-
PDF
- Split View
-
Views
-
Cite
Cite
Matti T. Leppanen, Sandrine Sourisseau, Robert M. Burgess, Stuart L. Simpson, Paul Sibley, Michiel T.O. Jonker, Sediment Toxicity Tests: A Critical Review of Their use in Environmental Regulations, Environmental Toxicology and Chemistry, Volume 43, Issue 8, 1 August 2024, Pages 1697–1716, https://doi.org/10.1002/etc.5861
- Share Icon Share
Abstract
Sediments are an integral component of aquatic systems, linking multiple water uses, functions, and services. Contamination of sediments by chemicals is a worldwide problem, with many jurisdictions trying to prevent future pollution (prospective) and manage existing contamination (retrospective). The present review assesses the implementation of sediment toxicity testing in environmental regulations globally. Currently, the incorporation of sediment toxicity testing in regulations is most common in the European Union (EU), North America, and Australasian regions, with some expansion in Asia and non‐EU Europe. Employing sediment toxicity testing in prospective assessments (i.e., before chemicals are allowed on the market) is most advanced and harmonized with pesticides. In the retrospective assessment of environmental risks (i.e., chemicals already contaminating sediments), regulatory sediment toxicity testing practices are applied inconsistently on the global scale. International harmonization of sediment toxicity tests is considered an asset and has been successful through the widespread adoption and deployment of Organisation for Economic Co‐operation and Development guidelines. On the other hand, retrospective sediment assessments benefit from incorporating regional species and protocols. Currently used toxicity testing species are diverse, with temperate species being applied most often, whereas test protocols are insufficiently flexible to appropriately address the range of environmental contaminants, including nanomaterials, highly hydrophobic contaminants, and ionized chemicals. The ever‐increasing and ‐changing pressures placed on aquatic resources are a challenge for protection and management efforts, calling for continuous sediment toxicity test method improvement to insure effective use in regulatory frameworks. Future developments should focus on including more subtle and specific toxicity endpoints (e.g., incorporating bioavailability‐based in vitro tests) and genomic techniques, extending sediment toxicity testing from single to multispecies approaches, and providing a better link with ecological protection goals. Environ Toxicol Chem 2024;43:1697–1716. © 2024 The Authors. Environmental Toxicology and Chemistry published by Wiley Periodicals LLC on behalf of SETAC.
INTRODUCTION
Sediments are important sinks for many chemicals, including organic and inorganic contaminants, nutrients, and trace elements, and play a vital role in the transport, fate, and bioavailability of chemicals in aquatic ecosystems (Bashir et al., 2020; Perelo, 2010; Song & Müller, 1999). Sedimentary chemical deposits can function as reservoirs for the reemergence of historical contamination (e.g., phased‐out chemicals like polychlorinated biphenyls [PCBs]) as well as more contemporary pollutants (e.g., new replacement per‐ and polyfluoroalkyl substances [PFAS]) following environmental perturbations (Chiaia‐Hernandez et al., 2022). In addition, sediment‐associated contamination can have prolonged, detrimental effects on benthic and pelagic organisms and communities. For instance, elevated sedimentary concentrations of PCBs and polycyclic aromatic hydrocarbons (PAHs) have been linked to decreased benthic invertebrate abundance (Kadokami et al., 2013); heavy metal contamination in sediments has been shown to adversely affect various aspects of riverine ecosystems, including fish populations (Affandi & Ishak, 2019); and pesticide residues in sediments have been associated with reduced biodiversity and altered ecosystem functioning in benthic macroinvertebrates (Li et al., 2017). Hence, sediment contamination and any resulting adverse ecological effects may have broad negative environmental impacts and potentially high socioeconomic costs, including impact to commercially important species, drinking water supplies, and recreation use (Apitz et al., 2005; Bashir et al., 2020; Burton & Johnston, 2010).
In the broader context of evaluating increasing pressures on ecosystems, assessing ecological risks and defining management measures have become fundamental pillars of environmental regulation. The importance of protecting water resources and, more specifically, assessing the ecological risks posed by contaminated sediments is demonstrated by the many regulations existing worldwide. However, despite this increasing environmental awareness, regulatory frameworks, protective threshold values, and toxicity testing methodologies are challenging to deploy due to geographical specificities, sediment complexity, and special technical features or requirements related to a regulation. For example, authorities in different countries use different approaches to define concentration limits protective for benthic life, such as sediment quality criteria (SQC). In fact, nine different methodologies have been identified for deriving SQC (Clozel‐Leloup & Freyssinet, 2003), which can be grouped into three categories: geochemical, equilibrium, and empirical (ecotoxicological) methods. In the geochemical (sediment background) approach, maximum acceptable limits correspond to the geochemical background, whereas with the equilibrium (equilibrium partitioning and tissue residue) approaches, equilibrium partition coefficients are used to translate quality criteria for nonionic organic chemicals in water or biota (e.g., no‐ or acceptable‐effect concentrations) into sediment concentrations. Finally, with ecotoxicological approaches (e.g., spiked‐sediment bioassay, screening level concentration, sediment quality triad, apparent effects threshold, weight of evidence (WoE), and ecological risk assessment) empirical links between biological effects and concentrations in sediments are established.
To determine actual sediment toxicity (e.g., when deriving SQC), experimental assays have been developed over the past 40 years. Typically, sediment toxicity tests are assays in which the biological response (e.g., survival, growth, or reproduction) of an aquatic organism is monitored upon controlled exposure to contaminated sediment. The standardization of sediment toxicity tests by entities like ASTM International, the European Food Safety Authority (EFSA), the International Organization for Standardization (ISO), the Organisation of Economic Co‐operation and Development (OECD), the US Environmental Protection Agency (USEPA), and Environment Canada has provided assays designed with repeatability, accuracy, and other quality assurance metrics, which supports their use in a range of ways within regulatory frameworks. For example, sediment toxicity tests are applied to screen for ecotoxicological effects in field sediments (retrospective risk assessment, applying whole‐sediment bioassays), to derive SQC, and for chemical or product safety assessments (prospective risk assessment, using spiked sediment toxicity tests). In the present review, generally, the assays will interchangeably be referred to as “sediment toxicity tests” or “bioassays,” regardless of whether they concern tests with spiked or field‐contaminated material. Depending on the specific application, certain tests can be applied internationally (e.g., those from OECD) but others only nationally.
In a period of increasing reliance on modeling contaminant exposure and potential toxicity, along with pressure to reduce animal testing, understanding the strengths and weaknesses of sediment toxicity testing in regulation is particularly important. However, a clear overview of when sediment toxicity tests need to be performed and guidance on which tests to use is lacking. In the present review, we critically survey the use of sediment toxicity testing in international environmental regulations, with the aim to identify where sediment toxicity testing is used effectively and where and how applications could be improved. In our review, a distinction is made between sediment toxicity testing aimed to protect sediments facing contamination by newer pollutants (prospective testing, i.e., for chemical or product safety assessments) and testing used to regulate historically contaminated sediments (retrospective assessments). Emphasis is on European, North American, and Australasia regulations; however, the limited relevant regulations from other parts of the world are also discussed.
CURRENT REGULATIONS FOR PROSPECTIVE SEDIMENT TOXICITY TESTING
Below, an overview of the various (inter)national regulations including sediment toxicity testing for prospective purposes is provided. A summary of these regulations is given in Table 1. In addition, Supporting Information, Table S1, compiles specific sediment toxicity testing methods used globally.
Environmental regulations and applications involving the performance of sediment toxicity testing as part of prospective decision‐making processes
Regulation | Guidance documents | Specific application | Guidance for using sediment toxicity testing |
Europe | |||
Registration, Evaluation, Authorisation and Restriction of Chemicals (1907/2006) | European Commission (2006) | Safety of produced or imported chemicals | Sediment toxicity information is required for substances produced or imported in quantities ≥1000 t/year and based on substance properties |
Directive for placing plant production products on the market (1107/2009) with additional regulations (283/2013, 284/2013) | European Commission (2009a, 2013a, 2013b) | Safety of placing plant protection products on the market | The directive provides rules for governing plant protection products and the active substances contained in those products. Ecotoxicity, fate and behavior, and degradation tests in sediment are required. |
United States | |||
Clean Water Act | US Environmental Protection Agency (1985) | Derivation of aquatic life criteria | Currently, based exclusively on water‐only toxicity testing data without specific guidance for using sediment toxicity data to directly derive criteria. In rare instances, bioaccumulation data, potentially from sediment toxicity testing, can be used to back‐calculate water‐only concentrations. |
Federal Insecticide, Fungicide, and Rodenticide Act | US Environmental Protection Agency (2014) | Permitting of pesticides | A set of primary “triggers” are prescribed to guide an assessment of a pesticide under consideration for registration: (1) likelihood of exposure, (2) physicochemical properties, and (3) toxicological relevance. |
Canada | |||
New Substances Notification Regulation for Chemicals and Polymers | Canadian Environmental Protection Act (1999) | New substances notification and registration | Currently, based exclusively on water‐only toxicity testing data without specific requirements for using sediment toxicity testing. For substances known to partition into sediment, data from one toxicity test on a sediment‐dwelling organism may be requested as part of the assessment. |
Pest Control Products Act | Health Canada (Pest Management Regulatory Agency) | Pesticide registration | Currently, based exclusively on water‐only toxicity testing data without specific requirements for using sediment toxicity testing. For substances known to partition into sediment, data from one toxicity test on a sediment‐dwelling organism may be requested as part of the assessment. |
Australasia | |||
Industrial Chemicals Act 2019 | Australian Industrial Chemicals Introduction Scheme website (Australian Government, 2023) | Chemicals risk assessment (importing and manufacture) | Does not prescribe sediment toxicity testing and primarily uses toxicity data that already exist from other government programs and in the literature. |
Agricultural and Veterinary Chemicals (Administration) Act 1992 | Australian Pesticides and Veterinary Medicines Authority website | Permitting of pesticides | Does not prescribe sediment toxicity testing and primarily uses toxicity data that already exist from other government programs and in the literature. |
Hazardous Substances Assessments Amendment Act, 2022 | Environmental Protection Agency/Industrial Areas/Hazardous substances website | Permitting of hazardous substances, including pesticides | Does not prescribe sediment toxicity testing and primarily uses toxicity data that already exists from other government programs and in the literature. |
Regulation | Guidance documents | Specific application | Guidance for using sediment toxicity testing |
Europe | |||
Registration, Evaluation, Authorisation and Restriction of Chemicals (1907/2006) | European Commission (2006) | Safety of produced or imported chemicals | Sediment toxicity information is required for substances produced or imported in quantities ≥1000 t/year and based on substance properties |
Directive for placing plant production products on the market (1107/2009) with additional regulations (283/2013, 284/2013) | European Commission (2009a, 2013a, 2013b) | Safety of placing plant protection products on the market | The directive provides rules for governing plant protection products and the active substances contained in those products. Ecotoxicity, fate and behavior, and degradation tests in sediment are required. |
