatric athletes presents some relevant challenges during the PPE because of the presence of potential confounding factors, such as sexual maturation and growth that may influence the interpretation of clinical data. The body of evidence is growing, although studies investigating the sensitivity and specificity of clinical parameters and setting specific cut-offs in this population are limited. In this review, we proposed a revision of the international criteria for their application to children practising sports, based on the current evidence and clinical expertise. This proposal has been adapted to paediatric athletes and may differ from the classification of normal and abnormal ECG findings suggested for adult athletes. Future studies are warranted to validate this proposal in paediatric athletes.
Conclusion
Paediatric athletes represent a specific population in which the interpretation of clinical data, specifically ECG, arrhythmias, and imaging, should be carefully conducted. The unique opportunity of performing a PPE to detect underlying conditions posing the young athlete at risk of SCD should be balanced with the risk of overdiagnosis and unwarranted disqualification from sports practice. The growing evidence suggests that the electrical and structural remodelling of the heart occurs early in the career of a competitive athlete, although differences exist in the degree of adaptation in comparison with adult athletes. Sexual maturation, years of training, training volume, and intensity are some of the factors that may influence this peculiar adaptation, highlighting the importance to conduct specific research projects and publish recommendations dedicated to the population of paediatric athletes. Further research is needed to increase the body of evidence and to validate our proposals for the classification of normal and abnormal ECG findings in paediatric athletes.
Authorship
G.L.R., L.C., E.C., and G.P. contributed to the conception or design and drafted the manuscript. F.D. contributed to the conception or design, drafted and critically revised the manuscript. S.M., G.E.M., M.C.P., S.V., M.F., M.C., and G.D.S. contributed to the interpretation and critically revised the manuscript. All gave final approval and agree to be accountable for all aspects of work ensuring integrity and accuracy.
References
Author notes
Conflict of interest: None declared.
Comments