-
PDF
- Split View
-
Views
-
Cite
Cite
Marlus Karsten, Elisabetta Salvioni, Pietro Palermo, Irene Mattavelli, Marco Scatigna, Massimo Mapelli, Giulia Grilli, Beatrice Pezzuto, Anna Apostolo, Gustavo dos Santos Ribeiro, Piergiuseppe Agostoni, Periodic breathing during exercise in heart failure: beyond the classic risk factors, European Heart Journal Supplements, Volume 27, Issue Supplement_1, February 2025, Pages i103–i108, https://doi.org/10.1093/eurheartjsupp/suae091
- Share Icon Share
Abstract
Periodic breathing is a peculiar ventilatory pattern in patients with heart failure (HF), characterized by cyclic oscillations in minute ventilation. This phenomenon has been observed in awake patients, during sleep, and during exercise. Periodic breathing during exercise, also known as exercise oscillatory ventilation (EOV), is an important marker of clinical and functional status, morbidity, and mortality in HF patients. However, a clear understanding of the meaning, causes, and possible occurrence of different forms of EOV is still currently lacking. This review focuses on what is known, likely known, or unknown about EOV in HF, including topics such as the definition of EOV, pathophysiological mechanisms, EOV phenotypes, treatment modalities, and the prognostic role of EOV in patients with HF. Finally, some perspectives on research opportunities and clinical practice are presented, to increase the knowledge of EOV, and the application of tools for its assessment, which may improve the chances of identifying and treating EOV, with a positive impact on patients with HF.
Introduction
Periodic breathing is a peculiar ventilatory pattern in patients with heart failure (HF), characterized by cyclic oscillations in minute ventilation with or without interposed apnoea. Its presence has been observed in awake patients (Cheyne–Stokes respiration), during sleep (central sleep apnoea), or during incremental exercise.1 Periodic breathing during exercise, also known as exercise oscillatory ventilation (EOV), is common in patients with advanced HF.2,3 Although several reports have addressed EOV in HF, a clear understanding of the meaning, causes, and possible presence of different forms of EOV is currently lacking. This review focuses on what is actually known, likely known, or unknown about EOV in HF.
Definition
Several definitions of EOV have been reported making comparisons between different studies difficult. In fact, a systematic review found four original definitions and several definitions based on combinations of the originals or that using different methods to identify EOV.4 This study also found an average EOV prevalence of 26.6%, ranging from 7% to 64% based on different definitions and sample characteristics. In another study, by Ribeiro et al.,5 the authors investigated the application of the three most commonly used definitions in a sample of HF patients and found different sensitivities and specificities among the EOV definitions for predicting 2-year major adverse cardiovascular outcomes. The classical EOV definitions that have been used to assess EOV are based on concepts that consider characteristics of the ventilatory pattern (amplitude, cycle length, and/or oscillation time).4,5
Figure 1 shows a representative exercise ventilation curve of an individual with HF and EOV and the illustrative criteria of five definitions. Briefly, Ben-Dov et al.6 proposed that EOV is characterized by two or more consecutive cycles with a mean minute ventilation (VE) ≥ 25% of the cycle and a cycle length between 30 and 60 s. Kremser et al.7 suggested that ventilatory oscillations should occur at least 66% of the total exercise time and have a cycle amplitude > 15% of the resting VE. Similarly, Corrà et al.8 suggested that ventilatory oscillations should occur for at least 60% of total cardiopulmonary exercise test (CPET), with the same criteria for cycle amplitude. Leite et al.9 suggested the presence of three or more regular oscillations with a standard deviation of length < 20% of the cycle average and a cycle amplitude greater than 5 L min−1. Finally, Sun et al.10 proposed the presence of three or more consecutive oscillatory cycles with a VE average ≥ 30% of the cycle and a cycle length between 40 and 140 s. In addition, oscillations must be present in at least three other CPET variables (Figure 1).