United States | |||
Clean Water Act | US Environmental Protection Agency (1985) | Derivation of aquatic life criteria | Currently, based exclusively on water‐only toxicity testing data without specific guidance for using sediment toxicity data to directly derive criteria. In rare instances, bioaccumulation data, potentially from sediment toxicity testing, can be used to back‐calculate water‐only concentrations. |
Federal Insecticide, Fungicide, and Rodenticide Act | US Environmental Protection Agency (2014) | Permitting of pesticides | A set of primary “triggers” are prescribed to guide an assessment of a pesticide under consideration for registration: (1) likelihood of exposure, (2) physicochemical properties, and (3) toxicological relevance. |
Canada | |||
New Substances Notification Regulation for Chemicals and Polymers | Canadian Environmental Protection Act (1999) | New substances notification and registration | Currently, based exclusively on water‐only toxicity testing data without specific requirements for using sediment toxicity testing. For substances known to partition into sediment, data from one toxicity test on a sediment‐dwelling organism may be requested as part of the assessment. |
Pest Control Products Act | Health Canada (Pest Management Regulatory Agency) | Pesticide registration | Currently, based exclusively on water‐only toxicity testing data without specific requirements for using sediment toxicity testing. For substances known to partition into sediment, data from one toxicity test on a sediment‐dwelling organism may be requested as part of the assessment. |
Australasia | |||
Industrial Chemicals Act 2019 | Australian Industrial Chemicals Introduction Scheme website (Australian Government, 2023) | Chemicals risk assessment (importing and manufacture) | Does not prescribe sediment toxicity testing and primarily uses toxicity data that already exist from other government programs and in the literature. |
Agricultural and Veterinary Chemicals (Administration) Act 1992 | Australian Pesticides and Veterinary Medicines Authority website | Permitting of pesticides | Does not prescribe sediment toxicity testing and primarily uses toxicity data that already exist from other government programs and in the literature. |
Hazardous Substances Assessments Amendment Act, 2022 | Environmental Protection Agency/Industrial Areas/Hazardous substances website | Permitting of hazardous substances, including pesticides | Does not prescribe sediment toxicity testing and primarily uses toxicity data that already exists from other government programs and in the literature. |
Environmental regulations and applications involving the performance of sediment toxicity testing as part of prospective decision‐making processes
Regulation | Guidance documents | Specific application | Guidance for using sediment toxicity testing |
Europe | |||
Registration, Evaluation, Authorisation and Restriction of Chemicals (1907/2006) | European Commission (2006) | Safety of produced or imported chemicals | Sediment toxicity information is required for substances produced or imported in quantities ≥1000 t/year and based on substance properties |
Directive for placing plant production products on the market (1107/2009) with additional regulations (283/2013, 284/2013) | European Commission (2009a, 2013a, 2013b) | Safety of placing plant protection products on the market | The directive provides rules for governing plant protection products and the active substances contained in those products. Ecotoxicity, fate and behavior, and degradation tests in sediment are required. |
United States | |||
Clean Water Act | US Environmental Protection Agency (1985) | Derivation of aquatic life criteria | Currently, based exclusively on water‐only toxicity testing data without specific guidance for using sediment toxicity data to directly derive criteria. In rare instances, bioaccumulation data, potentially from sediment toxicity testing, can be used to back‐calculate water‐only concentrations. |
Federal Insecticide, Fungicide, and Rodenticide Act | US Environmental Protection Agency (2014) | Permitting of pesticides | A set of primary “triggers” are prescribed to guide an assessment of a pesticide under consideration for registration: (1) likelihood of exposure, (2) physicochemical properties, and (3) toxicological relevance. |
Canada | |||
New Substances Notification Regulation for Chemicals and Polymers | Canadian Environmental Protection Act (1999) | New substances notification and registration | Currently, based exclusively on water‐only toxicity testing data without specific requirements for using sediment toxicity testing. For substances known to partition into sediment, data from one toxicity test on a sediment‐dwelling organism may be requested as part of the assessment. |
Pest Control Products Act | Health Canada (Pest Management Regulatory Agency) | Pesticide registration | Currently, based exclusively on water‐only toxicity testing data without specific requirements for using sediment toxicity testing. For substances known to partition into sediment, data from one toxicity test on a sediment‐dwelling organism may be requested as part of the assessment. |
Australasia | |||
Industrial Chemicals Act 2019 | Australian Industrial Chemicals Introduction Scheme website (Australian Government, 2023) | Chemicals risk assessment (importing and manufacture) | Does not prescribe sediment toxicity testing and primarily uses toxicity data that already exist from other government programs and in the literature. |
Agricultural and Veterinary Chemicals (Administration) Act 1992 | Australian Pesticides and Veterinary Medicines Authority website | Permitting of pesticides | Does not prescribe sediment toxicity testing and primarily uses toxicity data that already exist from other government programs and in the literature. |
Hazardous Substances Assessments Amendment Act, 2022 | Environmental Protection Agency/Industrial Areas/Hazardous substances website | Permitting of hazardous substances, including pesticides | Does not prescribe sediment toxicity testing and primarily uses toxicity data that already exists from other government programs and in the literature. |
Regulation | Guidance documents | Specific application | Guidance for using sediment toxicity testing |
Europe | |||
Registration, Evaluation, Authorisation and Restriction of Chemicals (1907/2006) | European Commission (2006) | Safety of produced or imported chemicals | Sediment toxicity information is required for substances produced or imported in quantities ≥1000 t/year and based on substance properties |
Directive for placing plant production products on the market (1107/2009) with additional regulations (283/2013, 284/2013) | European Commission (2009a, 2013a, 2013b) | Safety of placing plant protection products on the market | The directive provides rules for governing plant protection products and the active substances contained in those products. Ecotoxicity, fate and behavior, and degradation tests in sediment are required. |
United States | |||
Clean Water Act | US Environmental Protection Agency (1985) | Derivation of aquatic life criteria | Currently, based exclusively on water‐only toxicity testing data without specific guidance for using sediment toxicity data to directly derive criteria. In rare instances, bioaccumulation data, potentially from sediment toxicity testing, can be used to back‐calculate water‐only concentrations. |
Federal Insecticide, Fungicide, and Rodenticide Act | US Environmental Protection Agency (2014) | Permitting of pesticides | A set of primary “triggers” are prescribed to guide an assessment of a pesticide under consideration for registration: (1) likelihood of exposure, (2) physicochemical properties, and (3) toxicological relevance. |
Canada | |||
New Substances Notification Regulation for Chemicals and Polymers | Canadian Environmental Protection Act (1999) | New substances notification and registration | Currently, based exclusively on water‐only toxicity testing data without specific requirements for using sediment toxicity testing. For substances known to partition into sediment, data from one toxicity test on a sediment‐dwelling organism may be requested as part of the assessment. |
Pest Control Products Act | Health Canada (Pest Management Regulatory Agency) | Pesticide registration | Currently, based exclusively on water‐only toxicity testing data without specific requirements for using sediment toxicity testing. For substances known to partition into sediment, data from one toxicity test on a sediment‐dwelling organism may be requested as part of the assessment. |
Australasia | |||
Industrial Chemicals Act 2019 | Australian Industrial Chemicals Introduction Scheme website (Australian Government, 2023) | Chemicals risk assessment (importing and manufacture) | Does not prescribe sediment toxicity testing and primarily uses toxicity data that already exist from other government programs and in the literature. |
Agricultural and Veterinary Chemicals (Administration) Act 1992 | Australian Pesticides and Veterinary Medicines Authority website | Permitting of pesticides | Does not prescribe sediment toxicity testing and primarily uses toxicity data that already exist from other government programs and in the literature. |
Hazardous Substances Assessments Amendment Act, 2022 | Environmental Protection Agency/Industrial Areas/Hazardous substances website | Permitting of hazardous substances, including pesticides | Does not prescribe sediment toxicity testing and primarily uses toxicity data that already exists from other government programs and in the literature. |
European regulations
European regulations in which sediment toxicity testing is conditionally required as part of substance safety assessments or for registration purposes include the European Regulation (EC) 1907/2006 (European Commission, 2006) concerning the Registration, Evaluation, Authorisation and Restriction of Chemicals (REACH) and 1107/2009, which lays down procedures for authorization of plant protection products (PPPs; European Commission, 2009a).
The REACH regulation requires sediment toxicity information for substances produced or imported in quantities ≥1000 t/y (Annex X 9.5.1). An organic carbon–normalized sediment–water partition coefficient (log KOC) or octanol–water partition coefficient (log KOW) of ≥3 is generally used as a trigger value, although other considerations might also be important (e.g., non‐KOW/KOC‐driven binding to particles; European Chemicals Agency [ECHA], 2017; Hill et al., 1993). Registrants to REACH should choose the most appropriate testing protocol(s) based on substance properties (Tarazona et al., 2014). Test guidelines from the OECD are available for tests with midge larvae (Chironomus sp.; OECD, 2004a, 2010), oligochaetes (Lumbriculus sp.; OECD, 2007), and rooted plants (Myriophyllum spicatum; OECD, 2014; discussed below; Supporting Information, Table S1). Standardized tests from ASTM International, the USEPA, and the ISO are also available for other species, such as crustacean amphipods (Hyalella sp., Ampelisca sp., Gammarus sp.) and nematodes (Caenorhabditis elegans). Most of the ASTM International guidelines are designed to be short‐term tests (10‐day exposure) with mortality as the endpoint (ASTM International, 2023). Nevertheless, long‐term toxicity tests (28‐day exposure) with sublethal endpoints are recommended (e.g., Hyalella azteca, Chironomus sp., Leptocheirus plumulosus, Neanthes arenaceodentata). Polychaetes, amphipods, and mollusks (e.g., bivalves) are considered relevant test species for estuarine and marine environments. Test methods exist for Arenicola marina, Corophium volutator, Leptocheirus plumulosus, Amphiascus tenuramis, and early life stages of sea urchins or bivalves (e.g., ASTM International, 2023).
Data provided as part of the authorization process of PPPs on the European Union (EU) market must comply with requirements set out in EU Regulations 283/2013 and 284/2013 (European Commission, 2013a, 2013b). The potential persistence in water systems (bottom sediment and water, including suspended particles) and the extent to which water and sediment organisms are at risk should be assessed. Knowledge on the fate and behavior of the respective PPP in water and sediment (e.g., log KOC) is applied to describe probable contaminant distribution between water, suspended particles, and sediment, which subsequently allows quantifying sediment residues of concern and to which nontarget species are or may be exposed. All available biological effects data are then considered to characterize the ecotoxicity of a substance. This includes all potentially adverse effects found during routine ecotoxicological assessments including sediment toxicity testing. Where required by national authorities, additional studies should be carried out to investigate the toxic mechanisms involved. The scientific background of this prospective testing framework on ecological and ecotoxicological risk assessment for sediment‐dwelling organisms in edge‐of‐field surface waters has been reported by EFSA (EFSA Plant Protection Products Panel, 2015). In this guidance document, a tiered effect assessment procedure and proposals on how to link effects to exposure estimates are provided.
