-
PDF
- Split View
-
Views
-
Cite
Cite
Nazma F Ilahibaks, Zhiyong Lei, Joost P G Sluijter, Extracellular vesicles as vehicles for drug delivery to the heart, European Heart Journal, Volume 45, Issue 26, 7 July 2024, Pages 2273–2275, https://doi.org/10.1093/eurheartj/ehae099
- Share Icon Share
Traditional drug delivery methods in cardiovascular disease management encounter significant clinical challenges. Cell-derived nanoparticles, known as extracellular vesicles, emerge as a potentially innovative and effective vehicles for curative cardiac therapies.
Heart failure (HF) is a significant cause of morbidity and mortality, with a dire prognosis of 50% mortality within 4 years and a lack of specific curative treatments. While existing therapies can extend patients’ survival, they do not offer a cure, underscoring the need for new strategies that promote cardioprotection and repair. Among various emerging treatments, including cell-based, regenerative-, and gene intervention therapies via non-coding RNA targets, direct protein-based therapeutics emerge as a particularly promising avenue.1
Current treatments for cardiovascular diseases face significant drug delivery challenges, particularly in directly delivering functional repair molecules like proteins or RNA molecules into cells. Although recent preclinical research has introduced new therapeutic approaches and molecules, established drug delivery techniques encounter substantial difficulties in clinical settings. These include (i) effectively targeting diseased tissues and cells within specific organs, (ii) evading detection by the immune system, and (iii) ensuring effective cellular uptake and release of therapeutic agents at the right location inside cells. Such obstacles result in off-organ- and cell-targeting, the need for immunosuppressive strategies, and suboptimal treatment outcomes in cardiovascular care.2
The most clinically advanced delivery systems that can induce cardioprotective or reparative molecular mechanisms include miRNA therapeutics via anti-sense strategies, and adeno-associated virus (AAV), and lipid nanoparticle (LNP) delivery (Figure 1). AAVs, small virus-based vehicles, are preferred for their low pathogenicity and prolonged gene expression. Yet, they face challenges such as immune responses, high production cost, and limited genetic material capacity. Moreover, a limited gene transfer was observed in heart tissue, resulting in no functional benefit for HF patients, as observed by the CUPID 2 and SERCA-LVAD trial.3 Notably, AAVs can provoke the immune system to generate neutralizing antibodies,4 compromising treatment effectiveness and constraining their use for repeated doses. The latter is especially problematic for chronic diseases, where ongoing therapy is required.
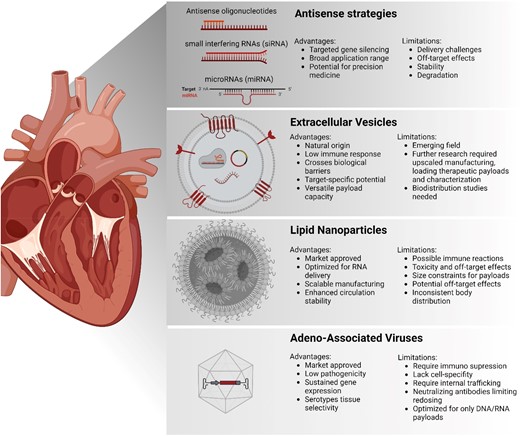
Overview of different cardiac delivery strategies. This diagram presents four primary methods for heart-targeted therapies, including anti-sense strategies, extracellular vesicles, lipid nanoparticles, and adeno-associated virus
Anti-sense strategies or LNP-based methods, having genetic material encapsulated in lipids as synthetic carriers, excel in encapsulating and delivering nucleic acids but face significant issues with toxicity and off-target effects. Safety concerns were raised following serious adverse events, including a fatality in a phase 1 CRISPR-based editor delivery trial,5 probably because of the need for high dosing.
Although careful dosing and the use of specific AAV serotypes that can offer tissue selectivity help to improve off-targeting, other systems are urgently needed for safer, more precise, and effective treatment options in cardiovascular patient care, paving the way for future advancements in this field.
Extracellular vesicles
The human body utilizes extracellular vesicles (EVs) for efficient and cell-specific intercellular communication. These cell-derived lipid bilayer-enclosed nanoparticles are key to maintaining tissue homeostasis. EVs represent a heterogeneous population of vesicles, including exosomes and micro-vesicles, and are released by almost all cell types.6 They transport RNAs, proteins, and lipids to target cells, aiding in cellular function and delivering warning signals. Interestingly, the paracrine effects previously observed in cardiac cell-based therapies were linked to the release of EVs by injected progenitor cells. However, scaling up EV production and identifying therapeutic molecules in progenitor-cell-derived EVs is challenging due to variability in donor cells and EV processing methods. This variability is particularly crucial in clinical scaling, as minor changes in processing can significantly affect EVs’ content and properties. Nonetheless, due to their natural functions and their effective intracellular routing, EVs hold great promise for drug delivery, extending beyond these current cell-free cardiac regeneration treatments (Figure 2).