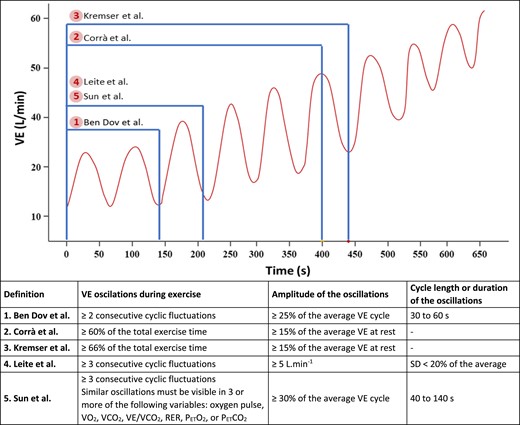
Representative exercise ventilation curve of a person with heart failure and exercise oscillatory ventilation and illustrative criteria of five exercise oscillatory ventilation definitions. *VO2, oxygen uptake; PETCO2, end-tidal pressure of carbon dioxide; PETO2, end-tidal pressure of oxygen; RER, respiratory exchange ratio; VCO2, carbon dioxide production; VE/VCO2, minute ventilation to carbon dioxide production ratio; VE, minute ventilation.
Pathophysiology
Exercise oscillatory ventilation is a complex phenomenon observed mainly in HF patients with reduced ejection fraction (HFrEF) or mid-range ejection fraction, although it occurs at different times during follow-up.3 Although the characteristic oscillatory pattern of this phenomenon is known, the factors that trigger it are not yet fully understood.1,11 The model developed to explain its pathophysiology is mainly based on research in patients with central sleep apnoea and Cheyne–Stokes respiration and is based on four adjacent and complementary physiological changes: (i) circulatory delay, (ii) pulmonary congestion, (iii) chemoceptor hyperactivity, and (iv) ergoreflex exacerbation.1,11 Indeed, these mechanisms are part of the pathophysiology of HF and may be related to the occurrence of EOV. However, it is not clear to what extent they could influence the development and worsening of the phenomenon.
Circulatory delay would be the main factor involved in this response, as the lower cardiac output observed in patients with HF causes an increase in pulmonary circulation time and consequently delayed feedback and changes in ventilatory drive to attempt to maintain acid-base balance during exercise.12 Indeed, it has been shown that EOV would be more strongly associated with impairments in haemodynamic function in response to high ventricular filling pressures and circulatory delay, with some implications for pulmonary congestion.13 This hypothesis has been supported in experimental models and clinical studies in resting patients. Of note, in left ventricular assist device (LVAD) recipients, an increase of LVAD speed, which implies an increase of cardiac output, reduces apnoea/hypopnoea during sleep as well as EOV, although the value of cardiac output at rest was not different between patients with and without EOV.14
The second factor in the model that strongly contributes to the pathophysiology of EOV is pulmonary congestion. Indeed, an increased pressure gradient in the left ventricle would increase alveolar pressure, which would stimulate J receptors in response to C fibre stretch.15,16 This mechanism would stimulate the ventilatory drive. However, there would be superficial hyperventilation in response to reduced pulmonary compliance, reduced arterial carbon dioxide pressure (PaCO2), which could lead to apnoea.16,17 In addition, one study proposed that the gas exchange characteristics observed during periodic breathing during exercise could be explained by primary ventilatory oscillations.18 The authors also suggested that periodic fluctuations in reflex control—secondary to increased chemoreflex sensitivity and instability of blood gas stores—and slowed haemodynamics could explain the ventilatory oscillations.18
These circulatory and ventilatory effects would be exacerbated by the instability of the ventilatory drive caused by both the exacerbated ergoreflex response observed in the peripheral muscles of patients with HF and the greater chemoreceptor activity during exercise due to sympathetic hyperactivity.19 The model proposed to explain the mechanism causing EOV is summarized in Figure 2.
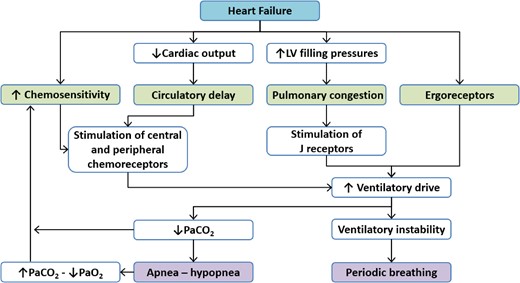
Mechanisms of generation of exercise oscillatory ventilation in patients with heart failure. *LV, left ventricular; PaO2, arterial partial pressure of oxygen; PaCO2, arterial partial pressure of carbon dioxide (adapted from Dhakal and Lewis16).
Prognostic role of exercise oscillatory ventilation
Several studies have shown a worse prognosis in patients with EOV compared with those without this phenomenon.3,9,17,20–22 Cardiovascular death, length of hospital stay, LVAD, and urgent heart transplantation are some of the outcomes that have been associated with the presence of EOV. A landmark study examined the clinical impact of EOV on mortality in patients with an indication for heart transplantation who were followed until death (mean follow-up: 49.7 months).9 The authors observed reduced short-term (1 year) and medium-term (2 years) survival in patients with EOV, with a three-fold higher risk of death than in patients without EOV.