The ecotoxicological assessment of PPPs is based on the chronic risk the active substance poses to nontarget organisms. When accumulation of an active substance in sediment is indicated or predicted, the impact on a sediment‐dwelling organism should be assessed. Based on information provided by Maltby et al. (2005, 2009), EU plant protection regulations recommend Chironomus tests for contaminants with insecticidal activity. Effects on survival and development of Chironomus riparius (OECD, 2004b), including effects on emergence of adults, are measured to provide endpoints for substances considered to interfere with insect molting hormones or that have other effects on insect growth or development. Guideline 233 from the OECD describes the assessment methodology of lifelong exposure effects to Chironomus spp., fully covering the first generation and the early part of the second generation. Two OECD guidelines are available for testing either spiked water or spiked sediment (OECD 218 and 219, respectively; see OECD, 2004a, 2004b). For substances with fungicidal activity, Lumbriculus tests are recommended. A spiked‐sediment test for Lumbriculus variegatus is described in OECD guideline 225 (OECD, 2007). Lumbriculus spp. are exposed for 28 days, and effects on reproduction and biomass are scored. For PPPs known to partition to sediment and for which root uptake in plants is a potentially important route of exposure, the Aquatic Macrophyte Risk Assessment for Pesticides book (Maltby et al., 2010, 1360–1361) recommends that an additional Myriophyllum test should be carried out. In case the first‐tier risk assessment shows that monocot species are clearly more sensitive than dicot species and exposure via sediment is identified as an important exposure route for the compound being assessed, Glyceria may be a suitable test species.
To reduce testing with benthic organisms, the EFSA Plant Protection Products Panel (2015) has proposed the use of chronic toxicity data for pelagic organisms (e.g., Daphnia) and the equilibrium partitioning approach as an initial screening tool in the environmental risk assessment for PPPs. An extrapolation (safety) factor of 10 for benthic fauna should then be applied. However, because the predictive power of this approach has been tested for a limited number of compounds and assays only, its applicability needs to be further investigated.
To minimize the impact of offshore chemicals on the marine environment, the Oslo–Paris Convention (OSPAR) has adopted a harmonized mandatory control system for use and reduction of discharges of offshore chemicals by the oil and gas industry. OSPAR is working toward harmonizing its approach with the EU REACH Regulation and Biocidal Products Regulation (OSPAR, 2023). A concrete application relies on the Offshore Chemical Notification Scheme, which applies to chemicals intended for use and discharge during the exploration, exploitation, and associated offshore processing of petroleum in the United Kingdom and The Netherlands. Each chemical substance is assessed on a “case‐by‐case” basis. Providing sediment toxicity data is not mandatory but is required when a substance has at least one of the following properties: (1) it is a “sinker,” (2) it has a log KOW > 4 or a log KOC > 3, or (3) it contains surfactants. Corophium volutator is recommended as the test species, for which a standard testing protocol is available (OSPAR, 2006).
US regulations
Environmental regulations in the United States are based around environmental legislation often focused on specific media. One example is the Clean Water Act (CWA), which is designed to protect human and ecological health related to water resources. Other regulations address air, soils, and sediments. Prospective regulations involving the use of sediment toxicity testing are focused on permitting pesticides via the CWA; the Federal Insecticide, Fungicide, and Rodenticide Act; and, to a lesser extent, the derivation of aquatic life criteria (ALC).
Regulation of pesticides
Since 2007, sediment toxicity testing with benthic invertebrates has been conditionally required by the USEPA to support registration of conventional pesticides (USEPA, 2007a). Similar to the European PPP regulations, the conditions for requiring sediment toxicity testing mainly depend on the likelihood of pesticide exposure of benthic invertebrates, the physicochemical properties of the pesticide, and the toxicological relevance of expected pesticide exposure. Subchronic (10‐day) and chronic (life‐cycle) tests are required depending on the environmental persistence of the pesticide, typically using both freshwater and marine organisms. Guidelines for subchronic tests with freshwater and estuarine/marine invertebrates are contained in OCSPP 850.1735 (USEPA, 2016a) and 850.1740 (USEPA, 2016b), respectively, whereas guidelines for chronic testing are provided by USEPA (2000; freshwater invertebrates) and USEPA (2001; estuarine/marine invertebrates), with incorporation of more recent modifications to improve method performance (ASTM International, 2020).
Detailed guidance on the requirements and use of sediment toxicity tests in pesticide ecological risk assessments is described in USEPA (2014). The primary triggers for sediment toxicity testing are the likelihood of exposure and certain physicochemical properties. The likelihood of exposure of benthic invertebrates is evaluated qualitatively depending on the use pattern of the pesticide. Generally, outdoor pesticide applications are assumed to contribute to potential exposure of benthic invertebrates via drift and runoff, whereas most indoor applications are not. Exceptions for indoor applications may occur when pesticides are likely to be released into drains connected to municipal wastewater‐treatment plants and subsequent receiving water bodies (USEPA, 2016c). Next, based on physicochemical properties, the propensity of a pesticide to partition to sediment particles is assessed. Sediment toxicity testing is required if any of the following specific conditions are met: (1) sediment–water partition coefficient ≥50, (2) KOC ≥1000, or (3) log KOW ≥3. These criteria suggest the pesticide has an affinity for and will accumulate in sediment. Information on the pesticide's environmental persistence is used primarily for determining whether subchronic (10‐day) or chronic (28‐ to 65‐day) sediment toxicity tests are most appropriate. Specifically, if the aerobic soil or aquatic metabolism half‐life is ≤10 days, a subchronic sediment toxicity test is generally considered more appropriate. Conversely, if this half‐life is >10 days, chronic (28‐ to 65‐day) sediment toxicity tests are considered more appropriate. In the final risk assessment step, the likelihood that estimated environmental concentrations (EECs) in sediment would lead to risks for benthic invertebrates is specifically considered. This toxicological relevance step essentially involves comparing pesticide EECs in sediment or interstitial water (modeled or monitored concentrations) to effect concentrations for benthic invertebrates or pelagic invertebrates (as a surrogate for benthic invertebrates), that is, no‐observed–adverse effect concentrations (NOAECs). If an EEC exceeds the NOAEC in either porewater or sediment, a potential risk is identified. When available, monitoring data of pesticide concentrations in sediment may also be compared with sediment NOAECs as an additional line of evidence demonstrating potential risk to benthic invertebrates. In cases where available information indicates sediment test organisms are highly sensitive to a pesticide compared with available water column test organisms (e.g., chironomid vs. daphnid), porewater‐based NOAECs from sediment toxicity tests may be used to evaluate risks to pelagic invertebrates via water column exposure.
Derivation of ALC
Development of ALC is performed under the CWA. In the 1970s, concern for controlling water pollution led to enactment of the Federal Water Pollution Control Act Amendments of 1972 (Office of the Law Revision Council, 2018). As amended in 1977, this law became commonly known as the CWA (Office of the Law Revision Council, 2018). It governs pollution control and water quality of the US waterways, aiming to restore and maintain the chemical, physical, and biological integrity of the waters (Office of the Law Revision Council, 2018). The CWA establishes permitting requirements for the regulated discharge of pollutants into US waters under the National Pollution Discharge Elimination System (NPDES) and authorized the USEPA to implement pollution control programs, such as setting wastewater standards for industry and water quality standards for many of the contaminants in surface waters (USEPA, 2010). In addition, the NPDES aims to prevent the discharge of toxic substances in all national waterways. To achieve this goal, a series of water toxicity tests were developed to measure the presence of toxicity in relevant media (e.g., effluents, receiving waters). In some cases, if toxicity is detected and the source is unknown, a toxicity identification evaluation (TIE) should be performed to identify the cause and develop a remediation plan (USEPA, 1991, 1996). In contrast to toxicity testing, the ALC are numeric concentration‐based values designed to reflect the occurrence of acute and chronic toxicity to sensitive species, if exceeded. For several decades, the ALC have been derived based on a set of guidelines (USEPA, 1985, 2013) that provide rules for selecting the type and amount of data needed for acceptable criteria determination. The guidelines’ minimum data requirements are summarized in Supporting Information, Table S2, and involve a specific combination of acute and chronic toxicity data, acute–chronic ratios (ACRs), plant toxicity data, and bioaccumulation data for freshwater and marine species. Except for the bioaccumulation data, all this information is derived from water‐only toxicity tests. Sediment toxicity data are not explicitly required for the derivation of ALC. However, in some instances, bioaccumulation data from a sediment exposure can be used to back‐calculate concentrations in tissues associated with toxic effects of some organic chemicals, although this indirect application is unusual and rarely applied.
Recently, new tools for generating acceptable data for the derivation of ALC have been developed. Many of these involve extrapolation techniques, allowing the estimation of toxicity for one group of untested organisms based on actual data for tested organisms (see Cao et al., 2023; Fox et al., 2020; Raimondo & Barron, 2020). For example, the interspecies correlation estimation (ICE) calculates the acute toxicity for an organism without toxicological information from known toxicity data for a different species. While the ACR approach has been used for decades to estimate chronic data for ALC, ICE has been applied in a more limited capacity but was used very recently to derive ALC for several PFAS compounds. In addition, species sensitivity distributions (SSDs) have been used for decades to derive ALC; and, recently, the types of models and statistics used with the SSDs have advanced with improving software. The ICE focuses primarily on pelagic species, but as the techniques evolves, the possibility for use with sediment toxicity testing species is likely to increase. In contrast, SSDs have been used for years to present sediment toxicity data for multiple species.
Canadian regulations
In Canada, there is no requirement for sediment toxicity testing as part of the prospective assessment of new substances under the New Substances Notification Regulation for Chemicals and Polymers of the Canadian Environmental Protection Act (CEPA, 1999). However, when a substance is known to partition into sediment, data from one toxicity test on a sediment‐dwelling organism may be requested as part of the assessment. Such requests for sediment toxicity information apply only to a small subset of notified substances and, as such, have not been included as a requirement in the regulations (CEPA, 1999).
Australasian regulations
Australia and New Zealand have no specific requirements for sediment toxicity testing as part of a prospective assessment and approval for importing or manufacturing chemicals. However, there is usually a requirement for the provision of some aquatic toxicity data (Australian Government, 2023; Australian Pesticides and Veterinary Medicines Authority, 2023; New Zealand Environmental Protection Authority, 2023). Chemicals are evaluated for hazards using toxicity data already available by searching through other governmental programs (e.g., REACH) and in the literature, and chemicals that are yet to be evaluated are prioritized for more detailed risk assessment (Australian Government, 2023). Acceptable test guidelines for environment hazard characteristics and properties are based on standardized international toxicity test procedures (e.g., OECD, USEPA, EU REACH), but no Australian or New Zealand aquatic or sediment toxicity tests are specified, despite a range of standardized methods existing for relevant native organisms in both countries (Australian and New Zealand Governments and Australian State and Territory Governments, 2018b).
