-
PDF
- Split View
-
Views
-
Cite
Cite
Corina E Schaer, Thomas U Wüthrich, Volker M Koch, Jörn Justiz, Diego Stutzer, Damien Maurer, Fernando G Beltrami, Christina M Spengler, Prediction of whole-body V˙O2peak using a novel incremental respiratory test, European Journal of Preventive Cardiology, Volume 29, Issue 12, September 2022, Pages 1707–1709, https://doi.org/10.1093/eurjpc/zwac064
- Share Icon Share
The peak oxygen consumption (V˙O2peak) is a strong predictor of mortality1 and perioperative cardiopulmonary complications.2 While incremental exercise tests are required to determine V˙O2peak, intense whole-body exercise may not be feasible for patients who, for example, suffer from muscular or joint problems of different aetiologies or that are bed-ridden. To circumvent the need for exercise, attempts have been made to predict V˙O2peak based solely on anthropometric data such as height, weight, sex, and vital capacity3 or the ratio between resting heart rate (HRrest) and predicted maximal heart rate (HRmax).4
Since it is known that the level of respiratory muscle performance is related to the level of physical fitness,5 we hypothesized that adding a functional measurement of respiratory muscles might further improve the accuracy of V˙O2peak prediction. To this end, we tested whether performance from an incremental respiratory muscle test (IncRMT) recently developed in our laboratory,6 combined with anthropometric and non-exercise-related individual characteristics, would improve V˙O2peak prediction in healthy subjects.
Thirty-six healthy subjects (18m/18f, age 26.1 ± 5.3 years, height 172 ± 8 cm, weight 66 ± 10 kg, V˙O2peak 48.9 ± 10.1 mL min−1 kg−1) gave written informed consent to participate in this study, which was approved by the local ethics committee. On the first visit, lung function, maximal inspiratory pressure, and maximal expiratory pressure were measured according to current guidelines. After 15 min of rest, subjects performed an incremental cycling test until volitional exhaustion, with load increments of 30 W every 2 min until exhaustion. Ventilation and gas exchange were measured breath by breath by the metabolic cart (Oxycon Pro; Jaeger, Höchberg, Germany). Heart rate (HR) and oxygen saturation were recorded (Nellcor; Covidien, Mansfield, MA, USA) and aligned with metabolic cart data. V˙O2peak was defined as the highest 30 s V˙O2 average in the cycling test. After another 15 min rest, participants were familiarized with the IncRMT protocol and instrumentation, without performing an all-out test. On the second visit, HRrest was measured following 12 min of supine rest on a stretcher and participants performed a second IncRMT to exhaustion for further familiarization. The HRmax was predicted from age using the formula of Tanaka et al.,7 i.e. HRmax = 208 − 0.7 age (years). On the third visit, the IncRMT was performed. In a sitting position, participants breathed through a mouthpiece connected to a self-built breathing apparatus while wearing a nose clip. Breathing frequency (fB) started at 16 breath min−1 with an increase of 2 breaths min−1 every 2 min, whereas tidal volume was set constant at 60% of forced vital capacity (FVC). For every 2 min, breathing resistance was increased to achieve, together with the increase in fB, a 10% increase in work of breathing (WOB). The test was terminated if subjects stopped voluntarily or after a third warning due to failing to maintain tidal volume or fB within target limits. The estimation of WOB was performed using mouth pressures, and a full description of the test and calculations can be found elsewhere.6
Work of breathing, IncRMT duration (Tlim), HRrest, HRrest HRmax−1, and FVC were used in a backward stepwise multiple regression analysis to predict V˙O2peak. Based on the results from Jones et al.,3 we chose to use FVC as a substitution of sex, height, weight, and age. Other measures of lung function or respiratory muscle strength were excluded from the model due to collinearity with FVC. In addition, HRrest and HRmax HRrest−1 were added based on the study by Uth et al.4 In addition to the coefficient of determination (R2), standard error of the estimate (SEE) for the differences between measured and estimated V˙O2peak was calculated. Statistical significance was set at P < 0.05.
Forced vital capacity as single variable was a statistically significant predictor of V˙O2peak [R2 = 0.634, F(1,34) = 59.0, P < 0.001, SEE = 441 mL min−1], whereas FVC in combination with WOBtot increased R2 significantly by 0.153 [R2 = 0.787, F(1,34) = 125.4, P < 0.001, SEE = 373 mL min−1]. No other parameter affected V˙O2peak prediction (Tlim, P = 0.313; HRrest, P = 0.341; HRmax HRrest−1, P = 0.385). The final model using FVC and WOBtot was V˙O2peak, pred = 105.9 WOB + 613.5 FVC − 442.1. Figure 1 shows predicted V˙O2peak plotted against measured V˙O2peak and Figure 2 shows Bland–Altman plots for both the prediction of FVC alone and FVC + WOB. Agreement between predicted and measured V˙O2peak revealed a bias of 0 ± 546 mL min−1 for the single-variable model with FVC only and a bias of −18 ± 417 mL min−1 for the model including FVC and WOBtot.