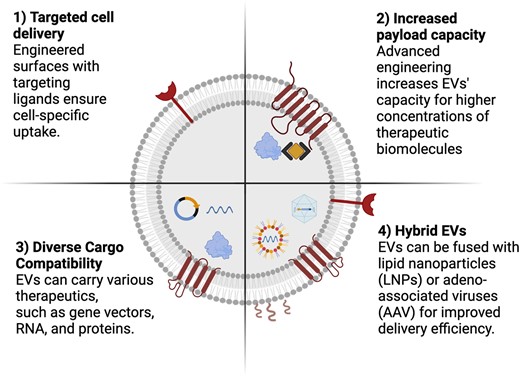
Enhanced engineering opportunities of extracellular vesicles as drug delivery systems. The development of extracellular vesicles as next-generation vehicles for targeted drug delivery could be achieved by further engineering their potential, including (i) cell-specific targeting through surface modification, enabling precise drug delivery to desired cells, (ii) optimized engineering techniques for loading therapeutic agents effectively into the EVs’ lumen, (iii) facilitation of intracellular delivery of diverse therapeutic agents, and (iv) the creation of novel hybrid EVs by combining them with lipid nanoparticles or adeno-associated viruses
EVs as drug delivery to the heart
The discovery of EVs as natural facilitators of cell-to-cell communication has led to their exploration as an innovative drug delivery platform. Traditional systems such as AAVs and LNPs often encounter immunogenicity and toxicity challenges. In contrast, EVs exhibit several ideal delivery characteristics: EVs have innate cell-targeting abilities, can traverse biological barriers like the blood–brain barrier, and carry various therapeutic payloads ranging from gene vectors to proteins. Their low immunogenic profile, particularly when derived from autologous cells, is also a significant advantage. EVs are thought to leverage natural cellular processes for precise delivery to target cells, for their efficient uptake, and especially their routing within cells. A direct in vitro comparison between EVs and their synthetic counterparts, LNPs, showed that EVs can function at a magnitude of scale more efficiently than LNPs.7 The potential of EV/LNP hybrids, which combines the advantages of both LNPs and EVs, offers improved efficacy and versatility for therapeutic use.8 Further research has probed EVs’ capabilities in delivering the CRISPR/Cas9 ribonucleoprotein complex, showing promise in ex vivo and preclinical models for conditions like cholesterol management and Duchenne Muscular Dystrophy.9,10
A significant advantage of EVs is their potential for low immunogenicity, which has been confirmed in various clinical trials where they were used for immunotherapy, demonstrating the feasibility of EV administration in humans. EVs’ potential in therapeutic drug delivery at a clinical scale depends heavily on overcoming specific technical challenges, including scalable isolation techniques, efficient drug loading inside the EVs, and further improving organ targeting. Understanding EV biology, primarily how EVs selectively target cells and the roles of different EV subtypes, is crucial for their development as effective drug carriers, particularly for cardiac diseases.
In summary, EVs present themselves as a potential alternative to traditional cardiovascular drug delivery methods such as AAVs and LNPs. Their natural origin, targeted delivery capabilities, and ability to overcome biological barriers underscore their potential in heart disease treatment. Their versatility is further demonstrated in advanced therapy delivery, including CRISPR/Cas9. Addressing challenges in EV isolation, drug loading, biodistribution, and understanding their biology more in detail is imperative for harnessing their full therapeutic potential. As research progresses, EVs potentially can transform cardiac drug delivery, offering safer and more effective treatment options. Future developments in this field are essential to fully realize their potential in combating cardiovascular diseases.
Declarations
Disclosure of Interest
N.I. is employed by Stichting Regenerative Medicine Crossing Borders (RegMed XB). Z.L. is affiliated with Nanocell Therapeutics. N.I., Z.L., and J.S. have jointly filed a patent related to an extracellular vesicle loading strategy, managed through the University Medical Center Utrecht. Funding for the present work was provided by EVICARE (725229) and TOP-EVICARE (101138069) of the European Research Council (ERC), NWO TTP HARVEY (2021/TTW/01038252), ZonMW PSIDER (10250022110004), NWO-Take-off 1 VINCI (2023/TTW/01494519), and Health Holland EV-Protect (TKI2306).