CURRENT REGULATIONS FOR RETROSPECTIVE SEDIMENT TOXICITY TESTING
The (inter)national regulations involving sediment toxicity testing (whole‐sediment bioassays) for retrospective assessments are discussed below. A summary of these regulations is provided in Table 2.
Environmental regulations and applications involving the performance of sediment toxicity testing as part of retrospective decision‐making processes
Regulation | Guidance documents | Specific application | Guidance for using sediment toxicity testing |
Europe | |||
Water Framework Directive (2000/60/EC) and the amendments (2008/105/EC, 2013/39/EU) | European Commission (2000b, 2008a, 2013c) | Good ecological and chemical status of all waters | There are no direct regulations for the use of bioassays in sediment risk assessment or environmental quality standards (EQSs) for sediment contaminants. The member states have a possibility to derive EQS values for sediments, and there is a guidance for these actions (European Commission, 2018). |
Marine Strategy Framework Directive (2008/56/EC) and the associated regulations (2017/845/EC, 2017/848/EU) | European Commission (2008b, 2017a, 2017b) | Good ecological and chemical status of marine waters | There are no direct regulations for the use of bioassays in sediment risk assessment or Environmental Quality Standards (EQSs) for sediment contaminants. The member states have a possibility to derive EQS values for marine sediments. and there is a guidance for these actions (European Commission, 2018). |
United States | |||
Clean Water Act; Marine Protection, Research, and Sanctuaries Act | US Environmental Protection Agency and US Army Corps of Engineers (1977, 1991, 1998) | Disposal of dredged materials | Formal tiered approach using sediment toxicity testing along with water‐only toxicity, comparison to aquatic life criteria, and bioaccumulation to determine if dredged materials can be ocean‐disposed. |
Comprehensive Environmental Response, Compensation, and Liability Act; Superfund Amendments and Reauthorization Act | US Environmental Protection Agency (1989, 1994a, 1994b, 2005a) | Remediation of contaminated sediment sites | Part of an ecological risk assessment including recommendations for performing acute and chronic sediment toxicity testing using the freshwater amphipod (Hyalella azteca) or midge (Chironomus tentans and Chironomus riparius) and/or marine amphipods (Rhepoxynius abronius, Eohaustorius estuarius, Ampelisca abdita, Grandidier lla japonica). |
Canada | |||
Federal Contaminated Sites Action Plan | Government of Canada (2012b) | Risk assessment of contaminated sediments | Technical guidance document intended to support federal custodians and risk‐assessment practitioners when conducting ecological risk assessments of contaminated federal soils and sediments. |
Canada–Ontario Agreement Respecting the Great Lakes Basin Ecosystem (COA) | Environment Canada and Ontario Ministry of Environment (2007) | COA decision‐making Framework for Assessment of Great Lakes Contaminated Sediment | Guidance document intended to support federal and provincial authorities and their practitioners when conducting ecological risk assessments of contaminated sediments in Canadian Great Lakes waters. |
Australia/New Zealand | |||
Water Quality Management Framework utilized by Australia (federal and state governments and New Zealand) | National Water Quality Management Strategy; Australian and New Zealand Governments and Australian State and Territory Governments (2018a); Simpson et al. (2013) | Risk assessment of contaminated environments, including sediments | Technical guidance document intended to support federal and state government regulators and risk‐assessment practitioners when conducting ecological risk assessments of contaminated sediments. |
National Assessment Guidelines for Dredging | Commonwealth of Australia (2009) | Risk assessment and approval of open‐sea disposal of dredged sediments | Assessment framework and guidance for the environmental impact assessment and permitting of the ocean disposal of dredged material. |
New Zealand; National Policy Statement for Freshwater Management | Freshwater National Policy Statement; New Zealand Ministry of Environment (2020) | Risk assessment of New Zealand freshwater environments, including sediments | The National Policy Statement for Freshwater Management is the main direction to local governments about how to manage freshwater in New Zealand. |
Regulation | Guidance documents | Specific application | Guidance for using sediment toxicity testing |
Europe | |||
Water Framework Directive (2000/60/EC) and the amendments (2008/105/EC, 2013/39/EU) | European Commission (2000b, 2008a, 2013c) | Good ecological and chemical status of all waters | There are no direct regulations for the use of bioassays in sediment risk assessment or environmental quality standards (EQSs) for sediment contaminants. The member states have a possibility to derive EQS values for sediments, and there is a guidance for these actions (European Commission, 2018). |
Marine Strategy Framework Directive (2008/56/EC) and the associated regulations (2017/845/EC, 2017/848/EU) | European Commission (2008b, 2017a, 2017b) | Good ecological and chemical status of marine waters | There are no direct regulations for the use of bioassays in sediment risk assessment or Environmental Quality Standards (EQSs) for sediment contaminants. The member states have a possibility to derive EQS values for marine sediments. and there is a guidance for these actions (European Commission, 2018). |
United States | |||
Clean Water Act; Marine Protection, Research, and Sanctuaries Act | US Environmental Protection Agency and US Army Corps of Engineers (1977, 1991, 1998) | Disposal of dredged materials | Formal tiered approach using sediment toxicity testing along with water‐only toxicity, comparison to aquatic life criteria, and bioaccumulation to determine if dredged materials can be ocean‐disposed. |
Comprehensive Environmental Response, Compensation, and Liability Act; Superfund Amendments and Reauthorization Act | US Environmental Protection Agency (1989, 1994a, 1994b, 2005a) | Remediation of contaminated sediment sites | Part of an ecological risk assessment including recommendations for performing acute and chronic sediment toxicity testing using the freshwater amphipod (Hyalella azteca) or midge (Chironomus tentans and Chironomus riparius) and/or marine amphipods (Rhepoxynius abronius, Eohaustorius estuarius, Ampelisca abdita, Grandidier lla japonica). |
Canada | |||
Federal Contaminated Sites Action Plan | Government of Canada (2012b) | Risk assessment of contaminated sediments | Technical guidance document intended to support federal custodians and risk‐assessment practitioners when conducting ecological risk assessments of contaminated federal soils and sediments. |
Canada–Ontario Agreement Respecting the Great Lakes Basin Ecosystem (COA) | Environment Canada and Ontario Ministry of Environment (2007) | COA decision‐making Framework for Assessment of Great Lakes Contaminated Sediment | Guidance document intended to support federal and provincial authorities and their practitioners when conducting ecological risk assessments of contaminated sediments in Canadian Great Lakes waters. |
Australia/New Zealand | |||
Water Quality Management Framework utilized by Australia (federal and state governments and New Zealand) | National Water Quality Management Strategy; Australian and New Zealand Governments and Australian State and Territory Governments (2018a); Simpson et al. (2013) | Risk assessment of contaminated environments, including sediments | Technical guidance document intended to support federal and state government regulators and risk‐assessment practitioners when conducting ecological risk assessments of contaminated sediments. |
National Assessment Guidelines for Dredging | Commonwealth of Australia (2009) | Risk assessment and approval of open‐sea disposal of dredged sediments | Assessment framework and guidance for the environmental impact assessment and permitting of the ocean disposal of dredged material. |
New Zealand; National Policy Statement for Freshwater Management | Freshwater National Policy Statement; New Zealand Ministry of Environment (2020) | Risk assessment of New Zealand freshwater environments, including sediments | The National Policy Statement for Freshwater Management is the main direction to local governments about how to manage freshwater in New Zealand. |
Environmental regulations and applications involving the performance of sediment toxicity testing as part of retrospective decision‐making processes
Regulation | Guidance documents | Specific application | Guidance for using sediment toxicity testing |
Europe | |||
Water Framework Directive (2000/60/EC) and the amendments (2008/105/EC, 2013/39/EU) | European Commission (2000b, 2008a, 2013c) | Good ecological and chemical status of all waters | There are no direct regulations for the use of bioassays in sediment risk assessment or environmental quality standards (EQSs) for sediment contaminants. The member states have a possibility to derive EQS values for sediments, and there is a guidance for these actions (European Commission, 2018). |
Marine Strategy Framework Directive (2008/56/EC) and the associated regulations (2017/845/EC, 2017/848/EU) | European Commission (2008b, 2017a, 2017b) | Good ecological and chemical status of marine waters | There are no direct regulations for the use of bioassays in sediment risk assessment or Environmental Quality Standards (EQSs) for sediment contaminants. The member states have a possibility to derive EQS values for marine sediments. and there is a guidance for these actions (European Commission, 2018). |
United States | |||
Clean Water Act; Marine Protection, Research, and Sanctuaries Act | US Environmental Protection Agency and US Army Corps of Engineers (1977, 1991, 1998) | Disposal of dredged materials | Formal tiered approach using sediment toxicity testing along with water‐only toxicity, comparison to aquatic life criteria, and bioaccumulation to determine if dredged materials can be ocean‐disposed. |
Comprehensive Environmental Response, Compensation, and Liability Act; Superfund Amendments and Reauthorization Act | US Environmental Protection Agency (1989, 1994a, 1994b, 2005a) | Remediation of contaminated sediment sites | Part of an ecological risk assessment including recommendations for performing acute and chronic sediment toxicity testing using the freshwater amphipod (Hyalella azteca) or midge (Chironomus tentans and Chironomus riparius) and/or marine amphipods (Rhepoxynius abronius, Eohaustorius estuarius, Ampelisca abdita, Grandidier lla japonica). |
Canada | |||
Federal Contaminated Sites Action Plan | Government of Canada (2012b) | Risk assessment of contaminated sediments | Technical guidance document intended to support federal custodians and risk‐assessment practitioners when conducting ecological risk assessments of contaminated federal soils and sediments. |
Canada–Ontario Agreement Respecting the Great Lakes Basin Ecosystem (COA) | Environment Canada and Ontario Ministry of Environment (2007) | COA decision‐making Framework for Assessment of Great Lakes Contaminated Sediment | Guidance document intended to support federal and provincial authorities and their practitioners when conducting ecological risk assessments of contaminated sediments in Canadian Great Lakes waters. |
Australia/New Zealand | |||
Water Quality Management Framework utilized by Australia (federal and state governments and New Zealand) | National Water Quality Management Strategy; Australian and New Zealand Governments and Australian State and Territory Governments (2018a); Simpson et al. (2013) | Risk assessment of contaminated environments, including sediments | Technical guidance document intended to support federal and state government regulators and risk‐assessment practitioners when conducting ecological risk assessments of contaminated sediments. |
National Assessment Guidelines for Dredging | Commonwealth of Australia (2009) | Risk assessment and approval of open‐sea disposal of dredged sediments | Assessment framework and guidance for the environmental impact assessment and permitting of the ocean disposal of dredged material. |
New Zealand; National Policy Statement for Freshwater Management | Freshwater National Policy Statement; New Zealand Ministry of Environment (2020) | Risk assessment of New Zealand freshwater environments, including sediments | The National Policy Statement for Freshwater Management is the main direction to local governments about how to manage freshwater in New Zealand. |
Regulation | Guidance documents | Specific application | Guidance for using sediment toxicity testing |
Europe | |||