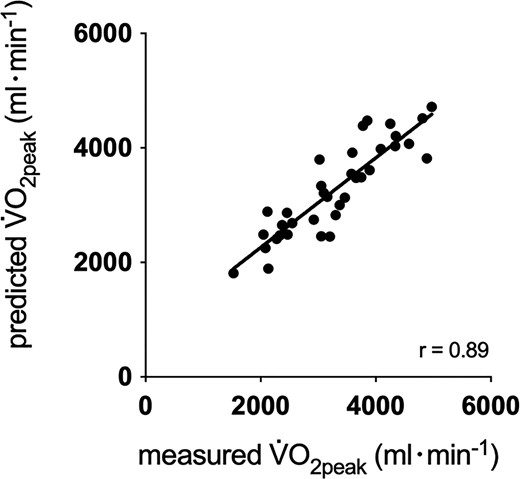
Measured vs. predicted V˙O2peak using total work of breathing and forced vital capacity.
Our results confirm prior research showing that FVC as a single variable is a useful predictor V˙O2peak3 but further indicates that adding a measure of functional capacity of the respiratory muscles can significantly improve the prediction (24% reduction in limits of agreement). Baseline measures of cardiovascular fitness, quantified with HRrest and HRmax HRrest−1 did not improve V˙O2peak prediction. In fact, the ratio of HRmax HRrest−1 as a single variable was a poor predictor of V˙O2peak (R2 = 0.109), in contrast to previous findings.4 It remains to be tested, however, to which degree this prediction needs adaptation for cardiac patients. Although the measure of respiratory muscle work capacity serves as an index of peripheral muscular function, and therefore of O2 extraction capacity, there is a smaller challenge to O2 delivery, which is likely a more prominent limiting factor to the V˙O2peak of cardiac patients .
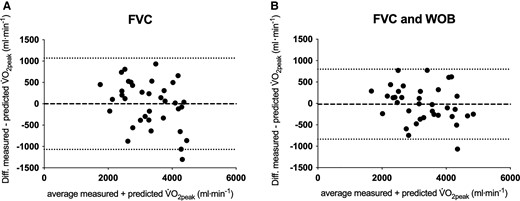
Bland–Altman plots using the average vs. the difference of measured and predicted peak oxygen consumption (V˙O2peak). (A) Single-variable prediction of V˙O2peak with forced vital capacity, (B) multivariable prediction of V˙O2peak with the combination of forced vital capacity and total work of breathing. Diff., difference.
Adding a measure of functional respiratory muscle capacity produced superior estimation of V˙O2peak than, for example, attempts using physical activity questionnaires.8 Indeed, the present 95% limits of agreement are in line with those reported in different populations using sub-maximal incremental tests up to level 17 on the Borg 6–20 rate of perceived exertion (RPE) scale in which measured V˙O2 was extrapolated to V˙O2peak at an RPE of 20.9 It must be acknowledged, however, that an SEE of 441 mL min−1 for V˙O2peak is still too large for clinical use, and further optimization of this prediction is needed. To this end, it is also vital to test whether the current relationship stands when participants are not pre-familiarized with the protocol on a separate day, as would likely be the case in a clinical setting. Nonetheless, given that in addition to V˙O2peak, the function of the respiratory muscles per se is an important predictor of overall post-operative complications,10 it is possible that our test might be suitable to identify at-risk patients beyond using V˙O2peak alone.
Authors’ contributions
C.M.S., C.E.S., T.U.W., and F.G.B. contributed substantially to conception and design of the work, data acquisition, analysis, and interpretation.
V.M.K., J.J., D.S., D.M., C.E.S., and C.M.S. contributed substantially to the design development and manufacturing of the testing apparatus as well as the associated data analysis. C.E.S., F.G.B., and C.M.S. drafted the manuscript, and V.M.K., J.J., D.S., and D.M. critically revised it. All gave final approval and agreed to be accountable for all aspects of work.
Acknowledgements
We would like to thank Adrian Sallaz, Markus Lempen, Bernard Schmutz, and Daniel Debrunner (Bern University of Applied Sciences, Department of Engineering and Information Technology, Switzerland) for contributing their engineering expertise. We are grateful to Andjela Cuic and Isabelle Manzoni (Exercise Physiology Lab, ETH Zurich, Zurich, Switzerland) for preliminary work to this study and to Desirée Erni, Dina Tageldin, and Donat Roduner (Exercise Physiology Lab, ETH Zurich, Zurich, Switzerland) for assisting with data collection.
Funding
Funding was provided by Zürcher Kantonalbank (ZKB), the Commission for Technology and Innovation (CTI, project no. 15650.1 PFLS-LS), and idiag AG (Fehraltorf, Switzerland).
Ethics approval and consent to participate
The study was approved by the ethics committee of ETH Zurich and was performed according to the Declaration of Helsinki 2008. Subjects gave their written informed consent to participate in this study.
Data Availability
The data sets used and/or analysed during the present study are available from the corresponding author on reasonable request.
References
Author notes
Conflict of interest: none declared.
Comments