Another study followed 156 patients with systolic HF for 50 months, stratifying deaths by pump failure or sudden death.20 The authors found that EOV was present in 100% of sudden death cases and ∼50% of pump failure cases. Variables such as peak oxygen uptake (pVO2) and VE/VCO2 slope were also analysed. The main predictor of all-cause death was EOV, followed by VE/VCO2 slope and pVO2. It is interesting to note that these values were even more predictive of sudden death. Although cardiovascular remodelling is different in systolic and diastolic HF, Guazzi et al.17 found similar results between the aetiologies, i.e. a greater predictive value of EOV compared with pVO2 and VE/VCO2 slope. The presence of this marker was associated with a two-fold higher risk of fatal events than the second-best predictor. Furthermore, the prevalence of EOV was similar across HF aetiologies (HFrEF: 31% vs. preserved ejection fraction: 35%). These data confirm the high predictive value of EOV.
More recently, a study followed 89 patients with HF for 60 months.22 The authors found a better predictive ability of EOV compared with VE/VCO2 slope [HR 2.2 (1.2–4.1) vs. 1.1 (1.0–1.1)] when three criteria of the algorithm used to identify EOV were confirmed. After adjustment for age, pVO2, and VE/VCO2 slope, EOV was the only variable that remained associated with adverse cardiac events [HR 2.0 (1.1–3.7); P = 0.035]. Unlike the aforementioned studies that investigated the clinical impact of EOV in patients with HF, Cornelis et al.21 conducted a systematic review to assess the relationship between EOV and other prognostic variables. The authors found that patients with EOV had lower pVO2 [MD −2.86 mL kg min−1 (−3.17 to −2.54); P < 0.001] and higher VE/VCO2 slope [MD 5.89 (4.59–7.19); P < 0.001] than their counterparts without EOV.
A study from our group identified 968 (16.9%) cases of EOV among 5721 HF patients.3 We found a similar prevalence between the HF classifications studied (HFrEF: 17%; HFmiddlerangeEF: 16%), and as shown in other studies, patients with EOV had lower pVO2 and higher VE/VCO2 slope values than patients without EOV. In addition, patients with EOV were associated with more severe HF and worse prognosis than those without EOV. We also confirmed the strong prognostic power of EOV in HF and showed that this phenomenon is a predictor of mortality independent of the MECKI score, a very powerful prognostic tool for patients with HF.3
It is important to emphasize that the occurrence of EOV is gender specific, with a greater number of men compared to women, regardless of the severity of HF. For example, in the overall MECKI score population, EOV is present in 18% of male patients and 13% of female patients.23 There is currently no accepted explanation for this phenomenon. However, some sex differences are known, such as peripheral muscle CO2 production, apnoeic CO2 threshold, and chemoreflex response to partial pressure of oxygen and carbon dioxide. These characteristics are probably related to the effects of sex hormones and may lead to the observed sex differences.
Exercise oscillatory ventilation phenotypes (entire exercise vs. only at the beginning)
Although periodic breathing during exercise is usually defined as present or absent according to definitions, its temporal behaviour (disappearance or persistence) during a CPET also implies a different clinical, functional, and prognostic profile.24–26 Patients with EOV that persists during exercise have more advanced HF, lower left ventricular ejection fraction, and pVO2 and higher VE/VCO2 slope and MECKI score values than those with EOV that disappears with increasing exercise.25,26 In their landmark study, Schmid et al.24 showed that EOV persisted during exercise in 58% of patients and disappeared during exercise in 42% of patients. Of the patients with EOV that disappeared during exercise, 50% had an increase in VO2/work ratio after EOV resolution, 25% had a decrease, and 25% remained unchanged. Since EOV is associated with increased work of breathing, it is suggested that the increase in VO2/work ratio after the disappearance of EOV is related to reduced work of breathing.