Water Framework Directive (2000/60/EC) and the amendments (2008/105/EC, 2013/39/EU) | European Commission (2000b, 2008a, 2013c) | Good ecological and chemical status of all waters | There are no direct regulations for the use of bioassays in sediment risk assessment or environmental quality standards (EQSs) for sediment contaminants. The member states have a possibility to derive EQS values for sediments, and there is a guidance for these actions (European Commission, 2018). |
Marine Strategy Framework Directive (2008/56/EC) and the associated regulations (2017/845/EC, 2017/848/EU) | European Commission (2008b, 2017a, 2017b) | Good ecological and chemical status of marine waters | There are no direct regulations for the use of bioassays in sediment risk assessment or Environmental Quality Standards (EQSs) for sediment contaminants. The member states have a possibility to derive EQS values for marine sediments. and there is a guidance for these actions (European Commission, 2018). |
United States | |||
Clean Water Act; Marine Protection, Research, and Sanctuaries Act | US Environmental Protection Agency and US Army Corps of Engineers (1977, 1991, 1998) | Disposal of dredged materials | Formal tiered approach using sediment toxicity testing along with water‐only toxicity, comparison to aquatic life criteria, and bioaccumulation to determine if dredged materials can be ocean‐disposed. |
Comprehensive Environmental Response, Compensation, and Liability Act; Superfund Amendments and Reauthorization Act | US Environmental Protection Agency (1989, 1994a, 1994b, 2005a) | Remediation of contaminated sediment sites | Part of an ecological risk assessment including recommendations for performing acute and chronic sediment toxicity testing using the freshwater amphipod (Hyalella azteca) or midge (Chironomus tentans and Chironomus riparius) and/or marine amphipods (Rhepoxynius abronius, Eohaustorius estuarius, Ampelisca abdita, Grandidier lla japonica). |
Canada | |||
Federal Contaminated Sites Action Plan | Government of Canada (2012b) | Risk assessment of contaminated sediments | Technical guidance document intended to support federal custodians and risk‐assessment practitioners when conducting ecological risk assessments of contaminated federal soils and sediments. |
Canada–Ontario Agreement Respecting the Great Lakes Basin Ecosystem (COA) | Environment Canada and Ontario Ministry of Environment (2007) | COA decision‐making Framework for Assessment of Great Lakes Contaminated Sediment | Guidance document intended to support federal and provincial authorities and their practitioners when conducting ecological risk assessments of contaminated sediments in Canadian Great Lakes waters. |
Australia/New Zealand | |||
Water Quality Management Framework utilized by Australia (federal and state governments and New Zealand) | National Water Quality Management Strategy; Australian and New Zealand Governments and Australian State and Territory Governments (2018a); Simpson et al. (2013) | Risk assessment of contaminated environments, including sediments | Technical guidance document intended to support federal and state government regulators and risk‐assessment practitioners when conducting ecological risk assessments of contaminated sediments. |
National Assessment Guidelines for Dredging | Commonwealth of Australia (2009) | Risk assessment and approval of open‐sea disposal of dredged sediments | Assessment framework and guidance for the environmental impact assessment and permitting of the ocean disposal of dredged material. |
New Zealand; National Policy Statement for Freshwater Management | Freshwater National Policy Statement; New Zealand Ministry of Environment (2020) | Risk assessment of New Zealand freshwater environments, including sediments | The National Policy Statement for Freshwater Management is the main direction to local governments about how to manage freshwater in New Zealand. |
European regulations
European regulations for retrospective sediment assessments include the Water Framework Directive (WFD), the Marine Strategy Framework Directive (MSFD) and Regional Sea Conventions, like OSPAR and the Helsinki Convention on the protection of the Baltic Sea (Helsinki Commission [HELCOM], 2021).
The WFD (European Commission, 2000b) is the primary legislation in the EU addressing the protection of the aquatic environment. Its main objective is to achieve a good ecological and chemical status of all waters. The physical–chemical quality of sediments is part of this status. Environmental quality standards (EQSs) are the main tools to assess the level of aquatic contamination. In the WFD, EQSs are defined following a methodology described in the Technical Guidance for Deriving Environmental Quality Standards (European Commission, 2018). The priority pollutant directive (European Commission, 2008a) and its amendment directive (European Commission, 2013c) specifically list EQSs for several contaminants in inland or other surface waters, but setting standards for sediments is optional and left to the member states. National‐level sediment EQSs can be established for priority substances with significant potential to accumulate in sediment. The derivation and application should then be communicated to the European Commission and the other member states. If member states decide to derive sediment EQSs for priority and other substances, effect concentrations (e.g., no‐observed‐effect concentration [NOEC], 10% effect concentration [EC10], EC50) obtained from bioassays using sediment‐inhabiting species is needed. Annex V of the WFD addresses the range of species that should be tested with regard to surface water, but the listing is limited and mentions only three major taxa (algae and/or macrophytes, Daphnia sp. or representative for marine waters, and fish). The WFD does not indicate which benthic species or tests should be used for sediment. Instead, the derivation of EQSs for the protection of freshwater and marine benthic organisms is described in the EU technical guidance document 27 (European Commission, 2018) and is based on ecotoxicity tests and equilibrium partitioning theory presented in the REACH legislation. It also adds a possibility to use field or mesocosm data. The results from chronic toxicity tests are preferred over those from acute assays because of a more realistic longer‐term exposure. The guidance document mentions that the sediment toxicity test standards from ASTM International, USEPA, and OECD could be applied for producing the data for the EQS derivation; and possible tests are listed in the guidance document's Appendix 1 (mainly OECD sediment tests). Also, the acceptable quality data do not have to conform to formal test guidelines; thus, other tests and species can be used as well. In addition to these standard approaches, passive samplers, Tenax extracts, and the acid‐volatile sulfide approach for metals are described for assessing porewater‐mediated bioavailability and for refining the exposure concentration.
The MSFD (European Commission, 2008b) is an equivalent directive to the WFD for marine waters, aiming to achieve and maintain a good environmental status (GES) in the marine environment. The qualitative descriptors for determining GES include contaminant concentrations in fish and other seafood at levels safe for human consumption. Member states should assess compliance with the EQSs set for the coastal waters (marine) in the WFD and may set threshold values (national EQSs) for other substances in a specific matrix, like sediment. There are no mandatory directive‐level guides or requirements for using sediment bioassays while assessing environmental status, but biological approaches are included as supplementary criteria to assess GES. Some member states evaluate compliance with the MSFD using several biomarkers in fish, mussels, birds, and benthic biota. When implementing the MSFD and determining GES of marine waters, member states are recommended to collaborate within each marine region or subregion, for example, by using Regional Sea Conventions. The Helsinki Commission (Baltic Marine Environment Protection Commission) is an example of a region‐specific action. For its GES assessment, the Baltic Sea Action Plan (HELCOM, 2021) uses concentrations of several core indicator contaminants measured in biota or sediment. Countries around the Baltic Sea have agreed with HELCOM on threshold values representing a GES (e.g., for copper and tributyltin in sediments). Some biological endpoints are also used as biomarkers (e.g., imposex, malformed amphipod embryos); but these are not formerly assessed (HELCOM, 2023), and specific whole‐sediment bioassays are not applied. On another multinational level, the Oslo–Paris (OSPAR) Convention (1992) promotes ecosystem approaches through the concept of ecological quality objectives (i.e., a large set of operational objectives and indicators to assess health of marine ecosystems), providing the impact assessment of found pressures. A two‐stage process is used that compares bioassay results to environmental assessment criteria and then against background (assessment) concentrations.
As discussed above, the chemical status assessment of the aquatic environment in the European Union is mainly based on concentrations of pollutants, and biological approaches are only voluntary. However, the European Commission has published guidance to enhance harmonization among member states in chemical monitoring of sediment and biota (European Commission, 2000b). This guidance document focusses on chemical monitoring but alsoly introduces complementary methods, stating “bioassays, biomarkers and other ecotoxicological tests are useful tools for the evaluation of the real state of sediment in which both known and unknown contaminants are present at concentrations sufficient to cause toxicity to the test organisms.” A triad approach (i.e., chemical, bioassays, ecology), TIE, and effect‐directed analysis (i.e., the identification of toxic, causative organic contaminants by a combination of sample fractionation, bioassays, and chemical analyses) are mentioned as useful approaches; but specific methods are not described. The triad approach is also presented in another recent EU document promoting integrated sediment management in the WFD implementation (European Union, 2022). Many of these approaches result from North American sediment assessment practices. The need for new approaches for chemical status assessment is acknowledged by the EU administration, and effect‐based methods (i.e., applying bioassays and biomarkers to detect effects of all active chemicals in a sample) are seen as one solution, for example, for identifying groups of chemicals characterized by a specific mode of action or effect. A specific working group has been established within the context of the common implementation strategy for the WFD, and a working draft for effect‐based monitoring has been published (European Union, 2021). The proposal addresses in vivo and in vitro methods for surface waters but acknowledges that a separate guidance document for sediment assessment “would be useful to help Member States identify a suitable battery of tests depending on the types of pressure, and to facilitate the assessment of the results.”
Dredging is a procedure performed in harbors, in inland waters, and close to shorelines to keep waterways open for navigation or aid construction works. Often, large sediment masses need to be relocated; this requires risk assessments of dredged material to identify suitable sites for disposal or reuse. Overarching EU regulations specifically concerning dredging do not exist, but several legislations related to nature conservation may influence dredging plans, including the Waste Directive (European Commission, 2008c), the MSFD (European Commission, 2008b), Natura 2000 area–related directives (European Commission, 1992, 2009b), and the WFD (European Commission, 2000a). In addition, international treaties like HELCOM, OSPAR, and the Barcelona Convention locally regulate and guide dredging and disposal. Nationally, many member states predominantly use contaminant threshold values for assessing the risks and relocation solutions for dredged material (Häkkinen et al., 2020). The use of bioassays appears to be very limited in Europe, but some guidelines and practices are in use (e.g., Italy, France, Germany) or have been part of regulations in the past (e.g., The Netherlands; Heise et al., 2020).
US regulations
Retrospective US regulations involving sediment toxicity testing apply to the disposal of dredged materials and remediation of contaminated sediment sites. Dredged materials are regulated via the CWA and the Marine Protection, Research, and Sanctuaries Act, whereas contaminated sediment sites are covered by the Superfund program, which is formally empowered by the Comprehensive Environmental Response, Compensation, and Liability Act and Superfund Amendments and Reauthorization Act.
Regulation of dredged materials
The goal of regulating dredged materials is to determine their suitability for ocean disposal versus some form of management (e.g., landfilling, incineration). It is important to note the dredging discussed here is “navigational dredging or dredging activities of essentially the same character as navigational dredging” (USEPA & US Army Corps of Engineers [USACE], 1998), in contrast to the remedial dredging that may occur under the Superfund program to remove contaminated sediments of concern to human or environmental health (see next section). The navigational dredging determination is based on chemical, physical, and biological evaluations as described in USEPA and USACE (1977, 1991, 1998). Figure 1 outlines the general tiered process for assessing whether dredged materials can be dealt with by ocean disposal. If in Tier 1 it is concluded that insufficient data are available to accurately assess the dredged material, a series of evaluations are performed in Tiers 2 to 4. Tier 2 emphasizes the measurement and modeling of dissolved and particulate contaminants for comparison to ALC and estimations of bioaccumulation potential, respectively. Along with chemical analysis of the dredged materials (targeting potentially over 100 organics and metals), grain size, total solids, and total organic carbon are also measured. Unique to the assessment of dredged materials is the performance of elutriate testing. The elutriate is intended to serve as a surrogate of dredged material mixing with ocean water during disposal. Following the Tier‐2 assessments, in Tiers 3 and 4 actual sediment toxicity testing and bioaccumulation studies are performed on the water column and dredged materials. To this end, a selection of organisms is provided in USEPA (1996) and USEPA & USACE (1998; Supporting Information, Table S3). Toxicity tests with the elutriates are generally performed in a dilution series design (e.g., 100%, 50%, 25%) for a range of durations and endpoints, depending on the organisms (e.g., 48 to 96 h, survival, growth). Toxicity tests on the dredged materials are whole‐sediment tests using primarily lethal endpoints (10‐day duration), although some may apply sublethal endpoints. For example, some progress in the development of sublethal endpoints has been made with the amphipods Hyalella azteca, Ampelisca abdita, and Leptocheirus plumulosus; the midges Chironomus dilutus and Chironomus riparius; the oligochaetes Tubifex tubifex and Lumbriculus variegatus; and the polychaete Neanthes arenaceodentata. For the bioaccumulation components in Tiers 3 and 4, Supporting Information, Table S3, provides a list of organisms. Like the toxicity testing, USEPA/USACE bioaccumulation methods are recommended for the studies (Supporting Information, Table S1). Once completed, bioaccumulated contaminants are compared with Food and Drug Administration action levels for poisonous and deleterious substances in fish and shellfish for human consumption. Action levels are available for methylmercury, several chlorinated pesticides, and PCBs. Finally, the toxicity testing and bioaccumulation data resulting from Tiers 2 to 4 are evaluated to make a decision about the suitability of the dredged material for ocean disposal or some form of management.
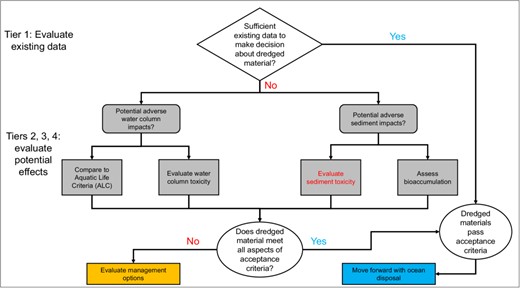
Simplified tiered process for determining possibilities for ocean disposal of dredged materials. Based on US Environmental Protection Agency and US Army Corps of Engineers (1991, 1998).
Regulation of Superfund sites
At contaminated sediment Superfund sites, sediment toxicity tests are generally applied as part of a broader ecological risk assessment for making decisions about remedial effectiveness (USEPA, 1989, 1994a, 1994b, 2005a). The current guidance documents provide background information on ecological risk assessment, toxicity testing, available toxicity testing methods, and remedial approaches. However, this guidance is not highly proscriptive and allows the Superfund site remedial project manager (RPM) a great deal of flexibility. The purposes of applying sediment toxicity testing at a Superfund site include to (1) demonstrate whether contaminants are bioavailable, (2) evaluate the aggregate toxic effects of all contaminants in the medium (i.e., sediment, soil), (3) assess the toxicity of substances whose biological effects may not have been well characterized, (4) frame the nature and distribution of a toxic effect at a site, (5) develop remedial goals, (6) perform monitoring, and (7) determine a site's postremediation potential to support a viable restored ecological community. Specific guidance provided to the RPM includes a discussion of the “elements in a toxicity assessment” (i.e., a description of variables to consider in applying toxicity testing at the site), including the toxicity assessment objectives, the medium (in this case sediment), reference sites, selection of test organisms and methods (e.g., static, static‐renewal, flow‐through), test site (field vs. laboratory), statistical design and analysis, and quality control/assurance. Again, while a range of options are described, no strict guidance is mandated.
In the 1990s, the National Oceanic and Atmospheric Administration published a tabulation of empirical sediment quality guidelines (SQGs) for a limited number of chemical contaminants routinely monitored at that time (Long & Morgan, 1990). These guidelines were based on the construct of relating sediment toxicity data to measured sediment contaminant concentrations, resulting in empirical causal relationships (see MacDonald et al., 2000; Nowell et al., 2016). Contaminants included conventional organics like PAHs, toxic metals, and current‐use pesticides. From these relationships, protective concentrations could be derived and used as SQGs (Batley & Simpson, 2013). In the early 2000s, the USEPA published mechanistic SQGs, called equilibrium partitioning sediment benchmarks (ESBs). These benchmarks were intended to provide sediment concentrations for a range of sediment contaminants protective to benthic organisms. Contaminants included the pesticides endrin and dieldrin, mixtures of PAHs and toxic metals, and several other hydrophobic organic contaminants (USEPA, 2003a, 2003b, 2003c, 2005b, 2008). The scientific foundations of the ESBs were based on the use of equilibrium partitioning (EqP) to estimate bioavailable concentrations of nonionic organic contaminants in sediment containing at least 0.2% of organic carbon (Burgess et al., 2013; Di Toro et al., 1991). This approach uses water‐only toxicity testing to estimate ecologically protective interstitial water concentrations. However, in some cases, when developing the ESBs, whole‐sediment toxicity testing was performed to “validate” the ESB values for selected contaminants. The empirical and mechanistic SQGs are not regulations but have been used at Superfund sites as part of ecological risk assessments. In addition, with the advent of passive sampling technologies, the need to use EqP to estimate bioavailable concentrations for the mechanistic SQGs has been replaced, in some cases, with passive sampling–based estimates of bioavailable concentrations (Burkhard et al., 2017; USEPA, 2012).
Canadian regulations
In Canada, protocols for the retrospective assessment of sediment quality and associated regulations fall under the jurisdiction of the federal government and provincial and territorial environmental ministries. Both entities establish standards and guidelines for use in conducting sediment‐based risk assessments and criteria for regulatory purposes. Environment and Climate Change Canada (ECCC), in cooperation with the Canadian Council of Ministers of the Environment (CCME), is the federal agency responsible for setting Canadian environmental and SQGs (CCME, 1995). In support of such regulations, ECCC has developed several standard reference toxicity test methods for both marine and freshwater sediment quality assessments (Government of Canada, 2023c). In the province of Ontario, SQGs are set and enforced by the Ontario Ministry of Environment Conservation, and Parks (OMECP). Some of the earliest SQGs in North America included those developed by the Ontario Ministry of the Environment (Ministry of the Environment, 1993, 1996). Since then, several frameworks and derivative approaches (e.g., sediment quality indices) have been developed for sediment risk‐assessment purposes (Environment Canada & Ontario Ministry of the Environment, 2007; Grapentine et al., 2002a, 2002b; Marvin et al., 2004; Ministry of the Environment, 2008). Most recently, under the 2002 Canada–Ontario Agreement Respecting the Great Lakes Basin Ecosystem (COA), the OMECP and ECCC developed a harmonized sediment assessment framework for evaluating contaminated sediments in the Great Lakes (Environment Canada & Ontario Ministry of the Environment, 2007). This framework capitalizes on the province's ecosystem approach to sediment assessment and applies a WoE metric based on information derived from four lines of evidence: sediment chemistry, toxicity, benthic community composition, and biomagnification potential. It further provides specific direction with respect to sediment management decisions. Procedurally, as described above for Superfund sites, potential sediment contamination is identified by comparing measured or modeled concentrations of substances in sediment to federal or provincial SQGs (PSQGs). The PSQGs constitute a set of numerical guidelines for the protection of sediment‐dwelling organisms and establish three levels of effect: no‐effect level, lowest‐effect level and severe‐effect level. When PSQGs are exceeded, additional biological assessments, such as sediment toxicity tests and other biological effects (e.g., assessment of benthic community composition, biomagnification potential), are evaluated as guided by the COA framework.
Areas of concern (AOCs) in Canada, first designated under the Great Lakes Water Quality Agreement in 1985 (Government of Canada, 2012a), are areas characterized by significant environmental degradation due to impairment of beneficial uses (there are 14 beneficial use impairment [BUI] categories, though only a subset of BUIs are typically listed for any one AOC). As directed through the participatory remedial action plans, removal of all designated BUIs in an AOC through remedial and restoration initiatives is needed for delisting. Many AOCs in Canada suffer from “degradation of benthos,” one of the designated BUIs, due to chemical contamination of the sediments. Sediment toxicity testing, therefore, is a key line of evidence needed for decision‐making with respect to delisting this type of BUI, as demonstrated in several recent studies (see Supporting Information, Supporting Text S1 for examples).
Wastewater systems effluent regulations are established under the Fisheries Act and include mandatory minimum effluent quality standards that can be achieved through secondary wastewater treatment. Requirements for monitoring, record‐keeping, reporting, and toxicity testing are specified in these regulations (Government of Canada, 2023a). Standardized biological test methods, like sediment toxicity testing, can be required to comply with wastewater regulations and are also used for chemical and ecological risk assessment (Chemicals Management Plan; Government of Canada, 2023b).
Australasian regulations
In the Australia and New Zealand guidelines, for fresh‐ and marine water quality (Australian and New Zealand Governments and Australian State and Territory Governments, 2018b), ecotoxicity testing on waters and sediments is recommended and utilized as a line of evidence when deriving management criteria and assessing environmental risks of chemical contaminants. The Water Quality Management Framework provides steps for planning and managing water or sediment quality and encompasses key requirements for long‐term management strategies to protect the community values of waterways (Australian and New Zealand Governments and Australian State and Territory Governments, 2018b; Australian Government, 2023; Australian Government Initiative, 2018): (1) good understanding of links between human activity and water/sediment quality; (2) clearly defined community values or uses, including the setting of management goals; (3) clearly identified and appropriate water/sediment quality objectives; and (4) adoption of cost‐effective strategies to achieve water/sediment quality objectives. Recently, for sediment quality assessments, updated sediment quality values for common chemical contaminants and a WoE framework were introduced (Simpson et al., 2013). The SQG values for metals were largely derived from the effects‐association database used by Long et al. (1995), while for organics, the threshold‐effects level and probable‐effects level values of MacDonald et al. (2000) were adopted as being more reliable. A range of sediment toxicity testing protocols are described with an increased focus on sublethal endpoints (Simpson & Batley, 2016). The most frequent applications have been for the evaluation of dredged sediments against Sea Dumping Permit criteria in accordance with the National Assessment Guidelines for Dredging (Commonwealth of Australia, 2009) and retrospective risk assessments with some examples setting remediation criteria (Simpson et al., 2020). More recently, guidelines have been established for contemporary pollutants such as perfluorooctane sulfonic acid (Simpson et al., 2021). Within both countries, a range of sediment toxicity test methods have been developed and standardized (e.g., by respective national agencies: Commonwealth Scientific and Industrial Research Organisation and National Institute of Water and Atmospheric Research; Supporting Information, Table S1) but not to the extent of the European Union, Canada, or the United States because of the limited usage.