Although currently unknown, understanding why EOV disappears during exercise and its consequences is of great importance. Ribeiro et al.5 have suggested that it may be due to an improvement in cardiac output or an increase in blood flow to the exercising muscles (skeletal and respiratory), both of which contribute to an increase in peripheral oxygen delivery, reducing metabo- and chemoreflex activation. On the other hand, the EOV persistence may be related to ergoreflex overactivity, which is mainly present in HF patients with skeletal myopathy and worse functional capacity and ventilatory response to exercise.27 Indeed, HF patients have significant skeletal muscle changes that result in excessive group III/IV afferent feedback (ergoreflex overactivity) during exercise, leading to lower ventilatory efficiency and a higher ventilatory overload.27 However, it must be emphasized that the above reports are hypotheses that need to be confirmed or rejected.
Treatment
As aforementioned, several studies have shown that EOV is a strong predictor of mortality and adverse outcomes, making treatment to reverse or mitigate its pathophysiology essential. Given the complexity of this phenomenon, several alternatives have been investigated as treatment options for patients with EOV. Protocols involving exercise training, drug administration, and ventilatory stimulation manoeuvres to stimulate ventilation have shown promising results. However, it must be emphasized that two different approaches are possible: (i) treatment of EOV as part of HF treatment and (ii) treatment of EOV per se.
A systematic review and meta-analysis of the effects of exercise training on metabolic and ventilatory changes in HF patients with EOV provided important information.28 The studies included aerobic training plus resistance training three times per week at different training volumes (intensity and duration). However, the protocols were performed according to current guidelines for cardiovascular rehabilitation in HF patients. Importantly, none of the trials reported adverse events, major complications, or loss to follow-up. The authors found that EOV was reversed in ∼70% of cases after 6 months of exercise training, in parallel with the improvement in aerobic power (pVO2) and ventilatory efficiency (VE/VCO2 slope).
The use of phosphodiesterase-5 (PDE5) inhibitors has been explored as a therapeutic option in the treatment of EOV due to the potential increase in alveolar pressure in response to pulmonary vasoconstriction. Thus, the PDE5 inhibitor would promote pulmonary vasodilation, improve oxygenation, reduce circulatory delay, and reverse EOV.15 A randomized clinical trial in 32 patients with EOV and pulmonary hypertension evaluated the effects of sildenafil (50 mg) administered three times daily.15 The authors found that the intervention led to an improvement in cardiac output, pVO2, ventilatory efficiency (VE/VCO2 slope), and EOV parameters, indicating a reversal of 87% of diagnosed cases at 6 months and 93% at 12 months.
Studies conducted by our group have investigated the effects of hyperventilation, through the addition of a ventilatory stimulus (CO2 inhalation and increased dead space) during exercise, on EOV. Firstly, Agostoni et al.12 showed that the addition of external dead space promotes the reversal of EOV in the early phase of exercise or prevents its appearance, depending on the amount of the dead space added (250 or 500 mL, respectively). The authors also observed that the addition of dead space was associated with poorer exercise performance, probably due to the increase in work of breathing. Apostolo et al.29 showed that the addition of 2% CO2 to breathing promoted the immediate reversal of EOV. They also showed that this effect was dose dependent, as the addition of 1% CO2 was not able to promote the reversal of EOV. This finding seems to be related to the increase in the eupnoeic PCO2 threshold towards the apnoeic PCO2 threshold. This shift prevents any increase in VE from bringing the arterial PCO2 below the apnoeic threshold, which could initiate a pause in breathing with a subsequent rapid hyperventilatory response, setting up the vicious cycle of EOV.
Perspectives
Although EOV has been studied extensively over the past few decades, there are still gaps in our knowledge of its pathophysiology and treatment options that present many opportunities for researchers. For example, in contrast to studies that have promoted further increases in hyperventilation, few studies have consistently explored the mitigation of hyperventilation that results from improved oxygen delivery to working muscles that inhibits ergoreflex exacerbation due to metabolite accumulation. Furthermore, most studies have focused on the central components of EOV and little is known about the involvement of peripheral muscles and peripheral mechanisms of oxygen-related ventilation regulation.
In clinical practice, given the complexity of making a clinical diagnosis based on the availability of several definitions and the visual observation of the curves of the CPET variables, it is crucial to reach a consensus on the model to follow to define the presence and the characteristics of EOV. Moreover, the introduction and use of computerized tools to standardize and facilitate the assessment of this phenomenon may improve the clinical diagnosis of EOV, a very important prognostic marker in clinical practice.30,31
Funding
This research was supported by the Italian Ministry of Health-Ricerca Corrente to Centro Cardiologico Monzino IRCCS (CUP B43C24000090001).
Data availability
No new data were generated or analysed in support of this research.
References
Author notes
Conflict of interest: none declared.