CHALLENGES AND NEEDS
As discussed above for several regions, different national and international regulations and regulatory frameworks exist that require or imply a need for sediment toxicity testing as part of either prospective or retrospective assessments. Some frameworks are well developed and clearly define when and how testing should be performed, whereas others merely provide guidelines and allow for localized or assessment‐specific interpretation. In both cases, there is often room for improvements in the underlying regulations and/or at the level of the actual sediment toxicity tests. One overall challenge, irrespective of the geographical context, is finding an acceptable balance between environmental relevance and regulatory acceptance of sediment toxicity testing, while guaranteeing a robust risk characterization. Below, an overview is presented of more specific challenges and needs, grouped into different categories.
Current status and possibilities for improving existing regulations and protocols
The status of sediment toxicity tests varies between different jurisdictions. Within Europe, effects‐based sediment assessment practices vary substantially, and toxicity tests rarely are part of the decision‐making frameworks (Den Besten et al., 2003). More recently, progress made by including biological methods in national regulatory frameworks was evaluated during a workshop (SedNet, 2018), and Heise et al. (2020) noted that any progress had been modest or even regressive. They listed several stakeholder reservations that likely challenge the progress, including that (1) biotest results are much less precise and accurate than chemical data, (2) the low number of test organisms cannot represent the ecosystem sensitivity, (3) laboratory testing does not reflect natural conditions, (4) agreement on how to assess biotest data is lacking, and (5) biotesting significantly increases the costs of sediment management. Some of these reservations may be misconceptions, biased, or inaccurate, but overall, they indicate the need for an improved understanding of the sediment matrix and the “pros and cons” of sediment toxicity testing. Moreover, the usefulness of bioassays to complement chemical analysis has been demonstrated in the scientific literature (see e.g., De Baat et al., 2019). Clearly, more discussions and interplay between science and policy are needed to increase acceptance and implementation of regional and international guidelines in legislations in different jurisdictions. The fact that contaminated sediments are only mentioned once in the recent EU Commission Zero Pollution Action Plan (European Commission, 2021) as being a historical pollution source does not facilitate these processes.
Guidelines and regulations require reliable and standardized methods (Supporting Information, Table S1) that need to be updated from time to time when the background science supporting the protocols has evolved. For example, ISO standards are regularly circulated among member countries who vote for confirmation or revision needs. On the other hand, OECD guidelines may remain unchanged for years, unless member countries or the OECD secretariat undertakes action. Although the process of updating or developing new OECD test guidelines is quite thorough, it is resource and time‐demanding. This guarantees the quality of the guidelines and resulting data, but it also hampers initiating an update or new development processes. Therefore, the OECD test guideline program secretariat has pleaded for an urgent need to increase national resources for test guideline development (OECD, 2023).
Other regions experience similar challenges. For example, in the United States, the USEPA and USACE sediment toxicity testing protocols are relatively “mature” with publication dates ranging from 1993 to 2007 (Supporting Information, Table S1). In addition, in the 21st century, there has been an effort to apply and/or adopt the OECD protocols in the United States along with the limitations discussed above. However, there is evidence that when new stressors are recognized, the OECD program will respond with necessary new or adapted protocols. For example, to address nanomaterials, adaptations for OECD toxicity testing protocols, including those for sediments, were considered to better address the unique exposure challenges of this class of contaminants (Petersen et al., 2015). Similar deliberations by the OECD for evaluating PFAS toxicity in sediments are probably needed in the near future.
International harmonization and expansion
Although some progress has been made, there continues to be a distinct need for more widespread harmonization with respect to international regulatory frameworks. For example, chemical safety testing guidelines and protocols performed under REACH and similar regulations in non‐EU jurisdictions are harmonized through OECD guidelines, which are developed under the Mutual Acceptance of Data (MAD) rule. As such, sediment toxicity tests performed using OECD guidelines and good laboratory practice are valid in all countries accepting the MAD. Different jurisdictions do select varying requirements for registration (e.g., test bundles), and it may therefore not be realistic to expect these approaches to become internationally harmonized, all the more so because OECD guidelines are for testing chemicals; and their use is strongly connected to market approval affected by regional policies. In contrast, for retrospective assessments, harmonization could be viable, although it may be challenging for different countries to agree on the exact approach and content of regulations. However, also for retrospective assessment, the local circumstances and policies dictate the need and selection of the standards. The governments are the regional key players that should advocate for accelerating the use of sediment toxicity tests. This could include increasing resources for toxicity test development, training of administration and laboratory personnel, publishing guidelines, and adding legal requirements for national legislation. This should stimulate the market for commercial laboratories and consultant activities as well as encourage the development of university education programs in the field of sediment toxicology.
While the extensive use of sediment toxicity testing in regulations is limited primarily to Europe, North America, and Australasia, some regulations similar to REACH are expanding into other countries and involve the potential for the application of sediment toxicity testing (see Figure 2). In many instances, this expansion has occurred relatively recently and is of a prospective nature. For example, in Asia, and specifically in South Korea, the first deadline for formal registration under Korea K‐REACH was on December 31, 2021. In Japan, the Chemical Substances Control Law of 1973 was amended in 2009 to minimize adverse effects of chemicals on the environment and ensure consistency between the Global Harmonized System of Classification and Labeling of Chemicals (GHS) and the 2006 Strategic Approach to International Chemicals Management. In 2009, Taiwan announced the draft Guidelines for Existing Chemical Substance Nomination and New Chemical Notification that is intended to implement and strengthen GHS introduction. Chemical management in China has moved toward a system in compliance with and supporting GHS. Also in 2009, China's Ministry of Environmental Protection proposed amending the “Measures for the Environmental Administration of New Chemical Substances” of 2003 and introduced a risk‐assessment regulation.
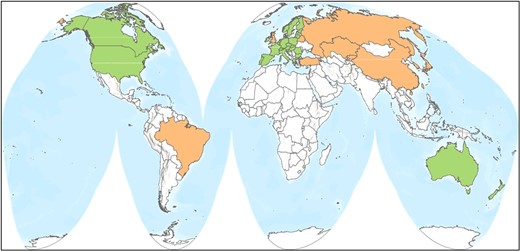
World map showing countries using sediment toxicity testing as part of their environmental regulations (green) and countries in which sediment toxicity testing is being considered (orange) for use in regulations (see text for specific country names). Countries without shading are currently not using sediment toxicity testing in their environmental regulations. Information in this figure reflects the authors’ understanding of the application of sediment toxicity testing at the time the manuscript was prepared and may change over time.
In non‐EU countries in Europe expansion is also occurring. For example, under the European Union (Withdrawal) Act 2018, the EU REACH Regulation was brought into UK law on January 1, 2021; and in Switzerland, a revision of the Swiss Chemicals Ordinance (ChemO, RS 813.11) came into force in 2009, which adopts REACH‐like registration requirements (new substances over 1 metric ton/y) (Swiss Federal Counsil, 2015). Norway has implemented the WFD with considerable attention to contaminated coastal sediments (Lehoux et al., 2020). The environmental administration has been active since the 1990s, and a set of guidelines has been developed for sediment management and remediation (see Miljødirektoratet, 2015). The contamination status is based on chemical analyses, complemented with three in vivo porewater bioassays and toxic unit analyses. In addition, if dioxin‐like effects are expected, sediment extracts can be tested with a DR‐CALUX in vitro assay; or if chronic effects should be evaluated, a whole‐sediment 10‐day test with an amphipod and a lungworm can be performed.
The Chemicals Management Regulation in Turkey also targets the adoption of REACH‐like regulations (substances imported or manufactured in 1–1000 t/year and >1000 t/year). The TR EAEU 041/2017 (Eurasian Union, 2017), sometimes called EURASIA REACH, established mandatory requirements for chemical substances as of June 2021 and applies to Russia, Kazakhstan, Belarus, Armenia, and Kyrgyzstan. All of these examples demonstrate the expansion of environmental regulations in global regions beyond the focus of our review (i.e., Europe, North America, and Australasia). However, the degree to which they include the application of sediment toxicity testing in these regulations is yet to be determined.
Interestingly, China has developed three whole‐sediment toxicity testing methods using chironomids and oligochaetes based on OECD guidance (Supporting Information, Table S1) and guidance documents for deriving and applying SQGs (Chinese Ministry of Ecology and Environment, 2023; Chinese Society of Environmental Sciences, 2002, 2020a, 2020b). In addition, sediment toxicity testing has been used with SQGs and SSDs for contaminated sediments in China (Wang et al., 2020; Zhang et al., 2017, 2022). In 2020, the Act for Environmental Management and Registration of New Chemicals (Act No. 12) issued by the Ministry of Ecology and Environment of the People's Republic of China incorporated sediment toxicity testing for the registration of new chemicals. Similar to the approach used by the USEPA, registration of pesticides, chemicals which are persistent, are bioaccumulative, and have a log KOC > 3 require sediment toxicity testing with benthic organisms.
Ecological and toxicological relevance of existing sediment toxicity tests
Because sediment toxicity testing generally aims to contribute to protecting key ecosystem functions provided by the sediment compartment, tests should represent field conditions and exposure routes and be applicable to all species inhabiting the sediments. These criteria are challenging because the number of available and appropriate test species are limited (i.e., either species generally used are easy to culture in the laboratory or their availability is guaranteed by a natural abundance or commercial suppliers; Wieringa et al., 2023), and the representativeness of these species for natural ecosystems is often questionable. However, covering all taxonomic groups when conducting toxicity testing is not feasible in practice. In prospective assessments, this problem is at least partly addressed by using either the most sensitive effect levels (e.g., EC10, NOEC) and assessment factors or SSD modeling and hazardous concentrations to protect 95% of species when deriving quality standards. Still, there is a need for developing standards for sediment toxicity testing that consider a more diverse selection of benthic species (Wieringa et al., 2023). As shown in Supporting Information Table S1, one approach to address this limitation is to test sediment interstitial waters and use pelagic organisms to expand the range of available organisms. However, this approach is not entirely satisfactory from the perspective of ecological relevance. Similarly, the geographical distribution of test species and guidelines currently seems to be limited to northern and southern hemisphere temperate species (Binet et al., 2018). Good progress has been made in testing tropical and other local species in surface water testing (see dos Santos et al., 2022; McKnight et al., 2023), but more international standards for southern hemisphere and tropical species and different environmental conditions need to be developed. Likewise, with the increasing exploration of deep‐seabed minerals, there is a need to assess risks of contaminants to abyssal benthic organisms, for which we have very limited understanding and currently rely on responses of “shallow” marine ecotoxicity data sets to infer adverse effects (Simpson & Spadaro, 2016; Stauber et al., 2022; Vare et al., 2018). Unfortunately, tests with deep‐sea species are very challenging, if not impossible.
In addition, testing conditions prescribed by standard guidelines sometimes substantially differ from environmental conditions. In such cases, using resident species may be a more appropriate option. This approach applies to both prospective (see Karjalainen et al., 2023) and retrospective (Jin et al., 2015) assessments and has the potential to provide direct information about toxic effects to local species or the respective region. Conversely, standardization of this approach is difficult and detailed, and reproducible test conditions (e.g., organism species identification, source, age, size, and test conditions) need to be reported for proper test interpretation and comparison. Also, using alternative species may increase the costs of conducting sediment toxicity tests.
Most of the standard sediment toxicity tests referred to or requested in regulations rely on scoring relatively simple endpoints (i.e., mortality and reproduction). To protect populations, communities, and ecosystems, rather than individuals, more subtle endpoints would be needed when deriving quality criteria or evaluating the risks of chemicals. For example, sublethal clinical signs as endpoints in fish acute toxicity tests (OECD, 2019a) can both support 3R principles in acute toxicity tests and offer an early warning connected to the viability of populations. However, more information on the significance of behavioral endpoints is still needed, especially in sediment toxicity testing. Fish tests are considered one of the main tools in surface water testing, but there are no fish standards for the sediment matrix (Supporting Information, Table S1). The zebrafish embryo test conducted in sediment exposures could correct this deficiency (see Kosmehl et al., 2006) and become an annex to the OECD 236 standard (OECD, 2013), and while fewer juvenile fish life stages are truly benthic, there are some that are ecologically relevant. Furthermore, whereas effect concentrations are generally based on either nominal or measured concentrations of the added chemicals in sediment or water, chemicals may degrade, microbiologically or chemically, or get metabolized in the test organism. Toxicity may thus be caused by the parent compound and/or its degradation products. Generally, this is not taken into account in current prospective regulations, which may potentially lead to under‐ or overestimated risks of chemicals.
Chemical relevance and quality of existing sediment toxicity tests
Existing test protocols and OECD guidelines have been designed primarily for relatively “easy”‐to‐test chemicals which potentially dissolve in water. Their application to other chemical groups, such as ionized chemicals (e.g., PFAS), nanomaterials, very hydrophobic chemicals, and substances of unknown or variable composition, complex reaction products, and biological materials (UVCBs), may result in biased data because of the occurrence of experimental artifacts (Jonker & Diepens, 2024b). There is standard guidance for alleviating this challenge, but this only applies to aqueous‐phase testing (OECD, 2019b). Not all chemicals have similar fate in the sedimentary compartment, and it would be beneficial to have distinct guidelines in terms of (1) exposure quantification to assess uptake routes and bioavailable or bioaccessible fractions with passive sampling or profile sampling, (2) spiking technique and equilibrium times, and (3) data interpretation (Jonker & Diepens, 2024a, 2024b). For instance, dedicated regulations and protocols need to be developed for nanomaterials and micro‐/nanoplastics because their fate and exposure are driven by other chemical and physical processes and not simply dissolution (e.g., agglomeration, precipitation, dissolution, and/or transformation). Consequently, measuring the actual exposure concentration of nanomaterials in the testing system can be very complicated and not feasible using common procedures (Handy et al., 2012; Lowry et al., 2012; Petersen et al., 2015). As an example, dissolution and ion release from some metallic nanoparticles may cause actual exposure to involve both parent nanoparticles and the dissolved species (Liu & Hurt, 2010; Ma et al., 2013; Petersen et al., 2015). Sediment toxicity testing standards are quite general in describing spiking procedures. Spiking, homogenization, and equilibrium time strongly affect bioavailability and consequently toxicity (see Costello et al., 2019; Jonker & Diepens, 2024a). These practices should be standardized, providing more details and taking advantage of developments in sediment research (Brumbaugh et al., 2013) to allow both scientific acceptance and regulatory use. In addition, data interpretation is key for reliable conclusions and transparent reporting where statistics play a major role. This has been recently acknowledged by the ISO and OECD standardization bodies, and an update project of statistical guidance standards (ISO, 2006; OECD, 2006) has been planned.
Modeling sediment toxicity
Sediment toxicity testing is laborious and costly. At least for certain groups of chemicals in prospective settings, modeling sediment toxicity can be an attractive alternative. For instance, sediment toxicity predictions for nonionic organic chemicals can be made by linking aquatic toxicity through equilibrium partitioning calculations (Burgess et al., 2023) provided that the organic carbon content of the sediment is at least 0.2%. Despite its ease and low costs, this approach is not yet used to its fullest potential. The main reasons are the low acceptance by regulators and the lack of high‐quality data to validate existing quantitative structure–activity relationship models for sediment toxicity (ECHA, 2017). Also, modeling is challenging for ionized chemicals and complex mixtures (Burgess et al., 2023), and different spiking regimes or insufficient mixing may cause concentration gradients or heterogeneity, which challenge accurate modeling (Fischer et al., 2021). Therefore, further development and validation for sediment toxicity modeling, as well as improved regulatory acceptance, are needed to support the use of modeling approaches in sediment assessments.
FUTURE PERSPECTIVE
Sediments act as a repository for many persistent, bioaccumulative, and toxic chemicals and, in most cases, contain complex mixtures of these contaminants. Simple chemical analyses, targeting a select set of chemicals, may fail to deliver complete and accurate risk assessments. In addition, site‐specific bioavailability issues may be involved but not adequately addressed. Hence, effect‐based monitoring or sediment toxicity testing is needed because these potentially integrate effects of different chemicals (i.e., mixture toxicity), as well as contaminant bioavailability. However, the current standard suite of in vivo sediment toxicity tests discussed in our review mainly focus on nonspecific effects (e.g., survival, reproduction, emergence) and would need to be complemented with new, more specific approaches to identify chemical groups with specific modes of toxic action (e.g., endocrine effects). For instance, these could include standardized in vitro assays applying cell cultures (e.g., DR/ER/AR‐CALUX) dosed with sediment extracts. Extracts should be obtained such that bioavailability is taken into account (i.e., preferably by applying passive samplers as passive dosing systems). As discussed throughout our review, understanding the bioavailability of contaminants in sediments being regulated is increasingly appreciated as critical to promulgating effective regulations. For nonionic organic contaminants and many toxic metals, the freely dissolved concentration (Cfree) and free ion form, respectively, are recognized as good surrogates for the bioavailable concentration (Burgess et al., 2013, 2023; Di Toro et al., 1991; Mayer et al., 2014). For nonionic organic contaminants, equilibrium passive sampling has been developed for measuring the Cfree and, in a regulatory context, is being increasingly used at contaminated sediment Superfund sites in the United States as a metric for decision‐making (see Grundy et al., 2023). The development and standardization of passive sampling have advanced rapidly over the last 20 years (see Booij et al., 2016; Jonker et al., 2020; USEPA, Strategic Environmental Research and Development Program, & Environmental Security Technology Certification Program, 2017), while the standardization of dosing using passive sampling extracts requires more development. Investigations by Reichenberg et al. (2008) and Schmidt et al. (2017; and the papers cited within) illustrate the promise of the approach, but future development of the methodology is needed. Finally, the use of bioaccessibility based on a Tenex extraction (Lydy et al., 2015) in the regulation of contaminated sediment has not been pursued very extensively.
In vitro assay analyses can also suggest candidate chemical groups responsible for specific effects, further aiding chemical identification (i.e., effect‐directed analysis and TIE; Brack et al., 2009, 2016; USEPA, 2007b) and possible remedial actions, including source identification. Recently, effect‐based trigger (EBT) values have been suggested as a tool for effect‐based methods to differentiate between acceptable and unacceptable water quality (Escher et al., 2018). This approach is most advanced for estrogenicity in vitro assessments for water samples (e.g., surface waters, effluents), and the associated, methodology‐specific EBT values are based on endocrine effects in fish (see Brion et al., 2019). Likewise, sediment retrospective risk assessments would also benefit from a standardized passive sampling procedure, followed by standardized in vitro effect‐based testing with discriminative thresholds.
Finally, as noted above, it is generally accepted that understanding the adverse effects of contaminated sediments on benthic populations and communities would better capture negative effects on aquatic ecosystems compared with looking at responses of single species. This has been frequently discussed in the scientific literature (see Burton et al., 2002; Burton & Scott, 1992; Chapman et al., 2002; de Castro‐Català et al., 2016) but continues to be understudied because of logistical and economic challenges of developing data sets that effectively compare the individual organism responses to population and community effects. The relatively recent development of genomic technologies for measuring the presence of a wide range of species in a given sediment sample offers the opportunity to assess adverse impacts to entire benthic communities in an increasingly cost‐effective and comprehensive fashion, compared with the conventional time‐ and labor‐intensive counting‐based taxonomical approaches (Giroux et al., 2022, 2023). For example, Giroux et al. (2022) investigated the use of RNA to detect only living organisms in marine sediments. This technique avoided some of the artifacts associated with using only DNA, which can also reflect the remains of nonliving organisms present in sediments. This type of genomic‐based approach, and others, represents a promising example of the potential evolution of the way we view and apply sediment toxicity testing in the future.
Supporting Information
The Supporting Information is available on the Wiley Online Library at https://doi.org/10.1002/etc.5861.
Acknowledgments
The authors thank the following colleagues for their input on the present review: A. Dorn (Bayer, Germany); Z. Farmani (Evonik, Germany); J. Häkkinen (Finnish Environment Institute, Finland); Y. Zhang (Nankai University, Tianjin, China); and M. Elias, M. Lambert, D. Charters, S. Raimondo, and K. Sappington (USEPA). This review evolved from a workshop funded by CEFIC‐LRI, through project ECO43.
Disclaimer
The views expressed in this article are those of the authors and do not necessarily represent the views or policies of their employing institutes.
Author Contribution Statement
Matti T. Leppanen, Sandrine Sourisseau, Stuart L. Simpson, Paul Sibley: Writing–original draft. Robert M. Burgess: Writing–original draft; Writing–review & editing. Michiel T. O. Jonker: Conceptualization; Project administration; Writing–original draft; Writing–review & editing.
Data Availability Statement
This review does not use any numerical data. All information used during the production of this review is cited and can be found on the internet.